Abstract
The apoptosis of peritoneal mesothelial cells (PMCs) and peritoneal fibrosis may induce failure of peritoneal membrane function. The study explored the changes of apoptosis and fibrosis in PMCs under lipopolysaccharides (LPS) culture and investigated whether Ligustrazine can affect LPS-induced apoptosis and fibrosis. We found that exposure of rat PMCs to 5 mg·L−1 LPS for 24 h resulted in a significant induction of apoptosis and increased levels in Reactive oxygen species, and caspase-3 activity. Fibronectin, Collagen I, p-p38, and matrix metalloprotein-9 (MMP-9) levels were also significantly increased by LPS. But superoxide dismutase levels were remarkably decreased. Ligustrazine can restore the changes induced by LPS. The protective effect of Ligustrazine on LPS-induced apoptosis and fibrosis may act through inhibition of oxidative stress and p38/MAPKS, ROS/MMP-9 activation in PMCs.
Introduction
The number of end-stage kidney disease patients increases each year in China. Continuous ambulatory peritoneal dialysis (CAPD) has been used as a treatment for chronic renal failure for over three decades. However, the use of peritoneal dialysis (PD) for treating end-stage renal disease has serious limitations as it shows a reduced efficacy after some times.Citation1 Long-term PD is accompanied by functional and histopathological alterations in the peritoneum.Citation2,Citation3 Damage to the mesothelial cell monolayer, which acts as a permeability barrier in the peritoneal membrane, seems to be the basis of peritoneal membrane dysfunction in patients undergoing CAPD.Citation4 The apoptosis of peritoneal mesothelial cells (PMCs) may induce failure of peritoneal membrane function. PD has been linked to the presence of apoptotic PMCs in vivo,Citation5 and changes in the expression and/or activity of several factors that regulate apoptosis have been also observed in cultured peritoneal cells and during peritoneal injury in vivo.Citation6
The other characteristic feature of chronic peritoneal damage in PD is decreased ultrafiltration capacity associated with submesothelial fibrosis, accumulation of extracellular matrix, and neoangiogenesis.Citation7 Peritoneal fibrosis which was regarded as a serious complication of long-term PD leads to the failure of ultrafiltration and peritoneal function. However, effective interventions on the prevention and treatment of peritoneal fibrosis are rather deficient now. Long-delayed healing of infections is the major reason of peritoneal dysfunction and peritoneal fibrosis. Lipopolysaccharides (LPS), which is the only endotoxin from Escherichia coli, is caused mainly by catheter-related infections in long-term PD. So, LPS is one of the important reasons leading to peritonitis and peritoneal fibrosis.Citation8,Citation9 LPS can trigger apoptosis in vascular endothelial cells,Citation10 MC3T3-E1 Cells,Citation11 PC12 cells.Citation12 LPS as an independent factor may also trigger fibrosis.Citation13 LiuCitation14 found that LPS could induce epithelial-to-mesenchymal transition (EMT) and lead to increased EMT events in PMCs. This text explored the changes of apoptosis and fibrosis in PMCs under LPS exposure.
Ligustrazine (C8H12N2) is a purified and chemically identified alkaloid of the Chinese herb Ligusticum chuanxiong hort (Umbelliferae). Isolated alkaloids from Chuanxiong, and purified synthetic Ligustrazine, have been used in China as medicinal agents for 30 years. Ligustrazine has been used widely in China to treat cardiovascular disorders. Ligustrazine was reported to possess a very broad spectrum of pharmacological actions. It has dose-dependent protective effects against cisplatin-induced renal tubular cells apoptosisCitation15 and can reduce cell apoptosis induced by irradiation.Citation16 It can decrease the expression of Bcl-2 protein and apoptosis in rabbit articular chondrocytes in monolayer culture.Citation17 Moreover, it has antioxidative effect and can reduce oxidative stress-induced hepatic fibrosis.Citation15 It could also be attributed to its ability to reduce peroxidation of renal tubular cells, as it attenuated cisplatin-induced malondialdehyde (MDA) increases.Citation18 A recent study indicated that the administration of Ligustrazine in vivo also can attenuate renal tubulointerstitial fibrosis after unilateral ureteral obstruction.Citation19
In the present study, we reported the effect of Ligustrazine on LPS-induced RPMCs apoptosis and fibrosis, in order to develop a new pharmacological and therapeutic approach to the prevention of PMCs injury, which could attenuate the complications and improve the outcomes of PD patients.
Material and methods
Isolation and primary culture of RPMCs
Male Wistar rats weighing 150–180 g were purchased from animals’ facility of Experimental animal center of Henan Province, China. The rats were treated under the guidelines of Institutional Animal Care and Use Committee (IACUC). The study was approved by the ethics committee of Xinxiang Medical University. Animals received an injection of 20 mL 0.25% trypsin (in 0.02% EDTA solution) intraperitoneally, and 2 h later, the abdominal fluid of individual rats was collected, followed by centrifuging at 150 × g for 15 min. Sedimented cells were suspended in DMEM/F12 culture medium (Power, Gibco, USA) containing 10% fetal bovine serum and cultured in flasks under 5% CO2 at 37 °C. The RPMCs were identified by immunostaining with an anti-cytokeratin antibody and an anti-vimentin antibody (Shanghai Kemin Biotechnology Inc).
Methyl thiazolyl tetrazolium (MTT) analysis
RPMCs were grown in 96-well plates at a density of 2 × 105 cells/well until subconfluence was confirmed by microscopy. The RPMCs were treated on the following conditions: (1) The Control group, (2) RPMCs were treated with LPS (5 mg·L−1) for 6, 12, 24, and 48 h, and (3) RPMCs were treated with Ligustrazine (30 mg/L) only for 24 h. Ligustrazine (Purity > 98%) was purchased from Sigma and was dissolved in DMSO. (4) RPMCs were cotreated with different concentrations of Ligustrazine (15, 30 and 60 mg/L) and LPS (5 mg·L−1) for 24 h. Each of the treated or control groups contained three parallel wells; 0.5 mg/mL MTT (Sigma, USA) was added to each of the cultured wells mentioned above and then incubated continuously for 2 h. The supernatant was removed and the formation of formazan was observed by monitoring the signal at 540 nm using a microplate reader.
Determination of caspase-3 activity
A caspase-3 colorimetric assay kit (Beyotime, China) was used for the measurement of caspase activitiesCitation20. Briefly, RPMCs were grown in six-well culture plates until 80% confluence was reached. After treatment, cells were washed twice with cold PBS and lysed in ice-cold lysis buffer for 15 min. After centrifugation at 15,000 g at 4 °C for 10 min, the protein concentrations in the supernatants were quantified by the Bradford assay (Beyotime, China). The cell extracts (2 mg/mL, 50 μl) were then incubated with reaction buffer (40 μl) and Ac-DEVD-pNA (2 mM, 10 μl) at 37 °C for 100 min. The absorbances were monitored at a wavelength of 405 nm.
Detection of intracellular ROS level
Intracellular formation of reactive oxygen species (ROS) was detected using the ROS assay kit (Beyotime, China) according to the manufacturer’s instructions. RPMCs (5 × 106) were incubated with 10 μM DCFH-DA probes at 37 °C for 35 min and washed with PBS three times to remove residual probes. DCFH-DA was deacetylated intracellularly by nonspecific esterase, which was further oxidized by ROS to the fluorescent compound 2,7-dichlorofluorescein (DCF). DCF fluorescence was detected by flow cytometer (BD FACScan, USA) with excitation at 488 nm and emission at 525 nm. The results were analyzed by Cell Quest software (Becton Dickinson).
Measurement of intracellular superoxide dismutase (SOD) activity
The activity of SOD was measured by commercial reagent kits (Nanjing Jiancheng Bioengineering Institute, China).
Real-time RT-PCR analysis
RNA expression was evaluated by real-time RT-PCR in cultured RPMCs. Total RNA was extracted using TRIzol reagent (Invitrogen, USA) according to the manufacturer’s protocol. Real-time PCR amplification was performed using the SYBR Green Master Mix (Beijing BLKW Biotechnology co., Ltd, China). The following primer sets were used to amplify: Fibronectin Forward sequence: 5′-AA GCCAGAGTCAGATAACCG-3′ Reverse sequence: 5′-GTTGGCACTGACGAAG AGCC-3′; CollagenI Forward sequence: 5′-GCGAGGACATGAGGAGTAGC-3′ Reverse sequence: 5′-CCTGTGACCAGGGATGATGTCTT-3′; GAPDH Forward sequence 5′-TCCCTCAAGATTGTCAGCAA-3′ Reverse sequence: 5′-AGATCCA CAACGGATACATT-3′. The cycling program of real-time RT-PCR reaction involved preliminary denaturation at 94 °C for 4 min, followed by 35 cycles of denaturation at 94 °C for 30 s, annealing at 60 °C for 30 s and elongation at 72 °C for 30 s, followed by a final elongation step at 72 °C for 7 min. Relative amounts of mRNA were normalized by GAPDH mRNA expression. The comparative 2 − ΔΔCT method was used for quantification and statistical analysis.Citation21
Western blot
Protein was extracted from RPMCs by RIPA buffer containing 1-mM PMSF; 30 μg of proteins were subjected to polyacrylamide gel electrophoresis. The protein was transferred onto nitrocellulose membrane after separating in PAGE. Following washing and blocking, the membrane was incubated overnight at 4 °C with primary antibody against p-p38 (Santa Cruze, 1: 500 dilution), p38 (Santa Cruze, 1: 500), and MMP-9 (Santa Cruze, 1: 400 dilution). The membrane was incubated with secondary antibody at 37 °C for 1 h after washing. Bands were visualized by ECL western blotting kit (Beyotime Institute of Biotechnology, China). Target proteins levels were normalized by β-action.
Statistical analysis
Statistical analysis was carried out with SPSS19.0. Comparisons among groups were performed by one-way ANOVA. Results are expressed as mean ± SD. p < 0.05 was considered statistically significant.
Results
Effect of LPS and Ligustrazine on cell viability
To investigate the growth inhibition effects of LPS on RPMC, the cells were treated with LPS (5 mg·L−1) for 6, 12, 24, or 48 h in the presence or absence of Ligustrazine (15 mg·L−1, 30 mg·L−1, and 60 mg·L−1). As shown in , 5 mg·L−1 of LPS had significant growth inhibition effects on the RPMC. However, cell viability was increased remarkably after the cells being cotreated with Ligustrazine (30 mg/L) for 24 h, and there was no difference between Ligustrazine and the control group (). And Ligustrazine increased the cell viability in a dose-dependent manner ().
Figure 1. Effect of Ligustrazine on RPMC’s viability stimulated by LPS. (A) Cells were treated with LPS (5 mg·L−1) for 6, 12, 24, and 48 h; the cell viability was determined by MTT assay. (B) Cells were treated with Ligustrazine (30 mg/L) for 24 h. (C) Cells were cotreated with Ligustrazine (15, 30, and 60 mg/L) and LPS (5 mg/L) for 24 h. All results are shown as mean ± SD and are representative of three independent experiments. *p < 0.05, **p < 0.01 versus LPS; #p <005, ##p < 0.01 versus Control. LG: Ligustrazine.
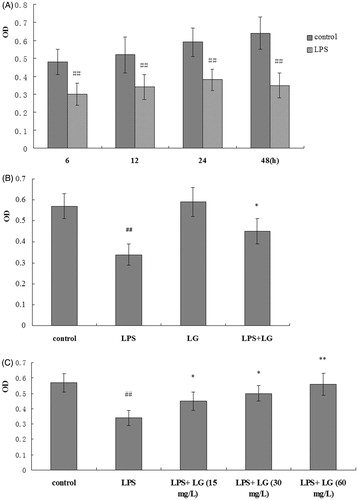
Effect of Ligustrazine on caspase-3 activity in RPMCs exposed to LPS
The results from shown that, in response to LPS for 24 h, caspase-3 activity increased significantly compared to control cells (no addition of LPS and Ligustrazine). However, Ligustrazine significantly inhibited LPS-induced caspase-3 activity (.
Figure 2. Effect of Ligustrazine on caspase-3 activity in RPMC’s exposed to LPS. The RPMCs were treated with 5 mg/L of LPS in the absence or the presence of Ligustrazine (15, 30, and 60 mg/L), and caspase-3 activity was measured as described in “Methods”. *p < 0.05, **p < 0.01 versus LPS; ##p < 0.01 versus Control. LG: Ligustrazine.

Effect of Ligustrazine on fibrosis-associated mRNA expression in RPMCs exposed to LPS
shows that RPMC exposure to 5 mg·L−1 LPS for 24 h caused a marked increase in FN and Col-I mRNA. Compared with the LPS group, the decrease in FN and COL-I mRNA expression were observed in the Ligustrazine group (. Ligustrazine significantly attenuated the elevated FN and COL-I mRNA level induced by LPS.
Figure 3. Effect of Ligustrazine on FN and COL-I mRNA expression in RPMCs exposed to LPS. Cells were treated for 24 h with 5 mg/L of LPS in the absence or the presence of Ligustrazine (15, 30, and 60 mg/L). Results are shown as mean ± SD and are representative of three independent experiment. *p < 0.05, **p < 0.01 versus LPS; #p < 0.05, ##p < 0.01 versus Control. LG: Ligustrazine.
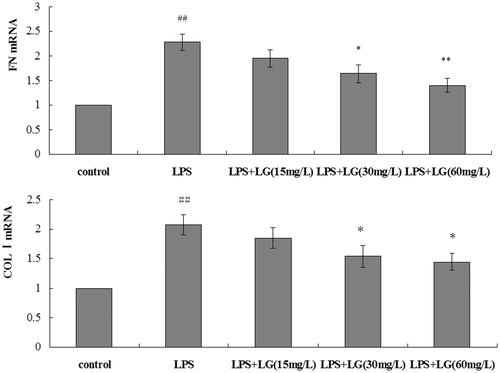
Effect of Ligustrazine on ROS generation and SOD activity induced by LPS in RPMCs
As shown in , treatment of RPMCs with 5 mg·L−1 of LPS for 24 h resulted in marked increases of ROS production but decrease of SOD activity compared with control group (p < 0.01). But Ligustrazine attenuated LPS-induced production of ROS and restored SOD activity in RPMCs.
Figure 4. Effect of Ligustrazine on ROS generation and SOD activity induced by LPS in RPMCs. Cells were treated for 24 h with 5 mg/L of LPS in the absence or the presence of Ligustrazine (15, 30, and 60 mg/L). ROS production was measured using DCF as described in Materials and Methods. Result represents mean ± SD of three independent experiments. *p < 0.05, **p < 0.01 versus LPS; ##p < 0.01 versus Control. LG: Ligustrazine.

Effect of Ligustrazine on phosphorylation of p38 MAPK induced by LPS in RPMCs
Exposure to 5 mg·L−1 of LPS for 24 h caused a marked increase in the phosphop38 MAPK/β-actin protein ratio compared to control cells (. To evaluate the influence of Ligustrazine on LPS-induced p38 phosphorylation, RPMCs were cotreated with Ligustrazine and 5 mg·L−1 of LPS for 24 h. As shown in , Ligustrazine reversed LPS-induced p38 phosphorylation.
Figure 5. Effect of Ligustrazine on phosphorylation of p38 MAPK induced by LPS in RPMCs. Cells were treated for 24 h with 5 mg/L of LPS in the absence or the presence of Ligustrazine (15, 30, and 60 mg/L). Both phosphor-38 MAPK and MAPK protein levels were determined by Western blot with β-actin used as an internal control. Data represent mean ± SD of three independent experiments. *p < 0.05, **p < 0.01 versus LPS; #p < 0.05, ##p < 0.01 versus Control. LG: Ligustrazine.
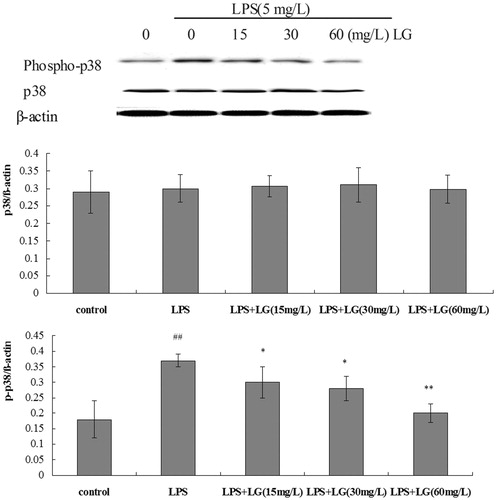
Effect of Ligustrazine on MMP-9 expression in RPMCs
RPMCs were exposed to 5 mg·L−1 of LPS, or a combined treatment of 5 mg·L−1 of LPS and Ligustrazine for 24 h, and the expression levels of MMP-9 were detected. From the results of , the expression levels of MMP-9 were significantly increased when cultured with LPS, and this effect was suppressed by Ligustrazine (p < 0.05).
Figure 6. Effect of Ligustrazine on MMP-9 expression induced by LPS in RPMCs. Cells were treated for 24 h with 5 mg/L of LPS in the absence or the presence of Ligustrazine (15, 30, and 60 mg/L). The histograms represent a densitometric analysis of MMP-9/β-actin ratio. Data represent mean ± SD of three independent experiments. *p < 0.05, **p < 0.01 versus LPS; #p < 0.05, ##p < 0.01 versus Control. LG: Ligustrazine.
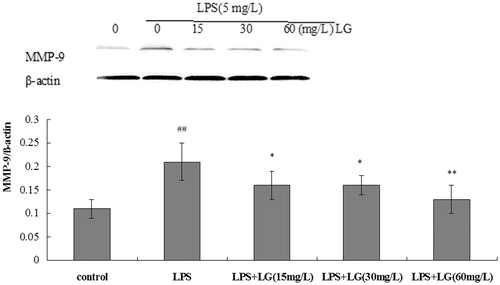
Discussion
CAPD is a form of renal replacement therapy for end-stage renal disease. During long-term PD, loss of peritoneal membrane integrity and peritoneal ultrafiltration capacity are major complications. Bacterial peritonitis caused mainly by catheter-related infections in long-term PD is one of the major causes of peritoneal dysfunction and morbidity in CAPD patients. LPS, the prototypical endotoxin, is the major component of the outer membrane of Gram-negative bacteria and through TLR4 can trigger proapoptotic pathways.Citation22 LPS can induce peripheral blood mononuclear cell-derived dendritic cell apoptosis.Citation23 The apoptosis in PMCs will lead to deterioration of the peritoneum. This study investigated the protective effect of Ligustrazine against LPS-induced toxicity using MTT assay. The results showed that treating cells with LPS resulted in cell viability loss. However, treatment with different concentrations (15–60 mg/L) of Ligustrazine greatly decreased the loss of cell viability (. These results indicate that Ligustrazine significantly protected RPMCs from LPS-induced cytotoxicity.
In the process of apoptosis, cysteine aspartyl-specific proteases (caspases) are activated, and they then cleave cellular substrates, resulting in the characteristic morphological and biochemical alterations of apoptosis.Citation24,Citation25 In this point, caspase-3 was considered to be an pro-apoptotic factor. In the present study, it was demonstrated that LPS can increase caspase-3, which shows that LPS certainly triggers cell apoptosis through the mitochondrial pathway. Ligustrazine could inhibit apoptosis via mitochondria-dependent apoptotic pathways by downregulating caspase-3 expression.
Long-term PD may result in oxidative stress, which damages PMCs and may cause peritoneal fibrosis and ultrafiltration loss.Citation26 A previous study confirmed that EMT of PMCs is generally regarded to be the result of oxidative stress; while, antioxidants effectively reverse the EMT in PMCs.Citation27 In fact, oxidative stress has also been proposed to play a major role in the development of peritoneal deteriorationCitation28 and apoptosis.Citation29 Among the diverse stimuli shown to induce apoptotic cell death, ROS seem to play a central role. In fact, when the cytoprotective and antioxidant capacity is insufficient, ROS will induce apoptosis.Citation30 The generation of excessive levels of ROS results in oxidative stress.Citation31 Overproduction of ROS by mitochondria can trigger cell death by modulating a series of intracellular signaling pathways. Indeed, antioxidants effectively reverse the apoptosis in human PMCs (HPMCs).Citation32 Our study found that Ligustrazine treatment could attenuate LPS-induced ROS generation. SOD, as an important antioxidant enzyme, provides a major defense against ROS generation. In the present study, we observed significant increase in the levels of SOD in RPMCs treated with Ligustrazine compared to LPS group. The role of Ligustrazine modulating oxidative stress has been recognized and Ligustrazine attenuates LPS-induced apoptosis and fibrosis which is related to the inhibition of oxidative stress.
The matrix metalloproteinase (MMP) family of endopeptidases represents the primary mediators of vascular ECM degradation.Citation33 Recent reports have shown that increasingly diverse stimuli, including ROS, can upregulate most MMPs in the vasculature.Citation34,Citation35 ROS-induced MMP-9 activity was proposed to be the key factor for ECM formation and collagens deposition.Citation36,Citation37 The stimulation of EMT through the ROS/MMP-9 signaling pathway and inhibition of ROS/MMP-9 signaling could prevent peritoneal fibrosis.Citation14,Citation38 In our study, it was found that LPS treatment resulted in a substantial increase of ROS production and increased MMP-9 expression, while Ligustrazine effectively reverses this effect and inhibits the incidence of fibrosis.
Consistent with ROS participation, LPS activates p38 kinase MAPK pathway, which are redox sensitive and are involved in cellular responses to stress and inflammation.Citation39 The activation of p38 MAPKs signaling pathways plays an important role in LPS-mediated apoptosis of RPMCs.Citation14 Phosphorylation of p38 MAPK is a generally accepted index of toxicity for RPMCs.Citation40 The p38 MAPK pathway is also an important intracellular signal transduction pathway for fibrosis. The activation of p38 MAPK makes renal epithelial cells express smooth muscle actin (SMA)Citation41 and inhibition of the p38 MAPK pathway ameliorates renal fibrosis in an NPHP2 mouse model.Citation42 In this study, we investigated whether modulation of P38MAPK was involved in the protective effect by Ligustrazine. As shown in , phosphorylated p38 proteins were barely detected in RPMCs in the control group. However, when activated by LPS, the phosphorylated proteins were highly increased. And further treatment with Ligustrazine inhibited the phosphorylation of p38 MAPK (. Our findings suggested that Ligustrazine attenuates LPS-induced apoptosis and fibrosis at least partially via inhibition of P38/MAPK phosphorylation.
In summary, Ligustrazine could inhibit LPS-induced apoptosis and fibrosis of RPMCs that maybe through several mechanisms, including abrogation of LPS-induced oxidative stress and ROS/MMP-9, p38/MAPKS activation in RPMCs. Furthermore, Ligustrazine reverses the activation of caspase-3, blocking the mitochondrial apoptosis pathway. These data provide a new perspective of the antiapoptosis and fibrosis effect of Ligustrazine. Peritoneal fibrosis and acute peritonitis are two main complications associated with PD, and these are associated with loss of mesothelial cells.Citation43 The protect effect of Ligustrazine can help to maintain peritoneal membrane function longer in patients undergoing CAPD, thus improve PD outcomes. However, further work is needed to explore the effects of Ligustrazine on peritoneal function in a proper animal model.
Conclusions
Ligustrazine could restrain LPS-induced apoptosis and fibrosis, which may be through inhibition of oxidative stress and p38/MAPKS, ROS/MMP-9 activation in PMCs.
Funding information
This work was supported by highly educated people funded projects of Xinxiang Medical University.
Disclosure statement
The authors reported no proprietary or commercial interest in any product mentioned or concept discussed in the article. The authors have no conflicts of interest to declare.
References
- Krediet RT, Lindholm B, Rippe B. Pathophysiology of peritoneal membrane failure. Perit Dial Int. 2000;20:S22–S42.
- Davies SJ, Phillips L, Griffiths AM, Russell LH, Naish PF, Russell GI. What really happens to people on long-term peritoneal dialysis? Kidney Int. 1998;54:2207–2217.
- Krediet RT. The peritoneal membrane in chronic peritoneal dialysis. Kidney Int. 1999;55:341–356.
- Yanez-Mo M, Lara-Pezzi E, Selgas R, et al. Peritoneal dialysis and epithelial-to-mesenchymal transition of mesothelial cells. N Engl J Med. 2003;348:403–413.
- Marchi E, Liu W, Broaddus VC. Mesothelial cell apoptosis is confirmed in vivo by morphological change in cytokeratin distribution. Am J Physiol Lung Cell Mol Physiol. 2000;278:L528–L535.
- Ortiz A, Catalan MP. Will modulation of cell death increase PD technique survival? Perit Dial Int. 2004;24:105–114.
- Williams JD, Craig KJ, Topley N, et al. Morphologic changes in the peritoneal membrane of patients with renal disease. J Am Soc Nephrol. 2002;13:470–479.
- Margetts PJ, Kolb M, Yu L, Hoff CM, Gauldie J. A chronic inflammatory infusion model of peritoneal dialysis in rats. Perit Dial Int. 2001;21:S368–S372.
- Borges FR, Silva MD, Cordova MM, Schambach TR, Pizzolatti MG, Santos AR. Anti-inflammatory action of hydroalcoholic extract, dichloromethane fraction and steroid α-spinasterol from Polygala sabulosa in LPS-induced peritonitis in mice. J Ethnopharmacol. 2014;151:144–150.
- Li J, He J, Yu C. Chitosan oligosaccharide inhibits LPS-induced apoptosis of vascular endothelial cells through the BKCa channel and the p38 signaling pathway. Int J Mol Med. 2012;30:157–164.
- Guo C, Yuan L, Wang JG, et al. Lipopolysaccharide (LPS) induces the apoptosis and inhibits osteoblast differentiation through JNK pathway in MC3T3-E1 cells. Inflammation. 2014;37:621–631.
- Khodagholi F, Tusi SK. Stabilization of Nrf2 by tBHQ prevents LPS-induced apoptosis in differentiated PC12 cells. Mol Cell Biochem. 2011;354:97–112.
- Zhao L, Yang R, Cheng L, Wang M, Jiang Y, Wang S. LPS-induced epithelial-mesenchymal transition of intrahepatic biliary epithelial cells. J Surg Res. 2011;171:819–825.
- Liu J, Zeng L, Zhao Y, Zhu B, Ren W, Wu C. Selenium suppresses lipopolysaccharide-induced fibrosis in peritoneal mesothelial cells through inhibition of epithelial-to-mesenchymal transition. Biol Trace Elem Res. 2014;161:202–209.
- Liu XH, Li J, Li QX, Ai YX, Zhang L. Protective effects of ligustrazine on cisplatin-induced oxidative stress, apoptosis and nephrotoxicity in rats. Environ Toxicol Pharmacol. 2008;26:49–55.
- Zheng H, Wang S, Zhou P, Liu W, Ni F. Effects of ligustrazine on DNA damage and apoptosis induced by irradiation. Environ Toxicol Pharmacol. 2013;36:1197–1206.
- Xiao S, Li KH, Lu HB. [Effect of ligustrazine on the expression of Bcl-2 protein and apoptosis in rabbit articular chondrocytes in monolayer culture]. Hunan Yi Ke Da Xue Xue Bao. 2003;28:224–226.
- Zhang F, Ni C, Kong D, et al. Ligustrazine attenuates oxidative stress-induced activation of hepatic stellate cells by interrupting platelet-derived growth factor-beta receptor-mediated ERK and p38 pathways. Toxicol Appl Pharmacol. 2012;265:51–60.
- Yuan XP, Liu LS, Fu Q, Wang CX. Effects of ligustrazine on ureteral obstruction-induced renal tubulointerstitial fibrosis. Phytother Res. 2012;26:697–703.
- Posadas I, Vellecco V, Santos P, Prieto-Lloret J, Cena V. Acetaminophen potentiates staurosporine-induced death in a human neuroblastoma cell line. Br J Pharmacol. 2007;150:577–585.
- Winer J, Jung CK, Shackel I, Williams PM. Development and validation of real-time quantitative reverse transcriptase-polymerase chain reaction for monitoring gene expression in cardiac myocytes in vitro. Anal Biochem. 1999;270:41–49.
- Lombardo E, Alvarez-Barrientos A, Maroto B, Bosca L, Knaus UG. TLR4-mediated survival of macrophages is MyD88 dependent and requires TNF-alpha autocrine signalling. J Immunol. 2007;178:3731–3739.
- Yen JH, Ganea D. Interferon beta induces mature dendritic cell apoptosis through caspase-11/caspase-3 activation. Blood. 2009;114:1344–1354.
- Ashkenazi A, Dixit VM. Death receptors: signaling and modulation. Science. 1998;281:1305–1308.
- Green DR, Reed JC. Mitochondria and apoptosis. Science. 1998;281:1309–1312.
- Lee HB, Yu MR, Song JS, Ha H. Reactive oxygen species amplify protein kinase C signaling in high glucose-induced fibronectin expression by human peritoneal mesothelial cells. Kidney Int. 2004;65:1170–1179.
- Lee HB, Ha H. Mechanisms of epithelial-mesenchymal transition of peritoneal mesothelial cells during peritoneal dialysis. J Korean Med Sci. 2007;22:943–945.
- Lu Y, Shen H, Shi X, Feng S, Wang Z, Shi Y. Hydrogen sulfide ameliorates high-glucose toxicity in rat peritoneal mesothelial cells by attenuating oxidative stress. Nephron Exp Nephrol. 2014;126:157–165.
- Wagener FA, Dekker D, Berden JH, Scharstuhl A, van der Vlag J. The role of reactive oxygen species in apoptosis of the diabetic kidney. Apoptosis. 2009;14:1451–1458.
- Brownlee M. The pathobiology of diabetic complications: a unifying mechanism. Diabetes. 2005;54:1615–1625.
- Zwolinska D, Grzeszczak W, Szczepanska M, Kilis-Pstrusinska K, Szprynger K. Lipid peroxidation and antioxidant enzymes in children on maintenance dialysis. Pediatr Nephrol. 2006;21:705–710.
- Gotloib L. Mechanisms of cell death during peritoneal dialysis. A role for osmotic and oxidative stress. Contrib Nephrol. 2009;163:35–44.
- Galis ZS, Khatri JJ. Matrix metalloproteinases in vascular remodeling and atherogenesis: The good, the bad, and the ugly. Circ Res. 2002;90:251–262.
- Rajagopalan S, Meng XP, Ramasamy S, Harrison DG, Galis ZS. Reactive oxygen species produced by macrophage-derived foam cells regulate the activity of vascular matrix metalloproteinases in vitro. Implications for atherosclerotic plaque stability. J Clin Invest. 1996;98:2572–2579.
- Zalba G, Fortuno A, Orbe J, et al. Phagocytic NADPH oxidase-dependent superoxide production stimulates matrix metalloproteinase-9: implications for human atherosclerosis. Arterioscler Thromb Vasc Biol. 2007;27:587–593.
- Ducharme A, Frantz S, Aikawa M, et al. Targeted deletion of matrix metalloproteinase-9 attenuates left ventricular enlargement and collagen accumulation after experimental myocardial infarction. J Clin Invest. 2000;106:55–62.
- Vassiliadis E, Veidal SS, Barascuk N, et al. Measurement of matrix metalloproteinase 9-mediated collagen type III degradation fragment as a marker of skin fibrosis. BMC Dermatol. 2011;11:6. doi: 10.1186/1471-5945-11-6.
- Tobar N, Villar V, Santibanez JF. ROS-NFkappaB mediates TGF-beta1-induced expression of urokinase-type plasminogen activator, matrix metalloproteinase-9 and cell invasion. Mol Cell Biochem. 2010;340:195–202.
- Kyriakis JM, Avruch J. Mammalian mitogen-activated protein kinase signal transduction pathways activated by stress and inflammation. Physiol Rev. 2001;81:807–869.
- Nakagami H, Morishita R, Yamamoto K, et al. Phosphorylation of p38 mitogen-activated protein kinase downstream of bax-caspase-3 pathway leads to cell death induced by high D-glucose in human endothelial cells. Diabetes. 2001;50:1472–1481.
- Li Y, Yang J, Dai C, Wu C, Liu Y. Role for integrin-linked kinase in mediating tubular epithelial to mesenchymal transition and renal interstitial fibrogenesis. J Clin Invest. 2003;112:503–516.
- Sugiyama N, Kohno M, Yokoyama T. Inhibition of the p38 MAPK pathway ameliorates renal fibrosis in an NPHP2 mouse model. Nephrol Dial Transplant. 2012;27:1351–1358.
- Mortier S, De Vriese AS, Lameire N. Recent concepts in the molecular biology of the peritoneal membrane – implications for more biocompatible dialysis solutions. Blood Purif. 2003;21:14–23.