Abstract
Rhabdomyolysis is an important cause of acute renal failure (ARF) and renal vasoconstriction is the main mechanism in the pathogenesis of ARF. Lipid peroxidation due to hydroxyl radical (.OH) formation and redox cycling of myoglobin also have a role. We investigated the disturbance in renal vascular reactivity to reveal the mechanisms leading to ARF. Female Wistar rats (n = 7) were injected with glycerol (10 mL/kg, 50% in saline) intramuscularly to induce rhabdomyolysis, and then the kidneys were isolated and perfused. We investigated acetylcholine (ACh)-induced endothelium-dependent and papaverine (PAP)-induced endothelium-independent vasodilation responses and renal nerve stimulation (RNS)-induced vasoconstrictions. These were also investigated both in rats which received either .OH scavenger, dimethylthiourea (DMTU: 500 mg/kg before glycerol injection and 125 mg/kg 8 h after glycerol injection, n = 7), or myoglobin redox cycling inhibitor, acetaminophen (ApAP: 100 mg/kg 2 h before glycerol injection and 100 mg/kg each 4 h, and 22 h after glycerol injection, n = 7). ACh-induced responses in glycerol group were decreased (p < 0.001), but PAP-induced vasodilation did not change. RNS-induced vasoconstriction in all kidneys was greater (p < 0.001) in glycerol group. DMTU restored both endothelium-dependent vasodilation and RNS-induced vasoconstriction. ApAP had no effect on vascular responses. Both DMTU and ApAP exerted a partial protective effect in renal histology without restoring serum creatinine and blood urea nitrogen (BUN) levels or creatinine clearance. This study showed that endothelial dysfunction and increased vasoconstriction developed during rhabdomyolysis. .OH plays an important role in the development of these vascular responses. These findings suggest that decreased endothelium-dependent vasodilation and augmented renal sympathetic tonus contribute to the development of renal vasoconstriction during rhabdomyolysis-induced ARF.
INTRODUCTION
Rhabdomyolysis is one of the leading causes of acute renal failure (ARF) and accounts for 5–7% of all ARF cases.Citation1 Since Bywaters and Beall first described the association between crush injury-induced rhabdomyolysis and ARF in 1941,Citation2 several studies have been done to elucidate the pathophysiology. These studies revealed three main mechanisms: renal vasoconstriction, intraluminal cast formation, and toxicity of myoglobin; among them renal vasoconstriction is the most noteworthy.Citation3 Several factors, such as nitric oxide (NO) scavenging effect of myoglobin and intravascular volume loss, account for the development of renal vasoconstriction.Citation3 Endothelial dysfunction, which is common in other forms of ARF,Citation4 is also suggested to be responsible for the development of renal vasoconstriction.Citation5 However, these mechanisms are still remote from fully explaining the development and final outcome of this pathology.
Alteration of the renal vascular tonus appears to be an important contributor of renal physiology both in health and in disease. Endothelium plays a crucial role in controlling vascular tonus. There are various vasodilator and vasoconstrictor substances originating from endothelium and normally there is a delicate balance between them.Citation6 In endothelial dysfunction this balance is disturbed favoring vasoconstriction.Citation7 Endothelial dysfunction is described as impaired vasodilation in response to endothelium-dependent vasodilators (acetylcholine [ACh], bradykinin) and plays important roles in the pathogenesis of various diseases (sepsis, diabetes mellitus, and hypertension) including ischemic renal failure.Citation8–10 NO plays a key role in endothelium-dependent vasodilation.Citation4 It is constitutively synthesized mostly by endothelial nitric oxide synthase (eNOS) in endothelial cells and the most important activator of eNOS is shear stress.Citation11 NO is tonically released by endothelium and there is a vasodilatory tone on the vascular system.Citation12 During NOS inhibition, renal blood flow (RBF), glomerular filtration rate, and urine output are decreased.Citation13,14 These findings show that NO has a tonic role in the control of RBF and glomerular filtration, and the presence of endothelial dysfunction in ARF may explain the development of renal vasoconstriction. Previous studies revealed impaired endothelium-dependent vasodilation in intact animals and isolated aortic rings during rhabdomyolysis-induced ARF.Citation15 To our knowledge, endothelium-dependent vasodilation in kidneys, in which vasoconstriction plays a key role in tissue injury, during rhabdomyolysis-induced ARF has not been investigated previously.
Kidneys are densely innervated by sympathetic nerves and electrical stimulation of these nerves leads to vasoconstriction.Citation16,17 Development of renal vasoconstriction during rhabdomyolysis may be due to augmentation of vasoconstrictive responses by renal nerve stimulation (RNS). This condition is reported to play an important role in the pathogenesis of ischemic renal failure.Citation4 Alterations in renal vascular responses by RNS during rhabdomyolysis-induced ARF have not been evaluated in previous studies.
It has been reported that rhabdomyolysis initiates liberation of excessive amounts of myoglobin from skeletal muscles into systemic circulation which leads to nephrotoxicity.Citation3 Myoglobin exerts its toxic effect by two main mechanisms. The first mechanism is related to liberation of free iron (Fe+2), leading to the formation of toxic and reactive hydroxyl radical (.OH) by Haber–Weiss reaction.Citation18 Dimethylthiourea (DMTU), a ·OH scavenger, was reported to prevent rhabdomyolysis-induced ARF.Citation19 The second mechanism is related to the entrance of heme group of myoglobin into the redox cycle, inducing lipid peroxidation.Citation20 Recently, acetaminophen (ApAP) is shown to inhibit redox cycling and prevent the development of ARF in rhabdomyolysis.Citation21
In this study, we tested the hypothesis that impaired renal endothelium-dependent vasodilation and enhanced vasoconstrictive responses to RNS are responsible for the development of exaggerated renal vasoconstriction in rhabdomyolysis-induced ARF. Therefore, we investigated the alterations of these vascular responses in isolated perfused kidneys in a glycerol-induced rhabdomyolysis model of ARF in rats. We also investigated the possible recovering effects of DMTU and ApAP treatments on these responses to further elucidate the pathogenesis of alterations in renal vascular reactivity.
RESULTS
With a constant perfusion rate of 5 mL/min, the kidneys displayed basal perfusion pressures of ∼40–50 mmHg (). After the stabilization period, RNS was applied. To investigate vasodilation responses, the perfusion pressure was elevated with 3 μM phenylephrine (PE). The kidneys responded to PE with a rapid elevation in perfusion pressure. Peak perfusion pressure displayed about 10% reduction and this stabilized level was mostly maintained throughout the experiments. Maximum and stabilized elevations of perfusion pressure in response to PE were similar among groups ().
Table 1. Basal perfusion pressure, maximum change, and stabilized change in perfusion pressure in response to 3 μM PE.
Vasoconstrictor Responses Induced by Renal Nerve Stimulation
All kidneys responded to RNS by a frequency-dependent increase in perfusion pressure. RNS responses of glycerol group were ∼100% higher than controls ( and ; n = 7 for each group, p < 0.001). The kidneys obtained from glycerol + DMTU-treated rats displayed RNS-induced vasoconstriction which was not different from controls and was significantly lower than that of glycerol group (n = 7, p < 0.001). DMTU alone did not appreciably alter RNS responses (n = 7). The RNS responses of glycerol + ApAP group were higher than controls (n = 7, p < 0.001). These results imply that RNS-induced vasoconstriction is augmented during rhabdomyolysis-induced ARF. Restoration of these increased RNS responses by DMTU, but not with ApAP, suggests that .OH plays an important role in the development of this condition.
Figure 1. Representative traces of renal nerve stimulation (RNS)-induced vasoconstriction responses in control group and glycerol-treated rats.
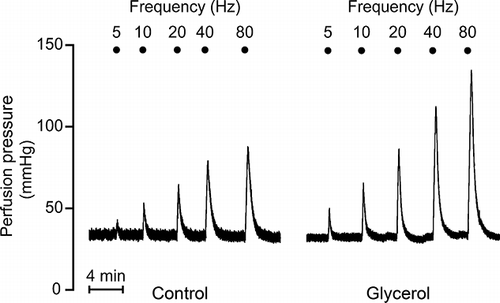
Figure 2. Renal nerve stimulation (RNS) responses of control group, glycerol-injected rats, and dimethylthiourea or acetaminophen-treated rats. Data were presented as mmHg (A) and percentage of 3 μM phenylephrine (PE)-induced maximum change in perfusion pressure (B). DMTU, dimethylthiourea; ApAP, acetaminophen; ns, not significant. The values are expressed as mean ± SEM. n = 7 for each group.
Note: *p < 0.05, **p < 0.01, ***p < 0.001.
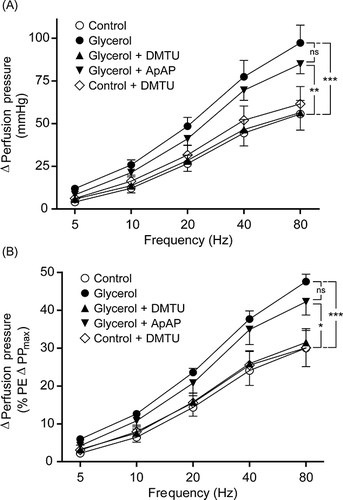
Renal Vasodilation Responses
All kidneys responded to ACh with a dose-dependent decrease in PE-elevated perfusion pressure. ACh-induced vasodilation of glycerol group was significantly lower than that of controls ( and ; n = 7 for each group, p < 0.001). After DMTU, the kidneys from glycerol-injected rats had ACh responses which were higher than that of glycerol group (n = 7, p < 0.001). DMTU alone had no effect on ACh responses (n = 7). On the other hand, ApAP treatment was not as effective as DMTU. Following ApAP treatment the ACh-induced vasodilation observed in kidneys obtained from glycerol-injected animals was not different from glycerol-injected rats alone, but lower than that of controls (n = 7, p < 0.001).
Figure 3. Representative traces of acetylcholine and papaverine-induced vasodilation in control group (A) and glycerol-treated rats (B). ACh, acetylcholine; PAP, papaverine; PE, phenylephrine.
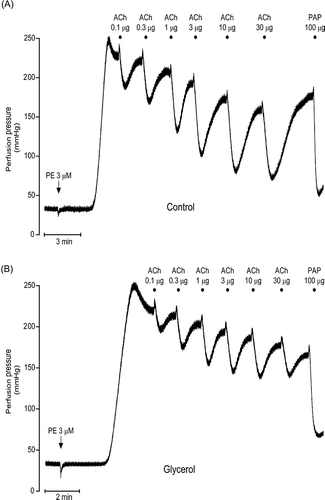
Figure 4. Acetylcholine-induced vasodilation responses of control group, glycerol-injected rats, and dimethylthiourea or acetaminophen-treated rats. Data were presented percentage of 3 μM phenylephrine (PE)-induced stabilized change in perfusion pressure. DMTU, dimethylthiourea; ApAP, acetaminophen; ACh, acetylcholine; ns, not significant. The values are expressed as mean ± SEM. n = 7 for each group.
Note: **p < 0.01, ***p < 0.001.
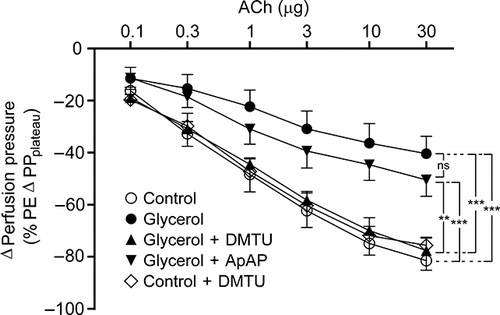
Following the responses elicited by ACh, we tested the kidneys for their responsiveness to papaverine (PAP), an endothelium-independent vasodilator. In kidneys from all groups, PAP induced a prompt decrease which reached to about 85% of PE-induced elevation of perfusion pressure which was similar in all groups ( and ). In another group of experiments endothelium denudation was performed by a bolus injection of Triton X (0.1 mL of 0.2% solution in saline).Citation22 Similar to kidneys of glycerol group, endothelium-denuded kidneys did not respond to ACh, but PAP still induced a significant vasodilation (data not shown). These findings suggest that the vasodilation capacity of the kidneys was not impaired in rhabdomyolysis-induced ARF and that the impaired renal vasodilation to ACh results from disturbed vasodilatory function of renal endothelium. Since DMTU, but not ApAP, restored impaired endothelium-dependent vasodilation, .OH again seems to contribute to the development of this aberrant vascular reactivity.
Figure 5. Papaverine (PAP)-induced maximum vasodilation responses of control group, glycerol-injected rats, and dimethylthiourea or acetaminophen-treated rats. Data were presented percentage of 3 μM phenylephrine (PE)-induced stabilized change in perfusion pressure. DMTU, dimethylthiourea; ApAP, acetaminophen. The values are expressed as mean ± SEM. n = 7 for each group.
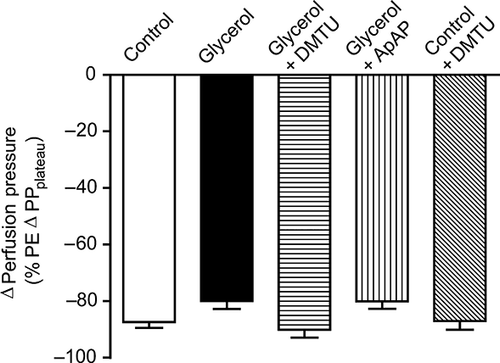
Histopathologic Examination
We examined the histological changes of kidneys and these results are presented in and . Glycerol injection resulted in severe necrosis (p < 0.001) (nuclear disappearance–fragmentation, brush border loss, apical blebbing, hyalinization, and tubular flattening) and cast formation (n = 7, p < 0.05) in cortical tubules. Both DMTU (n = 7, p < 0.01) and ApAP (n = 7, p < 0.001) effectively decreased the prevalence of necrotic tubules, but not cast formation in glycerol group.
Figure 6. Histological appearances of kidneys from control group, glycerol-injected rats, and dimethylthiourea or acetaminophen-treated rats. DMTU, dimethylthiourea; ApAP, acetaminophen. Hematoxylin and eosin, ×100 magnification.
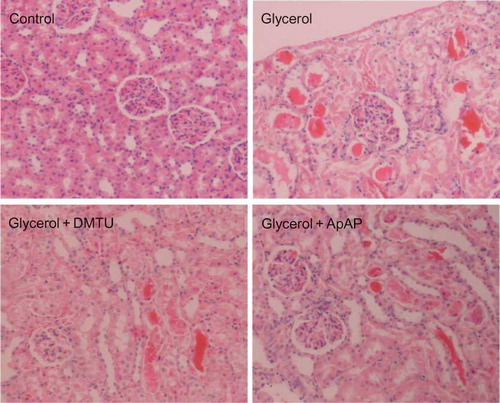
Figure 7. Necrotic changes (A) and cast formation (B) in renal cortical tubules of control group, glycerol-injected rats, and dimethylthiourea or acetaminophen-treated rats. Data were presented as percentage of total counted tubules. DMTU, dimethylthiourea; ApAP, acetaminophen; ns, not significant. The values are expressed as mean ± SEM. n = 7 for each group.
Note: *p < 0.05, **p< 0.01, ***p< 0.001.
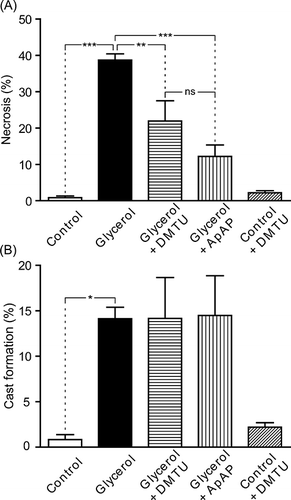
Kidney Function Tests
Kidney function tests are presented in . Glycerol injection caused a marked increase in serum creatinine (p < 0.001) and blood urea nitrogen (BUN) levels (p < 0.001) and a decrease in creatinine clearance (p < 0.001). We did not observe mortality due to glycerol injection. Neither DMTU nor ApAP had a protective effect on serum creatinine, BUN levels, or creatinine clearance.
Figure 8. Serum creatinine (A) level, blood urea nitrogen (BUN) (B) level, and creatinine clearance (C) of control group, glycerol-injected rats, and dimethylthiourea or acetaminophen-treated rats. DMTU, dimethylthiourea; ApAP, acetaminophen; ns, not significant. The values are expressed as mean ± SEM. n = 7 for each group.
Note: ***p < 0.001.
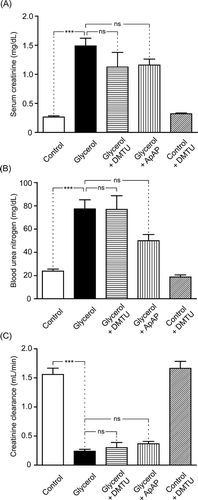
DISCUSSION
In this study we have shown, for the first time, the development of renal endothelial dysfunction during rhabdomyolysis-induced ARF. Concomitant with this endothelial predicament, RNS induced greater vasoconstrictor responses in the kidney vasculature. These findings may help to explain the development of renal vasoconstriction, which is considered to play a key role in the pathogenesis of this disease. Restoration of both of these responses by DMTU, but not by ApAP, strongly suggests that .OH plays a critical role in the development of endothelial dysfunction and enhanced vasoconstriction in response to RNS in this disease.
Under physiological conditions, endothelium exerts a robust vasodilatory tone in the kidneys, which plays a crucial role in the maintenance of RBF and glomerular filtration.Citation23 Disturbance of this tonus can lead to renal vasoconstriction and impaired glomerular filtration, since isolated perfused kidney reflects the global vascular reactivity of the renal tissue and globally decreased vasodilation can lead to the pathologies above. Three main factors—NO scavenging effect of myoglobin, renal ischemia, and .OH toxicity—contribute to the development of endothelial dysfunction in ARF. Myoglobin has NO scavenging effect and since endothelium-dependent vasodilation strongly depends on NO, alteration in the nitrergic tone may explain the development of endothelial dysfunction. It has been shown that in patients with malaria, serum-free hemoglobin levels are inversely correlated with endothelium-dependent vasodilation.Citation24 The fact that hemoglobin has NO scavenging effect akin to that of myoglobin and the fact that both of these heme proteins induce similar toxic effects in kidney may account for the endothelial dysfunction.
Trifillis et al.Citation25 demonstrated prompt development of renal ischemia at an early period after glycerol injection. Endothelial dysfunction could also result from renal ischemia, since ischemia was shown to cause endothelial dysfunction in various vascular beds, including kidneys, and in different models of ARF.Citation26,27
Hydroxyl radical plays an important role in the development of endothelial dysfunction in various diseases. It was shown that endothelial dysfunction develops when coronary arteries are exposed to .OH, and .OH scavengers restore endothelium-dependent vasodilation.Citation28,29 DMTU is an effective .OH scavenger and was also shown to restore endothelium-dependent vasodilation in various vessels of diabetic rats,Citation30–33 but this effect has not been investigated previously in ARF. In this study, we showed that DMTU treatment completely restored impaired endothelium-dependent vasodilation in rhabdomyolysis-induced ARF. Our findings strongly suggest that .OH plays an important role in the development of renal endothelial dysfunction in this model. Although we did not investigate the contribution of other factors, since DMTU completely restored endothelium-dependent vasodilation, we concluded that .OH radical is the major contributor.
In this study, vasoconstrictor response to a submaximal concentration of PE is shown to be similar in both control and glycerol groups. It was shown that renal responses to noradrenaline (NA) seem unchanged in a glycerol model.Citation34 Therefore, enhanced vasoconstrictor response to alpha-adrenergic stimulation seems unlikely. On the contrary, during RNS, neuropeptide Y (NPY) and ATP are reported to be released from sympathetic nerves together with NA and these substances lead to vasoconstriction.Citation35,36 Enhanced vasoconstriction responses to NPY and ATP may explain our findings, but there are no data regarding our assumption in the literature. In a study performed in glycerol model, renal NA and NPY immunoreactivity were shown to be decreased, suggesting degeneration of sympathetic nerves.Citation37 On the contrary, we demonstrated that RNS resulted in about a twofold increase in vasoconstrictor responses in ARF rats. This finding strongly implies that alteration of vascular reactivity of the ARF kidneys is related to presynaptic release mechanisms. Among these mechanisms, NO was shown to facilitate NA release and NOS inhibition was shown to decrease NA release from sympathetic nerves in rat kidneys.Citation38 Since myoglobin scavenges NO, increased NA release from adrenergic nerves seems to be unlikely to explain increased vasoconstrictor response to RNS in our model. Although NOS inhibition was reported to decrease presynaptic NA release, RNS-induced vasoconstrictor responses were also shown to be increased in the same study.Citation38 Additionally, endothelium denudation and guanylate cyclase inhibition were shown to increase sympathetic nerve stimulation-induced vasoconstriction in rabbit carotid arteries,Citation39 suggesting that endothelium and NO modulate sympathetic nerve stimulation responses and that increased RNS responses are due to endothelial dysfunction.
Complete restoration of RNS responses by DMTU suggests that .OH plays an important role in the pathogenesis of ARF. In a study performed on spontaneously hypertensive rats, the levels of .OH and other reactive oxygen metabolites were found to be increased in rostral ventrolateral medulla (RVLM) and antioxidant tempol was shown to reduce blood pressure and sympathetic overactivity. Tempol had no effect on these parameters in normotensive rats.Citation40 We demonstrated that DMTU treatment decreased RNS responses in glycerol-injected rats, but not in control rats. The effect of DMTU on glycerol-injected kidneys may be related to increased .OH in RVLM during rhabdomyolysis-induced ARF.
In previous studies utilizing the glycerol model, it has been shown that renal vasoconstriction develops a few hours after glycerol injection and RBF returns to normal levels 24 h after glycerol injection.Citation41–43 Our findings that basal perfusion pressures of glycerol-treated kidneys 24 h after glycerol injection are similar to controls are consistent with these studies. Although RBF returns to normal levels, glomerular filtration is still impaired 24 h after glycerol injection.Citation42,44 Increased preglomerular resistance and possible decreased postglomerular resistance are held responsible for this filtration failure.Citation44–46 Thus, our findings may explain the development of renal vasoconstriction and impaired glomerular filtration during rhabdomyolysis.
We clearly demonstrated that DMTU treatment restored the histopathological changes induced by ARF without appreciable improvement in kidney function. Shah et al.Citation19 showed that DMTU and sodium benzoate (.OH scavengers) had protective effects both in renal histology and in kidney function in glycerol-induced ARF model. Shah et al. used male rats, performed 24 h dehydration before glycerol injection, and used a lower glycerol dose (8 mL/kg). Our DMTU dosing regimen was similar to this study. Zager et al.Citation47 showed that sodium benzoate had no effect on renal histology or kidney function tests in glycerol model. Zager et al. used female rats, did not perform dehydration, and used a glycerol dose (10 mL/kg) similar to that applied in our study. Boutaud et al.Citation21 recently showed that ApAP had protective effect in renal histology and kidney function tests in glycerol model. They used male rats, did not perform dehydration, and used a glycerol dose (10 mL/kg) similar to our study. These findings suggest that .OH scavengers and ApAP are not always effective in glycerol model and our findings could be explained by differences in study design and/or gender differences.
In this study, we demonstrated that endothelial dysfunction develops in kidney vasculature due to rhabdomyolysis-induced ARF, evidenced by reduced responsiveness to vasodilator action of ACh and an increased responsiveness to RNS. .OH seems to take the major part in the pathologies underlying the development of disturbance in the renal vascular reactivity. Recovering effects of DMTU treatment strongly suggest the critical role of .OH in the development of endothelial dysfunction and enhanced vasoconstriction in response to RNS in ARF. Compounds with .OH-removing properties may be considered as a novel therapeutic approach for the treatment of crush injury-induced ARF.
METHODS
Animals
Female Wistar rats weighing 200–270 g obtained from the Animal Laboratory of Hacettepe University were used in this study. Animals were housed in communal cages with food and water available ad libitum and exposed to a 12 h light/dark cycle maintained at 21°C. The procedures in this study were approved by Hacettepe University Animal Care and Use Ethics Committee (2010/36-1).
Drugs
Glycerol was purchased from Merck KGaA (Darmstadt, Germany); PAP hydrochloride was purchased from Ciba-Geigy (Basel, Switzerland); and ACh chloride, PE hydrochloride, ApAP, DMTU, and Triton X-100 were purchased from Sigma Chemical Company (St. Louis, MO, USA).
Study Design
Rats were randomly divided into five groups. Group 1 was assigned as the control group (n = 7) and received i.p. saline in an equal dose to DMTU administered in groups 3 (glycerol + DMTU) and 5 (control + DMTU). Rhabdomyolysis was induced in groups 2 (glycerol), 3, and 4 (glycerol + ApAP).Citation21,48 Briefly, under diethyl ether anesthesia, 10 mL/kg glycerol (50% vol./vol. in saline) was injected in the hind limb muscles of the rats and half of the dose was administered in each hind limb muscle. Group 2 rats (glycerol, n = 7) received saline solution i.p. in an equal dose to DMTU (20 mg/mL in saline) administered in group 3 immediately after glycerol injection. Group 3 rats (glycerol + DMTU, n = 7) received DMTU (i.p. 500 mg/kg), starting at the same time as the glycerol injection and an additional dose of i.p. 125 mg/kg, 8 h after glycerol injection.Citation19 Group 4 animals (glycerol + ApAP, n = 7) received ApAP (i.p. 100 mg/kg, 10 mg/mL in saline) 2 h before glycerol injection. Rats in this group received two additional doses of ApAP (i.p. 100 mg/kg, each) 4 and 22 h, respectively, after glycerol injection.Citation21 Immediately after glycerol or saline injections, animals were placed into metabolic cages for 24 h urine collection with free access to food and water. To investigate the putative effects of DMTU on renal vascular reactivity in control rats, another group of animals was assigned as group 5 (control + DMTU, n = 7). They received i.p. 500 mg/kg DMTU before and an additional dose of 125 mg/kg 8 h after placing the rats in metabolic cages. After 24 h follow-up period, rats were anesthetized with diethylether and laparotomy was performed by a midline abdominal incision. Blood samples were obtained by intracardiac puncture. Right kidneys were excised for histological examination and left kidneys were isolated for perfusion experiments in all groups of rats. Blood and urine samples were centrifuged immediately (2000g for 10 min at 4°C). Samples were frozen and stored at −80°C until assayed.
Isolated Perfused Kidney Preparation and Perfusion Experiments
After rats were anesthetized with diethyl ether, laparotomy was performed by a midline incision. Left kidney, renal artery, and abdominal aorta were exposed and a 22 G cannula was inserted into the left renal artery through aorta. The kidney was flushed gently with heparinized (100 units/mL) Krebs–Henseleit solution. Then renal vein and ureter were cut proximally, the renal fascia was removed, and the kidney was transferred to an open circuit perfusion system without recirculation. The kidneys were immediately perfused with Krebs–Henseleit solution at 37°C which was aerated with 95% O2 + 5% CO2. The ionic composition of Krebs–Henseleit solution was 118 mM NaCl, 4.7 mM KCl, 2.5 mM CaCl2, 1.2 mM KH2PO4, 1.2 mM MgSO4, 25 mM NaHCO3, and 10 mM glucose. A peristaltic pump (EYELA MP-3, Tokyo Rikakikai Company, Tokyo, Japan) was used for perfusion and the constant rate of perfusion was 5 mL/min. Perfusion pressure (in mmHg) was continuously recorded using a Statham P23AC pressure transducer (Gould Instrument Systems, Cleveland, OH, USA) and Grass Model 79C Polygraph (Grass Instrument Company, Quincy, MA, USA). Data were digitized and analyzed by PowerLab/8SP Data Acquisition System (ADInstruments Incorporation, Colorado Springs, CO, USA) and Chart 5 for Windows (ADInstruments Incorporation, Colorado Springer, CO, USA), respectively. All experiments were performed following a stabilization period of at least 20 min. Since the perfusion rate was kept constant throughout the experiments, an increase in the perfusion pressure was considered as a “vasoconstrictor response” and a decrease in perfusion pressure was considered as “vasodilation.”
Evaluation of Renal Vascular Reactivity
To evaluate the alterations in the renal vascular reactivity of the rat kidneys, RNS was performed. Periarterial renal nerves were stimulated by using bipolar platinum electrodes, Grass S8800 Stimulator, and Grass SIU5 Stimulus Isolation Unit. The stimulation parameters were 100 V, 5 s train, and 2 ms pulse duration. Frequency–response curves were constructed using stimulation frequencies of 5, 10, 20, 40, and 80 Hz. The vascular responses were expressed as both “the maximum increase in perfusion pressure (mmHg) from the baseline” and “the percentage of maximum increase in perfusion pressure induced by 3 μM PE.”
For further evaluation of renal vascular reactivity, ACh- and PAP-induced vasodilation responses were recorded. To investigate vasodilation responses, the kidneys were first vasoconstricted by constant perfusion with Krebs–Henseleit solution containing 3 μM PE (the concentration that induces 75–80% of maximum PE-induced increase in perfusion pressure). After 2 min of stabilization period, ACh was injected into the rubber tubing close to renal artery in doses between 0.1 and 30 μg/0.1 mL to investigate endothelium-dependent vasodilation. After obtaining ACh-induced vascular responses, 100 μg/0.1 mL PAP was injected to examine the relaxation capacity of the kidney vessels. The vasodilation responses were measured as the difference (mmHg) between the lowest level of perfusion pressure achieved after each dose and PE-induced increased perfusion pressure. Then these responses were expressed as the percentage of PE-induced increase in perfusion pressure.
Renal Function
The serum and urine samples were used to assess renal function. Serum creatinine, BUN, and urine creatinine levels were measured and creatinine clearance was calculated. Creatinine and BUN levels were determined by a quantitative colorimetric assay (BioAssay Systems, Hayward, CA, USA). Creatinine clearance was calculated as mL/min.
Kidney Histology
Right kidneys were cut longitudinally and fixed in 10% formalin. Tissues were embedded in paraffin wax, and 5 μm sections were stained with hematoxylin and eosin. The extent of cortical tubular necrosis and that of intraluminal cast formation for each rat were evaluated semi-quantitatively by a pathologist (SG) analyzing the kidney sections without prior knowledge of the treatment group. Using Olympus BX-51 microscope (Olympus Optical Company, Tokyo, Japan) with a special ocular (U-OCMC 10/100XY, Olympus Optical Company) at ×40 magnification, 10 different areas in each kidney were examined and the percentages of necrotic tubules and cast formation were calculated.
Statistical Analysis
Data were expressed as mean ± SEM. Vasoconstriction induced by RNS and vasodilation elicited by ACh administration were analyzed with two-way analysis of variance (ANOVA); all other measurements were analyzed with one-way ANOVA and post hoc Bonferroni multiple comparisons test. For all data sets, p < 0.05 was accepted to be statistically significant.
ACKNOWLEDGMENT
This study was supported by Hacettepe University Scientific Research Unit (010 D09 101 005).
Declaration of interest: The authors report no conflicts of interest. The authors alone are responsible for the content and writing of the paper.
REFERENCES
- Holt S, Moore K. Pathogenesis and treatment of renal dysfunction in rhabdomyolysis. Intensive Care Med. 2001;27(5):803–811.
- Bywaters E, Beall D. Crush injuries with impairment of renal function. 1941 [classical article]. J Am Soc Nephrol. 1998;9(2):322–332.
- Zager RA. Rhabdomyolysis and myohemoglobinuric acute renal failure. Kidney Int. 1996;49(2):314–326.
- Bonventre JV, Weinberg JM. Recent advances in the pathophysiology of ischemic acute renal failure. J Am Soc Nephrol. 2003;14(8):2199–2210.
- Bosch X, Poch E, Grau JM. Rhabdomyolysis and acute kidney injury. N Engl J Med. 2009;361(1):62–72.
- Fatehi-Hassanabad Z, Chan CB, Furman BL. Reactive oxygen species and endothelial function in diabetes. Eur J Pharmacol. 2010;636(1–3):8–17.
- Raij L. Mechanisms of vascular injury: The emerging role of the endothelium. J Am Soc Nephrol. 1991;2(2):S2–S8.
- Guan Z, Gobe G, Willgoss D, Endre ZH. Renal endothelial dysfunction and impaired autoregulation after ischemia-reperfusion injury result from excess nitric oxide. Am J Physiol Renal Physiol. 2006;291(3):F619–F628.
- Molitoris BA, Sandoval R, Sutton TA. Endothelial injury and dysfunction in ischemic acute renal failure. Crit Care Med. 2002;30(5):S235–S240.
- Conger JD, Robinette JB, Hammond WS. Differences in vascular reactivity in models of ischemic acute renal failure. Kidney Int. 1991;39(6):1087–1097.
- Deanfield JE, Halcox JP, Rabelink TJ. Endothelial function and dysfunction: Testing and clinical relevance. Circulation. 2007;115(10):1285–1295.
- Corson MA, James NL, Latta SE, Nerem RM, Berk BC, Harrison DG. Phosphorylation of endothelial nitric oxide synthase in response to fluid shear stress. Circ Res. 1996;79(5):984–991.
- Haynes WG, Hand MF, Dockrell ME, . Physiological role of nitric oxide in regulation of renal function in humans. Am J Physiol Renal Physiol. 1997;272(3):F364–F371.
- Baylis C, Harton P, Engels K. Endothelial derived relaxing factor controls renal hemodynamics in the normal rat kidney. J Am Soc Nephrol. 1990;1(6):875–881.
- Warden DH, Croatt AJ, Katusic ZS, Nath KA. Characterization of acute reversible systemic hypertension in a model of heme protein-induced renal injury. Am J Physiol Renal Physiol. 1999;277(1):F58–F65.
- Oberhauser V, Vonend O, Rump LC. Neuropeptide Y and ATP interact to control renovascular resistance in the rat. J Am Soc Nephrol. 1999;10(6):1179–1185.
- DiBona GF. Neural control of the kidney: Past, present, and future. Hypertension 2003;41(3):621–624.
- Baliga R, Ueda N, Walker PD, Shah SV. Oxidant mechanisms in toxic acute renal failure. Drug Metab Rev. 1999;31(4):971–997.
- Shah SV, Walker PD. Evidence suggesting a role for hydroxyl radical in glycerol-induced acute renal failure. Am J Physiol Renal Physiol. 1988;255(3):F438–F443.
- Moore KP, Holt SG, Patel RP, . A causative role for redox cycling of myoglobin and its inhibition by alkalinization in the pathogenesis and treatment of rhabdomyolysis-induced renal failure. J Biol Chem. 1998;273(48):31731–31737.
- Boutaud O, Moore KP, Reeder BJ, . Acetaminophen inhibits hemoprotein-catalyzed lipid peroxidation and attenuates rhabdomyolysis-induced renal failure. Proc Natl Acad Sci USA. 2010;107(6):2699–2704.
- Ay I, Tuncer M. Both endothelium and afferent nerve endings play a role in acetylcholine-induced renal vasodilation. Life Sci. 2006;79(9):877–882.
- Baylis C, Qiu C. Importance of nitric oxide in the control of renal hemodynamics. Kidney Int. 1996;49(6):1727–1731.
- Yeo TW, Lampah DA, Tjitra E, . Relationship of cell-free hemoglobin to impaired endothelial nitric oxide bioavailability and perfusion in severe falciparum malaria. J Infect Dis. 2009;200(10):1522–1529.
- Trifillis AL, Kahng MW, Trump BF. Metabolic studies of glycerol-induced acute renal failure in the rat. Exp Mol Pathol. 1981;35(1):1–13.
- Conger J, Robinette J, Villar A, Raij L, Shultz P. Increased nitric oxide synthase activity despite lack of response to endothelium-dependent vasodilators in postischemic acute renal failure in rats. J Clin Invest. 1995;96(1):631–638.
- Mayhan WG, Amundsen SM, Faraci FM, Heistad DD. Responses of cerebral arteries after ischemia and reperfusion in cats. Am J Physiol Heart Circ Physiol. 1988;255(4):H879–H884.
- Todoki K, Okabe E, Kiyose T, Sekishita T, Ito H. Oxygen free radical-mediated selective endothelial dysfunction in isolated coronary artery. Am J Physiol Heart Circ Physiol. 1992;262(3):H806–H812.
- Shah Alam M, Ku K, Yamauchi M, . Protective effects of nicaraven, a new hydroxyl radical scavenger, on the endothelial dysfunction after exposure of pig coronary artery to hydroxyl radicals. Mol Cell Biochem. 1998;178(1):237–243.
- Pieper GM, Siebeneich W, Roza AM, Jordan M, Adams MB. Chronic treatment in vivo with dimethylthiourea, a hydroxyl radical scavenger, prevents diabetes-induced endothelial dysfunction. J Cardiovasc Pharmacol. 1996;28(6):741–745.
- Diederich D, Skopec J, Diederich A, Dai FX. Endothelial dysfunction in mesenteric resistance arteries of diabetic rats: Role of free radicals. Am J Physiol Heart Circ Physiol. 1994;266(3):H1153–H1161.
- Dai F, Diederich A, Skopec J, Diederich D. Diabetes-induced endothelial dysfunction in streptozotocin-treated rats: Role of prostaglandin endoperoxides and free radicals. J Am Soc Nephrol. 1993;4(6):1327–1336.
- Mayhan WG, Patel KP. Treatment with dimethylthiourea prevents impaired dilatation of the basilar artery during diabetes mellitus. Am J Physiol Heart Circ Physiol. 1998;274(6):H1895–H1901.
- Gould J, Bowmer CJ, Yates MS. Renal hemodynamic responses to adenosine in acute renal failure. Nephron. 1995;71(2):184–189.
- Bischo A, Avramidis P, Erdbrügger W, Münter K, Michel MC. Receptor subtypes Y1 and Y5 are involved in the renal effects of neuropeptide Y. Br J Pharmacol. 1997;120:1335–1343.
- von Kügelgen I, Krumme B, Schaible U, Schollmeyer PJ, Rump LC. Vasoconstrictor responses to the P2X-purinoceptor agonist, β, y-methylene-L-ATP in human cutaneous and renal blood vessels. Br J Pharmacol. 1995;116:1932–1936.
- Cheng JT, Shen CL, Huang JJ. Decrease of catecholamine and neuropeptide Y-like immunoreactivity in the glycerol-induced acute renal failure of rats. Res Exp Med (Berl). 1990;190(5):315–322.
- Tanioka H, Nakamura K, Fujimura S, . Facilitatory role of NO in neural norepinephrine release in the rat kidney. Am J Physiol Regul Integr Comp Physiol. 2002;282(5):R1436–R1442.
- Tesfamariam B, Weisbrod RM, Cohen RA. Endothelium inhibits responses of rabbit carotid artery to adrenergic nerve stimulation. Am J Physiol Heart Circ Physiol. 1987;253(4):H792–H798.
- Kishi T, Hirooka Y, Kimura Y, Ito K, Shimokawa H, Takeshita A. Increased reactive oxygen species in rostral ventrolateral medulla contribute to neural mechanisms of hypertension in stroke-prone spontaneously hypertensive rats. Circulation. 2004;109(19):2357–2362.
- Kurtz T, Maletz R, Hsu C. Renal cortical blood flow in glycerol-induced acute renal failure in the rat. Circ Res. 1976;38(1):30–35.
- Stein JH, Lifschitz MD, Barnes LD. Current concepts on the pathophysiology of acute renal failure. Am J Physiol Renal Physiol. 1978;234(3):F171–F181.
- Hsu CH, Kurtz TW. Renal hemodynamics in experimental acute renal failure. Nephron. 1981;27(4–5):204–208.
- Chedru MF, Baethke R, Oken DE. Renal cortical blood flow and glomerular filtration in myohemoglobinuric acute renal failure. Kidney Int. 1972;1(4):232–239.
- Ayer G, Grandchamp A, Wyler T, Truniger B. Intrarenal hemodynamics in glycerol-induced myohemoglobinuric acute renal failure in the rat. Circ Res. 1971;29(2):128–135.
- Oken DE. Hemodynamic basis for human acute renal failure (vasomotor nephropathy). Am J Med. 1984;76(4):702–710.
- Zager R, Foerder C, Bredl C. The influence of mannitol on myoglobinuric acute renal failure: Functional, biochemical, and morphological assessments. J Am Soc Nephrol. 1991;2(4):848–855.
- Ustundag S, Yalcın O, Sen S, Cukur Z, Ciftci S, Demirkan B. Experimental myoglobinuric acute renal failure: The effect of vitamin C. Ren Fail. 2008;30(7):727–735.