Abstract
The impact of sex dimorphism on chronic renal failure (CRF)-induced oxidative multiorgan damage and the effects of estradiol (E2) loss and E2 supplementation on the progress of CRF were studied. Sprague-Dawley rats underwent 5/6 nephrectomy (CRF), and a group of female rats had bilateral ovariectomy (OVX), while the sham-operated rats had no nephrectomy or OVX. Rats received either estradiol propionate (50 μg/kg/day) or vehicle for six weeks. Serum BUN levels were elevated in both male and female CRF groups treated with vehicle, while creatinine level was not significantly changed in the female CRF group. CRF-induced elevation in serum TNF-α of male rats was abolished when the animals were treated with E2, while OVX exaggerated TNF-α response. In OVX and male rats with CRF, E2 treatment reversed the malondialdehyde elevations in all the studied tissues (kidney, heart, lung, ileum, brain, liver, and gastrocnemius muscle), while depletion of glutathione in these tissues was prevented by E2 treatment. Similarly, increased levels of myeloperoxidase activity, lucigenin chemiluminescence, and collagen in most of the tissues were reversed by E2 treatment. The findings show that the extent of tissue injuries was relatively less in females, while ovariectomy exacerbated all the indices of oxidative injury. Moreover, the administration of E2, with its potent anti-oxidant and anti-inflammatory effects, markedly improved CRF-induced systemic inflammatory outcomes in both male and female rats by depressing tissue neutrophil infiltration and modulating the release of inflammatory cytokines.
INTRODUCTION
Chronic renal failure (CRF) is well known to be associated with oxidative stress, which promotes the production of reactive oxygen species (ROS) and cytokine release. In CRF, the imbalance between increased production of ROS and the limited or decreased antioxidant capacity results in a persistent formation of toxic lipid peroxidation products,Citation[1] with a subsequent risk of damage to organic molecules such as proteins, nucleic acids, and lipids, both in the vicinity of their origin and far from the site of their formation, contributing to the development of serious complications in CRF.Citation[2,3] Data obtained from many experimental models of renal disease in animals indicate that renal damage is tolerated differently by the two sexes, while several epidemiological studies indicate a sexual dimorphism in the progression of human renal disease.Citation[4] Although the incidence of chronic renal disease is lower in premenopausal women compared with age-matched men,Citation[5,6] the risk for development and progression of renal disease in women is increased with menopause,Citation[7,8] suggesting the important contribution of ovarian sex hormones in the regulation of renal function. There is substantial evidence that the sexual dimorphism in kidney aging, with females protected, is associated with beneficial vascular actionsCitation[9] and antioxidant properties of estrogens.Citation[10]
Estrogen, which is a fat-soluble hormone that can contribute to membrane fluidity by direct interactions with phospholipids, exerts potentially vasoprotective effects through modulation of pro-oxidant and anti-oxidant enzyme expression and activity.Citation[11,12] It has also been suggested that estrogen can suppress free radical-induced peroxidation chain reactions because of the similarity in structure to vitamin E, namely, the presence of the hydroxyl group in the phenolic A ring.Citation[13,14] Substantive data show that estrogen supplementation decreases oxidative damage and plays a major role in the protection of the heart,Citation[15] brain,Citation[16,17] liver,Citation[18] and skeletal muscleCitation[19] in different models of oxidant injury. We have previously reported that 17β-estradiol (E2) protects the liver and intestines against sepsis-induced damage,Citation[20] improves the healing of gastric and colonic injury,Citation[21] and ameliorates burn-induced liver and lung injury in rats.Citation[22] However, the effect of E2 treatment on the oxidative complications of CRF has not (to our knowledge) been previously addressed.
Based on the aforementioned observations, the aim of the present study was to determine the impact of sex dimorphism on CRF-induced oxidative multiorgan damage, and elucidate the effects of E2 loss in female rats and E2 supplementation in both sexes using biochemical and histopathological analysis.
MATERIALS AND METHODS
Animals
Both sexes of Sprague Dawley rats (200–250 g) were housed in a room at a mean temperature of 22 ± 2°C with a 12-hour light-dark cycle with free access to standard pellet chow and water. The study was approved by the Marmara University Animal Experiments Ethical Committee.
Surgery and Experimental Design
All surgical procedures were performed under ketamine anesthesia (100 mg/kg ketamine and 0.75 mg/kg chlorpromazine, intraperitoneally) using strict hemostasis and aseptic techniques. In order to induce CRF, male (n = 16) and female (n = 24) rats underwent 5/6 nephrectomy by surgical resection of the upper and lower thirds of the left kidney, followed by right nephrectomy, as described by Vaziri et al.Citation[23] During the same operation, a group of female rats (n = 16) had bilateral ovariectomy (OVX). In the sham-operated rats (n = 16), peritoneal cavity was exposed via a ventral abdominal incision during which both kidneys in all animals and the ovaries in females were gently manipulated.
In the CRF groups, rats were injected subcutaneously with either estradiol propionate (50 μg/kg/day in olive oil) or olive oil (1 mL/kg) for six weeks, while the sham-operated rats received injections of oil for the same period. Rats were decapitated at the end of six weeks, and blood was obtained for the determination of tumor necrosis factor (TNF)-α, blood urea nitrogen (BUN), and creatinine. Samples from kidney, heart, lung, brain, ileum, liver, and skeletal muscle (m. gastrocnemius) were taken for the measurement of tissue malondialdehyde and glutathione levels, myeloperoxidase activity, and reactive oxygen species. Additional tissue samples were placed in 10% formaldehyde for histological evaluation and for the determination of tissue collagen content as an index of tissue fibrotic activity.
Measurement of BUN, TNF-α, and Creatinine Levels
Blood urea nitrogenCitation[24] and serum creatinineCitation[25] concentrations were determined spectrophotometrically using an automated analyzer. Serum levels of TNF-α were quantified according to the manufacturer's instructions and guidelines using enzyme-linked immunosorbent assay (ELISA) kit specific for the previously mentioned rat cytokines (Biosource International, Nivelles, Belgium). These particular assay kits were selected because of their high degree of sensitivity, specificity, inter- and intra-assay precision, and small amount of serum sample required conducting the assay.
Malondialdehyde and Glutathione Assays
Tissue samples were homogenized with ice-cold 150 mM KCl for the determination of malondialdehyde (MDA) and glutathione (GSH) levels. The MDA levels were assayed for products of lipid peroxidation by monitoring thiobarbituric acid reactive substance formation, as described previously.Citation[26] Lipid peroxidation was expressed in terms of MDA equivalents using an extinction coefficient of 1.56 × 105 M−1 cm−1, and results were expressed as nmol MDA/g tissue. GSH measurements were performed using a modification of the Ellman procedure.Citation[27] Briefly, after centrifugation at 2,000 g for 10 min, 0.5 ml of supernatant was added to 2 ml of 0.3 mol/L Na2HPO4.2H2O solution. A 0.2 ml solution of dithiobisnitrobenzoate (0.4 mg/mL 1% sodium citrate) was added, and the absorbance at 412 nm was measured immediately after mixing. GSH levels were calculated using an extinction coefficient of 1.36 × 104 M−1 cm−1. Results were expressed in μmol GSH/g tissue.
Measurement of Tissue Myeloperoxidase Activity
The activity of tissue myeloperoxidase (MPO), an enzyme found predominantly in the azurophilic granules of polymorphonuclear leukocytes (PMN), correlates significantly with the number of PMN determined histochemically.Citation[28] Therefore, MPO activity is frequently utilized to estimate tissue PMN accumulation in inflamed tissues. MPO activity was measured in tissues in a procedure similar to that documented by Hillegas et al.Citation[29] Briefly, tissue samples were homogenized in 50 mM potassium phosphate buffer (PB, pH 6.0), and centrifuged at 41,400 g (10 min); pellets were suspended in 50 mM PB containing 0.5% hexadecyltrimethylammonium bromide (HETAB). After three freeze and thaw cycles, with sonication between cycles, the samples were centrifuged at 41,400 g for 10 min. Aliquots (0.3 ml) were added to 2.3 ml of reaction mixture containing 50 mM PB, o-dianisidine, and 20 mM H2O2 solution. One unit of enzyme activity was defined as the amount of MPO present that caused a change in absorbance measured at 460 nm for 3 min. MPO activity was expressed as U/g tissue.
Chemiluminescence (CL) Assay
To assess the role of reactive oxygen species in tissue damage, lucigenin chemiluminescence was measured as an indicator of O2−• formation. Lucigenin (bis-N-methylacridiniumnitrate) was obtained from Sigma (St Louis, Missouri, USA). Measurements were made at room temperature using Junior LB 9509 luminometer (EG&G Berthold, Germany). Specimens were put into vials containing PBS-HEPES buffer (0.5 M PBS containing 20 mM HEPES, pH 7.2). Counts were obtained at 1 min intervals and the results were given as the area under curve (AUC) for a counting period of 5 min. Counts was corrected for wet tissue weight (rlu/mg tissue).Citation[30]
Collagen Measurement
Tissue collagen was measured as a free radical-induced fibrosis marker. Tissue samples that were cut with a razor blade and fixed in 10% formalin were embedded in paraffin, and 15 μm-thick sections were obtained. The collagen content was determined according to the method published by Lopez de Leon and Rojkind,Citation[31] which is based on the selective binding of the dyes Sirius Red and Fast Green FCF to collagen and non-collagenous components, respectively. Both dyes were eluted readily and simultaneously using 0.1 N NaOH-methanol (1:1, v/v). Finally, the absorbances at 540 and 605 nm were used to determine the amount of collagen and protein, respectively.
Histological Analysis
For light microscopic investigations, tissue specimens from kidney, heart, lung, brain, ileum, liver, and gastrocnemius muscle were fixed in 10% formaldehyde, dehydrated in alcohol series, cleared in toluene, and embedded in paraffin. Paraffin sections (5 μm) were stained with hematoxylin and eosin (H&E) and examined under a photomicroscope (Olympus BH 2, Tokyo, Japan). All tissue sections were examined microscopically for the characterization of histopathological changes by an experienced histologist who was unaware of the treatment conditions.
Statistical Analysis
Statistical analysis was carried out using GraphPad Prism 4.0 (GraphPad Software, San Diego, California, USA). Each group consisted of eight animals. All data were expressed as means ± SEM. Groups of data were compared with an analysis of variance (ANOVA) followed by Turkey's multiple comparison tests. Values of p < 0.05 were regarded as significant.
RESULTS
Serum BUN and creatinine levels were assessed to evaluate the extent of chronic renal failure. BUN levels were elevated in both male (p < 0.001) and female (p < 0.05) CRF groups treated with vehicle, while ovariectomy caused an additional increase in BUN of female rats with CRF (p < 0.001; see ). Estradiol treatment did not alter BUN levels in male or female CRF groups. Serum creatinine level was significantly increased in the vehicle-treated male CRF group (p < 0.001), but it was not significantly changed in the corresponding female group. Estradiol treatment or OVX did not change creatinine levels in rats with CRF.
Table 1 Serum blood urea nitrogen (BUN), creatinine, and TNF-α levels in the experimental groups
In the vehicle-treated male rats with CRF, serum TNF-α level was significantly increased with respect to sham-operated control group (p < 0.01), but this CRF-induced elevation in TNF-α of male rats was abolished when the animals were treated with estradiol (p < 0.01). In female CRF rats, similar to male group, TNF-α level was also elevated (p < 0.05) when the animals were treated with vehicle. On the other hand, this increase in TNF-α was exaggerated in the female rats with OVX (p < 0.001) and reversed by E2 treatment back to the level of non-OVX group (p < 0.001; see ).
In ovariectomized female rats with CRF and in male CRF rats, MDA levels indicating enhanced lipid peroxidation were increased in all tissues except the gastrocnemius muscle (p < 0.05–0.001; see ). However, MDA levels in none of the tissues were changed in female rats with intact ovaries. Estradiol treatment, on the other hand, reversed the MDA elevations in all the studied tissues of both male and female CRF groups significantly (p < 0.05–0.001). Gastrocnemius muscle MDA levels that were not changed by CRF in either the female or male rats were further depressed with estradiol treatment (p < 0.05).
Figure 1. Malondialdehyde (MDA) levels in the kidney, heart, lung, brain, ileum, liver, and gastrocnemius muscle tissues. *p < 0.05, **p < 0.01, ***p < 0.001, compared to corresponding control group. +p < 0.05, ++p < 0.01, +++p < 0.001, compared to corresponding CRF group. &p < 0.05, &&p < 0.01, compared to ovariectomized (OVX) CRF group.
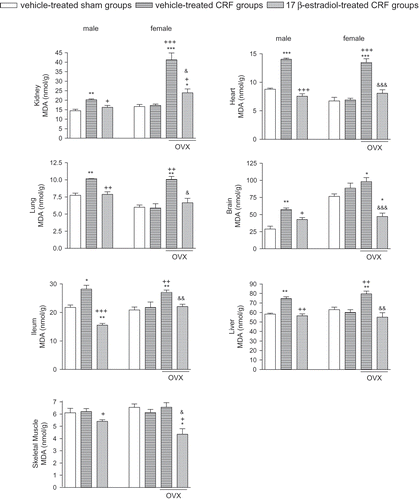
Chronic renal failure resulted in the depletion of antioxidant GSH in the kidney, brain, ileum, and liver of male and ovariectomized female rats (p < 0.05–0.001), while estradiol treatment in these groups increased tissue GSH contents back to control levels (p < 0.05–0.001; see ). Similarly, decreased GSH (p < 0.01) in the gastrocnemius muscle of male CRF rats was replenished back to control levels by E2 treatment (p < 0.01). However, skeletal muscle and cardiac GSH contents were not changed by CRF, OVX, or E2 in the female rats. In the lung and heart tissues of male CRF rats, GSH content was not changed with respect to control group, but was further elevated by estradiol treatment (p < 0.01). Likewise, reduced pulmonary GSH in the female CRF rats with OVX was elevated by E2 treatment (p < 0.01).
Figure 2. Gluthatione (GSH) levels in the kidney, heart, lung, brain, ileum, liver, and gastrocnemius muscle tissues. *p < 0.05, **p < 0.01, ***p < 0.001, compared to corresponding control group. +p < 0.05, ++p < 0.01, +++p < 0.001, compared to corresponding CRF group. &p < 0.05, &&p < 0.01, &&&p < 0.001, compared to ovariectomized (OVX) CRF group.
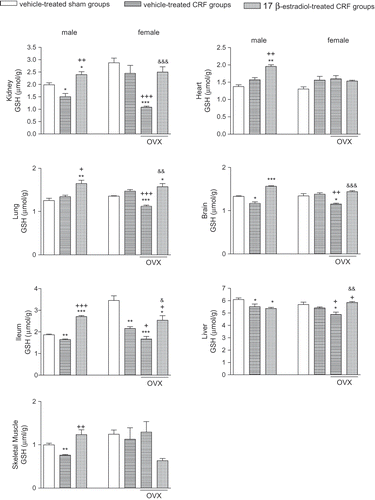
Myeloperoxidase activity representing the polymorphonuclear leukocyte accumulation in the target tissue was significantly elevated in all the studied tissues of male CRF rats (p < 0.05–0.001), while E2 treatment reduced MPO activity in all of these tissues except the gastrocnemius muscle (p < 0.05–0.001; see ). Similarly, increased MPO activity observed in all tissues of female CRF rats with OVX and in the muscle of CRF rats with intact ovaries was depressed by E2 (p < 0.01–0.001).
Figure 3. Myeloperoxidase (MPO) activity in the kidney, heart, lung, brain, ileum, liver, and gastrocnemius muscle tissues. *p < 0.05, **p < 0.01, ***p < 0.001, compared to corresponding control group. +p < 0.05, ++p < 0.01, +++p < 0.001, compared to corresponding CRF group. &&p < 0.01, &&&p < 0.001, compared to ovariectomized (OVX) CRF group.
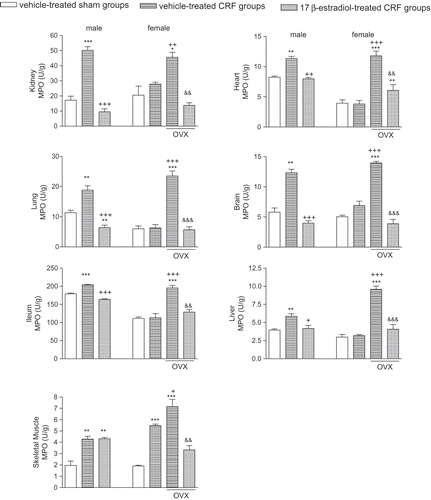
Lucigenin chemiluminescence (CL) was measured to elucidate the involvement of radical formation in CRF-induced tissue damage. Increased levels of lucigenin CL in the brain, muscle, liver, and ileum of female and male CRF groups (p < 0.05–0.001) were reversed by E2 treatment (p < 0.01–0.001; see ). Additionally, pulmonary CL level in male rats and renal CL in female rats, which were elevated by CRF, were also reversed by E2 treatment (p < 0.05–0.01). CRF has not altered CL levels in the kidney and heart tissues of male rats, while no significant change was noted in the cardiac and pulmonary tissues of female rats with CRF.
Figure 4. Lucigenin chemiluminescence levels as indicators of O2 formation in the kidney, heart, lung, brain, ileum, liver, and gastrocnemius muscle tissues. *p < 0.05, **p < 0.01, ***p < 0.001, compared to corresponding control group. +p < 0.05, ++p < 0.01, +++p < 0.001, compared to corresponding CRF group. &p < 0.05, &&p < 0.01, &&&p < 0.001, compared to ovariectomized (OVX) CRF group.
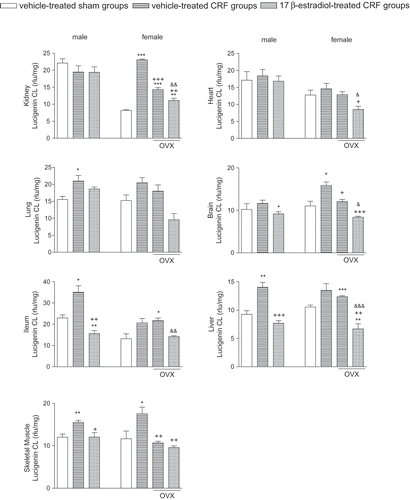
As an indicator of tissue fibrotic activity, collagen levels of all the studied tissues, except brain and muscle, were elevated in CRF groups of both sexes (p < 0.05–0.001), while this elevation in female rats was further increased in the ovariectomized group (p < 0.001; see ). On the other hand, the increments in tissue collagen levels were abolished in E2-treated groups (p < 0.05–0.001), except for the cardiac and ileal tissues of male and female rats, respectively.
Figure 5. Collagen measurements in the kidney, heart, lung, brain, ileum, liver, and gastrocnemius muscle tissues. *p < 0.05, **p < 0.01, ***p < 0.001, compared to corresponding control group. ++p < 0.01, +++p < 0.001, compared to corresponding CRF group. &p < 0.05, &&p < 0.01, &&&p < 0.001, compared to ovariectomized (OVX) CRF group.
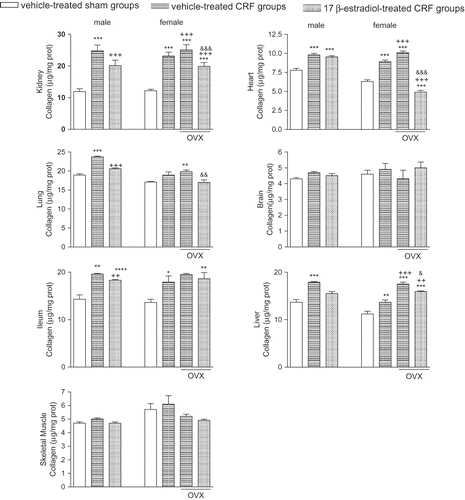
In CRF rats, histological analysis revealed degenerations in all of the observed tissues, while E2 treatment resulted in the amelioration of the damage that varied among the tissues. CRF induction devastated the kidney tissues, where the male rats (see , first row) were affected more than females (, first row). In female CRF rats with OVX that received vehicle treatment, the deterioration of tubules and interstitium was prominent (see , first row). The E2-treated male CRF group showed a reduction in the interstitial edema and tubular degeneration, but a mild congestion was still present (see , first row). The E2-treated CRF+OVX group presented similar morphology with the E2-treated male group with reduced edema and regenerated tubules (see , first row). Control cardiac muscle showed a regular alignment with fibers and capillaries (see , second row). CRF induction leading to severe congestion was more prominent in the male group (see , second row), but also present in the females as well (see , second row). In the OVX group with vehicle treatment, severe congestion and interstitial edema were present (see , second row), while in the E2-treated groups, cardiac muscle was settled to nearly normal organization with minor congestions (see and , second row). Similarly, in CRF-induced groups (see and , third row), lung tissue showed diffuse interstitial edema and congestion, while OVX resulted in severe edema and congestion (see , third row) with the fused alveoli that were dilated in some regions. E2-treated groups showed prominent reduction in both interstitial edema and congestion, and the alveoli structure was resettled (see and , third row). CRF in both male and female groups showed similar findings in the brain tissues with increased capillary structures and cellular dilations (see and , fourth row). In the vehicle-treated OVX group, a slight increase in glial cells and rare pyknotic nuclei were observed (see , fourth row). When treated with E2, a reduction in the density of capillaries was observed in the male rats (see , fourth row), while capillary density and cellular dilations were reduced in the female rats (see , fourth row). Control ileum with the villi and lamina propria showed regular morphology (see , fifth row), whereas the CRF groups presented degeneration of both epithelium and lamina propria that were more severe in male (see and , fifth row). In the OVX group with vehicle treatment, nearly half of the villi were desquamated and glandular deterioration was prominent (see , fifth row), while E2-treated groups showed the regeneration of villi and glandular structures (see and , fifth row). CRF led to a congestion and sinusoidal dilation in liver tissue (see and , sixth row), which was more intensive in males. In vehicle-treated OVX group, a diffuse congestion and severe sinusoidal dilation were observed (see , sixth row), while E2-treated groups presented a reduction in congestion and sinusoidal dilation (see and , sixth row). Skeletal muscle from CRF groups demonstrated an interstitial edema and congestion in perimysium (see and , last row), while in the OVX group without E2 treatment, edema was more apparent, and some detachments of the fibers were observed (see , last row). In the E2-treated groups, edema was reduced, and there were no detachments observed (see and , last row).
Figure 6. Kidney (first row): A. Control group: regular morphology with glomerulus (arrow) and tubules (*). B. CRF male group: deterioration in tubules (*) and glomeruli (arrows), interstitial edema. C. CRF female group: tubular (*) and glomerular (arrow) degeneration, intersitial edema (arrowhead). D. CRF + OVX + vehicle group: severe interstitial edema, degenerated glomeruli (arrows) and tubuli (*). E. CRF+ E2 male group: reduced but ongoing congestion (arrow); note the decreased interstitial edema. F. CRF + OVX+ E2 group: regeneration of the glomeruli (arrows) and tubuli (*); interstitial edema was decreased but still present (arrow head). Cardiac muscle (second row): A. Control group: regular alignment of cardiac muscle (arrow). B. CRF male group: severe congestion (**) among muscle fibers and edema of connective tissue (arrowhead). C. CRF female group: moderate congestion among muscle fibers (**). D. CRF + OVX + vehicle group: severe congestion among muscle fibers (arrows), interstitial edema. E. CRF + E2 male group: reduced congestion (**) and edema; note the regularity of fibers. F. CRF + OVX+ E2 group: minimal congestion among muscle fibers (**); note the loss of interstitial edema. Lung (third row): A. Control group: regular bronchial (arrow) and alveolar (*) morphology. B. CRF male group: dense edema and inflammation in interstitium (**), deteriorated alveoli (arrows). C. CRF female group: edema in parenchyme and alveolar degeneration (**) less severe than group B. D. CRF + OVX + vehicle group: severe interstial edema an inflammation (*) and constricted alveoli (a). E. CRF + E2 male group: regeneration of alveoli, moderate congestion and inflammation (**). F. CRF + OVX + E2 group: reconstitution of alveoli and mild congestion (**). Brain (third row): A. Control group: regular morphology with neurons and glial cells (arrow), scattered capillaries (*). B. CRF male group: increased density of capillaries (*), pericellular dilations (arrows). C. CRF female group: capillaries (*) and pericellular dilations present but less (arrow). D. CRF + OVX + vehicle group: increase in capillaries (*) and glial cells (arrows), E. CRF + E2 male group: reduction in capillar density (*) but pericellular dilations present (arrows). F. CRF + OVX + E2 group: reduction in capillar density (*) and pericellular dilations of neurons (arrowhead) and glial cells (arrows). Ileum (fourth row): A. Control group: regular ileal epithelium (arrows). B. CRF male group: severe desquamation of the villi (arrows) and inflammation in the lamina propria (arrowhead). C. CRF female group: moderate desquamation of the villi (arrow) and dilation of intestinal gland (arrowhead). D. CRF + OVX + vehicle group: severe desquamation of the villi (arrow) and dilation of the intestinal glands (*). E. CRF + E2 male group: regeneration of the villi morphology (arrows) and inflammatory cells in the lamina propria (arrowhead). F. CRF + OVX + E2 group: prominent regeneration of the epithelium (arrows) and some inflammatory cells in the lamina propria. Liver (fifth row): A. Control group: central vein (**) and radiating hepatic sinusoids. B. CRF male group: dilation of the central vein (**), congestion, interstitial edema (arrow) and degenerated sinusoids (arrowheads). C. CRF female group: minimal congestion in central vein (**) dilation of sinusoids (arrows). D. CRF + OVX + vehicle group: prominent congestion and dilation of sinusoids (arrows), interstitial edema (arrowhead), congestion of central vein(inset: **). E. CRF + E2 male group: reconstitution of central vein (**), mild congestion in sinusoids (arrows). F. CRF + OVX + E2 group: regenerated central vein (**) and sinusoid (arrows) morphology, mild inflammation at the periphery of hepatic canal. Skeletal muscle (last row): A. Control group: regular alignment of muscle fibers and connective tissue (*) among them. B. CRF male group: congestion among muscle fibers (*) and dilation of endomysium (arrowheads). C. CRF female group: minimal congestion (*) in the connective tissue, but moderate deterioration of general morphology. D. CRF + OVX + vehicle group: dilation in connective tissue (*);note detachments in the muscle fibers. E. CRF+ E2 male group: cross-section of muscle bundle; note the regular morphology (arrow) and minimal congestion (*). F. CRF + OVX + E2 group: ongoing congestion (*) among muscle fibers; note the regular alignment of muscle fibers (arrow). HE: ×200, inset: ×400.
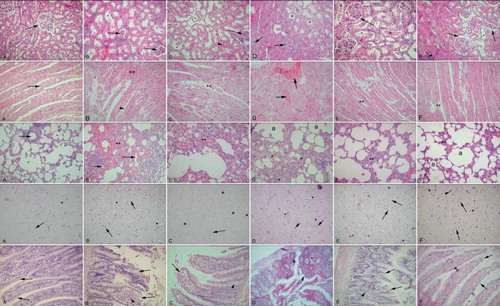
DISCUSSION
In the present study, surgically induced chronic renal failure, as assessed by increased serum creatinine and BUN levels, led to elevated serum TNF-α levels and significant increases in lipid peroxidation, collagen content, lucigenin levels, and myeloperoxidase activity along with decreased GSH levels in the kidney, as well as heart, lung, brain, ileum, liver, and gastrocnemius muscle, demonstrating the presence of systemic oxidative injury. On the other hand, the extent of tissue injuries and the renal dysfunction was relatively less in females, while ovariectomy exacerbated all the indices of oxidative injury. However, treatment with estradiol markedly improved oxidative damage in both male and female rats with CRF, suggesting the potent anti-oxidant and anti-inflammatory effects of estradiol against CRF-induced systemic inflammatory outcomes.
Similar to the sexual dimorphism observed in the progression of cardiovascular disease, clinical data suggest that premenopausal women are more resistant to and have slower progression of renal disease compared with age-matched men.Citation[32,33] The resistance of kidneys in female gender to the progression of renal disease is most frequently attributed to ovarian sex hormones.Citation[34] With menopause, however, the risk for renal disease is increased in women and becomes comparable to that found in men,Citation[35,36] suggesting that hormone replacement therapy would be a rational approach to attenuate the progression of renal disease in postmenopausal women. Several studies on acute renal injury have reported that females were relatively protected in comparison to males, have developed renal dysfunction in later periods of ischemic injury with lower BUN and creatinine levels, and had a lower mortality rate with a less severe tubular necrosis.Citation[37–39] Despite the abundance of clinical and animal studies demonstrating that estrogens are renoprotective, the mechanisms by which estrogens exert their protective effects on the kidney remain poorly understood.
Due to its hydroxyl group in the phenolic A ring, estrogen can suppress free radical-induced peroxidation chain reactionsCitation[13,14] and protect against atherosclerosis by binding free radicals.Citation[40] Being a fat-soluble hormone, estrogen can contribute to membrane fluidity by direct interactions with phospholipids and attenuate the peroxidation of vascular smooth muscle cells and peroxidation-induced cell growth and migration.Citation[41] On the other hand, age-related decline in estrogen secretion is accompanied by excessive oxidative stress and peroxidative cell damage,Citation[42] which are known features of CRF. The results of the present study show that lipid peroxidation products measured as MDA levels in kidney, heart, lung, intestine, brain, and liver were significantly increased in the male rats with CRF, while the unchanged tissue MDA levels in female rats were increased when ovaries were removed. Thus, the current results suggest that female gender is protective, but ovariectomy attenuates the protective effect of female gender on the development of CRF-induced oxidative injury. In addition, the administration of E2 caused significant inhibition of MDA production in both males and females, indicating a reduction in lipid peroxidation and cellular injury. In vitro, high concentrations of estradiol were shown to inhibit renal cell-mediated low-density lipoprotein oxidation.Citation[43] Accordingly, hormone replacement therapy was shown to have a favorable effect on oxidative stress in postmenopausal women with CRF, as evidenced by plasma MDA level and total antioxidant capacity.Citation[44] Similarly, female rats in experimental polycystic kidney disease exhibited normal renin status, lesser elevation of renal endothelin (ET-1), and proportionately lesser changes in vascular endothelial growth factor (VEGF) and endothelial nitric oxide synthase (eNOS), while ovariectomy was shown to attenuate the protective effect of female gender with a trend toward upregulation of ET-1 and a significant down-regulation of VEGF and eNOS.Citation[45] Furthermore, E2 supplementation was shown to be protective in the aging kidney by attenuating the downregulation of eNOS protein abundance and renal inflammation.Citation[46]
In uremia, toxic metabolites in blood activate PMN leukocytes, which then exhibit a burst of oxygen consumption,Citation[47] generating oxygen-derived reactive intermediates, superoxide anion, hydrogen peroxide, nitric oxide, and hypochlorous acid (HOCl).Citation[48] In the present study, in accordance with elevated tissue MDA levels, lucigenin chemiluminescence levels showed marked elevations in most of the studied target tissues of CRF rats, indicating the involvement of superoxide anion in associated tissue damages,Citation[30] while increased MPO activity clearly demonstrates that CRF-induced multiple organ damage is neutrophil-dependent. Activated neutrophils release MPO, causing production of large amounts of HOCl, which oxidizes and damages macromolecules, including proteins, lipids, carbohydrates, and nucleic acids.Citation[48] Our results are in agreement with similar studies showing that increased MPO activity plays a role in CRF-induced oxidative injury, and treating the animals with anti-inflammatory or antioxidant agents prevents tissue damage by inhibiting neutrophil infiltration.Citation[49,50] Moreover, the anti-inflammatory effect of E2 observed in all the CRF-injured tissues appears to include an inhibition on the accumulation of neutrophils and on the generation of superoxide radicals. Similarly, E2 was shown to inhibit neutrophil infiltration and ICAM-1 expression in endotoxin-induced glomerular inflammatory response.Citation[51]
Inflammatory diseases of the renal glomerulus and tubulointerstitium are characterized by destructive and restorative processes, mediated by cytokines, including TNF-α, that instruct target cells to alter their proliferation, differentiation, and migration.Citation[52] In chronically uremic patients, it was demonstrated that TNF-α gene expression is enhanced in circulating blood cells, suggesting the contribution of systemic inflammatory response in the complications of CRF.Citation[53] Accordingly, in another study, diminishing TNF-α expression significantly reduced ischemia-induced renal fibrosis and inflammation and improved renal functions.Citation[54] On the other hand, the administration of E2 depressed elevations in serum TNF-α levels following burn-injuryCitation[22] or trauma-hemorrhage.Citation[55] In addition, E2 inhibited the production of TNF-α by the unstimulated and hydrogen peroxide-stimulated macrophages from males and females.Citation[56] Parallel to these findings, the present data demonstrate that elevated serum TNF-α levels of CRF rats are depressed by exogenous E2 treatment, suggesting that E2-induced alleviation of CRF-induced oxidative damage involves the modulation of the synthesis of TNF-α.
To protect against the adverse effects of ROS, mammalian cells are equipped with various antioxidant scavengers. Apart from the major enzymatic antioxidants, such as superoxide dismutase, GSH peroxidase, and catalase, estrogens are also classified as nonenzymatic antioxidants that delay or inhibit age-associated pathological and biological changes.Citation[57] Several experimental studies have shown that antioxidant capacity of tissues is decreased in animals with CRF,Citation[58,59] while marked reductions in plasma glutathione concentration and glutathione peroxidase activity, as well as marked increases in the ratios of oxidized to reduced GSH were observed in CRF patients.Citation[60,61] Current findings show that the reduction in GSH content of the studied tissues of male and ovariectomized rats may be due to its consumption during CRF-induced oxidative stress. On the other hand, administration of E2, through its antioxidant properties and by inhibiting lipid peroxidation, maintained the glutathione levels and thereby protected the tissues against oxidative stress.
Estrogens influence the matrix deposition and scarring by stimulating the activity of collagen degrading enzymesCitation[62] and regulating the genes involved in extracellular matrix metabolism,Citation[63] thereby contributing to the progression of renal disease. In vitro studies confirm that estrogens inhibit the synthesis of type I and type IV collagen in renal tissue cultureCitation[64] via the suppression of transforming growth factor β (TGF-β) signal.Citation[65] Accordingly, in the TGF-β transgenic mouse, which develops extensive glomerulosclerosis, estradiol supplementation was shown to inhibit renal scarring.Citation[66] In parallel to these studies, current findings demonstrate that elevated collagen contents in the renal tissue as well as in the cardiac, pulmonary, ileal, and hepatic tissues of the male and female rats with CRF were reduced when the rats were treated with E2. Taken together, it appears that estradiol supplementation may delay the progression of chronic renal disease by limiting the oxidant-induced collagen accumulation.
A vast number of studies supporting the notion that systemic inflammation is activated during the progress of chronic renal failure provide a strong rationale for the use of anti-inflammatory treatments in uremia. The findings of the current study illustrate that exogenously administered estrogen acts as a potent antioxidant and reduces CRF-induced oxidative tissue damage by depressing tissue neutrophil infiltration and modulating the release of inflammatory cytokines. Extensive experimental and clinical studies will be necessary to further elucidate intracellular signals induced by estrogens on the target cells and thus to establish possibilities of therapeutic intervention in the progress to end-stage renal disease.
ACKNOWLEDGMENTS
The authors declare that there is no conflict of interest.
REFERENCES
- Cottone S, Lorito MC, Riccobene R, Nardi E, Mulè G, Buscemi S, Geraci C, Guarneri M, Arsena R, Cerasola G. Oxidative stress, inflammation and cardiovascular disease in chronic renal failure. J Nephrol. 2008;21:175–179.
- Siems W, Quast S, Carluccio F, Wiswedel I, Hirsch D, Augustin W, Hampi H, Riehle M, Sommerburg O. Oxidative stress in chronic renal failure as a cardiovascular risk factor. Clin Nephrol. 2002;58(Suppl. 1):S12–S19.
- Sener G, Paskaloğlu K, Satiroglu H, Alican I, Kaçmaz A, Sakarcan A. L-carnitine ameliorates oxidative damage due to chronic renal failure in rats. J Cardiovasc Pharmacol. 2004;43:698–705.
- Silbiger S, Neugarten J. Gender and human chronic renal disease. Gend Med. 2008;5(Suppl. A):S3–S10.
- Seliger SL, Davis C, Stehman-Breen C. Gender and the progression of renal disease. Curr Opin Nephrol Hypertens. 2001;10:219–225.
- Neugarten J. Gender and the progression of renal disease. J Am Soc Nephrol. 2002;13:2807–2809.
- Schulman IH, Aranda P, Raij L, Veronesi M, Aranda FJ, Martin R.. Surgical menopause increases salt sensitivity of blood pressure. Hypertension. 2006;47:1168–1174.
- Reckelhoff JF, Zhang H, Srivastava K, Granger JP. Gender differences in hypertension in spontaneously hypertensive rats: Role of androgens and androgen receptor. Hypertension. 1999;34:920–923.
- Baylis C. Sexual dimorphism of the aging kidney: Role of nitric oxide deficiency. Physiology (Bethesda). 2008;23:142–150.
- Irwin RW, Yao J, Hamilton RT, Cadenas E, Brinton RD, Nilsen J. Progesterone and estrogen regulate oxidative metabolism in brain mitochondria. Endocrinology. 2008;149:3167–3175.
- Gragasin FS, Xu Y, Arenas IA, Kainth N, Davidge ST. Estrogen reduces angiotensin II-induced nitric oxide synthase and NAD(P)H oxidase expression in endothelial cells. Arterioscler Thromb Vasc Biol. 2003;23:38–44.
- Strehlow K, Rotter S, Wassmann S, Adam O, Grohe C, Laufs K, Bohm M, Nickenig G. Modulation of antioxidant enzyme expression and function by estrogen. Circ Res. 2003;93:170–177.
- Sugioka K, Shimosegawa Y, Nakano M. Estrogens as natural antioxidants of membrane phospholipid peroxidation. FEBS Lett. 1987;210:37–39.
- Green PS, Gordon K, Simpkins JW. Phenolic A ring requirement for the neuroprotective effects of steroids. J Steroid Biochem Mol Biol. 1997;63:229–235.
- Zhai P, Eurell TE, Cotthaus R, Jeffery EH, Bahr JM, Gross DR. Effect of estrogen on global myocardial ischemia-reperfusion injury in female rats. Am J Physiol Heart Circ Physiol. 2000;279:H2766–H2775.
- Behl C. Oestrogen as a neuroprotective hormone. Nat Rev Neurosci. 2002;3:433–442.
- Feng Z, Zhang JT. Long-term melatonin or 17beta-estradiol supplementation alleviates oxidative stress in ovariectomized adult rats. Free Radic Biol Med. 2005;39:195–204.
- Lee MY, Jung SC, Lee JH, Han HJ. Estradiol-17beta protects against hypoxia-induced hepatocyte injury through ER-mediated upregulation of Bcl-2 as well as ER-independent antioxidant effects. Cell Res. 2008;18:491–499.
- Persky AM, Green PS, Stubley L, Howell CO, Zaulyanov L, Brazeau GA, Simpkins JW. Protective effect of estrogens against oxidative damage to heart and skeletal muscle in vivo and in vitro. Proc Soc Exp Biol Med. 2000;223:59–66.
- Sener G, Arbak S, Kurtaran P, Gedik N, Yeğen BC. Estrogen protects the liver and intestines against sepsis-induced injury in rats. J Surg Res. 2005;128(1):70–78.
- Günal O, Oktar BK, Ozçinar E, Sungur M, Arbak S, Yeğen B. Estradiol treatment ameliorates acetic acid-induced gastric and colonic injuries in rats. Inflammation. 2003;27:351–359.
- Ozveri ES, Bozkurt A, Haklar G, Cetinel S, Arbak S, Yeğen C, Yeğen BC. Estrogens ameliorate remote organ inflammation induced by burn injury in rats. Inflamm Res. 2001;50:585–591.
- Vaziri ND, Ni Z, Oveisi F, Liang K, Pandian R. Enhanced nitric oxide inactivation and protein nitration by reactive oxygen species in renal insufficiency. Hypertension 2002;39:135–141.
- Talke H, Schubert GE. Enzymatic urea determination in the blood and serum in the Warburg optical test. Wiener Klinische Wochenschrift. 1965;43:174–175.
- Slot C. Plasma creatinine determination: A new and specific Jaffe reaction method. Scand J Clin Lab Invest. 1965;17:381–387.
- Beuge JA, Aust SD. Microsomal lipid peroxidation. Methods in Enzymology. 1978;52:302–311.
- Beutler E. Glutathione. In Beutler E ( ed.). Red blood cell metabolism: A manual of biochemical methods. New York: Grune & Stratton; 1975:112–114.
- Bradley PP, Priebat DA, Christersen RD, Rothstein G. Measurement of cutaneous inflammation. Estimation of neutrophil content with an enzyme marker. J Invest Dermatol. 1982;78:206–209.
- Hillegass LM, Griswold DE, Brickson B, Albrightson-Winslow C. Assessment of myeloperoxidase activity in whole rat kidney. J Pharm Methods. 1990;24:285–295.
- Haklar G, Ulukaya-Durakbaşa C, Yüksel M, Daglı T, Yalcin AS. Oxygen radicals and nitric oxide in rat mesenteric ischemia-reperfusion: Modulation by L-arginine and N-nitro-L-arginine methyl ester. Clin Exp Pharmacol Physiol. 1998;25:908–912.
- Lopez De Leon A, Rojkind M. A simple micromethod for collagen and total protein determination in formalin-fixed parraffin-embedded sections. J Histochem Cytochem. 1985;33:737–743.
- Tozawa M, Iseki K, Iseki C, Kinjo K, Ikemiya Y, Takishita S. Blood pressure predicts risk of developing end-stage renal disease in men and women. Hypertension. 2003;41:1341–1345.
- Neugarten J. Gender and the progression of renal disease. J Am Soc Nephrol. 2002;13:2807–2809.
- Ball P, Emons G, Kayser H, Teichmann J. Metabolic clearance rates of catechol estrogens in rats. Endocrinology. 1983;113:1781–1783.
- Schulman IH, Aranda P, Raij L, Veronesi M, Aranda FJ, Martin R. Surgical menopause increases salt sensitivity of blood pressure. Hypertension. 2006;47:1168–1174.
- Reckelhoff JF, Zhang H, Srivastava K, Granger JP. Gender differences in hypertension in spontaneously hypertensive rats: Role of androgens and androgen receptor. Hypertension. 1999;34:920–923.
- Park KM, Kim JI, Ahn Y, Bonventre AJ, Bonventre JV. Testosterone is responsible for enhanced susceptibility of males to ischemic renal injury. J Biol Chem. 2004;279:52282–52292.
- Müller V, Losonczy G, Heemann U, Vannay A, Fekete A, Reusz G, Tulassay T, Szabó AJ. Sexual dimorphism in renal ischemia–reperfusion injury in rats: Possible role of endothelin. Kidney Int. 2002;62:1364–1371.
- Fekete A, Vannay A, Ver A, Sex differences in the alterations of Na(+), K(+)-ATPase following ischaemia–reperfusion injury in the rat kidney. J Physiol. 2004;555:471–480.
- Barp J, Araujo ASR, Fernandes TR, Myocardial antioxidant and oxidative stres changes due to sex hormones. Braz J Med Biol Res. 2002;35:1075–1081.
- Dubey RK, Tyurina YY, Tyurin VA, Estrogen and tamoxifen metabolites protect smooth muscle cell membrane phospholipids against peroxidation and inhibit cell growth. Circ Res. 1999;84:229–239.
- Hamden K, Carreau S, Ellouz F, Masmoudi H, El FA. Protective effect of 17beta-estradiol on oxidative stress and liver dysfunction in aged male rats. J Physiol Biochem. 2007;63:195–201.
- Chiang K, Parthasarathy S, Santanam N. Estrogen, neutrophils and oxidation. Life Sci. 2004;75:2425–2438.
- Chang SP, Yang WS, Lee SK, Min WK, Park JS, Kim SB. Effects of hormonal replacement therapy on oxidative stress and total antioxidant capacity in postmenopausal hemodialysis patients. Ren Fail. 2002;24:49–57.
- Stringer KD, Komers R, Osman SA, Oyama TT, Lindsley JN, Anderson S. Gender hormones and the progression of experimental polycystic kidney disease. Kidney Int. 2005;68(4):1729–1739.
- Maric C, Xu Q, Sandberg K, Hinojosa-Laborde C. Age-related renal disease in female dahl salt-sensitive rats is attenuated with 17β-estradiol supplementation by modulating nitric oxide synthase expression. Gend Med. 2008;5:147–159.
- Westhuyzen J, Adams CE, Fleming SJ. Evidence for oxidative stress during in vitro dialysis. Nephron. 1995;70:49–54.
- Kettle AJ, Winterbourn CC. Myeloperoxidase: A key regulator of neutrophil oxidant production. Redox Rep. 1997;3:3–15.
- Şener G, Sakarcan A, Sehirli O, Ekşioğlu-Demiralp E, Sener E, Ercan F, Gedik N, Yeğen BC. Chronic renal failure-induced multiple-organ injury in rats is alleviated by the selective CysLT1 receptor antagonist montelukast. Prostaglandins and Other Lipid Mediators. 2007;83:257–267.
- Lim CS, Vaziri ND. Iron and oxidative stress in renal insufficiency. Am J Nephrol. 2004;24:569–575.
- Faas MM, Bakker WW, Valkhof N, Schuiling GA. Effect of estradiol and progesterone on the low-dose endotoxin-induced glomerular inflammatory response of the female rat. Am J Reprod Immunol. 1999;41:224–231.
- Baud L, Ardaillou R. Tumor necrosis factor in renal injury. Miner Electrolyte Metab. 1995;21:336–341.
- Guarnieri G, Antonione R, Biolo G. Mechanisms of malnutrition in uremia. J Ren Nutr. 2003;13:153–157.
- Cau J, Favreau F, Zhang K. FR167653 improves renal recovery and decreases inflammation and fibrosis after renal ischemia reperfusion injury. J Vasc Surg. 2009;49:728–740.
- Hsieh CH, Nickel EA, Chen J, Schwacha MG, Choudhry MA, Bland KI, Chaudry IH. Mechanism of the salutary effects of estrogen on kupffer cell phagocytic capacity following trauma-hemorrhage: Pivotal role of Akt activation. J Immunol. 2009;182:4406–4414.
- Huang H, He J, Yuan Y, Aoyagi E, Takenaka H, Itagaki T, Sannomiya K, Tamaki K, Harada N, Shono M, Shimizu I, Takayama T. Opposing effects of estradiol and progesterone on the oxidative stress-induced production of chemokine and proinflammatory cytokines in murine peritoneal macrophages. J Med Invest. 2008;55:133–141.
- Borrás C, Sastre J, García-Sala D, Lloret A, Pallardó FV, Viña J. Mitochondria from females exhibit higher antioxidant gene expression and lower oxidative damage than males. Free Radic Biol Med. 2003;34:546–552.
- Şener G, Paskaloglu K, Toklu H, Kapucu C, Ayanoglu-Dulger G, Kacmaz A, Sakarcan A. Melatonin ameliorates chronic renal failure-induced oxidative organ damage in rats. J Pineal Res. 2004;36:232–241.
- Calunga JL, Zamora ZB, Borrego A, Río S, Barber E, Menéndez S, Hernández F, Montero T, Taboada D. Ozone therapy on rats submitted to subtotal nephrectomy: Role of antioxidant system. Mediators Inflamm. 2005;2005:221–227.
- Ceballos-Picot I, Witko-Sarsat V, Merad-Boudia M, Nguyen AT, Thévenin M, Jaudon MC, Zingraff J, Verger C, Jungers P, Descamps-Latscha B. Glutathione antioxidant system as a marker of oxidative stress in chronic renal failure. Free Radic Biol Med. 1996;21:845–853.
- Himmelfarb J, McMenamin E, McMonagle E. Plasma aminothiol oxidation in chronic hemodialysis patients. Kidney Int. 2002;61:705–716.
- Guccione M, Silbiger S, Lei J, Neugarten J. Estradiol upregulates mesangial cell MMP-2 activity via the transcription factor AP-2. Am J Physiol Renal Physiol. 2002;282:F164–F169.
- Potier M, Elliot SJ, Tack I, Expression and regulation of estrogen receptors in mesangial cells: Influence on matrix metalloproteinase-9. J Am Soc Nephrol. 2001;12:241–251.
- Neugarten J, Acharya A, Lei J, Silbiger S. Selective estrogen receptor modulators suppress mesangial cell collagen synthesis. Am J Physiol Renal Physiol. 2000;279:F309–F318.
- Zdunek M, Silbiger S, Lei J, Neugarten J. Protein kinase CK2 mediates TGF-β1-stimulated type IV collagen gene transcription and its reversal by estradiol. Kidney Int. 2001;60:2097–2108.
- Blush J, Lei J, Ju W, Silbiger S, Pullman J, Neugarten J. Estradiol reverses renal injury in Alb/TGF-β1 transgenic mice. Kidney Int. 2004;66:2148–2154.