Abstract
The pathogenesis of glycerol-induced myoglobinuric acute renal failure involves ischemia, vascular congestion and reactive oxygen metabolites. In this study, we have investigated for the first time, the role of ferulic acid in attenuating glycerol-induced nephrotoxicity. Male Wistar rats were injected intramuscularly with 8 mL/kg body weight of 50% glycerol, glycerol + ferulic acid at the dose of 5, 10, 15, 20 and 25 mg/kg body weight. After 24 h, the rats were sacrificed and the kidneys were removed for histological and immunohistochemical studies. Furthermore, determinations of lipid peroxidation (LPO) as well as antioxidant enzymes were also analyzed; blood, urine samples were collected in order to quantify renal function and nitric oxide generation, respectively. Glycerol-induced rats showed a significant increase in the level of urinary markers assessed in serum as well as kidney and these were reversed upon ferulic acid treatment. A significant increase in urine nitric oxide, serum as well as kidney LPO, decrease in activities of superoxide dismutase, catalase, glutathione peroxidase, glutathione-S-transferase and reduced glutathione were observed in glycerol-induced rats. Immunohistochemical study in glycerol-induced rats demonstrated an increase in the level of nuclear factor-kappaB (NF-κB). All these effects induced by glycerol were reduced upon treatment with ferulic acid in a dose-dependent manner. To conclude, ferulic acid enhances antioxidants and decreases NF-κB, thereby protecting the cells against stress induced by glycerol.
Introduction
Acute renal failure is characterized by deterioration of renal function over a period of hours to days. This results in the failure of the kidney to excrete nitrogenous waste products to maintain fluid and electrolyte homeostasis.Citation1–4 Most acute intrinsic renal failure is induced by ischemia and/or nephrotoxins, toxic agents such as mercuric chloride, amino glycosides, cisplatin, cyclosporine and glycerol.Citation5–9 Nephrotoxic acute renal failure represents the end result of diverse, overlapping biological pathways such as drug-induced renal vasoconstriction, access of drugs to cellular target via specific transport pathways, intracellular toxin processing activation of overlapping and possible integrated injury promoting pathways.Citation7 Interference with any of these processes may lead to cell injury and acute renal failure.
The transcription factors belonging to nuclear factor-kappaB (NF-κB)/Rel transcription family play a control role in inflammation by its ability to activate pro-inflammatory genes.Citation10 NF-κB has been shown to be highly activated in many human inflammatory diseases and is well-known to trigger pro-inflammatory cytokines, chemokines and adhesion molecules.Citation11
The mechanism of glycerol-induced nephrotoxicity is not completely known. However, studies have implicated that reactive oxygen metabolites proved to be the key mediators of tissue injury. Among the targets of oxidative stress, the peroxidation of lipids leads to spread of free radical reactions, thus lipid peroxidation (LPO) is considered as a biochemical hallmark of oxidative stress.Citation12–14 Abnormal production of such molecules may damage macromolecules, induce cellular injury as well as necrosis via several mechanisms, including protein denaturation and DNA damage.Citation15,Citation16 Accordingly, administration of compounds with antioxidant activity has been successfully used to prevent glycerol-induced nephrotoxicity.Citation17,Citation18 However, none of these strategies were found to be suitable/safe for clinical practice.
The large number of naturally occurring plant products exert their protective effects in glycerol-induced nephrotoxicity, mainly by scavenging free radicals and modulating antioxidant defense system. Recent studies have shown that proanthocyanidins extracted from seeds of grapes,Citation19 naringin, a bioflavonoid derived from grapes;Citation20 catechin, a flavonoid present in green and black tea;Citation21 quercetin, an extract from the plant Ginco bilobaCitation22 and rich red wine,Citation23 ameliorate myoglobin, attenuated renal functions due to their antioxidant and free radicals scavenging properties.
Ferulic acid (FA; 4-hydroxy-3-methoxycinnamic acid) is a plant constituent well-known to exhibit strong effects on scavenging free radicals and antioxidants activity.Citation24 FA is reported to have many pharmacological effects such as anti-inflammatory, anticancer, antidiabetic, anti-atherogenic and neuroprotective.Citation25 It also protects membranes from LPO, neutralized alkoxyl, peroxyl radicals,Citation26 scavenges hydroxyl radicals,Citation27 nitric oxide,Citation28 peroxynitriteCitation29,Citation30 and superoxide radicals.Citation31,Citation32 Recent studies have shown that NCX 2057, a nitric oxide releasing iNOS expression in raw 2647 macrophagesCitation33 as well as FA inhibit the azoxymethane-induced colon carcinogenesis in rat.Citation34 More recently, we reported that FA has shown to protect against alloxan-induced diabetes in mice.Citation14 However, the literature pertaining to the mechanism involved in protective effects of FA on glycerol-induced nephrotoxicity is scanty.
Therefore, in continuation of search for a potential agent to modulate glycerol-mediated renal oxidative stress and damage, this is the first study to demonstrate notable efficacy of FA administrated in glycerol-induced nephrotoxicity of Wistar rats.
Materials and methods
Experimental animals
Male Wistar albino rats (100–150 g) were procured from the National Institute of Nutrition (Hyderabad, India). All experiments were approved by the Institutional Animal Ethical Committee (IAEC), India, guidelines. Animals were housed in an air-conditioned room at 22 ± 10 °C with a lighting schedule of 12 h light and 12 h dark. Rats were fed a balanced commercial rat diet (Hindustan UniLever, Mumbai, India) and water was given ad libitum.
Experimental design
The rats were randomized into seven groups (six in each):
Group I – Controls
Group II – Glycerol exposure 50% (one intramuscular injection; 8 mL kg/body weight)
Group III – The animals were administered with 50% glycerol (one intramuscular injection; 8 mL/kg body weight) simultaneously treated with 5 mg/kg body weight FA (oral dose)
Group IV – The animals were administered with 50% glycerol (one intramuscular injection; 8 mL/kg body weight) simultaneously treated with 10 mg/kg body weight FA (oral dose)
Group V – The animals were administered with 50% glycerol (one intramuscular injection; 8 mL/kg body weight) simultaneously treated with 15 mg/kg body weight FA (oral dose)
Group VI – The animals were administered with 50% glycerol (one intramuscular injection; 8 mL/kg body weight) simultaneously treated with 20 mg/kg body weight FA (oral dose)
Group VII – The animals were administered with 50% glycerol (one intramuscular injection; 8 mL/kg body weight) simultaneously treated with 25 mg/kg body weight FA (oral dose)
Tissue preparation
After treatment, rats were sacrificed by means of intraperitoneal dose of pentobarbital at 50 mg/kg body weight, given. Tissues were immediately dissected out, washed in ice-cold saline to remove blood and frozen at −70 °C. Before use, tissue samples (kidney) of each group were homogenized in 10% 0.1 M Tris–HCl buffer (pH 7.2) and centrifuged (12,000 rpm, 30 min, 4 °C). The supernatants obtained were used for the analysis. To obtain serum, blood was collected by cardiac puncture before sacrifice, allowed to clot at room temperature (RT) and then centrifuged (3000 rpm, 5 min). The supernatant (serum) was frozen at −80 °C till use. Urine from rats was collected by individually placing the animals on a metabolic cage. Urine was immediately used for analyses.
Biochemical estimations
Estimation of urea
Urea was estimated by the method described previously by Natelson et al.Citation35 To 0.1 mL of serum and kidney homogenates, 3.3 mL of double-distilled water, 0.3 mL of 10% sodium tungstate and 0.3 mL of 0.67 N sulfuric acids were added. The samples were centrifuged (4000 rpm, 15 min, 4 °C) and to 1 mL of the supernatant, 1 mL of double-distilled water, 0.4 mL of diacetyl monoxime reagent and 0.6 mL of sulfuric acid–phosphoric acid mixture were added. The samples were incubated in a boiling water bath for 30 min, cooled to room temperature and the absorbance was read at 480 nm in a Shimadzu UV-160 A spectrophotometer (Tokyo, Japan). A set of standard urea solutions was also treated similarly and the values were expressed in milligram per deciliter samples.
Estimation of uric acid
Uric acid was estimated using the method described previously by Caraway.Citation36 To 0.6 mL of serum and kidney homogenates, 5.4 mL of dilute tungstic acid was added, mixed and centrifuged (4000 rpm, 15 min, 4 °C). To 3 mL of the supernatants, 0.6 mL of sodium carbonate and 0.6 mL of phosphotungstic acid reagent were added, mixed and incubated in a water bath at 25 °C for 10 min. At the end of incubation, absorbance was read at 700 nm in a Shimadzu UV spectrophotometer. A set of standard uric acid solutions was also treated similarly and the values were expressed in milligram per deciliter samples.
Estimation of creatinine
Creatinine was estimated using the method described previously by Broad and SirotaCitation37 using Jaffe’s reaction. To 1.0 mL of serum and kidney homogenates, 8.0 mL of double-distilled water, 0.5 mL of 2/3 N sulfuric acid and 0.5 mL of 10% sodium tungstate were added. This mixture was centrifuged at 4000 rpm for 15 min at 4 °C, 5.0 mL of the clear supernatant was taken to which 1.5 mL of saturated picric acid and 1.5 mL of 0.75 N sodium hydroxide were added. The absorbance was read at 460 nm after 15 min in a Shimadzu UV-160 A spectrophotometer. Standard and blank were also processed similarly and the creatinine levels were expressed in milligram per deciliter.
Determination of LPO
LPO was determined using the method described previously by Ohkawa et al.Citation38 The principle of this method being that malondialdehyde (MDA), an end-product of LPO, reacts with thiobarbituric acid (TBA) to form a pink chromogen. For this assay, 0.2 mL of 8.1% SDS, 1.5 mL of 20% acetic acid (pH 3.5) and 1.5 mL of 0.8% thiobarbituric acid aqueous solution were added successively in a reaction tube. To this reaction mixture, 0.2 mL of the tissue homogenates (serum and kidney) was added and heated in a boiling water bath for 60 min. After cooling to room temperature, 5 mL of butanol: pyridine (15:1, v/v) solution was added. The mixture was then centrifuged (5000 rpm, 15 min), following which the upper layer was separated and intensity of resulting pink color was read at 532 nm in a spectrophotometer. Tetramethoxypropane was used as an external standard and the level of lipid peroxidase was expressed as nmol of MDA formed per gram wet weight.
Quantitative analysis of antioxidant status
Prior to biochemical analysis, tissue samples (kidney) of each group were homogenized in 10% 0.1 M Tris–HCl buffer (pH 7.2) and centrifuged (12,000 rpm, 30 min, 4 °C). The supernatant obtained was used for the analysis of enzymatic as well as non-enzymatic antioxidants. Protein in each sample was estimated using the method described previously by Lowry et al.Citation39
Catalase
Catalase (CAT) activity was determined using the method described by Sinha.Citation40 In this test, dichromatic acetic acid was reduced to chromic acetate when heated in the presence of H2O2, with the formation of perchloric acid as an unstable intermediate. In the test, the green color development was read at 590 nm against blank in a spectrophotometer. The activity of CAT was expressed as μmole of H2O2 consumed per milligram protein per minute.
Superoxide dismutase
Superoxide dismutase (SOD) activity was determined using the method described by Marklund and Marklund.Citation41 In this test, the degree of inhibition of pyrogallol auto-oxidation by tissue supernatants (kidney and serum) was measured. The change in absorbance was read at 470 nm against blank every 3 min on a spectrophotometer and the enzyme activity was expressed as units per milligram protein.
Glutathione peroxidase
The glutathione peroxidase (GPx) activity was determined essentially as described by Rotruck et al.Citation42 The rate of glutathione oxidation by H2O2, as catalyzed by the GPx present in the supernatant was determined and the color developed was read against a reagent blank at 412 nm in a spectrophotometer. In the test, the enzyme activity was expressed as μmole of glutathione oxidized per milligram protein per minute.
Reduced glutathione
The glutathione (GSH) content was estimated using the method of Moron et al.Citation43 Tissue homogenates (kidney and serum) was centrifuged (5000 rpm, 15 min, 4 °C) and to the resulting supernatants, 0.5 mL of 10% trichloroacetic acid was added. This reaction mixture was again centrifuged and the resulting protein-free supernatant was allowed to react with 4 mL of 0.3 M Na2HPO4 (pH 8.0) and 0.5 mL of 0.04% (w/v) 5.5-dithiobis-2-nitrobenzoic acid. The absorbance of the resulting yellow color was read spectrophotometrically at 412 nm and the results were expressed as μmol per gram wet weight.
Glutathione-S-transferase
The glutathione-S-transferase (GST) activity was determined by the method of Habig et al.Citation44 The conjugation of GSH with 1-chloro-2,4-dinitrobenzene (CDNB), a hydrophilic substrate was observed spectrophotometrically at 340 nm and the results were expressed in conjugate per μmol of CDNB with GSH per minute.
Estimation of nitric oxide generation
The generation of nitric oxide in urine was determined using the method of Ozbek et al.Citation45 with slight modifications. Briefly, 100 μL of clear urine was mixed with 150 μL Tris–HCl (pH 7.4), incubated with 5 μL of 0.01 U nitrate reductase (Sigma, St. Louis, MO) and 10 μL of 2 mM β-NADH (Sigma, St. Louis, MO), for 20 min at 22 °C in dark with frequent shaking. After this, 50 μL of 1% sulfanilamide (Sigma, St. Louis, MO), followed by 50 μL of 0.1% naphthyl ethylenediamine dihydrochloride (Sigma, St. Louis, MO) were added and incubated for 10 min at RT. At the end of incubation, the samples were centrifuged (12,000 rpm, 15 min, 4 °C) and absorbance of the supernatants were read at 540 nm in a Shimadzu (UV-160 A) spectrophotometer against a reagent blank. The nitrite (=NO) generated by the urine was determined using sodium nitrite in a standard curve and the amount of nitrite present was expressed as μmol nitrite per gram of urine.
Histological examinations
The kidney tissues of rats were fixed in 10% formalin solution for 24 h and embedded in paraffin wax. Tissues were then sectioned at 5 μm, stained with hematoxylin and eosin (H & E). A semiquantitative evaluation of renal tissues was accomplished by scoring the degree of severity according to the formerly published criteria by Teixeira et al.Citation46 For each renal section, whole slide was examined under bright field using a Carl Zeiss Axioskop microscope (Germany).
Immunohistochemistry
Immunohistochemistry was carried out using the method previously explained by Manikandan et al.Citation47 on 5 -μm paraffin-embedded tissue sections on poly-l-lysine-coated glass slides. The tissue sections were deparaffinized by placing the slides in an oven at 60 °C for 10 min and then rinsed twice in xylene for 10 min each. The slides were then hydrated in graded ethanol series (100, 90, 70, 50 and 30% for 10 min each) and then finally in double-distilled water for 10 min. The sections were incubated with 1% H2O2 in double-distilled water for 15 min at 22 °C, to quench endogenous peroxidase activity. The sections were rinsed with Tris–HCl containing 150 mM NaCl (pH 7.4) and blocked in blocking buffer: tris-buffered saline (TBS), 0.05% Tween 20, 5% non-fat dry milk for 1 h at 22 °C. After washing with TBS containing 0.05% Tween 20, the sections were incubated with primary antibody, rabbit polyclonal IgG to rat NF-κB (BD Biosciences, San Jose, CA) at a dilution of 1:500, overnight at 4 °C. After incubation, the tissue sections were rinsed with TBS containing 0.05% Tween 20 twice, incubated with secondary antibody, goat anti-rabbit IgG-HRP conjugate (Genei, Bangalore, India), at a dilution of 1:3000, for 1 h at 4 °C. After another wash with TBS containing 0.05% Tween 20, the immunoreactivity was developed with 0.05% diaminobenzidine (DAB) and 0.01% H2O2 for 1–3 min. The tissue sections were observed for brown color formation under bright field using a Carl Zeiss Axioskop microscope.
Statistical analysis
Quantitative data were reported as mean ± SD and the statistical significance of observed differences between values in different groups were determined by Student’s t test where p < 0.05 and p < 0.001 was regarded as statistically significant.
Results
Biochemical assays
Renal function assessment
Urea, uric acid and creatinine levels were observed in serum and kidney. After glycerol exposure, group II animals exhibited a significant increase in the levels of urea, uric acid and creatinine, when compared to control (group I; p > 0.05) animals (), whereas, FA treatment (groups III and IV) failed to produce any effect. Interestingly, in animals treated with FA for 15 mg/kg body weight (group V), 20 mg/kg body weight (group VI), there was a significant (p > 0.001) decrease in the levels of urea, uric acid and creatinine when compared to group II animals. Such a decrease was also seen in group VII, where animals were treated with FA for 25 mg/kg body weight, showed a significant (p > 0.05) decrease in the levels of urea, uric acid and creatinine.
Table 1. The effect of FA on basic biochemical markers in normal and treated Wistar albino rats.
Levels of LPO
The administration of glycerol caused a significant increase in malondialdehyde concentrations in serum and kidney (; p > 0.05) indicating glycerol-induced oxidative stress (group II). However, in groups III and IV animals, treated with FA for 5 and 10 mg/kg body weight, respectively, failed to produce any change. Interestingly, in animals treated with FA for 15 mg/kg body weight (group V), 20 mg/kg body weight (group VI), there was a significant (p > 0.001) decrease in the LPO levels when compared with group II animals. Such a decrease was observed in group VII animals too (p > 0.05; ).
Figure 1. Effect of FA on the lipid peroxidase levels in serum and kidney of Wistar rats. Each bar represents mean ± SD of five determinations using samples from different preparations. The lipid peroxidase levels in serum and kidney of glycerol-exposed animals were significantly different from the control. The difference in lipid peroxidase levels observed between groups I and II and groups II and III--VII animals were statistically significant at ap < 0.001 and bp < 0.05.
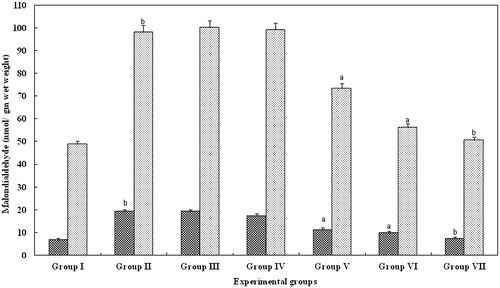
Superoxide dismutase
SOD activity was measured in the serum, kidney as it is a specific scavenger of superoxide anion and glycerol. The mean SOD activity in serum and kidney of group II were significantly reduced (p > 0.05). However, in groups III (5 mg/kg body weight) and IV (10 mg/kg body weight), there was no significant change. In contrast, in serum and kidney belonging to groups V (15 mg/kg body weight) and VI (20 mg/kg body weight), there was a significant (p > 0.001) increase in the level of SOD activity when compared with group II animals. However, in group VII, animals treated with FA for 25 mg/kg body weight, there was a significant (p > 0.05) increase and the levels were equal to that observed with control serum and kidney (group I; ).
Figure 2. Effect of FA on the superoxide dismutase levels in serum and kidney of Wistar rats. Each bar represents mean ± SD of five determinations using samples from different preparations. The superoxide dismutase levels in serum and kidney of glycerol-exposed animals were significantly different from the control. The difference in superoxide dismutase levels observed between groups I and II and groups II and III--VII animals were statistically significant at ap < 0.001 and bp < 0.05.
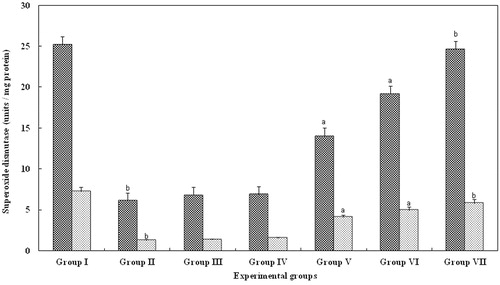
Catalase
CAT is a specific scavenger of hydrogen peroxide and was significantly reduced in glycerol-treated serum and kidney (group II; p > 0.05) when compared to control (group I), whereas, FA treatment (groups III--IV) failed to produce any changes. Interestingly, level of catalase in groups V (15 mg/kg body weight) and VI (20 mg/kg body weight) animals were significantly increased (p > 0.001) when compared with group II animals. In contrast, animals treated with FA at 25 mg/kg body weight showed a significant (p > 0.05) increase and the levels were recovered to that of the control animals ().
Figure 3. Effect of FA on the catalase levels in serum and kidney of Wistar rats. Each bar represents mean ± SD of five determinations using samples from different preparations. The catalase levels in serum and kidney of glycerol-exposed animals were significantly different from the control. The difference in catalase levels observed between groups I and II and groups II and III--VII animals were statistically significant at ap < 0.001 and bp < 0.05.
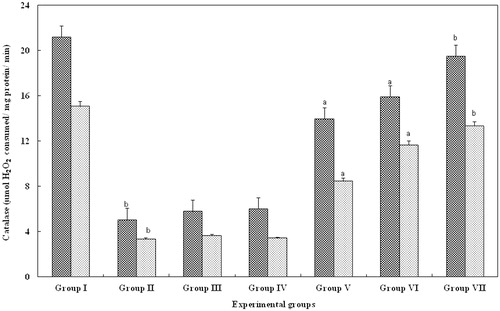
Glutathione peroxidase
GPx is important for scavenging hydrogen peroxide and along with catalase is a potent barrier against LPO in the serum and kidney. The GPx level in serum and kidney of group II were significantly decreased (p > 0.05), whereas, GPx levels in groups III and IV (10 and 20 mg/kg body weight, respectively) animals were not significantly increased, when compared to group II animals. Interestingly, levels of GPx in groups V (15 mg/kg body weight) and VI (20 mg/kg body weight) animals were significantly increased (p > 0.001) when compared with group II animals. However, in group VII, animals treated with FA for 25 mg/kg body weight, there was a significant increase (p > 0.05) which was equal to that observed with control serum and kidney of group I ().
Figure 4. Effect of FA on the GPx levels in serum and kidney of Wistar rats. Each bar represents mean ± SD of five determinations using samples from different preparations. The GPx levels in serum and kidney of glycerol-exposed animals were significantly different from the control. The difference in GPx levels observed between groups I and II and groups II and III--VII animals were statistically significant at ap < 0.001 and bp < 0.05.
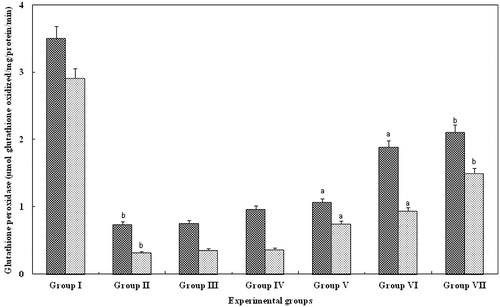
Glutathione-S-transferase
GST, an important component involved in the recycling of glutathione levels were found to be reduced in the serum and kidney of group II (glycerol alone). However, in groups III (5 mg/kg body weight) and IV (10 mg/kg body weight), there was no significant changes. In contrast, serum and kidney belonging to groups V (15 mg/kg body weight) and VI (20 mg/kg body weight), showed a significant (p > 0.001) increase in the level of GST activity when compared with group II animals. However, in group VII animals treated with FA for 25 mg/kg body weight, there was a significant (p > 0.05) increase and the levels were equal to that observed with control serum and kidney (group I; ).
Figure 5. Effect of FA on the CAT levels in serum and kidney of Wistar rats. Each bar represents mean ± SD of five determinations using samples from different preparations. The CAT levels in serum and kidney of glycerol-exposed animals were significantly different from the control. The difference in glutathione-S-transferase levels observed between groups I and II and groups II and III--VII animals were statistically significant at ap < 0.001 and bp < 0.05.
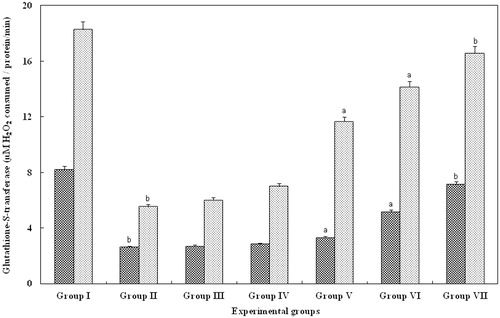
Reduced glutathione
The GSH activity in tissue samples (serum and kidney) of group II were significantly (p < 0.05; ) lower than control animals. In contrast, in groups treated with FA (III and IV) reduced glutathione failed to produce any significant change. Interestingly, levels of GSH in groups V (15 mg/kg body weight) and VI (20 mg/kg body weight) animals were significantly increased (p > 0.001) when compared with group II animals. However, in group VII, animals treated with FA for 25 mg/kg body weight, there was a significant increase (p > 0.05), which was equal to that observed with control serum and kidney of group I ().
Figure 6. Effect of FA on the reduced glutathione levels in the serum and kidney of Wistar rats. Each bar represents mean ± SD of five determinations using samples from different preparations. The reduced glutathione levels in serum and kidney of glycerol-exposed animals were significantly different from the control. The difference in reduced glutathione levels observed between groups I and II and groups II and III--VII animals were statistically significant at ap < 0.001 and bp < 0.05.
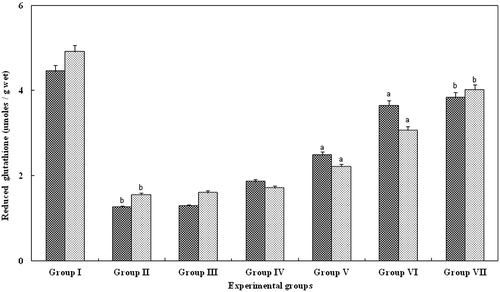
Nitric oxide
Nitric oxide generation was measured in urine to understand the relative contribution of this free radical glycerol-induced nephrotoxicity. The administration of glycerol caused a significant increase in nitric oxide concentrations in urine (; p > 0.05) indicating glycerol-induced oxidative stress (group II). However, in groups III and IV animals treated with FA for 5 and 10 mg/kg body weight, respectively, failed to produce any change. Interestingly, in animals treated with FA for 15 mg/kg body weight (group V) and 20 mg/kg body weight (group VI), there was a significant (p > 0.001) decrease in the nitric oxide levels when compared with group II animals. Such a decrease was observed in group VII animals (p > 0.05; ).
Table 2. The effect of FA on nitrite in normal and treated Wistar albino rats.
Histopathology
The histological studies of kidney from glycerol-treated rats showed damaged glomerular structure, hemorrhagic and hyaline cast deposits, tubular necrosis apical blabbing, vacuolar degeneration changes in the epithelial cells, cellular proliferation with fibrosis, thickening of capillary walls and glomerular tuft atrophy (). However, in groups III and IV there was no significant change (Figure 7C and D). Alterations were found to be minimal in the animals treated with FA for 15 and 20 mg/kg body weight (groups V and VI; ). Animals treated with FA for 25 mg/kg body weight (group VII; ) showed a normal architecture similar to the control animals (Figure 7A).
Figure 7. Hematoxylin and eosin-stained kidney sections of Wistar rats. (A) Physiological saline-injected rat (group I). (B) Animals administered with glycerol alone (group II). (C) The animals were administered with glycerol simultaneously treated with 5 mg/kg body weight FA. (D) The animals were administered with glycerol simultaneously treated with 10 mg/kg body weight FA. (E) The animals were administered with glycerol simultaneously treated with 15 mg/kg body weight FA. (F) The animals were administered with glycerol simultaneously treated with 20 mg/kg body weight FA. (G) The animals were administered with glycerol simultaneously treated with 25 mg/kg body weight FA.
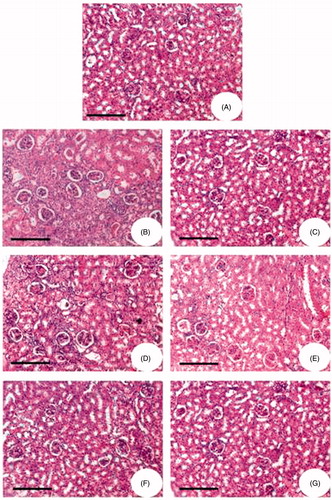
Immunohistochemistry
Immunohistochemical studies also showed that the staining for NF-κB was more intense in the renal cortex of glycerol-induced animals (groups II–V; ). In the case of glycerol alone administered animals (group II) the staining was very intense, when compared to control animals wherein there was no staining. In the case of FA-treated animals, groups III and IV also showed a similar response as that of group II animals. However, at higher concentrations of FA, the intensity of staining went down (group V) and was completely absent in groups VI and VII animals.
Figure 8. Immunohistochemistry of NF-κB in kidney of Wistar rats. Bright field photomicrographs show kidney sections from: (A) Physiological saline-injected rat (group I); (B) the animals administered with glycerol alone (group II); (C) the animals were administered with glycerol simultaneously treated with 5 mg/kg body weight FA; (D) the animals were administered with glycerol simultaneously treated with 10 mg/kg body weight FA; (E) the animals were administered with glycerol simultaneously treated with 15 mg/kg body weight FA; (F) the animals were administered with glycerol simultaneously treated with 20 mg/kg body weight FA; (G) the animals were administered with glycerol simultaneously treated with 25 mg/kg body weight FA. Kidney sections were preincubated with rabbit polyclonal IgG to rat NF-κB (1:500 dilution) and subsequently with goat anti-rabbit IgG-HRP conjugate (1:3000 dilution). The immunoreactivity was developed with 0.01% DAB and 2% H2O2.
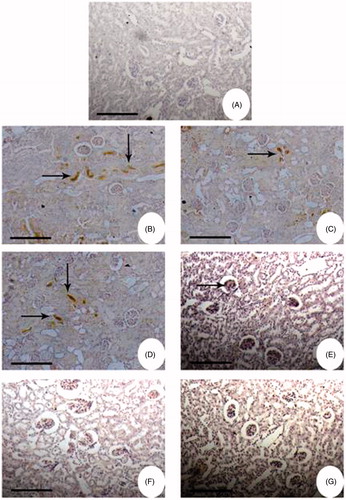
Discussion
The intramuscular administration of hypertonic glycerol induces myolysis and hemolysis. It affords a faithful and widely utilized model of heme protein-induced renal injury.Citation48,Citation49 The heme protein-induced renal injury represents the integrated effects of three major pathophysiologic mechanisms, namely, renal vasoconstriction, direct cytotoxicity and cast formation.Citation48,Citation49 The biochemical findings of induced renal injury have produced persuasive evidence incriminating oxidative stress and the catalytic effect of redox-active iron as important mechanisms for such injury.Citation48,Citation12,Citation13, The pigments (hemoglobin and myoglobin) themselves are unlikely to induce acute renal failure, but their presence within the systemic circulation during periods of acidosis, dehydration, shock or other conditions associated with reduced renal perfusion may lead to both direct toxic and hemodynamic abnormalities resulting in acute renal failure.Citation50
The mechanism by which glycerol injected intramuscularly or subcutaneously causes functional and anatomic changes of acute renal failure is unknown. Still several studies suggested that after glycerol infection, iron is released from heme pigments, namely, hemoglobin and myoglobin. This promotes free radical formation, LPO and renal dysfunction,Citation51,Citation52 which are the characteristics of nephrotoxicity. Therefore, it is necessary to raise a potential remedy to protect the glycerol-induced nephrotoxicity that would have a significant clinical consequence in increasing safety of the drug.
Phenolic compounds from dietary plants are known to be good scavengers of reactive oxygen species.Citation14 Thus in the present study, we assessed whether the nephrotoxic effects caused by acute administration of glycerol could be prevented or ameliorated by treatment with FA, a herbal compound which possesses a strong antioxidant property.Citation53,Citation54 Several dosage schemes have been reported for glycerol administration and an intramuscular injection dose of 8 mL/kg body weight for 24 h was used, which is an ideal dosage scheme reported to cause significant nephrotoxicity in rats.Citation55,Citation56
There are some experimental data suggesting that nephrotoxic drugs can alter the levels of kidney markers, glutathione and other antioxidant enzymes,Citation45,Citation16 which are commonly used to monitor the development and extent of renal tubular damage due to oxidative stress. The results of this study show that, glycerol administration to Wistar rats (group II) produced a typical pattern of nephrotoxicity which was manifested by increase in urinary marker enzymes. On the other hand, FA administration showed a significant decrease in the levels of creatinine, urea as well as uric acid in serum and kidney. The protective effect of FA on the kidney markers can be attributed to its antioxidant properties as it has been found that reactive oxygen species may be involved in the impairment of glomerular filtration rate.Citation57,Citation58
NO, a short-lived lipophilic molecule has been shown to play a crucial role in regulating local blood flow and aqueous out flow in blood vessels. NO levels were enhanced in the cisplatin-administrated group and such an effect has been recently reportedCitation59 and also glycerol-induced renal failure.Citation60 Administration of FA treated with glycerol-induced nephrotoxicity was significantly reduced in a dose-dependent manner.
In this study, glycerol-induced renal dysfunction was associated with significant increase in the LPO levels, while GSH, GPx, SOD, CAT and GST levels were reduced in the serum and kidney. Similar results were also observed in earlier study.Citation20 Depletion of renal GSH is one of the primary factors which permit LPO, suggested to be closely related to glycerol-induced tissue damage. Glycerol nephropathy was associated with low activities of antioxidant enzymes in renal cortex which could aggravate the oxidative damage. Our results show that FA treatment significantly attenuated the glycerol-mediated increase of LPO levels in serum and kidney. Furthermore, FA in a dose-dependent manner reversed the effect of glycerol by significantly increasing activities of GSH, GPx, SOD, CAT and GST content in serum and kidney tissues. The apparent protective effect might be due to the ability of FA to neutralize the increase in free radicals caused by glycerol. It should be noted that in relation to urea, uric acid and creatinine levels, the antioxidant enzymes were enhanced in animals that were administered with 25 mg/kg FA. This shows the protective effect of FA against oxidative stress-induced increase in urea, uric acid and creatinine, while it facilitated enhanced activity of antioxidant enzymes, thereby counteracting the effect of glycerol-induced free radicals.
The histological studies of kidney from glycerol-treated rats showed damaged glomerular structure, hemorrhagic and hyaline cast deposits, tubular necrosis as well as apical blabbing. Similar changes were also reported by Chander and colleaguesCitation61,Citation62 and these alterations were found to be minimal in the animals treated with FA for 15 and 20 mg/kg body weight (groups V and VI). Animals treated with FA for 25 mg/kg body weight (group VII) showed a normal architecture similar to the control animals, thus showing its curative effect against glycerol-induced tissue damage.
To further understand the protective role of FA in glycerol-induced nephrotoxicity, the expression of NF-κB was analyzed by immunohistochemistry. NF-κB is a highly conserved family of transcription factors that has a critical role in mediating inflammation, apoptosis and growth in chronic disease.Citation63 Our immunohistochemical studies also showed that the staining for NF-κB was more intense in the renal cortex of glycerol-induced animals (Group II). Free radicals released by damaged tissues and inflammatory cells are capable of inducing NF-κB activation.Citation64,Citation65 Activation of NF-κB might play a role in synthesis of inflammatory substances such as cytokines, growth factors, vascular adhesion molecules, intracellular adhesion molecules and enzymes. Interestingly, FA treatment in a dose-dependent manner led to a slight reduction in the expression of NF-κB, which further confirms its curative role against glycerol-induced nephrotoxicity.
In summary, we have confirmed that FA affords curative role against nephrotoxicity induced by glycerol exposure. According to our biochemical findings, which were supported by histopathological and immunohistochemistry analysis, administration of FA rescued the cells from effects of glycerol. These findings indicate that FA administration may reduce glycerol-induced renal injury. Therefore, we are proposing for the first time that FA might be a potential candidate against glycerol-induced nephrotoxicity via its antioxidant and anti-inflammatory properties.
Declaration of interest
The authors report no conflicts of interest. The authors alone are responsible for the content and writing of the paper.
References
- Finn WF. Diagnosis and management of acute tubular necrosis. Med Clin North Am. 1990;74:873–891
- Thadhani R, Pascual M, Bonventre JV. Acute renal failure. N Eng Med. 1996;334:1448–1460
- Brady HE, Singe GG. Acute renal failure. Lancet. 1995;346:1533–1540
- Mindel JA, Chertow GM. A practical approach to acute renal failure. Med Clin North Am. 1997;81:731–749
- Houghton DC, Plamp CE, De Fehr JM, Bennett WM, Porter G, Gilbert D. Gentamicin and tobramycin nephrotoxicity. A morphologic and functional comparison in the rat. Am J Pathol. 1978;93:137–152
- Baliga R, Ueda N, Walker PD, Shan SV. Oxidative mechanism in toxic acute renal failure. Am J Kidney Dis. 1997;29:465–477
- Zager RA. Pathogenetic mechanism in nephrotoxic acute renal failure. Semin Nephrol. 1997;17:3–14
- Natochin YV, Bakhteeva VT, Karpenko LA. Renal failure and nephrotoxic drug-induced disturbances in rat kidney tissue. Ren Fail. 1994;16:687–696
- Baliga R, Ueda N, Walker PD, Shah SV. Oxidant mechanisms in toxic acute renal failure. Drug Metab Rev. 1999;31:971–997
- Baldwin AS. The NF-kappa B and I kappa B proteins: new discoveries and insights. Annu Rev Immunol. 1996;14:649–683
- Tak PP, Firestein GS. NF-κB: a key role in inflammatory diseases. J Clin Invest. 2001;107:7–11
- Paller MS. Hemoglobin- and myoglobin-induced acute renal failure in rats: role of iron in nephrotoxicity. Am J Physiol. 1988;255:539–544
- Shah SV, Walker PD. Evidence suggesting a role for hydroxyl radical in glycerol-induced acute renal failure. Am J Physiol. 1988;255:F438–F443
- Manikandan R, Beulaja M, Thiagarajan R, et al. Protective effect of ferulic acid and resveratrol against alloxan-induced diabetes in mice. Eur J Pharmocol. 2012;690:226–235
- Baliga R, Zhan Z, Baliga M, Ueda N, Shah SV. In vitro and in vivo evidence suggesting a role for iron in cisplatin-induced nephrotoxicity. Kidney Int. 1998;53:394–401
- Parlakpinar H, Ozer MK, Sahna E, Vardi N, Cigremis Y, Acet A. Amikacin-induced acute renal injury in rats: protective role of melatonin. J Pineal Res. 2003;35:85–90
- Cuzzocrea S, Mazzon E, Dugo L, et al. A role for superoxide in gentamicin-mediated nephropathy in rats. Eur J Pharmacol. 2002;450:67–76
- Karahan I, Atessahin A, Yilmaz S, Ceribasi AO, Sakin F. Protective effect of lycopene on gentamicin-induced oxidative stress and nephrotoxicity in rats. Toxicology. 2005;215:198–204
- Stefanovic V, Ardaillou N, Vlahovic P, Placier S, Ronco P, Ardaillo R. Interferon-gamma induces dipeptidyl peptidase IV expression in human glomerular epithelial cells. Immunology. 1993;80:465–470
- Singh D, Chander V, Chopra K. Protective effect of naringin, a bioflavonoid on glycerol-induced acute renal failure in rat kidney. Toxicology. 2004;201:143–151
- Chander V, Singh D, Chopra K. Attenuation of glycerol-induced acute renal failure in rats by trimetazidine and deferoxamine. Pharmacology. 2003;67:41–48
- Chander V, Singh D, Chopra K. Reversal of experimental myoglobinuric acute renal failure in rats by quercetin, a bioflavonoid. Pharmacology. 2005;73:49–56
- Rodrigo R, Bosco C, Herrera P, Rivera G. Amelioration of myoglobinuric renal damage in rats by chronic exposure to flavonol rich red wine. Nephrol Dial Transplant. 2004;19:2237–2244
- Srinivasan M, Sudheer AR, Menon VP. Ferulic Acid: therapeutic potential through its antioxidant property. J Clin Biochem Nutr. 2007;40:92–100
- Mukhopadhyay A, Basu N, Ghatak N, Gujral PK. Anti-inflammatory and irritant activities of curcumin analogues in rats. Agents Actions. 1982;12:508–515
- Trombino S, Serini S, Di Nicuolo F. Antioxidant effect of ferulic acid in isolated membranes and intact cells: synergistic interactions with alpha-tocopherol, beta-carotene, and ascorbic acid. J Agric Food Chem. 2004;52:2411–2420
- Ogiwara T, Satoh K, Kadoma Y, et al. Radical scavenging activity and cytotoxicity of ferulic acid. Anticancer Res. 2002;22:2711–2717
- Wenk GL, McGann-Gramling K, Hauss-Wegrzyniak B. Attenuation of chronic neuroinflammation by a nitric oxide-releasing derivative of the antioxidant ferulic acid. J Neurochem. 2004;89:484–493
- Pannala AS, Razaq R, Halliwell B, Singh S, Rice-Evans CA. Inhibition of peroxynitrite dependent tyrosine nitration by hydroxycinnamate: nitration or electron donation? Free Radic Biol Med. 1998;24:594–606
- Dinis TC, Santosa CL, Almeida LM. The apoprotein is the preferential target for peroxynitrite-induced LDL damage protection by dietary phenolic acids. Free Radic Res. 2002;36:531–543
- Kaul A, Khanduja KL. Plant polyphenols inhibit benzoyl peroxide-induced superoxide anion radical production and diacylglyceride formation in murine peritoneal macrophages. Nutr Cancer. 1999;35:207–211
- Kikuzaki H, Hisamoto M, Hirose K, Akiyama K, Taniguchi H. Antioxidant properties of ferulic acid and its related compounds. J Agric Food Chem. 2002;50:2161–2168
- Ronchetti D, Impagnatiello F, Guzzetta M, et al. Modulation of iNOS expression by a nitric oxide releasing derivative of the natural antioxidant ferulic acid in activated RAW 264.7 macrophages. Eur J Pharmacol. 2006;532:162–169
- Han M, Wen JK, Zheng B, Zhang DQ. Acetyl britannilatone suppresses NO and PGE2 synthesis in RAW 264.7 macrophages through the inhibition of iNOS and COX-2 gene expression. Life Sci. 2007;75:675–684
- Natelson S, Scott ML, Beffa C. A rapid method for the estimation of urea in biological fluids. Am J Clin Path. 1951;21:275–281
- Caraway WI. Uric acid. In: Seligson D, ed. Standard Methods of Clinical Chemistry. New York: Academic Press; 1963:239–249
- Broad J, Sirota JH. Renal clearance of endogenous creatinine in man. J Clin Invest. 1948;27:646
- Ohkawa H, Ohishi N, Yagi K. Assay for lipid peroxides in animal tissues by thiobarbituric acid reaction. Anal Biochem. 1979;95:351–358
- Lowry OH, Rusebrough NJ, Farr AL, Randall RJ. Protein measurement with folin-phenol reagent. J Biol Chem. 1951;193:265–275
- Sinha AK. Colorimetric assay of catalase. Anal Biochem. 1972;47:389–394
- Marklund S, Marklund G. Involvement of the superoxide anion radical in the autoxidation of pyrogallol and a convenient assay for superoxide dismutase. Eur J Biochem. 1974;47:469–474
- Rotruck JT, Pope AL, Ganther HE, Swanson AB, Hafeman DG, Hoekstra WG. Selenium: biochemical role as a component of glutathione peroxidase. Science. 1973;179:588–590
- Moron MS, Depierre JW, Mannervik B. Levels of glutathione, glutathione reductase and glutathione-S-transferase activities in rat lung and liver. Biochim Biophys Acta. 1979;582:67–68
- Habig WH, Pabst MJ, Jacoby BW. Glutathione-S-transferase. The first enzymatic step in mercapturic acid formation. J Biol Chem. 1974;249:1730–1737
- Ozbek K, Turkoz Y, Sahna E, Ozugurlu F, Mizrak B, Ozbek M. Melatonin administration prevents the nephrotoxicity induced by gentamicin. BJU Int. 2000;85:742--746
- Teixeira RB, Kelly J, Alpert H, Pardo V, Vaamonde CA. Complete protection from gentamicin-induced acute renal failure in the diabetes mellitus rat. Kidney Int. 1982;21:600–612
- Manikandan R, Sundaram R, Thiagarajan R, Sivakumar MR, Meiyalagan V, Arumugam M. Effect of black tea on histological and immunohistochemical changes in pancreatic tissue of normal and streptozotocin-induced diabetic mice (Mus musculus). Microsc Res Tech. 2009;72:723–726
- Zager RA. Rhabdomyolysis and myoglobinuric acute renal failure. Kidney Int. 1996;49:314–325
- Dubrow A, Flamenbaum W. Acute renal failure associate with myoglobinuria. In: Brenner BM, Lazarus JM, eds. Acute Renal Failure. New York: Churchill Livingstone; 1988:279–293
- Dubrow A, Flamcnbanum W. Acute renal failure associated with myoglobin and hemoglobinuria. In: Soles K, Whelton A, eds. Acute Renal Failure. New York: Marcel Dekker; 1983:279–293
- Abassi ZA, Hoffman A, Better OS. Acute renal failure complicating muscle crush injury. Sem Nephrol. 1998;18:558–565
- Singh D, Chander V, Chopra K. Carvediol, an antihypertensive drug with antioxidant properties, protects against glycerol-induced acute renal failure. Nephrology. 2003;23:415–421
- Graf E. Antioxidant potential of ferulic acid. Free Radic Biol Med. 1992;13:435–448
- Scott BC, Butler J, Halliwell B, Aruoma OI. Evaluation of the antioxidant action of ferulic acid and catechins. Free Radic Res Commun. 1993;19:241–253
- Mohammad N, Zivar Y, Adebayo O. Role of PPAR-γ on the pathogenesis and vascular changes in glycerol-induced acute renal failure. Pharmacol Res. 2006;54:234–240
- de Jesus Soares T, Volpini RA, Francescato HD, Costa RS, da Silva CG, Coimbra TM. Effects of resveratrol on glycerol-induced renal injury. Life Sci. 2007;81:647–656
- Hughes AK, Stricklett K, Padilla E, Kohan DE. Effect of oxygen species on endothelin-1 production by human mesangial cells. Kidney Int. 1996;49:181–189
- Sarumathy K, Vijay T, Jayakanthia J, Dhana Rajan MS. A protective effect of Caesalpinia sappan (CS) on acetaminophen induced nephrotoxicity and oxidative stress in male albino rats. J Pharmacol Toxicol. 2011;2:2229–6859
- Debnath B, Babre N, Manjunath YS, Mallareddy V, Parameshwar P, Hariprasath K. Nephroprotective evaluation of ethanolic extract of the seeds of papaya and pumpkin fruit in cisplatin-induced nephrotoxicity. J Pharmaceut Sci Technol. 2010;2:241–246
- Hyun JY, Moon ME, Haeng SP, et al. Effects of chitosan oligosaccharide (COS) on the glycerol-induced acute renal failure in vitro and in vivo. Food Chem Toxicol. 2008;46:710–716
- Chander V, Chopra K. Protective effect of nitric oxide pathway in resveratrol renal ischemia--reperfusion injury in rats. Arch Med Res. 2006;37:19–26
- Chander V, Singh D, Chopra K. Catechin, a natural antioxidant protects against rhabdomyolysis-induced myoglobinuric acute renal failure. Pharmacol Res. 2003;48:503–509
- Wardle EN. Nuclear factor-κB for the nephrologist. Nephrol Dial Transplant. 2001;16:1764–1768
- Hill CS, Treisman R. Transcriptional regulation by extracellular signals: mechanisms and specificity. Cell. 1995;80:199–211
- Guijarro C, Egido J. Transcription factor-кB (NF-кB) and renal disease. Kidney Int. 2001;59:415–424