Abstract
The coexistence of hypertension and diabetes results in the rapid development of nephropathy. Hydrogen sulfide (H2S) is claimed to control the vascular and renal functions. This study tested the hypothesis that exogenous H2S lowers the blood pressure and decreases the progression of nephropathy in spontaneously hypertensive rats (SHR) that were diabetic. Eighteen SHR were divided into three groups: SHR, SHR diabetic, and SHR diabetic treated with a group of Wistar–Kyoto rats serving as normotensive nondiabetic control. Diabetes was induced with streptozotocin (STZ) in two groups and one diabetic group received sodium hydrosulfide (NaHS), a H2S donor for 5 weeks. Blood pressure was measured in conscious and anesthetized states and renal cortical blood perfusion in acute studies. Plasma and urinary H2S levels, creatinine concentrations, and electrolytes were measured on three different occasions throughout the 35-day period. Diabetic SHR had higher blood pressure, lower plasma and urinary H2S levels, and renal dysfunction as evidenced by increased plasma creatinine, creatinine clearance, and decreased urinary sodium-to-potassium ratio and renal cortical blood perfusion. NaHS reduced blood pressure, increased H2S levels in plasma and urinary excretion, and reversed the STZ-induced renal dysfunction. The findings of this study suggest that the administration of exogenous H2S lowers the blood pressure and confers protection against the progression of STZ-induced nephropathy in SHR.
INTRODUCTION
Hypertension and diabetes are common comorbidities that often coexist. During diabetes, the chances of developing hypertension are 2.5 times greater as compared with the normal state.Citation1 Similarly, previous data have suggested that there is increased incidence of diabetes during hypertension and about 20% of hypertensive individuals have coexisting diabetes.Citation2 Increased activity of the renin angiotensin aldosterone system (RAAS) in hypertension and diabetes has been proposed.Citation3 This upregulation of RAAS results in increased oxidative stressCitation4 and the formation of reactive oxygen species (ROS), which in turn affect glucose utilization and blood pressure in a setting of hypertension and diabetes.Citation5 Similarly aldosterone-induced increased sympathetic nervous system activity and reduced baroreceptor activity have also been observed.Citation6 Nephropathy is one of the major microvascular complications of diabetes. Hypertension, whether related to diabetes or not, additionally damages the kidney resulting in complex patterns of nephropathy.Citation7 It has been reported that in the presence of hypertension the induction of diabetes with streptozotocin (STZ) results in accelerated renal injury.Citation8 The existence of hypertension with diabetes disturbs renal hemodynamics, increases glomerular basement membrane thickness, and promotes fibrosis and inflammation through the activation of RAAS.Citation9 Renal disorders are mostly responsible for the secondary hypertension. However, it is also possible that hypertension may act as a pathogenic factor in the development of nephropathy in association with diabetes.Citation10
Endogenous hydrogen sulfide (H2S) has been recognized as a novel gaseous transmitterCitation11 with the concentration of ∼46 μM in rat serum.Citation12 Recent studies have shown that vascular tissues are also capable of generating measurable amounts of H2S.Citation12 The two pyridoxal phosphate-dependent enzymes, cystathionine beta-synthase (CBS) and cystathionine gamma-lyase (CSE), are responsible for the majority of the endogenous production of H2S in mammalian tissues that use l-cysteine as the main substrate.Citation13 The expression of CBS and CSE has been identified in many human and other mammalian cells, including those from liver, heart, blood vessels, kidney, brain, skin fibroblasts, and blood lymphocytes.Citation14 Since the realization that H2S is an important biomolecule, its antihypertensive activity has been widely studied. It has been reported that it has the ability to relax vascular smooth muscle and reduce blood pressure.Citation12 H2S has been linked as pathogenic factor in different settings of hypertension, including spontaneous hypertension,Citation15 renovascular hypertension,Citation16 and l-nitro-arginine methyl ester (L-NAME)-induced hypertension.Citation17 Similarly it has been suggested that suppressed CSE-catalyzed endogenous H2S production in renal tissue by persistent hyperglycemia plays a role in the pathogenesis of diabetic nephropathy.Citation18
Previous studies have led to the common view that hypertension combined with diabetes results in profound renal injury, and H2S is involved in the pathogenesis of hypertension and diabetic nephropathy. This study explores the antihypertensive and renoprotective potentials of exogenous H2S in spontaneously hypertensive rats (SHR) that were diabetic.
MATERIALS AND METHODS
Experimental Animals
SHR and Wistar–Kyoto (WKY) rats weighing 235 ± 15 g were obtained from the animal house and breeding facility of Universiti Sains Malaysia. Rats were acclimatized for 1 week before any experimental procedure. All the animals were housed in the same environmental conditions with free access to food (Gold Coin, Sdn. Bhd., Penang, Malaysia) and drinking water ad libitum. The measurement of conscious blood pressure was carried out in SHR to demonstrate the hypertension using the tail-cuff method. Only animals exhibiting a systolic blood pressure of 150 mmHg or above were used. The procedures and protocols used in this study were according to the guidelines of Universiti Sains Malaysia animal ethics committee.
Experimental Protocol
SHR were randomly divided into three groups (n = 6): SHR (II), SHR diabetic (III), and SHR diabetic treated (IV). Equal numbers of WKY rats of matching body weight and age served as normotensive control (I). Diabetes was induced in groups III and IV with a single injection of STZ (Nova Laboratories, Sdn. Bhd., Selangor, Malaysia) at a dose of 40 mg/kg. STZ was dissolved in freshly prepared ice-cold sodium citrate buffer (0.1 mol/L, pH 4.5) and injected intraperitoneally.Citation19 The SHR group of rats (II) received an equal amount of buffer intraperitoneally. After 3 days, a drop of blood was obtained by nicking the tail of overnight fasted rats and glucose concentrations were obtained using glucometer (GlucoSure plus, Apex Biotechnology Corp., Hsinchu, Taiwan) to determine the extent of hyperglycemia. Only animals exhibiting fasting blood glucose above or equal to 350 mg/dL were included for further study. After the confirmation of diabetes the rats of group IV were treated with sodium hydrosulfide (NaHS) (Sigma Aldrich, Selangor, Malaysia), a donor of exogenous H2S.Citation15–17 NaHS was freshly prepared in saline and was injected at dose of 56 μmol/kg intraperitoneally at the same time daily for 5 weeks.Citation15 Groups II and III served as SHR and SHR diabetic control, respectively, and did not receive any treatment.
Blood Pressure Measurement
The arterial blood pressure was determined indirectly by the tail-cuff method using the Model 29 pulse amplifier and Model 20NW cuff pump coupled to a computerized data acquisition system (PowerLab®, ADInstruments, Sydney, Australia) on days 0 and 21. On day 34 of this study, an invasive blood pressure measurement was obtained under ketamine and xylazine anesthesia in surgically prepared animals.
Collection of Metabolic Data and Plasma
Metabolic data were collected from all the groups of animals on days 0, 21, and 34 of 35 days of this study. Rats were placed in metabolic cages for 24 h after which water intake and urine output were measured. Similarly, blood samples were obtained on days 0, 21, and 34 and placed in a pre-cooled heparinized Eppendorf tube and plasma was obtained by centrifugation of the blood sample at 2500 rpm for 5 min. Plasma and urine samples were stored at −30°C until analysis for H2S, creatinine, sodium, and potassium.
Surgical Procedure
All the rats were fasted overnight before the acute experiment and anesthetized with a mixture of ketamine (Ilium, Smithfield, NSW, Australia) 80 mg/kg and xylazine (Ilium) 10 mg/kg intraperitoneally,Citation20 and were supplemented intravenously with ketamine at a dose of 50 mg/kg if required. Tracheotomy was then performed to maintain a clear airway by using the endotracheal cannula (PP 240, Portex Ltd., Kent, UK). The left jugular vein was catheterized with PP 50 tubing (Portex Ltd.) to permit the infusion of supplementary anesthesia and drugs as necessary. The right carotid artery was cannulated and the cannula was advanced up to the aortic arch. Thereafter, a midline abdominal incision was made and the left kidney and iliac artery were exposed. The left iliac artery was catheterized and the cannula was pushed up to the abdominal aorta just proximal to the point where iliac bifurcation starts. Both the cannulas were connected to pressure transducers (P23 ID Gould, Statham Instruments, London, UK) linked to a data acquisition system (PowerLab®, ADInstruments) through a Quad Amp (ADInstruments) using chart Pro (V.5.5) software (ADInstruments). A laser Doppler flow probe (OxyFlow, ADInstruments was positioned on the dorsal surface of the posterior end of the exposed left kidney in order to measure renal cortical blood perfusion. The probe was connected to a laser Doppler flowmeter (ADInstruments) which was directly linked to data acquisition system (PowerLab®, ADInstruments).
The animals were allowed to stabilize for 1 h upon completion of the above surgical procedure. After the stabilization period, renal cortical blood perfusion, mean arterial pressure, systolic blood pressure, pulse pressure, and heart rate were recorded continuously for 30 min.
Measurement of Plasma and Urine H2S Levels
H2S levels in plasma and urine were measured spectrophotometrically according to a previously described method.Citation15 Briefly, 100 μL aliquots of the samples were mixed with 50 μL distilled water in microcentrifuge tubes containing 300 μL zinc acetate (1% w/v) to trap H2S. The reaction was terminated after 5 min by adding 200 μL N, N-2-dimethyl-p-phenylenediamine sulfate (20 mM in 7.2 M HCl) and immediately followed by the addition of 200 μL FeCl3 (30 mM in 1.2 M HCl). The mixture was kept in the dark for 20 min. In order to precipitate protein from the samples, 150 μL trichloroacetic acid (10% w/v) was added. The mixture was centrifuged at 10,000 rpm for 10 min. The absorbance of the resulting supernatant was measured at 670 nm using a 96-well plate reader (BioTek Instruments, Inc., VT, USA). All samples were assayed in duplicates. Finally, H2S concentration in the plasma or urine was calculated against the calibration curve of standard H2S solutions (NaHS: 3.125–100 μM). All chemicals used were obtained in pure form from Sigma Aldrich.
Biochemical Analysis and Urinary Sodium and Potassium Ratio
Plasma and urinary creatinine concentrations were measured spectrophotometrically (Jaffe’s reaction). Sodium and potassium concentrations in urine were measured using a flame photometer (Jenway Ltd., Felsted, UK). Creatinine clearance was calculated from plasma and urinary creatinine values along with urine flow rate and was expressed per 100 g body weight. Urinary sodium-to-potassium ratio was calculated by dividing the urinary sodium concentration by potassium concentration.
Statistical Analysis
All the data were expressed as means ± SEM. Statistical significance was set at p < 0.05. Statistical analysis was performed using one-way analysis of variance (ANOVA) followed by Bonferroni/Dunn all means post hoc test using the statistical package Superanova (Abacus Inc., Lincoln, CA, USA).
RESULTS
The single intraperitoneal (i.p.) injection of STZ effectively induced diabetes in SHR as reflected by the significant hyperglycemia (p < 0.05), decrease in body weight, polyuria, and polydipsia that occurred in the SHR diabetic groups as compared with nondiabetic controls (). There were no significant differences (all p > 0.0) in blood glucose concentration and body weight at the end of the treatment period between the SHR diabetic-treated group and the SHR diabetic control (). On the other hand, the SHR diabetic-treated group exhibited an increased urine flow rate and water intake in the later part of the treatment period as compared with the SHR diabetic control (both p < 0.05; ).
Table 1. Effect of streptozotocin (STZ) and exogenous hydrogen sulfide (H2S) on blood glucose, body weight, urine flow rate, and water intake in STZ-induced diabetic spontaneously hypertensive rats (SHR).
There was no significant difference in plasma creatinine () between the SHR and WKY control group of rats (all p > 0.05); however, the induction of diabetes resulted in the elevation of plasma creatinine in the SHR diabetic group at all three observation time points compared with the SHR control group. The administration of NaHS significantly reduced the plasma creatinine () in the treated group as compared with the SHR diabetic control group on days 21 and 34 of treatment (p < 0.05). Moreover, there was a higher creatinine clearance () in the SHR diabetic group as compared with the SHR control group (p < 0.05). It was found that the SHR diabetic NaHS-treated rats exhibited increased creatinine clearance () on days 21 and 34 compared with the SHR diabetic control group (both p < 0.05). The sodium-to-potassium ratio of the SHR group was significantly higher (p < 0.05) compared with the WKY control group (). In addition, the urinary sodium-to-potassium ratio of the diabetic SHR group was significantly lower compared with the SHR control group on days 21 and 34 of the study period (both p < 0.05). With NaHS treatment, it was found that the sodium-to-potassium ratio of the treated SHR group was significantly higher by day 34 of treatment as compared with the SHR diabetic control group (p < 0.05) ().
Table 2. Effect of exogenous hydrogen sulfide (H2S) (56 μmol/kg intraperitoneally) on plasma creatinine, creatinine clearance, and urinary sodium-to-potassium ratio in streptozotocin (STZ)-induced diabetic spontaneously hypertensive rats (SHR).
Figure 1. Renal cortical blood perfusion of Wistar–Kyoto (WKY), spontaneously hypertensive (SHR), SHR diabetic, and SHR diabetic-treated groups of rats. The values are mean ± SEM (n = 6). Statistical analysis was done by one-way analysis of variance (ANOVA) followed by Bonferroni/Dunn all means post hoc test for all groups. Observation was made on day 34 of this study.
Note: *p < 0.05 versus WKY control; **p < 0.05 versus SHR control; ***p < 0.05 versus SHR diabetic control.
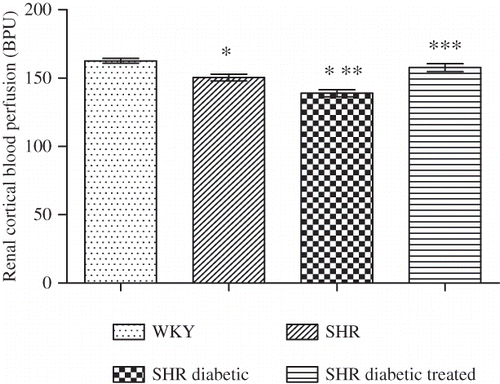
Figure 2. Mean arterial blood pressure (A) and systolic blood pressure (B) of Wistar–Kyoto (WKY), spontaneously hypertensive (SHR), SHR diabetic, and SHR diabetic-treated groups of rats. Measured in conscious state on days 0 and 21 and in anesthetized state on day 34. The values are mean ± SEM (n = 6). Statistical analysis was done by one-way analysis of variance (ANOVA) followed by Bonferroni/Dunn all means post hoc test for all groups in respective days.
Note: *p < 0.05 versus WKY control; ***p < 0.05 versus SHR diabetic control.
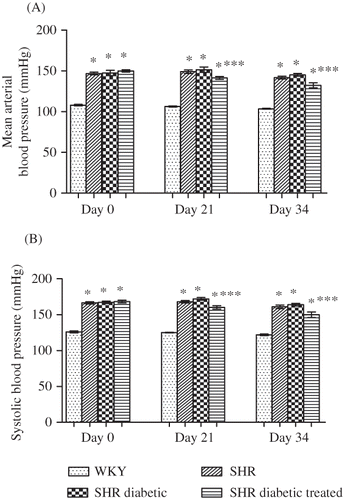
Renal cortical blood perfusion in the SHR control and SHR diabetic groups () was significantly lower compared with the WKY control group (all p < 0.05). Moreover, the SHR diabetic group of rats exhibited a lower renal cortical perfusion compared with the SHR control group (p < 0.05). With NaHS treatment, the renal cortical blood perfusion of the treated group () was significantly higher compared with the SHR diabetic control group (i.e., 158 ± 3 vs. 139 ± 3 BPU; p < 0.05).
Figure 3. Plasma (A) and urinary (B) hydrogen sulfide (H2S) of Wistar–Kyoto (WKY), spontaneously hypertensive (SHR), SHR diabetic, and SHR diabetic-treated groups of rats. The values are mean ± SEM (n = 6). Statistical analysis was done by one-way analysis of variance (ANOVA) followed by Bonferroni/Dunn all means post hoc test for all groups in respective days.
Note: *p < 0.05 versus WKY control; **p < 0.05 versus SHR control; ***p < 0.05 versus SHR diabetic control.
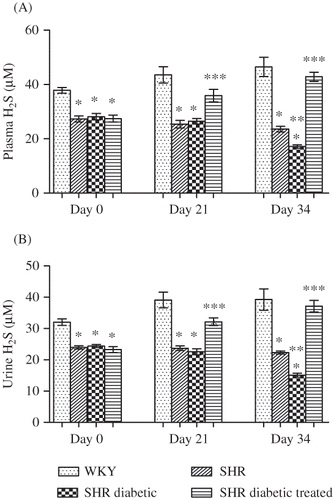
The mean arterial blood pressure and systolic blood pressure () of SHR, SHR diabetic, and diabetic SHR-treated groups remained significantly higher on all three points of observation as compared with the WKY control group (all p < 0.05). There was no significant difference in mean arterial blood pressure and systolic blood pressure between the SHR and SHR diabetic groups (all p > 0.05). It was evident that with NaHS treatment (), the mean arterial blood pressure of the treated SHR was significantly lower on days 21 and 34 compared with the SHR diabetic control, that is, 141 ± 2 versus 152 ± 3 mmHg and 132 ± 3 versus 145 ± 2 mmHg, respectively (all p < 0.05). Similarly, the systolic blood pressure was significantly lower on days 21 and 34 of treatment in the SHR diabetic-treated group compared with the SHR diabetic control, that is, 160 ± 2 versus 172 ± 2 mmHg and 150 ± 4 versus 164 ± 2 mmHg, respectively (all p < 0.05; ).
Plasma and urinary levels of H2S () in the SHR and SHR diabetic groups were significantly lower on all 3 days of observation compared with the WKY control group (all p < 0.05). Following the induction and progression of diabetes, the SHR diabetic group exhibited a significantly lower level of H2S in both plasma and urine () on day 34 compared with the SHR control group (both p < 0.05). With NaHS treatment, it was found that the plasma and urinary levels of H2S in the treated diabetic SHR () were significantly (all p < 0.05) higher on days 21 and 34 compared with the SHR diabetic control, that is, 35.9 ± 2.3 versus 26.5 ± 1.0 μM on day 21 and 42.9 ± 1.7 versus 17.2 ± 0.7 μM on day 34 in plasma, and 32.2 ± 1.23 versus 22.6 ± 1.0 μM on day 21 and 37.1 ± 1.9 versus 15.1 ± 0.7 μM on day 34 in urine.
DISCUSSION
This study investigated the antihypertensive and renoprotective effects of H2S in a combined state of hypertension and diabetes. This was undertaken by studying the effects of supplementation of H2S in SHR diabetic rats. The major findings of this study were that the exogenously administered H2S in the form of NaHS reduced blood pressure, increased H2S levels, significantly reversed the STZ-induced increase in plasma creatinine and decrease in urinary sodium-to-potassium ratio, and reduced renal cortical blood perfusion.
In this study STZ was used to induce diabetes in the SHR and it was found that the SHR presented with typical features of diabetes mellitus, that is, hyperglycemia, polyuria, and polydipsia. The degree of hyperglycemia produced by STZ was about the same in all groups, and the action of STZ is known to produce an irreversible O-glycosylation of intracellular proteins resulting in β-cell death.Citation21 Moreover, hyperglycemia together with the significant weight loss in the STZ-treated group of rats is a characteristic feature of insulin-dependent diabetes mellitus. These findings are consistent with earlier studiesCitation22 and can be explained on the basis of a lack of insulin. The NaHS-treated rats remained hyperglycemic and continued to lose weight throughout the study period, suggesting that the exogenous H2S did not have any effect on blood glucose and body weight. On the other hand, it was apparent that with NaHS treatment the urine flow rate was elevated. This finding is in accord with an earlier study demonstrating increased urine volume in a dose-dependent manner following administration of H2S into the renal artery.Citation23 It was previously reported that the abnormalities in sodium reabsorption and excretion in diabetes have been linked to the developing nephropathy.Citation24,25 In 2009, it was reported by Xia et al. that exogenous H2S in the form of NaHS increased urinary sodium excretion by inhibiting the renal Na+/K+-2Cl co-transport mechanism and renal Na+/K+-ATPase activity. The observation of an increased urine flow rate with NaHS treatment can possibly be explained on the basis of a natriuresis produced by the exogenous H2S such that the water passively followed the sodium which resulted in an increase in urine output.
It has been reported that hypertension combined with diabetes results in an accelerated progression of nephropathy in diabetic SHR and is a suitable model for the evaluation of antihypertensive therapy.Citation10 Plasma creatinineCitation26 and creatinine clearance, a measure of glomerular filtration rate (GFR),Citation8 are commonly used to evaluate the renal hemodynamic function. Khan et al.Citation27 reported that the SHR-STZ model of genetic hypertension and diabetes is one which develops the typical changes in renal injury early in the course of the disease. Interestingly, we have also demonstrated renal injury in terms of increased plasma creatinine and creatinine clearance in the diabetic SHR group. Together, these findings suggest that a single i.p. injection of STZ in SHR rats produced a consistent model of experimental diabetic nephropathy. It is widely accepted that there is a considerable increase in GFR in the early stage of diabetes,Citation28,29 and a comparable hyperfiltration, reflected by the increased creatinine clearance, was found in this study. Many factors, including hyperglycemia, prostanoids, nitrogen oxide, atrial natriuretic factor, growth hormone, glucagon, insulin, angiotensin II (ANG II), and others, have been considered as potential agents causing hyperperfusion and hyperfiltration.Citation30 A primary cause of hyperfiltration is likely to be an increased glomerular capillary pressure and glomerular plasma flowCitation7 which could be due to a defect in the autoregulatory mechanism, as enhanced systemic and renal perfusion pressures are associated with preglomerular vasoconstriction in order to keep the GFR at a constant rate.Citation31 Furthermore, it was also found that the SHR diabetic group exhibited a decreased renal cortical blood perfusion compared with the SHR control group. The results of this study can be best explained on the basis of augmented renovascular resistance probably due to the hyperglycemic-induced activation of local ANG II.Citation32 The administration of exogenous H2S caused plasma creatinine concentration in the treated rats to decrease, but creatinine clearance and renal cortical blood perfusion were significantly higher compared with the SHR diabetic control. An increase in creatinine clearance and renal cortical blood perfusion can be explained on the basis of a vasodilator effect of H2S possibly causing the dilatation of afferent arterioles. The findings are consistent with a previous report indicating that exogenously administered H2S produces a greater vasodilatation of preglomerular arterioles than postglomerular arterioles.Citation23 However, Sprague Dawley (SD) normotensive and nondiabetic rats were used in that study.Citation23 The evidence of this study and previous reports would support the view that the exogenous H2S causes the relaxation of renal blood vessels in a setting of increased renovascular resistance. However, the above suggestion requires further exploration.
The RAAS plays a fundamental role in the development of diabetic nephropathy.Citation33–35 Hyperglycemia-mediated increased production of ROS is responsible for the upregulation of RAAS.Citation36 Recently, aldosterone acting independently from ANG II has been linked to the development of nephropathy.Citation37 The urinary sodium-to-potassium ratio is a marker of renal reabsorptive function and is inversely proportional to the aldosterone level acting on renal tubules.Citation38,39 In this study, diabetic SHR exhibited a significantly lower urinary sodium-to-potassium ratio indicative of increased aldosterone activity. By contrast, treating the rats with exogenous H2S increased the sodium-to-potassium ratio. This would suggest that exogenous H2S decreased aldosterone activity either indirectly via the inhibition of angiotensin-converting enzymeCitation40 or directly via antagonism of aldosterone. However, a possible direct antagonism of aldosterone action by H2S needs to be further investigated.
The complex interaction between vasodilator and vasoconstrictor substances plays an important role in preserving the vascular wall integrity functionally as well as structurally.Citation41 H2S has been shown to be a vasodilator molecule because by using the gas in water or by using the NaHS (as a donor of H2S) there was a vasodilatation of different blood vessels, including rat aorta, portal vein, mesenteric, and hepatic arteries.Citation42 In this study, systolic blood pressure and mean arterial blood pressure of the SHR group were significantly higher compared with the WKY control which was associated with decreased plasma and urinary levels of H2S. These findings imply that H2S is a vasodilator molecule contributing to the maintenance of the normotensive state and is in line with a previous report suggesting that the downregulation of CSE/H2S system is involved in the development of spontaneous hypertension.Citation15 Endothelial dysfunction is considered to be the first step in the pathogenesis of micro- and macrovascular complications in hypertension and diabetes.Citation43 Hyperglycemia associated with diabetes modifies endothelial function through a number of complex mechanisms, including oxidative stress,Citation44 glycation of protein and lipids,Citation45 and activation of protein kinase C.Citation46 Recently, the vascular endothelium was reported to express 3-mercaptopyruvate sulfurtransferase and cysteine aminotransferase which produce H2S from cysteine and α-ketoglutarateCitation47 suggesting an alternative source of H2S rather than vascular smooth muscle cells. In this study, plasma and urinary levels of H2S in the diabetic rats were significantly decreased in later part of this study without changes in blood pressure as compared with the SHR control group which concurs with an earlier study.Citation48 This was possibly due to the endothelial dysfunction which is a common occurrence in diabetes.Citation43,49 The possible mechanisms of reduced plasma and urinary H2S in hypertensive diabetic rats include the persistent hyperglycemia, as found in this study, and increased levels of ROS due to enhanced formation of free radicals. The augmented production of ROS might have then resulted in oxidative stress and compromised endothelial function.Citation49 Moreover, treating the diabetic hypertensive rats with NaHS (donor of exogenous H2S) reduced the systolic and mean arterial blood pressures and increased plasma and urinary H2S levels. This may be explained on the basis of earlier findings that exogenous H2S relaxes vascular smooth muscle cells through the opening of KATP channels while the administration of NaHS possibly upregulates CSE enzyme activity resulting in increased endogenous H2S production.Citation50 However, the measurement of CSE messenger ribonucleic acid (mRNA) by quantitative reverse transcription polymerase chain reaction should be done in order to comment precisely on the downregulation and upregulation of CSE/H2S system. Alternatively, the increased plasma and urinary H2S levels in diabetic-treated rats suggests that exogenous H2S might have improved endothelial function due to its reducing oxidative stress property as it has been reported to be a potent antioxidant molecule.Citation51,52 Nevertheless, the above hypothesis deserves further verification.
CONCLUSION
The data obtained from this study showed that decreasing the blood pressure by the use of exogenous H2S in the form of NaHS significantly attenuated the progression of early diabetic nephropathy in SHR diabetic rats. On the basis of the above findings, it has been suggested that the pharmacological augmentation of H2S may have the beneficial effect in the progression of nephropathy in a combined state of hypertension and diabetes.
Declaration of interest: The authors report no conflicts of interest. The authors alone are responsible for the content and writing of the paper.
REFERENCES
- National High Blood Pressure Education Program Working Group. Hypertension. 1994;23:145–158.
- Contreras F, Rivera M, Vasquez J, De la Parte MA, Velasco M. Endothelial dysfunction in arterial hypertension. J Hum Hypertens. 2000;14:S20–S25.
- Ogihara T, Asano T, Ando K, . Angiotensin II-induced insulin resistance is associated with enhanced insulin signaling. Hypertension. 2002;40:872–879.
- Blendea MC, Jacobs D, Stump CS, . Abrogation of oxidative stress improves insulin sensitivity in the Ren-2 rat model of tissue angiotensin II overexpression. Am J Physiol Endocrinol Metab. 2005;288:E353–E359.
- Sowers JR. Insulin resistance and hypertension. Am J Physiol Heart Circ Physiol. 2004;286:H1597–H1602.
- McFarlane SI, Sowers JR. Special features—CARDIOVASCULAR ENDOCRINOLOGY 1: aldosterone function in diabetes mellitus—effects on cardiovascular and renal disease. J Clin Endocrinol Metab. 2003;88:516–523.
- Wolf G. New insights into the pathophysiology of diabetic nephropathy: From hemodynamics to molecular pathology. Eur J Clin Invest. 2004;34:785–796.
- Tesch GH, Allen TJ. Rodent models of streptozotocin induced diabetic nephropathy (methods in renal research). Nephrology. 2007;12:261–266.
- Allen TJ, Cao Z, Youssef S, Hulthen UL, Cooper ME. Role of angiotensin II and bradykinin in experimental diabetic nephropathy. Functional and structural studies. Diabetes. 1997;46:1612–1618.
- Cooper ME, Allen TJ, Jerums G, Doyle AE. Accelerated progression of diabetic nephropathy in the spontaneously hypertensive streptozotocin diabetic rat. Clin Exp Pharmacol Physiol. 1986;13:655–662.
- Wang RUI. Two’s company, three’s a crowd: Can H2S be the third endogenous gaseous transmitter? FASEB J. 2002;16:1792–1798.
- Zhao W, Zhang J, Lu Y, Wang R. The vasorelaxant effect of H2S as a novel endogenous gaseous KATP channel opener. EMBO J. 2001;20:6008–6016.
- Stipanuk MH, Beck PW. Characterization of the enzymic capacity for cysteine desulphhydration in liver and kidney of the rat. Biochem J. 1982;206:267–277.
- Levonen AL, Lapatto R, Saksela M, Raivio KO. Human cystathionine gamma-lyase: Developmental and in vitro expression of two isoforms. Biochem J. 2000;347:291–295.
- Yan H, Du J, Tang C. The possible role of hydrogen sulfide on the pathogenesis of spontaneous hypertension in rats. Biochem Biophys Res Commun. 2004;313:22–27.
- Yang Y, Geng X, Tang C. Hydrogen sulfide system in the pathogenesis of renovascular hypertension in rats. J Geriatr Cardiol. 2008;5:1–5.
- Zhong GZ, Chen FR, Cheng YQ, Tang CS, Du JB. The role of hydrogen sulfide generation in the pathogenesis of hypertension in rats induced by inhibition of nitric oxide synthase. J Hypertens. 2003;21:1879–1885.
- Yuan P, Xue H, Zhou L, Rescue of mesangial cells from high glucose-induced over-proliferation and extracellular matrix secretion by hydrogen sulfide. Nephrol Dial Transplant. 2011;26(7):2119–2126.
- Ramesh B, Pugalendi KV. Antioxidant role of umbelliferone in STZ-diabetic rats. Life Sci. 2006;79:306–310.
- Suckow MA, Weisbroth SH, Franklin CL. The Laboratory Rat. Washington, DC: Academic Press; 2006:655.
- Lee TN, Alborn WE, Knierman MD, Konrad RJ. The diabetogenic antibiotic streptozotocin modifies the tryptic digest pattern for peptides of the enzyme O-GlcNAc-selective N-acetyl-[beta]-d-glucosaminidase that contain amino acid residues essential for enzymatic activity. Biochem Pharmacol. 2006;72:710–718.
- Wong KK, Tzeng ES. Appearance of different diabetic symptoms after streptozocin administration: A comparison study. Biochem Mol Biol Int. 1993;30:1035–1041.
- Xia M, Chen L, Muh RW, Li PL, Li N. Production and actions of hydrogen sulfide, a novel gaseous bioactive substance, in the kidneys. J Pharmacol Exp Ther. 2009;329:1056–1062.
- Thomson SC, Vallon V, Blantz RC. Kidney function in early diabetes: The tubular hypothesis of glomerular filtration. Am J Physiol Renal Physiol. 2004;286:8–15.
- Montilla P, Barcos M, Munoz MC, Bujalance I, Munoz-Castaneda JR, Tunez I. Red wine prevents brain oxidative stress and nephropathy in streptozotocin-induced diabetic rats. J Biochem Mol Biol. 2005;38:539–544.
- Chen H, Brahmbhatt S, Gupta A, Sharma AC. Duration of streptozotocin-induced diabetes differentially affects p 38-mitogen-activated protein kinase (MAPK) phosphorylation in renal and vascular dysfunction. Cardiovasc Diabetol. 2005;4:3.
- Khan MAH, Sattar MA, Abdullah NA, . Functional subtypes of renal-adrenoceptor in spontaneously hypertensive rats with streptozotocin-induced experimental diabetic nephropathy. Kidney Blood Press Res. 2009;32:349–359.
- Casey RG, Joyce M, Roche-Nagle G, Chen G, Bouchier-Hayes D. Pravastatin modulates early diabetic nephropathy in an experimental model of diabetic renal disease. J Surg Res. 2005;123:176–181.
- Vallon V, Osswald H. Dipyridamole prevents diabetes-induced alterations of kidney function in rats. Naunyn Schmied Arch Pharmacol. 1994;349:217–222.
- Hostetter TH. Hyperfiltration and glomerulosclerosis. Semin Nephrol. 2003;23:194–199.
- Ruggenenti P, Schieppati A, Remuzzi G. Progression, remission, regression of chronic renal diseases. Lancet. 2001;357:1601–1608.
- Arima S, Ito S. The mechanisms underlying altered vascular resistance of glomerular afferent and efferent arterioles in diabetic nephropathy. Nephrol Dial Transplant. 2003;18:1966–1969.
- Wolf G, Ziyadeh FN. The role of angiotensin II in diabetic nephropathy: Emphasis on nonhemodynamic mechanisms* 1. Am J Kidney Dis. 1997;29:153–163.
- Vidotti DB, Casarini DE, Cristovam PC, Leite CA, Schor N, Boim MA. High glucose concentration stimulates intracellular renin activity and angiotensin II generation in rat mesangial cells. Am J Physiol Renal Physiol. 2004;286:F1039–F1045.
- Singh R, Singh AK, Alavi N, Leehey DJ. Mechanism of increased angiotensin II levels in glomerular mesangial cells cultured in high glucose. J Am Soc Nephrol. 2003;14:873–880.
- Hsieh TJ, Zhang SL, Filep JG, Tang SS, Ingelfinger JR, Chan JSD. High glucose stimulates angiotensinogen gene expression via reactive oxygen species generation in rat kidney proximal tubular cells. Endocrinology. 2002;143:2975–2986.
- Zhang SL, To C, Chen X, . Essential role (s) of the intrarenal renin-angiotensin system in transforming growth factor–ß1 gene expression and induction of hypertrophy of rat kidney proximal tubular cells in high glucose. J Am Soc Nephrol. 2002;13:302–312.
- Alexander WD, Branch RA, Levine DF, Hartog M. The urinary sodium: Potassium ratio and response to diuretics in resistant oedema. Postgrad Med J. 1977;53:117–121.
- Edmonds CJ, Wilson GM. The action of hydroflumethiazide in relation to adrenal steroids and potassium loss. Lancet. 1960;1:505–509.
- Laggner H, Hermann M, Esterbauer H, . The novel gaseous vasorelaxant hydrogen sulfide inhibits angiotensin-converting enzyme activity of endothelial cells. J Hypertens. 2007;25:2100–2104.
- Raij L. Workshop: Hypertension and cardiovascular risk factors. Role of the angiotensin II-nitric oxide interaction. Hypertension. 2001;37:767–773.
- Li L, Moore PK. Putative biological roles of hydrogen sulfide in health and disease: A breath of not so fresh air? Trends Pharmacol Sci. 2008;29:84–90.
- Wong WT, Wong SL, Tian XY, Huang Y. Endothelial dysfunction: The common consequence in diabetes and hypertension. J Cardiovasc Pharmacol. 2010;55:300–307.
- Laight DW, Carrier MJ, Änggård EE. Antioxidants, diabetes and endothelial dysfunction. Cardiovasc Res. 2000; 47:457–464.
- Vlassara H, Fuh H, Makita Z, Krungkrai S, Cerami A, Bucala R. Exogenous advanced glycosylation end products induce complex vascular dysfunction in normal animals: A model for diabetic and aging complications. Proc Natl Acad Sci USA. 1992;89:12043–12047.
- Hink U, Li H, Mollnau H, . Mechanisms underlying endothelial dysfunction in diabetes mellitus. Circ Res. 2001;88:e14–e22.
- Shibuya N, Mikami Y, Kimura Y, Nagahara N, Kimura H. Vascular endothelium expresses 3-mercaptopyruvate sulfurtransferase and produces hydrogen sulfide. J Biochem. 2009;146:623–626.
- Jain SK, Bull R, Rains JL, . Low levels of hydrogen sulfide in the blood of diabetes patients and streptozotocin-treated rats causes vascular inflammation? Antioxid Redox Signal. 2010;12:1333–1337.
- De Vriese AS, Verbeuren TJ, Van de Voorde J, Lameire NH, Vanhoutte PM. Endothelial dysfunction in diabetes. Br J Pharmacol. 2000;130:963–974.
- Chunyu Z, Junbao D, Dingfang B, Hui Y, Xiuying T, Chaoshu T. The regulatory effect of hydrogen sulfide on hypoxic pulmonary hypertension in rats* 1. Biochem Biophys Res Commun. 2003;302:810–816.
- Kimura Y, Kimura H. Hydrogen sulfide protects neurons from oxidative stress. FASEB J. 2004;18:1165–1167.
- Hong-ling WEI, Zhang CY, Hong-fang JIN, Chao-Shu T, Jun-bao DU. Hydrogen sulfide regulates lung tissue-oxidized glutathione and total antioxidant capacity in hypoxic pulmonary hypertensive rats. Acta Pharmacol Sin. 2008;29:670–676.