Abstract
Nephrotoxicity, associated with the administration of iodinated X-ray contrast media (ICM), continues to be a major side effect in a significant number of vulnerable patients undergoing diagnostic X-ray imaging procedures. The molecular mechanisms underlying these adverse effects on the kidneys are unclear despite several decades of investigation. Side effects are more common after exposure to high-osmolar compared with low-osmolar ICM, suggesting that osmolality may be an important physical–chemical property related to nephrotoxicity. This investigation in cultured NRK 52-E cells, a cell line of renal origin, compares the in vitro toxicity of the iso-osmolal ICM iodixanol with the low-osmolal ICM iohexol, iopromide, and ioversol. The cellular toxicity was evaluated with the trypan blue exclusion assay, the MTT assay, and incidences of cell death. A qualitative assessment of vacuolation of the cultured NRK 52-E cells was taken as a measure of intracellular uptake of ICM. A difference in cell death incidence was observed between the iso-osmolal iodixanol and the low-osmolal iohexol, iopromide, and ioversol contrast media, with the iso-osmolal iodixanol having the least effect in each of the in vitro systems tested. The osmolality of the contrast media appeared to be the major cause for the observed in vitro toxicity.
INTRODUCTION
Contrast-induced nephropathy (CIN) remains one of the most important adverse effects seen in patients treated with iodinated x-ray contrast media (ICM),Citation1 and CIN has been reported to be the third leading cause of acute renal failure in hospitalized patientsCitation2 accounting for 10% of total cases.Citation3 In patients with pre-existing renal impairment, CIN is more frequently reported, and the poorer the renal function the higher the incidence of CIN.Citation4 The pathophysiology of CIN is poorly understood, although hemodynamic effects, direct tubular toxicity, and oxidative stress are considered to be amongst the most important factors.Citation5,Citation6
The osmolality of the ICM, and hence the osmotic load delivered to the kidneys, is thought to play a critical role in the pathogenesis of CIN,Citation6,Citation7 but it is not known whether this is a direct or indirect effect.Citation6 In vitro studies support the hypothesis that the toxicity is related to the osmolality of the ICM, as low-osmolal ICM (LOCM) have considerably less toxic effects than high-osmolal ICM (HOCM) on various cell types, with the observed toxicity being dose dependent.Citation8,Citation9 The ionic force of the molecule seems to be irrelevant to the toxicity.Citation8 However, other studies have shown that mannitol, when tested at equal osmolality to the contrast media, induced less toxicity than ICM.Citation10,Citation11 This indicates that physicochemical properties other than osmolality may be important for the toxicity of ICM. The molecular mechanisms of ICM-induced cytotoxicity are still unclear; however, cellular energy failure, disruption of calcium homeostasis, disturbance of tubular cell polarity, apoptosis, altered cellular metabolism, pathological changes, and intracellular enzyme release are among the observations reported.Citation2,Citation8
The aim of the present study was to evaluate the toxicity of four ICM with different physicochemical properties in the rat kidney cell line NRK 52-E by using the trypan blue exclusion assay and the MTT assay, in addition to scoring of dead cells. The ICM selected were the iso-osmolal iodixanol, and the low-osmolal iohexol, iopromide, and ioversol. All ICM selected are commercially available and are frequently used for radiographic examinations.
MATERIALS AND METHODS
Chemicals
Iodixanol (Visipaque®, 320 mg I/mL) and iohexol (Omnipaque®, 350 mg I/mL) were obtained from GE Healthcare, Oslo, Norway; iopromide (Ultravist®, 300 mg I/mL) was from Bayer Schering Pharma, Berlin, Germany; ioversol (Optiray®, 300 mg I/mL) was from Tyco Healthcare, Asker, Norway. The physicochemical properties of the ICM and the dilutions used in the current evaluation are listed in .
Table 1. Physicochemical properties for iodixanol, iohexol, iopromide, and ioversol
Cell Culture
A rat kidney epithelial cell line (NRK 52-E) was purchased from the European Collection of Cell Cultures. The cell line is cloned from a mixed culture of normal rat kidney cells and has epithelial-like morphology. The cells were routinely grown in 75 cm2 cell culture flasks in Dulbecco's Modified Eagle's medium (DMEM) supplemented with 10% fetal bovine sera, 1% 2 mM l-glutamine, 1% non-essential amino acids, and 1% penicillin/streptomycin solution. Cells were maintained in a sterile incubator at 37°C with 5% CO2 under saturated humidity. The cell medium was changed every third or fourth day. Passages 3–20 were used, and the cells remained stable without any notable differences between the passages.
Trypan Blue Exclusion Assay
NRK 52-E cells were sub-cultivated in 6-well plates and grown to confluence. Confluent cells were washed with Dulbecco'r phosphate buffered salime (PBS) and exposed in duplicate to incubation medium (DMEM with 1% 2 mM l-glutamine, 1% non-essential amino acids, and 1% penicillin/streptomycin solution) (control), iodixanol, iohexol, iopromide, and ioversol for 1, 3, 6, 12, and 24 h. Based on pilot studies, all ICM were tested at a concentration of 150 mg I/mL (diluted in incubation medium). At the termination of the experiment, the supernatants were transferred to separate centrifuge tubes to include possible detached dead cells. The adherent cells were then detached with trypsin-EDTA solution and transferred to their respective centrifuge tubes along with 7 mL fresh incubation medium. The tubes were centrifuged at 200 g for 10 min. Supernatants were removed and the pellets were re-suspended in incubation medium (100–300 µL, depending on the size of the pellet). Equal volumes of cell suspension and 0.4% trypan blue solution were mixed and incubated for 1 min before light microscopic examination to distinguish and count living and dead cells (Bürker Türk chamber , Bürker Türk, Marienfeld, Lauda-Königshofen, Germany). Cell death was calculated and expressed as a percentage [(dead cells/total cells) × 100]. For each time point a minimum of 200 cells were counted . Each experiment was performed in duplicate and repeated five times.
MTT Assay
The MTT [3-(4,5-dimethylthiozol-2-yl)2,5-diphenyltetrazolium bromide] assay is based on the method originally described by Mosmann,Citation14 and performed with modifications adapted from Sigma-Aldrich's MTT-based in vitro toxicology kit. NRK 52-E cells were sub-cultured in 96-well plates and grown to confluence. When confluence was reached, the cell medium was removed and incubation medium (control) (see Trypan blue exclusion assay section), iodixanol, iohexol, iopromide and ioversol were added, 100 µL to each well. All ICM were tested at a concentration of 150 mg I/mL (diluted in incubation medium). Cell viability was assessed after 0.5, 1, 2, 3, 6, 12, and 24 h. At the end of the exposure time, 10 µL of MTT solution (0.05 mg/mL in PBS) was added to each well and the plates were incubated for 4 h. At the end of the MTT incubation period, 100 µL MTT solubilization solution (10% Triton X-100 plus in 0.1 M HCl in anhydrous isopropanol) was added to each well. The plates were incubated at 37°C overnight for the formazan crystals to dissolve. Absorbance was measured at 570 nm using 630 nm as reference. Cell viability is expressed as a percentage of the control values: ODsample/ODcontrol × 100 = cell viability as percent of control. Each experiment was repeated five to eight times.
Cell Morphology
NRK 52-E cells were sub-cultured on cell culture cover slips in thermanox plastic in 24-well plates until confluence was reached. The cells were washed in PBS before incubation, in duplicate, with incubation medium (control) (see Trypan blue exclusion assay section), iodixanol, iohexol, iopromide, and ioversol. Cell morphology, both quantitative (counting dead cells) and descriptive (description of confluence, vacuolation, etc.), was assessed after 0.5, 1, 2, 3, 6, 12, and 24 h incubation. All ICM were tested at a concentration of 150 mg I/mL (diluted in incubation medium). At the termination of the experiment, the supernatants were removed and the cover slip was washed in PBS followed by the immediate addition of 1–2 mL of 10% neutral-buffered formalin to fix the cells (minimum 24 h). The cells were stained in hematoxylin for 1 h, washed in sodium chloride solution (9 mg/mL), and then left to air dry for 30 min. Finally the cover slips were mounted onto glass microscope slides with mountant. Necrotic and apoptotic cells were scored by light microscopic examination of hematoxylin-stained sections at 600 times magnification. The morphological features used to identify and score necrotic and apoptotic cells have been described previously.Citation15–19 To determine the cell death index (CDI), five fields were selected from the two specimens (two counts from one and three counts from the other). For each time point and in each field the number of viable cells and necrotic or apoptotic (dead or dying) cells were recorded. The CDI is presented as a percentage of the total number of cells (±SEM). Each experiment was repeated twice; therefore no statistical analyses were performed. A short description of the morphological features for all exposure periods is also given. All cell morphology evaluations were performed by a pathologist using light microscopy.
Statistical Analysis
The results are expressed as mean ± SEM. The statistical analyses were performed in SigmaStat version 3.1 by using one-way ANOVA followed by Dunnet's test to compare treatment groups against a control group, and Duncan's test for multiple comparisons. Extreme values and outliers, defined as <Q1 – 1.5 IQR (inter-quartile range) and >Q3 + 1.5 IQR, were omitted from the statistical analysis. Differences were considered to be statistically significant when p < 0.05.
RESULTS
Cell Death Assessed by the Trypan Blue Exclusion Assay
Assessment of cell death by the trypan blue exclusion assay after exposure to iodixanol, iohexol, iopromide, and ioversol for 1, 3, 6, 12, and 24 h revealed differences in cell death which increased with increasing osmolality of the ICM (). At early time points (up to 6 h exposure), the cell death induced by the four ICM was similar to the control group. After 6 h exposure, cell death caused by the ICM increased compared with the controls. At 12 and 24 h exposure, iohexol caused the greatest cell death of the four ICM (34% and 81%, respectively), and at both time points cell death was significantly higher than the control and the other ICM. Ioversol caused a slightly higher cell death than iopromide at 12 and 24 h exposure time (42% and 58% vs. 37% and 55%, respectively), and both induced a significantly greater cell death than the control. However, there was no statistically significant difference when compared with each other. Iodixanol caused the least cell death of the four ICM at 12 and 24 h exposure (11% and 37%). At 12 h exposure, the cell death caused by iodixanol was not statistically significantly different from the control; however it was significantly less than the other ICM. At 24 h exposure, the cell death induced by iodixanol was significantly greater than the control, but significantly less than the other ICM. To test the relationship between cell death and osmolality of the ICM, a linear regression analysis was performed at the 24 h time point (graph not shown). An r2-value of 0.7605 indicates a reasonably good relationship between cell death and osmolality.
Table 2. Cell death (%, mean ± SEM), measured by the trypan blue exclusion assay, in NRK 52-E cells after exposure to incubation medium (control), iodixanol, iohexol, iopromide, and ioversol (150 mg I/mL)
Cell Viability Assessed by the MTT Assay
The results from the MTT assay show that iodixanol, iohexol, iopromide, and ioversol inhibited mitochondrial dehydrogenase activity, as determined by MTT reduction, compared with the control (100% at all exposure times) (). Furthermore, the MTT conversion was reduced with increasing osmolality. Even at early time points, the MTT conversion by mitochondrial dehydrogenases was reduced to between 35% and 46% of the control values. The MTT conversion was further reduced as the exposure time increased. All four ICM inhibited the mitochondrial dehydrogenase activity significantly more than the control at all exposure time points. From 0.5 to 6 h exposure time, the reduction in MTT conversion was stable, and the four ICM caused a similar inhibition of mitochondrial dehydrogenase activity. At the 12 and 24 h exposure time points, MTT conversion was further reduced and the difference in mitochondrial dehydrogenase inhibition by the four ICM became more pronounced. Iohexol showed the strongest reduction of the MTT conversion at 12 and 24 h exposure (16% and 6%, respectively). At both exposure times, mitochondrial dehydrogenase inhibition caused by iohexol was significantly greater than the other ICM. Iopromide and ioversol reduced the MTT conversion to a slightly less degree than iohexol at 24 h (11% and 8% vs. 6%, respectively). The reduction in MTT conversion caused by these two ICM was statistically significantly different when compared with each other, and both MTT conversion reductions were significantly lower than iohexol. Iodixanol induced the least reduction in MTT conversion of the four ICM tested. After 12 h exposure, the reduction of the MTT conversion caused by iodixanol seemed to stabilize. At 24 h, the MTT conversion by iodixanol was reduced to approximately 22%, significantly less reduction than the other ICM.
Table 3. Cell viability (percent of control, mean ± SEM), as determined by mitochondrial dehydrogenase activity, in NRK 52-E cells after exposure to incubation medium (control), iodixanol, iohexol, iopromide, and ioversol (150 mg I/mL)
To test the relationship between the reduced cell viability and osmolality, a linear regression analysis was performed on cell viability after 24 h exposure (graph not shown). An r2-value of 0.9658 indicated a strong relationship between the reduced viability and the osmolality of the four ICM.
Cell Death Assessment of Formalin-Fixed NRK 52-E Cells
shows differences in the onset and severity of cell death overtime after exposure to iodixanol, iohexol, iopromide, and ioversol. Cell death caused by the four ICM began to increase 3 h after the start of exposure and continued to increase over time. Iodixanol induced the least cell death at most time points, and the difference between iodixanol and the other ICM was greatest at the 6, 12, and 24 h exposure time points. Iohexol, iopromide, and ioversol seemed to induce approximately the same degree of cell death at all exposure times. To test the relationship between cell death and osmolality, a linear regression analysis was performed after 24 h exposure. The analysis (graph not shown) indicated that there is a strong relationship between the cell death and osmolality (r2 = 0.9987).
Table 4. Cell death (%, mean ± SEM) assessed on formalin-fixed NRK 52-E cells after exposure to incubation medium (control), iodixanol, iohexol, iopromide, and ioversol (150 mg I/mL) (n = 5: two counts from the first specimen and three from the last)
Cell Morphology
All ICM-treated cells at the 0.5, 1, and 2 h time points appeared to have normal morphology when compared with the control. The cells showed distinct cell borders, moderate amount of cytoplasm, and a large nucleus that contained coarsely stippled chromatin and often one or two nucleoli. At the 3 h time point, the cells exposed to iohexol, iopromide, and ioversol had numerous individual dead cells. The dead cells showed condensed or fragmented nuclei, contracted cytoplasm, or indistinct cell border that was not in contact with the surrounding cells. At 3 h, cell death after exposure to iodixanol was similar to the control. However, numerous small clear round vacuoles were noted in the cytoplasm of the iodixanol-treated cells. At 6 h, the cells exposed to iohexol, iopromide, and ioversol showed an increase in cell death and a loss of confluence of the cell layer. Also, within the cytoplasm in the iodixanol-, iohexol-, iopromide-, and ioversol-treated cells, there were numerous small clear round vacuoles. The iohexol-treated cells contained similar numbers of vacuoles, but these were smaller in size than those seen in the iodixanol-treated group, whereas the ioversol- and iopromide-treated cells contain fewer and smaller vacuoles when compared with the cells in the iodixanol-treated group. At 6 h, the cells exposed to iodixanol showed less cell death than cells exposed to the other ICM, and the size and number of vacuoles were greater than in the cells exposed to the other ICM, as well as compared with the cells exposed to iodixanol for 3 h. At 12 h, cell death and loss of confluence had increased in all ICM groups compared with the 6 h exposure and the control. The degree of vacuolation was similar to the 6 h time point. At the 24 h time point in all the ICM groups, there was extensive cell death and a total loss of confluence. The vacuolation was not observable in the dead cells. In the viable cells exposed to iodixanol, the degree of vacuolation was similar to that seen at the 3 h time point. A–D shows representative pictures of the cell morphology after exposure to ICM.
Figure 1. (A) Hematoxylin-stained NRK 52-E cells (control – incubation media treated) (×200). Note the confluence of the cell layer. Randomly scattered throughout the culture are cells that have separated from the surrounding cells and contain condense dark nuclei (arrows). These are degenerating/dying cells. (B) Hematoxylin-stained NRK 52-E cells after 1 h exposure to 150 mg I/mL ioversol (×400). Note the increased number of dead cells scattered throughout the cell culture layer. (C) Hematoxylin-stained NRK 52-E cells after 3 h exposure to 150 mg I/mL ioversol (×400). There are more necrotic cells and the confluence of the cell culture layer is lost. (D) Hematoxylin-stained NRK 52-E cells after 12 h exposure to 150 mg I/mL ioversol (×400). Note that almost all the cells are either necrotic or dying. Also cell-to-cell contact is minimal.
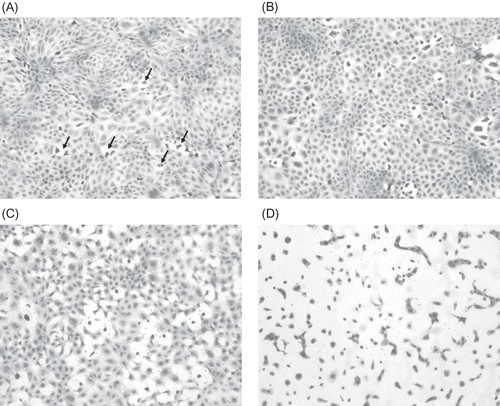
DISCUSSION
This study with the cultured NRK 52-E rat kidney cell line indicates that the osmolality of the ICM seems to be a major factor that contributes to the observed cytotoxicity, as the induced cell death or reduced cell viability correlates with increasing osmolality of the ICM tested. Furthermore, the chemical structure of the LOCM may also contribute to the observed cytotoxicity as the trypan blue exclusion assay revealed difference in their potential to induce cell death. In this study, the toxicity (cell death or cell viability) induced by iodixanol was significantly less than that caused by iopromide, ioversol, and iohexol.
Exposure periods of several hours are required to induce toxicity in NRK 52-E cells, and this is consistent with the findings of others.Citation8 This prolonged exposure is relevant to the clinical setting as renal retention of low doses of ICM in the kidney has been reported for up to 32 h after administration of ICM.Citation20 The concentration of the ICM used in the current study was selected on the basis of previously reported in vitro studies,Citation21,Citation22 and also on the fact that ICM are concentrated in the kidneys compared with blood plasma. Urinary concentrations of ICM can be up to 50–100 times that in plasma.Citation23 Furthermore, Spataro et al.Citation24 reported urinary concentration up to approximately 140 mg I/mL at 60 min after administration of 450 mg I/kg ioxaglate in rabbits.
The degree of cell death, as determined by the trypan blue exclusion assay reported for iodixanol, iohexol, and iopromide, was generally lower than the control after 1–6 h exposure. Berg et al.Citation25 showed that iodixanol, iohexol, ioxaglate, and diatrizoate (contrast media from all classes of ICM) all possess antioxidant properties in vitro. The reason for this is unknown, but it is possible (although speculative) that the antioxidant properties of the ICM may contribute to the lower cell death at early time points.
The trypan blue exclusion assay is one of the most commonly used in vitro methods to assess cell death after ICM exposure, and experiments using trypan blue are frequently reported.Citation10,Citation26–30 The findings from the trypan blue exclusion assay indicate that cell death can in part be explained by the osmolality of the ICM, as this increases with increasing osmolality, supported by regression analysis which showed a reasonably good correlation between the two (r2 = 0.76). However, the three non-ionic monomers differ in their potential to induce cell death. This finding suggests that the chemical structure of the contrast media may also contribute to toxicity.
The reduction in MTT conversion after 24 h exposure can be explained by the osmolality of the ICM. The regression analysis showed a strong correlation between the reduced cell viability and the osmolality of the ICM (r2 = 0.97). The reductions in MTT conversion caused by the four ICM were significantly different from each other when compared pairwise. However, the small differences in the inhibition of mitochondrial dehydrogenases caused by iohexol, iopromide, and ioversol after 24 h exposure are considered not to have any toxicological relevance.
When cell death was assessed by morphology on formalin-fixed NRK 52-E cells, iodixanol induced the least cell death at most time points (≥3 h exposure time), whereas iohexol, iopromide, and ioversol all caused a similar increased cell death compared with iodixanol. The regression analysis revealed a strong correlation between cell death and osmolality of the ICM (r2 = 0.99).
The morphological evaluation performed on NRK 52-E cells showed considerable cytoplasmic vacuolation after 6 h exposure and was most pronounced in the iodixanol-treated cells. A lesser effect was also observed in the iohexol-, iopromide-, and ioversol-treated cells, where numerous small clear round vacuoles were observed. The observed cytoplasmic vacuolation is believed to be due to uptake and retention of ICM in the cells, and this finding is in accordance with what is previously observed in in vitro and in vivo experiments.Citation26,Citation31 The morphological observations indicated that the uptake into cells could be ranked in the following decreasing order: iodixanol > iohexol > iopromide > ioversol. The osmolality appears to play a role, with the iso-osmolal ICM (IOCM) being most extensively internalized followed by the three LOCM. The cellular mechanism for vacuolation is poorly understood. Nordby et al.Citation32 proposed two possible mechanisms to explain the intracellular uptake of ICM; ICM may cause a transitory, low-grade membrane damage with leakage of extracellular medium into the cells and may pass through leaky channels or be actively transported by endocytosis or pinocytosis. The first explanation is speculative as membrane damage is not needed in living cells for uptake of ICM. On the other hand, iodixanol has been used as a marker of fluid-phase endocytosis,Citation33 and this is the more probable mechanism of uptake of ICM into the renal proximal tubule cells.
To our knowledge, no other in vitro study on renal cells has previously reported such pronounced differences in cell death or reduced cell viability when comparing IOCM (iodixanol) and LOCM (iohexol, iopromide, and ioversol). Heinrich et al.Citation21 compared different contrast media with the MTT assay, and when ICM were compared at equal iodine concentrations (75 mg I/mL), they found that dimeric contrast media showed a slightly weaker effect on inhibition of mitochondrial dehydrogenases than monomers, but this difference was not statistically significant. In the same study, the contrast media were also compared at molar basis and it was shown that dimeric ICM were significantly more cytotoxic than monomers on cultured renal cells. However, as ICM are dosed in terms of iodine concentration, not on a molar basis, this basis for comparison can be questioned. Dimeric contrast media contain twice the number of iodine atoms per molecule and are therefore administered at half the molar dose.Citation34 Another study by Heinrich et al.Citation35 showed no difference in toxicity on LLC-PK1 cells between iodixanol and iomeprol over a broad range of concentrations and incubation times. In a study by Itoh et al.Citation36 it was shown that iotrolan reduced the cell viability (determined by WST-8 assay), whereas iodixanol did not. The two contrast media have about the same osmolality, and hence other properties than osmolality must have been responsible for the decrease in cell viability induced by iotrolan. Furthermore, two in vitro studiesCitation27,Citation29 did not find any significant differences between iodixanol and LOCM in cultured renal cells. Hardiek et al.Citation27 reported that when iopamidol, iomeprol, iodixanol, sodium meglumine diatrizoate, and ioxaglate sodium meglumine were compared, no specific property could be attributed to the observed toxicity. The authors concluded that the iconicity seemed to have the greatest influence on MTT reduction, followed by the chemical structure of the contrast agent. The ICM that revealed the least pronounced toxicity in the study was iomeprol. Iomeprol is currently the only ICM that does not contain ethylenediaminetetraacetic acid (EDTA) as an additive in the contrast solution. It is possible that in in vitro systems with long exposure times (24 h), the absence of the chelating agent EDTA may account for the reduced toxicity. In the study by Schick and Haller,Citation29 iodixanol and iohexol induced the least pronounced toxicity of the four ICM compared. However, the exposure time was only 6 h. Such a short exposure time might be more clinically relevant, but as shown in the present work, 6 h exposure is too short to reveal the differences in cytotoxicity between the contrast media. Romano et al.Citation37 did not find any difference in the extent of cell injury when comparing iodixanol and iobitridol, and concluded that the cytotoxic effect does not seem to be caused by iodine or osmolality ≤830 mOsm/L.
In conclusion, the iso-osmolal contrast medium iodixanol was found to be less toxic than the LOCM iopromide, ioversol, and iohexol in cultured cells of rat proximal tubule origin. The different osmolalities of the contrast media were shown to correlate with several different indices of cellular toxicity, and support the conclusion that this is a major contributory factor in CIN.
Declaration of interest
: The authors report no conflicts of interest. The authors alone are responsible for the content and writing of the paper.
REFERENCEs
- Rihal CS, Textor SC, Grill DE, Incidence and prognostic importance of acute renal failure after percutaneous coronary intervention. Circulation. 2002;105:2259–2264.
- Waybill MM, Waybill PN. Contrast media-induced nephrotoxicity: Identification of patients at risk and algorithms for prevention. J Vasc Interv Radiol. 2001;12:3–9.
- Persson PB. Contrast-induced nephropathy. Eur Radiol. 2005;15(Suppl. 4):D65–D69.
- Morcos SK. Contrast media-induced nephrotoxicity – Questions and answers. Br J Radiol. 1998;71:357–365.
- Persson PB, Tepel M. Contrast medium-induced nephropathy: The pathophysiology. Kidney Int Suppl. 2006;69:S8–S10.
- Tumlin J, Stacul F, Adam A, Pathophysiology of contrast-induced nephropathy. Am J Cardiol. 2006;98:14K–20K.
- Mehran R, Nikolsky E. Contrast-induced nephropathy: Definition, epidemiology, and patients at risk. Kidney Int Suppl. 2006;100:S11–S15.
- Haller C, Hizoh I. The cytotoxicity of iodinated radiocontrast agents on renal cells in vitro. Invest Radiol. 2004;39:149–154.
- Duan S, Zhou X, Liu F, Comparative cytotoxicity of high-osmolar and low-osmolar contrast media on HKCs in vitro. J Nephrol. 2006;19:717–724.
- Haller C, Schick CS, Zorn M, Kubler W. Cytotoxicity of radiocontrast agents on polarized renal epithelial cell monolayers. Cardiovasc Res. 1997;33:655–665.
- Hizoh I, Strater J, Schick CS, Kubler W, Haller C. Radiocontrast-induced DNA fragmentation of renal tubular cells in vitro: Role of hypertonicity. Nephrol Dial Transplant. 1998;13:911–918.
- Davidson C, Stacul F, McCullough PA, Contrast medium use. Am J Cardiol. 2006;98:42K–58K.
- Morcos SK. Contrast media induced nephrotoxicity. In: Dawson P, Cosgrove DO, Grainger RG, eds. Textbook of Contrast Media. Oxford, UK: Isis Medical Media; 1999:135–148.
- Mosmann T. Rapid colorimetric assay for cellular growth and survival: Application to proliferation and cytotoxicity assays. J Immunol Methods. 1983;65:55–63.
- Bursch W, Taper HS, Lauer B, Schulte-Hermann R. Quantitative histological and histochemical studies on the occurrence and stages of controlled cell death (apoptosis) during regression of rat liver hyperplasia. Virchows Arch B Cell Pathol Incl Mol Pathol. 1985;50:153–166.
- Levin S. Apoptosis, necrosis, or oncosis: What is your diagnosis? A report from the Cell Death Nomenclature Committee of the Society of Toxicologic Pathologists. Toxicol Sci. 1998;41:155–156.
- Levin S, Bucci TJ, Cohen SM, The nomenclature of cell death: Recommendations of an ad hoc Committee of the Society of Toxicologic Pathologists. Toxicol Pathol. 1999;27:484–490.
- Mohr U. Commentary: Comments on the cell death nomenclature recommendations of the Society of Toxicologic Pathologists. Toxicol Pathol. 1999;27:497.
- Stephens LC, Ang KK, Schultheiss TE, Milas L, Meyn RE. Apoptosis in irradiated murine tumors. Radiat Res. 1991;127:308–316.
- Rickards D, Jakobsen JA, Klaveness AJ, Kjaersgaard P. Delayed CT of the kidneys after iopentol (Imagopaque 350) injection in patients with normal renal function. An assessment of the gradual decrease in opacification over 32 h after injection. Eur Radiol. 1997;7(Suppl. 4):S149–S151.
- Heinrich MC, Kuhlmann MK, Grgic A, Heckmann M, Kramann B, Uder M. Cytotoxic effects of ionic high-osmolar, nonionic monomeric, and nonionic iso-osmolar dimeric iodinated contrast media on renal tubular cells in vitro. Radiology. 2005;235:843–849.
- Wasaki M, Sugimoto J, Shirota K. Glucose alters the susceptibility of mesangial cells to contrast media. Invest Radiol. 2001;36:355–362.
- Katzberg RW. Urography into the 21st century: New contrast media, renal handling, imaging characteristics, and nephrotoxicity. Radiology. 1997;204:297–312.
- Spataro RF, Fischer HW, Boylan L. Urography with low-osmolality contrast media: Comparative urinary excretion of Iopamidol, Hexabrix, and diatrizoate. Invest Radiol. 1982; 17:494–500.
- Berg K, Skarra S, Bruvold M, Brurok H, Karlsson JO, Jynge P. Iodinated radiographic contrast media possess antioxidant properties in vitro. Acta Radiol. 2005;46:815–822.
- Andersen KJ, Christensen EI, Vik H. Effects of iodinated x-ray contrast media on renal epithelial cells in culture. Invest Radiol. 1994;29:955–962.
- Hardiek K, Katholi RE, Ramkumar V, Deitrick C. Proximal tubule cell response to radiographic contrast media. Am J Physiol Renal Physiol. 2001;280:F61–F70.
- Heinrich MC, Kuhlmann MK, Kohlbacher S, Cytotoxicity of iodinated and gadolinium-based contrast agents in renal tubular cells at angiographic concentrations: In vitro study. Radiology. 2007;242:425–434.
- Schick CS, Haller C. Comparative cytotoxicity of ionic and non-ionic radiocontrast agents on MDCK cell monolayers in vitro. Nephrol Dial Transplant. 1999;14:342–347.
- Schick CS, Bangert R, Kubler W, Haller C. Ionic radiocontrast media disrupt intercellular contacts via an extracellular calcium-independent mechanism. Exp Nephrol. 2002;10:209–215.
- Dobrota M, Powell CJ, Holtz E, Wallin A, Vik H. Biochemical and morphological effects of contrast media on the kidney. Acta Radiol Suppl. 1995;399:196–203.
- Nordby A, Tvedt KE, Halgunset J, Kopstad G, Haugen OA. Incorporation of contrast media in cultured cells. Invest Radiol. 1989;24:703–710.
- Kjeken R, Mousavi SA, Brech A, Gjoen T, Berg T. Fluid phase endocytosis of [125I]iodixanol in rat liver parenchymal, endothelial and Kupffer cells. Cell Tissue Res. 2001;304:221–230.
- Skotland T, Grant D. In vitro cytotoxic effects of iodinated contrast media on a renal tubular cell line. Radiology. 2006;238:759–760.
- Heinrich M, Scheer M, Heckmann M, Bautz W, Uder M. Reversibility and time-dependency of contrast medium induced inhibition of 3-(4,5-dimethylthiazol-2-yl)-2,5-diphenyl-tetrazolium bromide (MTT) conversion in renal proximal tubular cells in vitro: Comparison of a monomeric and a dimeric nonionic iodinated contrast medium. Invest Radiol. 2007;42:732–738.
- Itoh Y, Yano T, Sendo T, Involvement of de novo ceramide synthesis in radiocontrast-induced renal tubular cell injury. Kidney Int. 2006;69:288–297.
- Romano G, Briguori C, Quintavalle C, Contrast agents and renal cell apoptosis. Eur Heart J. 2008; 29:2569–2576.