Abstract
Objective: To investigate the effect of Shenfushu granule (SFSG) and atropine treatment on microvessels of the kidney and intestine after chronic renal failure (CRF) induced by 5/6 nephrectomy. Methods: Sprague Dawley rats were randomly divided into a sham group, a model group, an SFSG group, and an SFSG + atropine group. SFSG was administered daily 1 week after inducing CRF. Rats were sacrificed at the end of the eighth week. Urinary protein and stool and serum urea nitrogen (UN) and creatinine (Cr) levels were assessed. Hematoxylin and eosin and periodic acid-Schiff staining of the kidney and examination of the vascular endothelial growth factor (VEGF) and microvessel density (MVD) levels in kidney and intestine were performed. Results: The Cr and UN levels were significantly increased in blood and stool of the model group. SFSG significantly improved renal function, and the protective effects were further enhanced with the addition of atropine. Glomerular sclerosis, tubulointerstitial fibrosis, and microvessel loss were observed in CRF rats, and these pathological changes were ameliorated in the two treatment groups (p < 0.05), especially in the SFSG + atropine group. The expression of VEGF and MVD was decreased in the CRF rats compared with the sham group. SFSG treatment increased the expression of these proteins and reversed the degree of microvessel loss, glomerular sclerosis, and tubulointerstitial fibrosis (p < 0.05). Co-treatment with atropine enhanced these effects. Conclusions: SFSG alleviated renal function, upregulated the expression of VEGF and MVD in the kidney and intestine, and attenuated the degree of microvessel loss, glomerular sclerosis, and tubulointerstitial fibrosis in the early stages of CRF in rats, and addition of atropine enhanced these effects.
INTRODUCTION
Chronic kidney disease (CKD) is a common disease worldwide, with almost 11.7% incidence in China.Citation1 Chronic renal failure (CRF) is the end stage of CKD. Glomerulosclerosis and interstitial fibrosis are the main pathological features leading to progressive loss of renal function. Recent studies have reported that microvasculature loss occurs in models of progressive renal disease, and the degree of microvessel loss directly correlates with the severity of the glomerulosclerosis and interstitial fibrosis, thus influencing the amount of deterioration of renal function.Citation2
Effective treatments for CRF and the elimination of uremic toxins have traditionally included dialysis or kidney transplants. Only 15% of the world’s uremic patients can afford dialysis treatment, as it is a very expensive, time-consuming, and complicated technique. As a result of the global shortage of kidney donors for kidney transplants, the high costs linked with transplant surgery, and the high probability of organ rejection, most patients have very few options for effective treatment after kidney failure. There is great urgency in the search for an unconventional and affordable therapy for patients who cannot afford expensive dialysis or kidney transplants.Citation3 Medical scientists are currently attempting to research and develop an innovative and inexpensive new therapy for CKD.
Shenfushu granule (SFSG) is a Chinese medicine that is manufactured by the author and widely used as an oral dialysis drug in China. In our previous research, we reported that SFSG could significantly reduce blood urea nitrogen (UN) and serum creatinine (Cr). Additionally, this treatment could remove the medium molecule substance and improve the endogenous Cr clearance rate (data not shown); however, the capacity to protect residual renal function and maintain renal microvascular structure and function was not clarified. Meanwhile, in in vivo studies, we observed that atropine, a vasodilation drug, could promote the clearance of UN and Cr through the intestinal tract, but the exact mechanism of this action is unknown.Citation4 We hypothesize that treatment with both SFSG and atropine can stabilize renal function and inhibit microvasculature loss. This study was designed to observe the effect of SFSG on renal function, expression of vascular endothelial growth factor (VEGF), and microvessel density (MVD) in the kidney and intestine on CRF rat models. The effect of adding atropine and the potential synergistic effects were also examined. By researching the effect of both drugs, it is possible to determine the mechanism of the increased renal function. This treatment may be a cost-effective and noninvasive method for the treatment of CRF.
MATERIALS AND METHODS
Animals
Thirty-eight healthy 8-week-old male Sprague Dawley rats weighing between 160 and 180 g were purchased from the Central South University Xiangya School of Medicine’s animal department. Renal failure was induced in rats by 5/6 nephrectomies, as reported previously. Briefly, two-thirds of the left kidney was resected. One week later, the entire right kidney was removed. At the same time, eight rats underwent sham operations as the controls. After the operations, every rat was administrated an intramuscular injection of 20,000–30,000 units of penicillin per day to prevent infection for 3 days. One week after the second operation, the remaining 36 nephrectomy rats were randomly divided into four groups: (1) sham rats with saline 3 mL/d intragastric administration (sham group, n = 8); (2) model rats with saline 3 mL/d intragastric administration (model group, n = 8); (3) model rats treated with 3 mL/d intragastric administration of 4 g/kg/d of SFSG dissolved in water (SFSG group, n = 10); and (4) model rats treated with 3 mL/d intragastric administration of 4 g/kg/d of SFSG and 0.03 mg/kg/d of atropine dissolved in water (SFSG + atropine group, n = 10). The drugs were administered for 8 weeks, and all rats were weighed every 2 weeks. The drug treatments were adjusted according to the updated weights. Food and feeding methods were the same among the four groups. All rats were sacrificed after 8 weeks of therapy. The appropriate animal care and use committee approved all animal care protocols in this study.
Urine, Stool, and Blood Measurements
The rats were placed in metabolic cages with free access to water. Twenty-four hour urine samples were collected for urinary protein quantitation using the Bio-Rad method (Shanghai Sun Biological Company, Shanghai, PR China). Stool samples were collected for UN and Cr detection. Blood samples were obtained from eyeballs at the time of sacrifice and used for measuring blood UN and Cr levels. All components were determined using an automatic biochemistry analyzer (Hitachi 7170A; Hitachi Ltd., Tokyo, Japan).
Histology Examination
The formaldehyde-fixed kidneys were embedded in paraffin, and sections were prepared and stained with either periodic acid-Schiff (PAS) or hematoxylin and eosin (HE).
The PIPS-2020 system (Tianhai Inc., Chongqing, PR China) was used to detect the glomerular diameter and glomerulus acreage and to determine the extent of the injuries to the glomeruli of each group. The degree of glomerulosclerosis was determined using a previously published semiquantitative scoring method. Fifty glomeruli per section were randomly selected and scored as followsCitation5: grade 0, normal glomeruli; grade 1, sclerotic area ≤25% of the total glomerular area or distinct adhesion present between the capillary tuft and the Bowman’s capsule; grade 2, sclerotic area between 25% and 50%; grade 3, sclerotic area between 50% and 75%; and grade 4, sclerotic area between 75% and 100% of the total glomerular area. The ultimate score was obtained by multiplying the degree of changes by the percentage of glomeruli with the same degree of injury and summing the scores.
Tubulointerstitial injury (TI) in the renal cortex and outer medulla was defined as the presence of tubular dilation or atrophy, interstitial fibrosis, or inflammatory cell infiltration. Based on the percent of the damaged cortex or outer medulla, the amount of injury was graded on a scale of 0–4 as described previouslyCitation5: grade 0, normal; grade 0.5, small focal areas of damage; grade 1, <10% of the cortex or medulla has damage; grade 2, 10–25%; grade 3, 25–75%; and grade 4, >75%.
Immunohistochemical Staining for the Expression of VEGF and MVD in the Kidney and Intestine
The tissue sections were incubated for 20 h at 4°C with an antibody against VEGF that was diluted 1:200 in phosphate-buffered saline (PBS) with 1% bovine serum albumin (BSA). The sections were washed in PBS and incubated for 1 h at room temperature with a biotinylated goat antibody against rabbit or a goat secondary antibody against mouse that was diluted 1:200 in PBS. Then a streptavidin-peroxidase complex was added. The reaction was visualized by adding 3,3-diaminobenzidine (DAB) in the DAB Substrate Kit (Maixin Inc., Fuzhou, PR China) working solution and incubated for 5 min. The sections were washed in PBS for 10 min between each step, dehydrated, and mounted in BIO-G medium. Nonspecific background staining from the secondary antibody was tested through omission of the primary antibody from the control sections and replacement with BSA.
MVD was evaluated by CD141 immunostaining. Sections were stained for endothelial cells using a monoclonal anti-CD141 antibody. Sections were dewaxed through two changes of xylene and industrial methylated spirits. Endogenous peroxidase activity was blocked with a mixture of H2O2/methanol (12 mL H2O2 in 400 mL methanol) for 12 min. Antigen retrieval was performed for the endothelial markers by treating the sections with 0.2 g of trypsin and 0.4 g of calcium chloride in 440 mL of Tris-buffered saline [TBS (50 mM Tris–HCl, 150 mM NaCl, pH 7.4)] at 37°C for 20 min. Prior to staining with the polyclonal antiserum, the sections were treated with a mixture of 5% BSA/TBS (1 g BSA in 20 mL TBS) for 10 min. The anti-CD141 antibody was diluted 1:10 in 5% BSA/TBS. The sections were incubated with primary antibodies at room temperature for 40 min. Secondary antibodies were added and incubated for 40 min using the EnVision Labeled Polymer (DAKO laboratories Inc., Carpinteria, CA, USA)(mouse or rabbit as appropriate). The sections were washed with TBS between the incubation steps. DAB was used as a chromogen. The last two steps were carried out using a commercial kit. Omission of the primary antibody was used as a negative control.
Staining for each specific protein was evaluated by light microscopy (40 randomly selected fields per section) at a magnification of 400×. Digital images of each microscopic field were captured, stored in a computer, and subsequently analyzed for stain density with the use of free image software.
Statistical Analysis
The results were analyzed using SPSS software v.11.5 (SPSS Inc., Chicago, IL, USA). Data were expressed as the mean ± standard deviation (X ± SD). Statistical significance of the differences among the three groups was determined by one-way analysis of variance. Least significant differences were used for comparisons within the designated groups. Correlation analysis was performed for all parameters using the Pearson’s correlation coefficient test. Differences in means with p < 0.05 were considered statistically significant.
RESULTS
The Effect of SFSG on the General Condition, Urinary Protein and Stool and Serum UN and Cr of Rat Models with 5/6 Nephrectomy
The sham group rats demonstrated alert and responsive behavior. These rats had neat, shiny, and dense fur, and their growth, feeding, and activities were normal. Their weight increased, and there was no death. The model group rats demonstrated malnutrition, low spirits, slow activity, loss of appetite, and fluffy and withered fur. In this group, the weight increased slowly. Six weeks after therapy, the rats from the two treatment groups had markedly higher weight than the untreated control group (p < 0.05). There was no difference in weight between the two treatment groups (, ). The 24 h urinary albumin excretion rate (UAER) and the stool and serum UN and Cr levels in the 5/6 nephrectomy group were significantly higher than in the sham group (p < 0.05). After 8 weeks of treatment, there were significant differences in UAER, stool UN and Cr excretion, and serum UN and Cr levels between the SFSG treatment group and the model group (p > 0.05). Meanwhile, the body weight was significantly higher after 6 weeks of treatment with SFSG compared with the model group (p > 0.05). In the SFSG group, one rat died in the first week and one rat died in the seventh week. In the SFSG + atropine group, there was one death in the second, fourth, and fifth weeks. There were no deaths in the other two groups. At the end of the experiment, there were eight rats in the sham group, model group, and SFSG group and only seven rats in the SFSG + atropine group. The cause of death for all five rats was likely infection ().
Figure 1. Glomerulosclerosis index.
Note: *p < 0.05 compared with sham group; **p < 0.05 compared with model group; ***p < 0.05 compared with SFSG group.
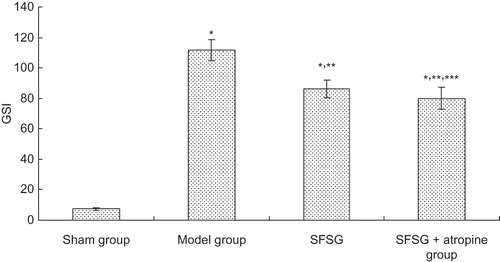
Figure 2. Tubulointerstitial score.
Note: *p < 0.05 compared with sham group; **p < 0.05 compared with model group; ***p < 0.05 compared with SFSG group.
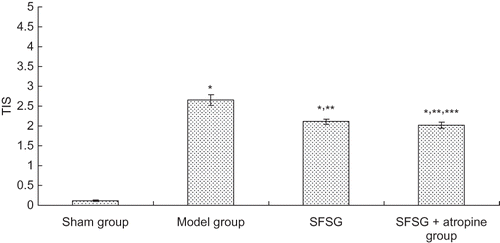
Figure 3. Representative light micrographs of kidney sections, stained with periodic acid-Schiff technique: (A and B) sham group; (C and D) model group; (E and F) SFSG group; (G and H) SFSG + atropine group. Magnifications: ×100 in (A, C, E, and G); ×400 in (B, D, F, and H).
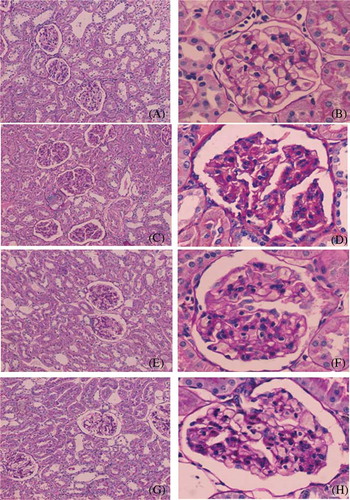
Table 1. Weight changes of rats with 5/6 nephrectomy.
Table 2. Twenty-four h urine volume and urine protein.
Table 3. Serum UN and Cr of rats with 5/6 nephrectomy.
HE and PAS Staining of the Kidney in Different Groups
There was no glomerular sclerosis, tubular expansion, interstitial infiltration of inflammatory cells, or fibrous proliferation in the sham group. At the end of the eighth week, some of the kidney samples in the model group showed enlargement of glomerular volume and increases in the mesangial matrix. There was some capillary expansion or occlusion and glomerular wall thickening and part of the glomerulus presented segmental sclerosis. Proximal tubular epithelial cells showed decreased or completely removed brush borders, and in some renal tubular epithelial cells, vacuolar degeneration or even separation was observed. Interstitial infiltration of inflammatory cells and fibrosis could be observed. However, glomerular sclerosis and renal interstitial fibrosis in the SFSG groups at the end of the eighth week were slightly ameliorated, especially in the SFSG + atropine group, when compared with the model group. Meanwhile, glomerulosclerosis index (GSI) and tubulointerstitial score (TIS) were more significantly ameliorated in the therapy groups than in the model group, and the SFSG + atropine group demonstrated the best outcomes (p < 0.05) ().
The Expression of VEGF and MVD in the Kidney and Intestine of the Different Groups
VEGF mainly deposited on the glomerular visceral epithelial cells (podocytes) and tubular epithelial cells of the rats in sham group. Immunohistochemical staining of the glomerulus and renal tubule indicated that the positive percentage of VEGF and MVD deposition in the model group and therapy groups were lower than in the sham group. However, the degree of VEGF and MVD deposition in the therapy groups was significantly higher than in the model group, and the SFSG + atropine group demonstrated the most deposition (p < 0.05). Immunohistochemical staining of the small intestine indicated that the positive percentage of VEGF and MVD deposition in the model group and therapy groups was lower than in the sham group. However, the percent of VEGF and MVD deposition in the therapy groups was significantly higher than in the model group, and the SFSG + atropine group had the most deposition (p < 0.05) ().
Figure 5. VEGF expression of the kidney in different groups: (A and B) sham group; (C and D) model group; (E and F) SFSG group; (G and H) SFSG + atropine group. Magnifications: ×100 in (A, C, E, and G); ×400 in (B, D, F, and H).
Note: *p < 0.05 compared with sham group; **p < 0.05 compared with model group; ***p < 0.05 compared with SFSG group.
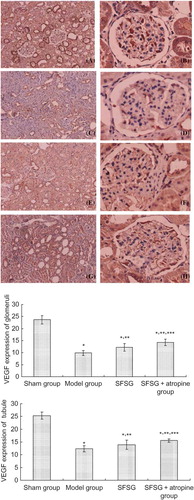
Figure 6. MVD (CD141) expression of the kidney in different groups: (A and B) sham group; (C and D) model group; (E and F) SFSG group; (G and H) SFSG + atropine group. Magnifications: ×100 in (A, C, E, and G); ×400 in (B, D, F, and H).
Note: *p < 0.05 compared with sham group; **p < 0.05 compared with model group; ***p < 0.05 compared with SFSG group.
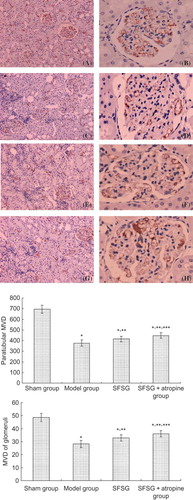
DISCUSSION
CRF is the end stage of most CKDs. Once established, CRF progresses irreversibly to the final stage, resulting in patient death. In contrast with the capacity of the kidneys to regain function following acute renal injury, renal injury of a more prolonged nature often leads to progressive and irreversible destruction of the nephron mass. Such a reduction of renal mass can then cause structural and functional hypertrophy of the surviving nephrons. This compensatory hypertrophy is due to an adaptive hyperfiltration mediated by increases in glomerular capillary pressures and flows. Eventually, these adaptations prove maladaptive, predisposing the patient to sclerosis of the residual glomerular population.
In experimental animal studies, CRF is most often induced by 5/6 nephrectomy. This model may be achieved by either ligation of renal vessels supplying the renal poles or surgical removal of both renal poles followed by contralateral nephrectomy.Citation6 However, one of the most common complications in the model of renal vessels ligation is hypertension.Citation7 Animals subjected to subtraction of 5/6 of the total renal mass experience accelerated induction of CRF and possess several characteristics that are typically seen in human chronic renal diseases.Citation8,9 In addition to marked structural heterogeneity, progressive azotemia, proteinuria, and glomerular and sclerotic TI are presented in this model. Approximately 1–2 weeks after 5/6 nephrectomy, all glomeruli demonstrate hyperfiltration and have elevated glomerular capillary pressure. Some studies have shown that the single nephron filtration rate of albumin ranges from 1 to 15 times the normal rate in the remaining nephrons of Munich-Wistar rats 4–6 weeks after 5/6 nephrectomy.Citation10 In our study, the UN and Cr and urinary albumin levels after 5/6 nephrectomy were significantly higher than in the normal control group after 8 weeks. Renal pathology showed that the residual glomerular diameter and the cross-sectional area increased significantly, the mesangial cell proliferated, the matrix increased, parts of the glomerulus presented with segmental sclerosis, and there was apparent renal tubular damage and interstitial inflammation. All these results demonstrated the successful establishment of the CRF model.
The intrarenal vasculature, which plays an important role in the maintenance of the structure and function of the kidney, is the most affected structure in CRF. The damage to the vasculature prevents appropriate blood flow and favors the progression of glomerular sclerosis.Citation5,11–14 The degree of microvasculature loss in models of progressive renal disease correlates with the severity of glomerulosclerosis and interstitial fibrosis. For that reason, improvement of the rheological properties of the blood could delay the progression of the CRF. Elucidating the mechanism of microvasculature loss and exploring effective therapeutic measures will be of great significance for delaying the progression of renal failure. Unfortunately, there are no ideal drugs that can fully reverse microvasculature loss and prevent glomerulosclerosis and interstitial fibrosis.
Traditional Chinese medicine has the advantages of fewer side effects, increased safety, and the ability to act against multiple targets, which will be important in the discovery drugs against glomerulosclerosis and interstitial fibrosis. SFSG is a very useful traditional Chinese medicine for the treatment of CRF. SFSG has been developed by our team and is widely used in the south central region of China. SFSG is mainly composed of Folium sennae, Carthamus tinctorius, and Codonopsis pilosula. Our previous in vivo studies have found that SFSG could inhibit the renin–angiotensin system (RAS), thus reducing the RAS activity and angiotensin levels and decreasing the expression of transforming growth factor beta (TGF-β1) and TGF-β1MRNA. This leads to an attenuation of renal fibrosis (data not shown). Moreover, C. tinctorius, the major component of SFGS, could eliminate oxyradicals and reduce the degree of injury to the kidney. Additionally, the main component of F. sennae is anthraglucosennin, which can be broken down into rhein anthrone and anthrone by the intestinal flora. These three substances can reduce intestinal absorption and increase the intestinal excretion of electrolytes and water. They can also stimulate intestinal peristalsis, thus promoting the excretion of toxins from the intestine. Meanwhile, in our previous research, we observed that atropine, a vasodilation drug, could further promote the clearance of UN and Cr through the intestinal tract. In this study, serum Cr and UN levels in the treatment group decreased significantly compared with the model group. Additionally, stool Cr and UN levels increased significantly in the two treatment groups (p < 0.05), especially in the group treated with both SFGS and atropine. This implied that SFSG could promote the intestinal excretion of uremic toxins, likely through the action of F. sennae, which causes a reduction of the serum levels of the toxins. The addition of atropine enhanced the effect of the SFSG.
The renal microvasculature includes the glomerular capillaries and the peritubular capillaries, which play an important role in the maintenance of the structure and function of the kidney. Some studies have found that, although there is an early proliferative response of the peritubular capillary endothelium, the proliferation is not sustained. Progressive capillary loss (rarefaction) occurs in models of progressive renal disease, such as in the remaining kidney cells after nephrectomy, the aging kidney, or after chronic cyclosporine A nephropathy.Citation15–18 The mechanism of capillary loss is mediated in part by a reduction in the endothelial proliferative response, and this impairment in capillary repair is mediated by the expression of VEGF. VEGF is an important endothelial cell angiogenic, survival, and trophic factor that is constitutively expressed in the kidney. VEGF is localized primarily in the podocytes and tubular epithelial cells in the outer medulla and medullary rays of the kidney.Citation19–21 Several studies documented a loss of podocyte VEGF levels in glomerulosclerosis and a loss of tubular VEGF in chronic interstitial disease, such as during chronic renal transplant rejection.Citation22,23 Similarly, Kang et al. demonstrated that renal VEGF expression is also reduced in a 5/6 nephrectomy model of progressive renal failure. The reduction of VEGF expression was correlated with decreased peritubular capillary density and with more severe interstitial fibrosis. Both experimental and human progressive renal diseases are associated with capillary loss and a reduction in renal VEGF expression. There is intriguing evidence that the maintenance of the microvasculature may stabilize renal function and slow progression of CRF, and this benefit may occur independently of effects on BP or proteinuria.Citation2,24
Histologically, glomerulosclerosis and tubulointerstitial fibrosis are observed by GSI and TIS, respectively, while microvasculature loss in 5/6 nephrectomy rats is characterized by a decrease of VEGF. Our study showed that, compared with the model group, the 5/6 nephrectomy rats treated with SFSG had less mesangial proliferation and interstitial inflammatory cell infiltration. This implied that SFSG treatment could alleviate glomerulosclerosis and protect the remnant nephrons. Obvious glomerulosclerosis and renal interstitial fibrosis could be observed at the eighth week. Meanwhile, immunohistochemical results showed that the expression of VEGF and MVD in the kidney and intestine markedly decreased. These data demonstrated that the animal models presented with glomerulosclerosis, tubulointerstitial damage, and microvasculature loss in both the kidney and the intestine.
Previous reports demonstrated that chronic treatment with VEGF could maintain the microvasculature and thereby slow the progression of CRF. SFSG could reduce the relative area of glomerulosclerosis and tubulointerstitial damage; increase the expression of VEGF in podocytes, renal tubular epithelial cells, and intestinal mucosal cells; and increase the MVD. Addition of atropine enhanced all of the therapeutic effects of SFSG treatment. From these results, we hypothesize that SFSG could alleviate the glomerulosclerosis and tubulointerstitial damage of the CRF rats and stabilize renal function by increasing the expression of VEGF and decreasing the loss of MVD. Atropine could enhance the effects of SFSG by expanding the blood vessels of the kidney and intestine.
In the 5/6 nephrectomy model, the deterioration of renal function was associated with impaired angiogenesis and reduced capillaries, which implied that microvascular disease played an important role in the deterioration of renal function. In our study, the Chinese medicine SFSG increased the intestinal and renal microcirculation, promoted the clearance of uremic toxins from the intestinal tract, and improved the renal function. SFSG could enhance the expression of VEGF and MVD in residual kidney and intestine, protect the endothelial cells, and maintain the microvasculature of the kidney, leading to a slower progression of CRF. In addition, co-treatment with SFSG and atropine in the CRF rats improved the intestinal and renal microcirculation, enhanced the effects of the clearance of the uremic toxins from the intestinal tract, and improved renal function compared with treatment using SFSG alone. Due to the few side effects, the low cost, and the easy availability, atropine is an important drug to use in combination with SFSG to delay the progress of CRF. The long-term efficacy of this combination therapy is worthy of further observation. In future studies, it is important to focus on the possible toxicity and side effects of this compound and the molecular mechanisms of action.
Declaration of interest: The authors report no conflicts of interest. The authors alone are responsible for the content and writing of the paper
REFERENCES
- Sabanayagam C, Lim SC, Wong TY, . Ethnic disparities in prevalence and impact of risk factors of chronic kidney disease. Nephrol Dial Transplant. 2010;25(8):2564–2570.
- Kang DH, Kanellis J, Hugo C, . Role of the microvascular endothelium in progressive renal disease. J Am Soc Nephrol. 2002;13(3):806–816.
- Jain P, Shah S, Razek C, . Potentials and limitations of microorganisms as renal failure biotherapeutics. Biologics Targets Ther. 2009;3:233–243.
- Zheng H, Yunsheng J, Ji-an L. Influence factors in the clearance of urea and creatinine through gastrointestinal tract. Bull Hunan Med Univ. 1999;24(6):513–532.
- Wang Y, Wang DH. Aggravated renal inflammatory responses in TRPV1 gene knockout mice subjected to DOCA‐salt hypertension. Am J Physiol Renal Physiol. 2009;297(6):F1550–F1559.
- Chow KM, Liu ZC, Chang TM, . Animal remnant kidney model of chronic renal revisited. Hong Kong J Nephrol. 2003;5(2):57–64.
- Švíglerová J, Kuncová J, Nalos L, . Cardiovascular parameters in rat model of chronic renal failure induced by subtotal nephrectomy. Physiol Res. 2010;59(Suppl. 1): S81–S88.
- Calunga JL, Zamora ZB, Borrego A, . Ozone therapy on rats submitted to subtotal nephrectomy: Role of antioxidant system. Mediators of inflammation. Mediators Inflamm. 2005;2005(4):221–227.
- Sasaki S, Yokozawa T, Cho EJ, . Protective role of gamma-aminobutyric acid against chronic renal failure in rats. J Pharm Pharmacol. 2006;58(11):1515–1525.
- Yoshioka T, Shiraga H, Yoshiyuki Y, . “Intact nephrons” as the primary origin of proteinuria in chronic renal disease. J Clin Invest. 1988;82(5):1614–1623.
- Fauci AS, Braunwald E, Isselbacher KJ, . Chronic renal failure. In: Braunwald E, Hauser SL, Fauci AS, Longo DL, Kasper DL, Jameson JL, eds. Harrison’s Principles of Internal Medicine. New York: McGraw-Hill; 1998:1513–1520.
- Lane PH. Long-term furosemide treatment in the normal rat: Dissociation of glomerular hypertrophy and glomerulosclerosis. Am J Kidney Dis. 1999;33(6):1058–1063.
- Slomowitz L. Tubuloglomerular feedback in chronic renal failure. Nephron. 1987;45(4):264–271.
- Sheldin DW, Gusbech G. The kidney physiology and physiopathology. In: Roven P, ed. The Kidney Physiology and Physiopathology. New York: Roven Press; 1985:1901–1905.
- Kang DH, Anderson S, Kim YG, . Impaired angiogenesis in the aging kidney: Vascular endothelial growth factor and thrombospondin-1 in renal disease. Am J Kidney Dis. 2001;37(3):601–611.
- Kang DH, Joly AH, Oh SW, . Impaired angiogenesis in the remnant kidney model (I): Potential role of vascular endothelial growth factor and thrombospondin-1. J Am Soc Nephrol. 2001;12(7):1434–1447.
- Thomas SE, Anderson S, Gordon KL, . Tubulointerstitial disease in aging: Evidence for peritubular capillary damage, a potential role for renal ischemia. J Am Soc Nephrol. 1998;9(2): 231–242.
- Ohashi R, Kitamura H, Yamanaka N. Peritubular capillary injury during the progression of experimental glomerulonephritis in rats. J Am Soc Nephrol. 2000;11(1):47–56.
- Gerber HP, Hillan K, Ryan A, . VEGF is required for growth and survival in neonatal mice. Development. 1999;126: 1149–1159.
- Brown LF, Berse B, Tognazzi K, . Vascular permeability factor mRNA and protein expression in human kidney. Kidney Int. 1992;42:1457–1461.
- Kretzler M, Schroppel B, Merkle M, . Detection of multiple vascular endothelial growth factor splice isoforms in single glomerular podocytes. Kidney Int Suppl. 1998;67:S159–S161.
- Shulman K, Rosen S, Tognazzi K, . Expression of vascular permeability factor (VPF/VEGF) is altered in many glomerular diseases. J Am Soc Nephrol. 1996;7:661–666.
- Grone J-J, Simon M, Grone EF. Expression of vascular endothelial growth factor in renal vascular disease and renal allografts. J Pathol. 1995;177:259–267.
- Kang D-H, Joly AH, Oh S-W, . Impaired angiogenesis in the remnant kidney model. I. Potential role of vascular endothelial growth factor and thrombospondin-1. J Am Soc Nephrol. 2001;12:1434–1447.