Abstract
Aim: Adenosine 5’-monophosphate (AMP)-activated protein kinase (AMPK) is known as a mammalian cell energy sensor, which could regulate cellular energy metabolism via sensing the alterations of energy balance, such as oversupply or lack of glucose and fatty acid. Recent studies have suggested that AMPK could also regulate many other biological processes, including cell cycling, inflammation, protein synthesis, and so on. In this study, AMPK signaling in high-glucose-induced dysfunction of mesangial cells (MCs) was investigated. Methods: Established rat glomerular MCs were treated under normal glucose (5.6 mM glucose) or high-glucose conditions (30 mM glucose). mRNA levels of AMPK subunits were detected by reverse transcriptase-polymerase chain reaction. Expressions of AMPKα, phosphorylated AMPKα (p-AMPKα), phosphorylated acetyl-CoA carboxylase (p-ACC), and collagen IV were measured by Western blot. Results: Under high-glucose conditions, AMPKα protein expression and mRNA levels were significantly decreased. High-glucose treatment also induced a notable decrease in p-AMPKα and p-ACC expression. AMPKα activation by 5-aminoimidazole-4-carboxamide-1-β-d-ribofuranoside (AICAR) effectively ameliorated high-glucose-induced dysfunction of MCs, including cell proliferation, cell-cycle progression, and collagen IV production. Conclusion: High glucose impaired AMPKα in its expression and activity; AICAR significantly ameliorated high-glucose-induced proliferation of MCs and collagen IV production, indicating a role of AMPKα in high-glucose-induced dysfunction of MCs.
INTRODUCTION
Diabetic nephropathy has become a leading cause of end-stage renal failure worldwide and affected about 25–40% of diabetes patients.Citation1 As the precise pathogenesis of diabetic nephropathy is not fully elucidated, current treatment could only focus on the control of risk factors associated with the disease progression, such as hyperglycemia, hypertension, and proteinuria.Citation2 Although the effectiveness of controlling these risk factors on diabetic nephropathy have been well demonstrated by ample perspective clinical researches,Citation3–5 there are still large portions of patients with diabetic nephropathy progressing into end-stage renal failure despite intensive glucose and blood pressure control, indicating that it is still of great significance and urgency to get a further understanding of diabetic nephropathy.
Histologically, diabetic nephropathy is mainly characterized by the thickening of basement membrane and expansion of glomerular mesangium. Multiple resident and nonresident renal cells, along with inflammatory mediators, participate in this pathogenesis, among which mesangial cells (MCs) have been proposed as the major player.Citation6 Related studies have well demonstrated that high-glucose-induced dysfunction of MCs, including cell-cycle progression and excessive extracellular matrix (ECM) synthesis, might not only directly be associated with mesangium expansion and basement membrane thickening but also be fundamentally linked to endothelial and podocyte damage and secondary tubulointerstitial fibrosis.Citation7,8 As an important intervention target for diabetic nephropathy, the mechanisms of dysfunction of glomerular MCs are to be further elucidated.
Adenosine 5’-monophosphate (AMP)-activated protein kinase (AMPK) is known as a mammalian cell energy sensor, which could regulate cellular energy metabolism via sensing the alterations of energy balance, such as oversupply or lack of glucose and fatty acid.Citation9,10 There are studies suggesting that AMPK could also affect many other biological processes, including protein synthesis, cell-cycle progression, and ECM turnover,Citation11,12 the latter two of which were demonstrated as two key events in high-glucose-induced dysfunction of mesangial cells.Citation7,8 Therefore, in this study, we investigated the alterations of AMPK expression and examined its role in cultured rat MCs under high-glucose conditions.
Acetyl-CoA carboxylase (ACC) is a citrate-activated enzyme that can be phosphorylated in vitro by AMPK.Citation13 In addition, as the major biological effect of AMPK is to regulate intracellular fatty acid oxidation via inhibiting phosphorylated ACC (p-ACC), we consider the latter could be a good measure of the activation of AMPK.Citation9,14 In this study, the changes of p-ACC expression in both protein and mRNA levels were detected. To further confirm the role of AMPK in high-glucose-induced dysfunction of MCs, we used 5-aminoimidazole-4-carboxamide-1-β-d-ribofuranoside (AICAR), a widely used activator of the AMPK.
Although plenty of studies in the recent years have extensively investigated the mechanisms responsible for high-glucose-induced dysfunction of MCs and multiple signaling pathways,Citation2,6,15 there are studies that indicate the important role in diabetic rats and rat glomerular epithelial cells (GECs).Citation16–18 However, studies about the role of AMPK in high-glucose-induced dysfunction of MCs are still lacking. From this point of view, we investigated AMPK signaling in high-glucose-induced dysfunction of rat MCs in this study. In addition, it was found that under high-glucose conditions, AMPKα protein expression and mRNA levels were significantly decreased. High-glucose treatment also induced a notable decrease in p-AMPKα and p-ACC expression. AMPKα activation by AICAR effectively ameliorated high-glucose-induced dysfunction of MCs, including cell proliferation, cell-cycle progression, and collagen IV production.
MATERIALS AND METHODS
Cell Culture
Established rat glomerular MCs were obtained from Life Science Academy of Wuhan University (Wuhan, China). Cells were cultured in RPMI 1640 (Gibco Invitrogen Corporation, Carlsbad, CA, USA) supplemented with 10% fetal calf serum, 2 mM glutamine, 5 ng/mL insulin, 100 U/mL penicillin, and 100 μg/mL streptomycin at 37°C, 5% CO2. MCs between passages 10 and 18 were used for experiments. After a 24-h preincubation period, cells were either treated with normal glucose or high glucose with or without AICAR (1 mM) for 24 or 12 h. AICAR was purchased from Sigma-Aldrich (St. Louis, MO, USA).
Western Blot Analysis
Western blot was performed as described previously.Citation14,23 In brief, after treatment with different compounds, MCs were harvested and lysed using a lysis buffer containing 25 mM HEPES-NaOH, 1.5 mM MgCl2, 0.3 M NaCl, 0.2 mM EDTA, 0.1% Triton X-100, 0.5 mM Dithiothreitol (DTT), 20 mM β-glycerophosphate, 100 mM NaVO4, 2 mg/mL leupeptin, and 100 mg/mL Phenylmethanesulfonyl fluoride (PMSF). Protein concentrations were determined using the Lowry method. Equal amounts of protein were loaded, then separated using sodium dodecyl sulfate polyacrylamide gel electrophoresis, and transferred to nitrocellulose membranes. After being blocked with 5% fat-free milk, the membranes were then incubated overnight at 4°C with specific antibodies for AMPKα, p-AMPKα, p-ACC, and collagen IV. After incubation with the respective second antibodies, the immune complexes were detected using the electrochemiluminescence (ECL) method, and immunoreactive bands were quantified using an Alphaimager 2200. Values were corrected using the absorbency of the internal control (actin). Antibodies for AMPKα and p-AMPKα were purchased from Cell Signaling Technologies (Beverly, MA, USA). Antibodies for p-ACC are a product of Upstate (Lake Placid, NY, USA), while other antibodies are purchased from Santa Cruz Biotechnology (Santa Cruz, CA, USA).
Reverse Transcriptase-PCR
RT-PCR was used to determine the steady-state mRNA levels of AMPKα1 and AMPKα2. Briefly, after extraction and reverse transcription of renal total RNA, cDNA was used as a template for the PCR reactions using gene-specific primer pairs. Generally, 30–35 cycles for amplification in the linear range were used. Quantification of PCR products was performed using densitometry, and the relative mRNA levels were calculated and compared after normalization to beta-actin. The primers for the RT-PCR reaction were purchased from Sangon Biotech (Shanghai, China). The sequences were as follows: AMPKα1, sense 5′-GTTGGCACGTGGTCATCATCA-3′, antisense 5′-GGTTTAATTCATCTAGGGTGT-3′; AMPKα2, sense 5′-TTGAGATACTCCGGGATG-3′, antisense 5′-CTGGCTTGGTTCATTATT-3′.
MTT Assay
MTT analysis was performed as described previously.Citation24 In brief, after treated with different compounds as described above, 20 μL MTT (5 mg/mL; Essen, Fluko, Germany) was added to each well. Cells were further cultured for 4 h and then were lysed using dimethylsulfoxide (100 μL/well; Sigma, St. Louis, MO, USA). When the formazan crystals completely dissolved, the optical density (OD) was measured at 570 nm using a multiscan reader (Labsystems, Helsinki, Finland). The arithmetic mean OD of four wells for each group was calculated.
Flow Cytometry Analysis
Cell-cycle analysis was performed using flow cytometry.Citation24 After treatment with different glucose conventions and compounds, cells were harvested with 0.25% trypsin (Gibco Invitrogen Corporation) and washed three times with phosphate buffer saline (PBS)buffer. Then, cells were centrifuged at 100 × g at 4°C for 8 min, and cell pellets were resuspended in RNase (1 mg/mL) for 20 min. Cells were stained with propidium iodide in PBS (final concentration, 40 μg/mL) for 15 min before being analyzed with FACSCAN (Becton Dickinson, Franklin Lakes, NJ, USA). In every sample, 1 × 104 cells were detected and the cell cycle was analyzed using CellQuest software (Becton Dickinson).
Data Analysis
All experiments were repeated at least three times. Values are given as means ± SD. Data were analyzed using SPSS 10.0 software (SPSS Inc, Chicago, IL, USA). Statistical significance was assessed by ANOVA and unpaired Student’s t-test. p-Values of less than 0.05 were considered to be statistically significant.
RESULTS
High-Glucose Treatment Decreased AMPKα Protein Expression
AMPKα protein levels were detected by Western blot. When compared with the control (5.6 mM glucose), 26% decrease after 12 h and 44% decrease after 24 h of AMPKα protein levels were detected under high-glucose treatment (p < 0.05), suggesting that high-glucose stimulation might impair AMPKα expression, and in a time-dependent manner ().
Figure 1. High-glucose treatment decreased AMPKα and p-AMPKα expression in mesangial cells. AMPKα and p-AMPKα protein levels were detected by Western blot. (A) Compared with cells less than 5.6 mM glucose, AMPKα expression decreased by 26% in cells under high glucose for 12 h and 44% for 24 h (p < 0.05). (B) p-AMPKα expression decreased by 36% in cells under high glucose for 12 h and 49% for 24 h (p < 0.05), compared with cells in 5.6 mM glucose. AICAR treatment elevated p-AMPKα protein levels by 80%, compared with that in cells treated only by high glucose for 24 h (p < 0.05). Values are given as means ± SD and p < 0.05 is considered statistically significant.
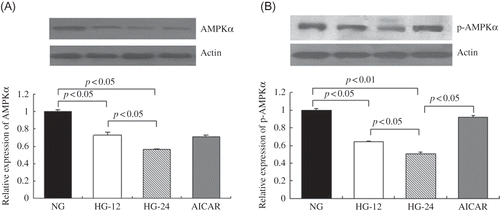
High-Glucose Treatment Decreased both AMPKα1 and AMPKα2 mRNA Levels
Respectively, AMPKα1 and AMPKα2 mRNA levels in cells under high-glucose incubation were significantly decreased by reverse transcriptase-polymerase chain reaction (RT-PCR), compared with the control, in a time-dependent manner, as 27% reduction of AMPKα1 and 32% reduction of AMPKα2 mRNA expression were determined after 12 h of high-glucose treatment (p < 0.05) and a further decrease of both AMPKα1 and AMPKα2 mRNA levels after 24 h (p < 0.05) ().
Figure 2. High-glucose treatment decreased both AMPKα1 and AMPKα2 mRNA levels. AMPKα1 and AMPKα2 mRNA levels were determined by RT-PCR. Compared with that in cells less than 5.6 mM glucose, AMPKα1 mRNA levels decreased by 27% in cells in high glucose for 12 h and 46% for 24 h (p < 0.05). Similarly, AMPKα2 mRNA levels were also decreased by high-glucose treatment compared with the control (p < 0.05). Values are given as means ± SD and p < 0.05 is considered statistically significant.
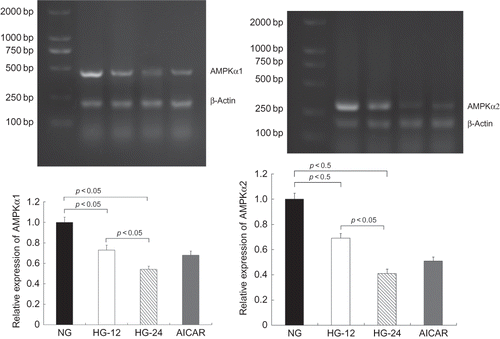
High-Glucose Treatment Decreased p-AMPKα Levels
p-AMPKα was measured by Western blot. When compared with the control, high-glucose treatment significantly decreased p-AMPKα by 36% after 12 h (p < 0.05) and by 49% after 24 h, respectively (p < 0.05). This suggested that high-glucose stimulation could also inhibit AMPKα activation, and AICAR induced a 80% elevation of p-AMPKα expression compared with cells under high-glucose incubation for 24 h (p < 0.05) ().
High-Glucose Treatment Decreased p-ACC Levels by Western Blot
p-ACC expression also was significantly inhibited by high glucose, evidenced by 26% and 35% decrease after 12 and 24 h of high-glucose treatment, respectively (p < 0.05). In addition, p-ACC levels were elevated about 38% after AICAR treatment compared with cells treated by high glucose only for 24 h (p < 0.05; ).
Figure 3. High-glucose treatment decreased p-ACC levels and AMPK activation ameliorated high-glucose-induced collagen IV expression.
(A) p-ACC protein levels were detected by Western blot. When compared with cells in normal glucose, p-ACC expression decreased by 26% after treatment with high glucose for 12 h and 35% for 24 h (p < 0.05). When compared with cells treated with high glucose for 24 h, AICAR treatment elevated p-ACC protein levels by 38% (p < 0.05). (B) Collagen IV was detected by Western blot. High glucose respectively resulted in 48% and 67% increase of collagen IV levels after treatment with high glucose for 12 h or 24 h (p < 0.05). However, AICAR treatment decreased collagen IV contents by 15%, compared with that after high glucose only for 24 h (p < 0.05). Values are given as means ± SD and p < 0.05 is considered statistically significant.
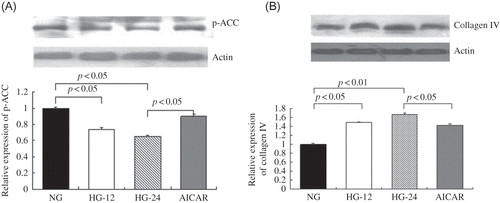
Table 1. Cell-cycle analysis of mesangial cells.
AMPK Activation by AICAR Ameliorated High-Glucose-Induced Mesangial Cells Cell-Cycle Progression
Cell-cycle progression was evaluated by flow cytometry analysis. When compared with the control, 12 h of high-glucose treatment significantly elevated cell proportion in G2/M phase by 37.5% (p < 0.05). Further stimulation by high glucose for 24 h elevated cell proportion in S phase by 40.7% and cell proportion in G2/M phase by 62.5% (p < 0.05). This suggested that high glucose might promote cell-cycle progression in MCs. AICAR treatment effectively ameliorated high-glucose-induced cell-cycle progression, evidenced by 15.8% decrease of cell proportion in S phase and 38.5% decrease in G2/M phase (p < 0.05; ).
AMPK Activation by AICAR Ameliorated High-Glucose-Induced Proliferation of Mesangial Cells
Proliferation of MCs was further determined by methyl thiazole tetrazolium (MTT) assay. When compared with control, 12 and 24 h of high-glucose treatment induced a 30.7% and 53.2% increment of proliferation of MC, respectively (p < 0.05), suggesting that high-glucose level might promote proliferation of MCs. Consistent with the findings of flow cytometry analysis, AMPK activation by AICAR effectively blocked high-glucose-induced proliferation of MCs, evidenced by a 12.5% decrease of cell proliferation (p < 0.05; ).
Figure 4. AMPK activation ameliorated high-glucose-induced proliferation of mesangial cells. Proliferation of mesangial cells was determined by MTT assay. When compared with the control, 12 or 24 h of high-glucose treatment, respectively, increased proliferation of mesangial cells by 30.7% or 53.2% (p < 0.05). Values are given as means ± SD and p < 0.05 is considered statistically significant.
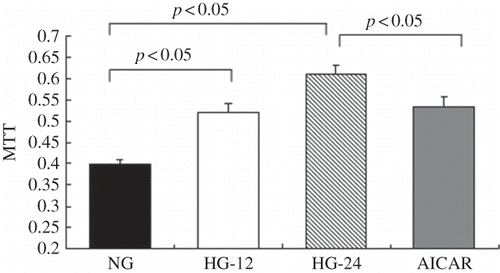
AMPK Activation by AICAR Ameliorated High-Glucose-Induced Collagen IV Expression
High-glucose treatment for 12 and 24 h significantly elevated collagen IV protein contents by 48% and 67%, respectively (p < 0.05). When compared with cells under 24 h of high-glucose incubation, AICAR treatment significantly decreased collagen IV protein levels by 15% (p < 0.05), indicating that AMPK activation could ameliorate high-glucose-induced collagen IV overexpression in MCs ().
DISCUSSION
The intracellular signal pathways responsible for high-glucose-induced dysfunction of MCs have been extensively investigated. Both metabolic signaling, such as Protein kinase C-mitogen-activated protein kinase (PKC-MAPK) pathway and hexosamine pathway, and nonmetabolic signaling, such as TGF-β system, have been evidenced to be involved.Citation15 AMPK is a heterotrimeric complex consisting of one catalytic alpha subunit (AMPKα) and two regulatory β and γ subunits. In addition, AMPKα consists of two isoforms, namely alpha1 subunit (AMPKα1) and alpha2 subunit (AMPKα2). Known as a mammalian cell energy sensor, AMPK could regulate cellular energy metabolism via sensing the alterations of energy balance, such as oversupply or lack of glucose and fatty acid. Recent studies have suggested that AMPK could also regulate many other biological processes, including cell cycling, inflammation, protein synthesis, and so on. Our previous study has demonstrated that oversupply of fatty acid could decrease AMPKα expression to significantly lower levels in rodents’ skeletal muscles, and such impairment of AMPKα is a key mechanism of fat-induced insulin resistance.Citation14 Besides oversupply of fatty acid, overload of protein, glucose, and ethanol is also known to impair AMPK signaling.Citation19–21 Therefore, we decided to investigate the role of AMPK in the dysfunction of MCs that was induced by high glucose.
These results showed that high-glucose treatment lead to a significant decrease of AMPKα expression, and are in a time-dependent manner, evidenced by 26% decrease after 12 h and 44% decrease after 24 h of high-glucose treatment, respectively. High glucose also markedly reduced mRNA expression of both AMPKα1 and AMPKα2 in a time-dependent manner. These findings suggested that both AMPKα1 and AMPKα2 might be involved in high-glucose-induced decrease of AMPKα expression. However, such results are inconsistent with that of our previous study. In rats’ skeletal muscles, oversupply of dietary fat resulted in a significant decrease of AMPKα2 mRNA levels, whereas it had no effects on AMPKα1 mRNA levels.Citation14 Such discrepancy might be due to the different tissue distribution of the two isoforms. When compared with AMPKα1, which was ubiquitously expressed in mammalian tissues, AMPKα2 was found to predominantly locate in skeletal muscle, heart, and liver and contribute primarily to AMPK activities.Citation12 Similar to our present findings that both AMPKα1 and AMPKα2 participate in the decrease of AMPKα expression by high-glucose treatment, a recent study also showed that both the expression and activity of these two isoforms could be detected in rat glomeruli.Citation22 AMPK activation was further estimated by detecting its active form, p-AMPKα expression. When compared with the control, high-glucose treatment resulted in a significant decrease of p-AMPKα levels, indicating that besides decreasing AMPKα expression, high glucose might also inhibit AMPK activation. Furthermore, the inhibitory effect on AMPK activation of high glucose is time dependent, evidenced by a 36% decrease after 12 h and 49% decrease after 24 h treatment of high glucose.
ACC is a citrate-activated enzyme that can be phosphorylated in vitro by AMPK. In addition, as the major biological effect of AMPK is to regulate intracellular fatty acid oxidation via p-ACC, we consider the latter could be a good measure of the AMPK activation.Citation9,14 Our results showed that high glucose leads to a significant decrease of p-ACC levels time dependently and that high-glucose treatment could inhibit AMPK activation.
AICAR is a widely used AMPK activator, and its AMPK-activating effects were also demonstrated in this study. When compared with cells under high-glucose incubation for 24 h, addition of AICAR induced a 80% elevation of p-AMPKα levels. Accompanied by the activation of AMPK, AICAR treatment also resulted in a 12.5% decrease of high-glucose-induced proliferation of MCs. At the same time, collagen IV synthesis also decreased by 15% after AICAR treatment. Such results suggested that AMPK activation might effectively ameliorate the dysfunction of MCs by high glucose. Furthermore, the effectiveness of AICAR on ameliorating high-glucose-induced dysfunction of MCs also indicated that AMPK activation might be a novel intervening method for diabetic nephropathy.
In conclusion, high-glucose treatment could inhibit both the expression and activation of AMPKα in rat MCs; and AMPK activation by AICAR could effectively ameliorate proliferation of MCs and ECM synthesis induced by high glucose. These results suggested an important role of AMPK in high-glucose-induced dysfunction of MCs and could provide novel theoretical basis for further research and clinical intervention and treatment of diabetic nephropathy.
ACKNOWLEDGMENT
This research was supported by the Shandong Natural Science Foundation (Q2006C15) and Shandong Doctoral Foundation of China (2006BSB14022).
Declaration of interest:The authors report no conflicts of interest. The authors alone are responsible for the content and writing of the paper.
REFERENCES
- Hovind P, Tarnow L, Rossing K, . Decreasing incidence of severe diabetic microangiopathy in type 1 diabetes. Diabetes Care. 2003;26:1258–1264.
- Bloomgarden ZT. Diabetic nephropathy. Diabetes Care. 2005;28:745–751.
- Brenner BM, Cooper ME, de Zeeuw D, . Effects of losartan on renal and cardiovascular outcomes in patients with type 2 diabetes and nephropathy. N Engl J Med. 2001;345:861–869.
- Lewis EJ, Hunsicker LG, Clarke WR, . Renoprotective effect of the angiotensin-receptor antagonist irbesartan in patients with nephropathy due to type 2 diabetes. N Engl J Med. 2001;345:851–860.
- The Diabetes Control and Complications Research Group. Effect of intensive therapy on the development and progression of diabetic nephropathy in the Diabetes Control and Complications Trial. Kidney Int. 1995;47:1703–1720.
- Schena FP, Gesualdo L. Pathogenetic mechanisms of diabetic nephropathy. J Am Soc Nephrol. 2005;16:S30–S33.
- Eddy AA. Progression in chronic kidney disease. Adv Chronic Kidney Dis. 2005;12:353–365.
- Schnaper HW, Hayashida T, Hubchak SC, Pobcelet AC. TGF-beta signal transduction and mesangial cell fibrogenesis. Am J Physiol Renal Physiol. 2003;284:F243–F252.
- Rutter GA, Silva Xavier DA, Leclerc I. Roles of 5-AMP-activated protein kinase (AMPK) in mammalian glucose homoeostasis. Biochem J. 2003;375:1–16.
- Myung-Ja L, Denis F, Meenalakshmi MM, . A role for AMP-activated protein kinase in diabetes-induced renal hypertrophy. Am J Physiol Renal Physiol. 2007;292:F617–F627.
- Hiroyuki M, Barry JG, Motoyuki I, Eiichi A. AMPK and cell proliferation—AMPK as a therapeutic target for atherosclerosis and cancer. J Physiol. 2006;574:63–71.
- Hardie DG. The AMP-activated protein kinase pathway—New players upstream and downstream. J Cell Sci. 2004;117:5479–5487.
- Park SH, Gammon SR, Knippers JD, . Phosphorylation-activity relationships of AMPK and acetyl-CoA carboxylase in muscle. J Appl Physiol. 2002;92:2475–2482.
- Liu Y, Wan Q, Guan QB, Gao L, Zhao JJ. High-fat diet feeding impairs both the expression and activity of AMPKa in rats’ skeletal muscle. Biochem Biophys Res Commun. 2006;339:701–707.
- Bieke FS, De Vriese AS, Flyvbjerg A. From hyperglycemia to diabetic kidney disease: The role of metabolic, hemodynamic, intracellular factors and growth factors/cytokines. Endocrinol Rev. 2004;25:971–1010.
- Myung JL, Denis F, Mariappan M, . A role for AMP-activated protein kinase in diabetes-induced renal hypertrophy. Am J Physiol Renal Physiol. 2007;292:F617–F627.
- Philippe GC, Irene L, Gingras D, Bendayan M. Control of glycogen synthase through ADIPOR1-AMPK pathway in renal distal tubules of normal and diabetic rats. Am J Physiol Renal Physiol. 2008;294:F881–F889.
- Wyatt M, Zanescu DI, Sood V, Beale EG, Prabhakar SS. The role of 5’-AMP-activated protein kinase (AMPK) in diabetic nephropathy: A new direction? Curr Enzyme Inhib. 2009;5:44–50.
- Eduardo RR, José RP, Maria Fernanda AF, . A central role for neuronal AMP-activated protein kinase (AMPK) and mammalian target of rapamycin (mTOR) in high-protein diet–induced weight loss. Diabetes. 2008;57:594–605.
- Feng L, Gao L, Guan QB, . Long-term moderate ethanol consumption restores insulin sensitivity in high-fat-fed rats by increasing GLUT4 in the adipose tissue by AMPK activation. J Endocrinol. 2008;199:95–104.
- Salt IP, Johnson G, Ashcroft SJ, Hardie DG. AMP-activated protein kinase is activated by low glucose in cell lines derived from pancreatic beta cells, and may regulate insulin release. Biochem J. 1998;335:533–539.
- Cammisotto PG, Bendayan M. Adiponectin stimulates phosphorylation of AMP-activated protein kinase alpha in renal glomeruli. J Mol Histol. 2008;39:579–584.
- Yi L, Lei J, Zun CL, . Emodin ameliorates high-glucose induced mesangial p38 over-activation and hypocontractility via activation of PPARγ. Exp Mol Med. 2009;41:648–655.
- Wang R, Wan Q, Zhang Y, . Emodin suppresses interleukin-1beta induced mesangial cells proliferation and extracellular matrix production via inhibiting p38 MAPK. Life Sci. 2007;80:2481–2488.