Abstract
A prototype electronic cigaret device and three formulations were evaluated in a 90-day rat inhalation study followed by a 42-day recovery period. Animals were randomly assigned to groups for exposure to low-, mid- and high-dose levels of aerosols composed of vehicle (glycerin and propylene glycol mixture); vehicle and 2.0% nicotine; or vehicle, 2.0% nicotine and flavor mixture. Daily targeted aerosol total particulate matter (TPM) doses of 3.2, 9.6 and 32.0 mg/kg/day were achieved by exposure to 1 mg/L aerosol for 16, 48 and 160 min, respectively. Pre-study evaluations included indirect ophthalmoscopy, virology and bacteriological screening. Body weights, clinical observations and food consumption were monitored weekly. Plasma nicotine and cotinine and carboxyhemoglobin levels were measured at days 28 and 90. After days 28, 56 and 90, lung function measurements were obtained. Biological endpoints after 90-day exposure and 42-day recovery period included clinical pathology, urinalysis, bronchoalveolar fluid (BALF) analysis, necropsy and histopathology. Treatment-related effects following 90 days of exposure included changes in body weight, food consumption and respiratory rate. Dose-related decreases in thymus and spleen weights, and increased BALF lactate dehydrogenase, total protein, alveolar macrophages, neutrophils and lung weights were observed. Histopathology evaluations revealed sporadic increases in nasal section 1–4 epithelial hyperplasia and vacuolization. Following the recovery period, effects in the nose and BALF were persistent while other effects were resolved. The no observed effect level based upon body weight decreases is considered to be the mid-dose level for each formulation, equivalent to a daily TPM exposure dose of approximately 9.6 mg/kg/day.
Introduction
Electronic cigarets (also known as “e-cigarets”) are battery-powered products intended to provide adult vapers (e-cigaret users) with a pleasurable sensory experience while delivering nicotine and/or flavors in fine particulate aerosols via inhalation. A large variety of devices and flavor formulations are currently in the marketplace. The process of inhaling vapors (or, more accurately, aerosols) produced by e-cigarets is known as “vaping”. While the population of vapers is relatively small compared with conventional cigaret users, this population is projected to grow rapidly and surpass cigaret sales by 2047 (Robehmed, Citation2013).
Scientists have expressed divergent views about the potential individual and population risks associated with e-cigaret use (Jensen et al., Citation2015; Kosmider et al., Citation2014; Lerner et al., Citation2015). Some experts suggest that e-cigarets may be a revolutionary game changer capable of improving public health by decreasing exposure to conventional cigaret smoke (Etter & Bullen, Citation2014), decreasing the potential for addiction (Etter & Bullen, Citation2014) and facilitating cessation in some motivated smokers (Bullen et al., Citation2013; Etter & Bullen, Citation2014). Further, e-cigarets may have potential as “modified risk tobacco products” (MRTPs). A draft guidance document distributed by Food and Drug Administration (FDA) regarding MRTPs notes that “the modified risk tobacco product provisions of the FD&C [Federal Food, Drug and Cosmetic] Act may be valuable tools in the effort to promote public health by reducing the morbidity and mortality associated with tobacco use, particularly if companies take advantage of these provisions by making bold, innovative product changes that substantially reduce or even eliminate altogether, either the toxicity or addictiveness of tobacco products, or both” (US DHHS, Citation2012).
Most e-cigaret formulations contain vehicles (propylene glycol and/or glycerol), generally recognized as safe (GRAS) flavor ingredients and nicotine. GRAS status is useful to assure the ingredients’ safety for their intended use in food, but most ingredients have not been evaluated for inhalation exposure (CDC, Citation2015; Farsalinos & Pelosa, Citation2014). Inhalation testing is particularly useful to assess pulmonary function and pathological and/or pathophysiological effects in the respiratory tract, and would also be necessary to assess potential vehicle and flavor toxicity (CDC, Citation2015). Waldum et al. (Citation1996) found no harmful effects of nicotine per se via inhalation in a two year inhalation study in rats. Studies on the biological responses from e-cigaret vehicle components such as inhaled propylene glycol (Suber et al., Citation1989; Werley et al., Citation2011) and glycerol (Renne & Gideon, Citation2006; Renne et al., Citation1992) are available. For most flavors and formulations, however, there are no publicly available scientific reports or publications about the toxicological evaluation of e-cigaret aerosols using a standardized 90-day inhalation safety test. Further, the combination of vehicle, flavor ingredients, nicotine and device components may potentially produce unexpected chemical changes, fugitive particles or fibers and metals in the e-cigaret aerosol.
Before our inhalation testing began, we conducted an ingredient screening and toxicological evaluation of the formulation ingredients under normal conditions of use in the e-cigaret device. Similar to the approach suggested by Costigan & Meredith (Citation2015) for ingredients and flavor formulations, we screened all formulation ingredients for food grade and GRAS status, purity, potential chemical interactions in formulation and quantitative structure activity relationship alerts. We excluded any known carcinogenic, genotoxic and reproductive toxicants, as well as any respiratory sensitizers. Our group conducted a 90-day inhalation safety study with a 42-day post-exposure recovery period in rats as part of a comprehensive toxicological evaluation program to evaluate a prototype e-cigaret and three flavor formulations. This study was compliant with good laboratory practice, and the evaluations were conducted according to guidelines established by FDA and the Organization for Economic Co-operation and Development (OECD, Citation2009).
Materials and methods
Test materials
A MarkTen® prototype e-cigaret (CVR 1.3; hereafter called prototype e-cigaret), a vehicle control (a mixture of glycerin and propylene glycol), two e-cigaret flavor formulations and a commercial reference e-cigaret product were evaluated in this study.
Prototype e-cigaret
A cross-sectional diagram of the prototype e-cigaret is shown in . A comprehensive evaluation of the prototype e-cigaret was conducted prior to this study. An individual assessment of each component was performed using an evaluation approach consisting, in part, of extractables and leachables studies (Norwood et al., Citation2008), aerosol mass output, aerosol characterization for ingredient and nicotine degradants and stability of the device containing formulation over a minimum 60-day duration.
Test aerosol formulations
Two test aerosol formulations were evaluated. Both formulations were compared with a vehicle control, which contained 23% glycerol and 77% propylene glycol. Formulation 1 contained 22.5% glycerol, 75.5% propylene glycol and a small amount (2.0% w/w) of USP grade nicotine. Formulation 2 contained 18.1% glycerol, 62.3% propylene glycol, 2.0% w/w USP grade nicotine and 17.6% proprietary flavor.
Reference product
A commercial e-cigaret with 2% nicotine and similar product performance puffing characteristics to the prototype e-cigaret was selected as the Reference product for comparison in this study. The Intellicig® (Intellicig-USA.com, Corona, CA) was selected as the Reference product.
Inhalation study
The 90-day nose-only rat inhalation study was conducted by WIL Research (Ashland, OH). The study design is outlined in . In general, the study design included pre-study and weekly evaluations as well as biological examinations. Subgroups of rats were euthanized at 28, 90 and 132 days for necropsy (interim, primary and recovery necropsy groups, respectively) to measure treatment-related effects and potential biological changes.
Table 1. Study design, treatment groups and exposure group assignments for male and female Sprague–Dawley rats exposed to prototype e-cigaret aerosol formulations.
Animal subjects
Crl:CD(SD) rats (Charles River Laboratories, Raleigh, NC) (210/sex; nulliparous and non-pregnant females) approximately 5–7 weeks old were individually housed in suspended wire mesh cages and provided food (LLC Certified Rodent LabDiet®, LabDiet, St. Louis, MO) and water ad libitum in an environmentally controlled room (71 ± 5 °F; relative humidity 50 ± 20%) with a 12-h light/dark cycle. Daily animal enrichment was provided to each animal through their food container. No food or water was provided during the daily inhalation exposure period. Animals were acclimated to nose-only restraint with increasing periods of restraint over a 4-day period (30 min on the first day of acclimation, 1 h on the second day, 2 h on the third day and 3 h on the fourth day). Animals were randomized into 1 of 10 exposure groups () based upon body mass stratification with each animals’ body mass within ±20% of the mean for each sex. All facilities were fully accredited by the Association for Assessment and Accreditation of Laboratory Animal Care International.
The Sprague–Dawley rat is a commonly used rat strain and extensive historical baseline data sets are available for comparison in ongoing studies. In this study, the study director and clinical and veterinary pathologists referenced the laboratory internal database for this strain as is customary, as well as external databases as needed. Here, we reference two resources for the interested reader who wishes to compare our data set with historical data from other studies conducted over time. Petterino & Argentino-Storino (Citation2006) and Matsuzawa & Inoue (Citation1998) are excellent resources in this regard.
Exposures and daily dose
A single nose-only exposure system (CH Technologies, Westwood, NJ) was used to provide three different nominal dose levels (hereafter called dose) of aerosol exposure to each treatment group (vehicle control, Formulation 1 and Formulation 2). The dose levels were generated by varying the length of exposure. The aerosol for each treatment group was generated from a custom built, rotary smoking machine (RM-20, Borgwaldt, Richmond VA) modified to use prototype e-cigarets. A continuous aerosol was supplied by a pair of prototype e-cigarets that were alternatively puffed for a total of 12 puffs (6 puffs each) with each puff being 5 s in duration. A second pair of prototype e-cigarets was subsequently utilized, with the original pair being replaced with a new pair of prototype e-cigarets. The prototype e-cigaret aerosol was diluted with 38–42 L/min of HEPA-filtered air to provide a target exposure concentration of 1 mg/L. The Reference product (Intellicig) aerosol generation was accomplished exactly as was done for the prototype e-cigaret.
The targeted daily dose levels (low, mid and high) were achieved for each treatment group (vehicle control, Formulation 1 and Formulation 2) by exposing the rats to 1 mg/L of the test aerosol for the appropriate duration of 16, 48 or 160 min/day for the length of the study to attain nominal daily doses of 3.2, 9.6 and 32.0 mg/kg/day.
All exposure monitoring was conducted at an animal exposure port on the nose-only exposure system. Exposure concentrations were monitored in real time using an infrared forward scattering monitor (Microdust Pro™, Casella CEL Ltd., Bedford, UK). The average exposure concentration was measured gravimetrically once per week using a pre-weighed Cambridge filter pad. Weekly measurement of nicotine in the exposure atmosphere was performed using a Cambridge filter pad followed by an XAD-4 sorbent tube (SKC Inc., Eighty Four, PA). Particle size measurements were made gravimetrically using a stainless steel seven-stage cascade impactor (Intox Products, Moriarty, NM) on a weekly basis.
Markers of exposure
Blood was obtained from 5 rats/sex/exposure group that were assigned to the Recovery necropsy via the retro-obital sinus under isoflurane anesthesia on exposure days 28 and 90 and following recovery. Blood carboxyhemoglobin (COHb) and blood nicotine and cotinine levels were measured using established methods (Klimisch et al., Citation1974; VanVunakis et al., Citation1987).
Biological endpoints
Ophthalmic evaluations were made on all rats prior to the study by a board-certified veterinary ophthalmologist. Body weight and food consumption were measured once per week. Daily observations were performed before and within 90 min after aerosol exposure for each rat. Detailed physical examinations of each rat were also performed weekly. Single chamber-restraint plethysmography (Buxco Electronics, Inc., Wilmington, NC) was used to measure lung function (tidal volume and respiratory rate) prior to exposure on days 28, 56 and 90 in each of the 5 rats/sex/exposure group that were assigned to the recovery necropsy group.
Rats were fasted prior to necropsy. On the day following the last exposure, the rats were anesthetized with isoflurane inhalation, and blood was collected for hematology and blood chemistry analysis prior to exsanguination. The left lung of each animal was lavaged three times using Hanks’ balanced salt solution without calcium, magnesium or phenol red to obtain bronchoalveolar lavage fluid (BALF) at necropsy. Lavage volume was based upon body mass (mL lavage fluid = 14 × body mass for first lavage and mL lavage fluid = 11.55 × body mass for second and third lavages). The second and third BALF samples were combined and saved in case analytical problems occurred with the first BALF sample. Total and differential cell counts (basophils, eosinophils, lymphocytes, neutrophils and macrophages), as well as, lactate dehydrogenase (LDH), total protein and alkaline phosphatase (ALP) were measured using BALF. Organ weights were determined at necropsy for the following organs: lungs (prior to inflation with fixative), brain, heart, kidneys, liver, reproductive organs, spleen, thyroid/parathyroids, adrenal glands and thymus. All tissue and organs collected were preserved in 10% neutral-buffered formalin, except for the reproductive organs (males: epididymides and testes; females: ovaries with oviducts), which were preserved in Davidson’s solution. For histopathology, after the brain was removed and the head was decalcified, five cross-sections of the nasal cavities were prepared for microscopic examination in accordance with the methods described by Mery et al. (Citation1994) and Morgan (Citation1991).
shows a summary of the biological parameters evaluated at 28, 90, 132 days to vehicle, Formulation 1 and Formulation 2.
Table 2. Summary of biological parameters under evaluation for 28, 90 and 132 days to vehicle, Formulation 1 and Formulation 2.
Statistical analysis
All statistical comparisons were conducted at the 0.05 significance level, by sex and treatment interval, with an analysis of variance (ANOVA) including treatment group as the fixed effect. All 10 treatment groups were included in the statistical model. From this overall ANOVA, subset analyzes were conducted to make valid comparisons as follows:
Within each subset of comparisons, the false discovery rate approach (i.e. the modified Benjamin Hochberg method) was used to adjust p values for multiple comparisons (Ellis et al., Citation2000). Respiratory endpoints were analyzed with a two-way ANOVA including treatment group, sex and treatment * sex interaction. All the 10 treatment groups were included in the statistical model. If the treatment * sex interaction was significant, the subset group comparisons were conducted separately for each sex and overall across the pooled sexes. If the interaction was not significant, the subset group comparisons were conducted overall across the pooled sexes only.
Plasma nicotine and cotinine data were log transformed before analysis using a two-way analysis of variance because the standard deviations at higher exposures were greater than at lower exposures. All quantitative variables were evaluated for statistical significance compared with the vehicle controls with statistical significance level of p ≤ 0.05 without correction for multiple comparisons. Statistical analysis was performed by BioSTAT Consultants, Inc. (Portage, MI), using SAS version 9.2 software (SAS Institute, Inc., 2002–2008, Cary, NC).
Results
Exposure concentration measurements
Inhalation exposure concentrations for each exposure group are shown in . Throughout the duration of the study, the cumulative mean exposure to the targeted aerosol mass based upon gravimetric determinations was within 3% of the target level in each group.
Table 3. Cumulative aerosol mass target and actual aerosol concentrations for exposure groups 1–10 during 90-day inhalation exposure to aerosol formulations.
The mean mass median aerodynamic diameter (MMAD) and geometric standard deviation (GSD) are shown in . The MMAD ranged from 1.1 to 1.3 μm in each exposure group for the duration of the study, indicating that the aerosol particles were fully respirable in the rat.
Table 4. Cumulative mass median aerodynamic diameter (MMAD) and geometric standard deviation (GSD) for exposure groups 1–10 during 90-day inhalation exposure to aerosol formulations.
Body weight
Absolute body weight and gains for males and females in each treatment group are shown in . Treatment-related body weight losses and lower gains over the 90-day exposure interval were observed in animals from the Formulation 1 and Formulation 2 groups, compared with the vehicle control group. In general, rats exposed to high-dose levels had lower body weights and lower gains for each formulation tested, and males tended to have proportionally greater declines than females. Body weights in males and females from the Reference product group exposed to aerosol from the reference Intellicig® were similar to high-dose group exposed rats.
Clinical observations
There were no treatment-related clinical observations. All clinical findings in the Formulation 1 and Formulation 2 treated groups were noted with similar incidence in the vehicle control group, or were common findings for laboratory rats of the same age and strain (data not shown).
Nicotine and cotinine markers of exposure
Plasma nicotine and cotinine were measured in all treatment groups at study days 28 and 90. That data for all treatment groups are shown in . In general, plasma cotinine levels are proportional to the daily delivered dose, and the plasma doses are elevated in proportion to the dose progression. Therefore, for example, the high dose plasma cotinine levels are generally 10-fold higher than the low-dose levels. Nicotine levels, on the other hand, appear to be depressed at the high-dose exposure levels, likely due to the short biological half-life of nicotine and its metabolism to cotinine before sampling and analysis were completed. Since cotinine has a significantly longer half-life, cotinine in plasma is a better estimator in rat plasma in this study. There were no exposure- or treatment-related differences in plasma nicotine and cotinine for males and females when comparing study days 28 and 90.
Table 5. Plasma nicotine and cotinine levels in male and female rats at day 28 and day 90.
Food consumption
Initial food consumption decreases in males was noted for Formulation 1 and 2 groups in the high dose level. Some of these decreases were statistically significant up to about Day 49, after which they were stabilized and more similar to the low and mid groups. The declines for Formulation 2 high group males persisted for nearly the entire 90-day exposure duration. Food consumption was greater in Vehicle exposed males. Females’ food consumption was relatively unaffected by dose and treatment group compared to males during the study. In general, decreased food consumption in males was correlated with lower body weights during the 90-day exposure interval, and this persisted during recovery (data shown in the Supplemental Tables S1 and S2).
Organ, tissue and body weights
Non-significant dose-related reductions in spleen and thymus weights without microscopic correlates or dose response were noted in males only from the low-, mid- and high-dose levels of the Formulation 1, Formulation 2 and the Reference product groups (shown in the Supplemental Table S3) at the 28-day necropsy.
At the 90-day necropsy, higher lung weights were noted in high-dose exposed animals from all treatment groups compared with low- and mid-dose levels of the respective groups (shown in the Supplemental Table S4). Higher lung weights were associated with vehicle-related microscopic findings. The changes resolved during recovery (shown in Supplementary material; Supplemental Table S5).
Clinical chemistry
At day 28, mean total protein and albumin values were slightly lower in high-dose Formulation 1 and 2 group males, compared to the corresponding group low- and mid-dose group males (shown in the Supplemental Table S6). Albumin was also slightly lower for high dose Formulation 1 exposed females. At day 90 (shown in the Supplemental Table S7), there were some increases in serum phosphorus levels in male and female in the high-dose vehicle and Formulation 1 exposed groups, which persisted in high-dose Formulation 1 group males at the end of recovery (shown in the Supplemental Table S8). At recovery necropsy, the total protein and albumin differences observed at earlier time points were resolved.
Hematology
There were no clear treatment-related vehicle or test formulation-related alterations in hematology or coagulation parameters at the 28-, 90- or 132-day intervals (shown in the Supplemental Tables S9–S11, respectively).
BALF analysis
The results of BALF analysis at the 28-, 90- or 132-day necropsies are shown in , respectively. Vehicle-related findings were consistent for vehicle control and test formulation-treated groups for both sexes, and the findings correlated with treatment durations.
Table 6. Analysis of BALF from male and female rats exposed to various dose levels of vehicle, Formulation 1, Formulation 2 or Reference product by inhalation for 28 days.
Table 7. Analysis of BALF from male and female rats exposed to various dose levels of vehicle, Formulation 1, Formulation 2 or Reference product by inhalation for 90 days.
Table 8. Analysis of bronchoalveolar lavage fluid from male and female rats exposed to various dose levels of vehicle, Formulation 1, Formulation 2 or Reference product by inhalation for 90 days followed by a 42-day recovery period.
Rats from the high-dose vehicle control group had increased BALF total protein and ALP at 28 days, and higher BALF total protein, ALP and LDH at 90 days compared with the low- and mid-dose vehicle control groups. In females from the high-dose Formulation 1 group at 90 days, BALF showed higher total protein and ALP values compared with those from the low-dose and mid-dose Formulation 1 groups.
Rats from the Formulation 2 high-dose group had elevated ALP, LDH and total protein values compared with the low- and mid-dose Formulation 2 at 90 days. In general, the mean BALF total protein, ALP and LDH values in males and females from the high-dose Formulation 2 groups at days 28 and 90 were lower than the respective mean values for males and females from the high-dose vehicle control group. By day 132 following recovery, all BALF clinical chemistry changes were resolved.
Bronchoalveolar lavage fluid cytology showed higher levels of neutrophils and lymphocytes, and lower proportions of large mononuclear cells in males and females at days 28 and 90 in the high-dose vehicle control groups compared with low-dose and mid-dose vehicle control groups. Lymphocytes were slightly higher in the high-dose vehicle control at days 28 and 90.
Bronchoalveolar lavage fluid cytology findings in the Formulation 2 group showed higher proportions of neutrophils and lower levels of large mononuclear cells in the high-dose at days 28 and 90 compared with low- and mid-dose levels. In general, at days 28 and 90, animals from the high-dose Formulation 2 group demonstrated a proportion of neutrophils that were lower or similar to the respective proportion of neutrophils in males and females from the high-dose vehicle control group. These findings did not completely resolve during recovery.
Histopathology
Microscopic evaluation () after 28 days exposure to vehicle control, Formulation 1, Formulation 2 and Reference product showed changes in the nose, lung and larynx.
Table 9. Histopathology incidence findings in male and female rats exposed to various dose levels of vehicle, Formulation 1, Formulation 2 or Reference product by inhalation for 28 days.
Mild mucus cell hyperplasia was observed in the anterior nasal sections with a similar incidence and severity in all groups. Mild vacuolation of ciliated respiratory epithelium was observed in nasal sections () in all groups which persisted at day 90 and recovery necropsies. There was no dependence upon exposure formulation or level.
Figure 3. Photomicrograph of section through nose of male rat at nasal section 1 depicting mucus cell hyperplasia of the epithelium after 90-days inhalation exposure to high dose of Formulation 2 (20 × magnification).
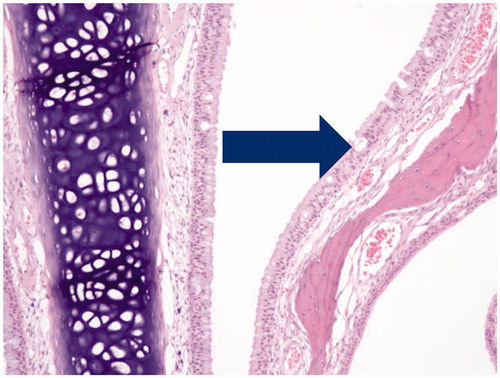
Dose-related increased incidence of alveolar macrophages was noted in the lungs of males and females from groups exposed to low-, mid- and high-doses of vehicle control, Formulation 1 and Formulation 2. For the high-dose groups, the highest incidence of this finding was observed in the vehicle control and Formulation 1 groups, and the lowest incidence was observed in the Reference product group. The histopathologic finding is shown in .
Figure 4. Photomicrographs of pulmonary alveolar macrophages (“foam cells”) in the lung of male rats after 90-days of inhalation exposure to high dose of (A) vehicle control or (B) Formulation 2 (20 × magnification).
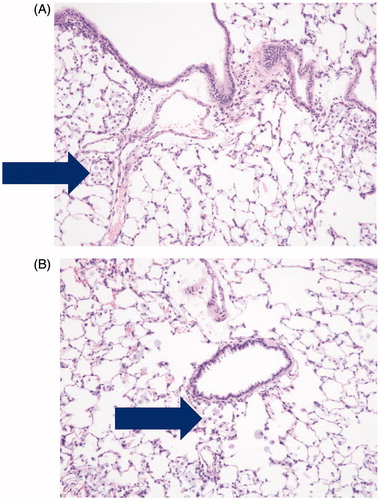
A mucin luminal exudate without epithelial changes was noted in the larynx of all treatment groups and was not dose related (). Histopathology incidence and severity are summarized in for days 28, 90 and through the recovery period.
Figure 5. Cross-section through the larynx of a male rat exposed to high dose of Formulation 2, (4 × magnification depicting luminal exudate, primarilly mucin).
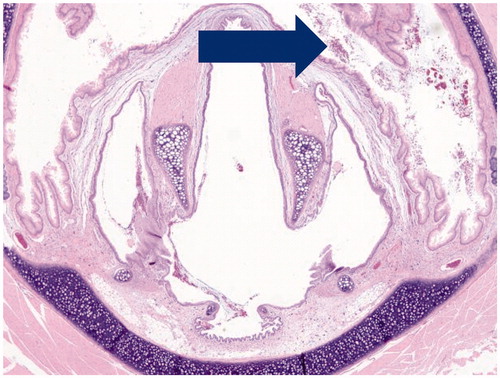
Table 10. Histopathology incidence findings for males and females rats exposed to various dose levels of vehicle control, Formulation 1, Formulation 2 or Reference product by inhalation for 90 days.
Table 11. Histopathology incidence findings in rats exposed to various dose levels of vehicle control, Formulation 1, Formulation 2 or Reference product by inhalation for 90 days followed by a 42-day recovery period.
At recovery, vehicle-related findings noted in the nose, lung and larynx at the 28- and 90-day necropsies were still observed () in rats from all treatment groups. There was persistent non-progressive, mucous cell hyperplasia in the nasal cavity and reduced level of alveolar macrophages in the lungs. The relative incidence of alveolar macrophages was lower in almost all treatment groups when compared with the 90-day necropsy, which indicated partial recovery. At recovery, centriacinar and subpleural alveolar macrophages were the main finding. An alveolar septal lesion with minimal fibrosis was found in a focal lesion of one rat.
A few males exposed to Formulation 1 had moderate to severe dental dysplasia of the incisors with grossly observed malaligned incisors considered unrelated to exposure as dental lesions are a common background finding in longer term studies (Losco, Citation1995).
Discussion
There are a number of reports in the scientific literature on the evaluation of e-cigaret ingredient components, formulations and emissions using analytical chemistry (Kim & Shin, Citation2013; Laugesen, Citation2008,Citation2009; Westenberger, Citation2009) and standardized in vitro assays such as cytotoxicity assays using various cell lines (Bahl et al., Citation2012; Behar et al., Citation2014; Farsalinos & Polosa, Citation2014; Romagna et al., Citation2013). A few studies have evaluated the biological effects from inhaled propylene glycol (Robertson et al., Citation1947; Suber et al., Citation1989; Werley et al., Citation2011) and glycerol (Renne & Gideon, Citation2006; RJ Reynolds Tobacco Company, Citation1988), two commonly used vehicles in e-cigaret aerosol formulations. An abundance of literature exists on nicotine and its pharmacologic, physiologic, toxicologic and sensory effects when delivered through many different routes of exposure (Fagerström, Citation2014).
The modern e-cigaret product is a device that is used in to generate a respirable aerosol and formulation. The formulations are usually composed of GRAS flavors, vehicle ingredients and nicotine. All these components may potentially contribute to the chemical composition of the aerosol to which the vaper is exposed. E-cigaret aerosol is a complex mixture that is not yet fully characterized, and it may contain unintended and unknown chemical entities (Burstyn, Citation2014). These chemical compounds may be formulation components per se, result from device materials or components, result from chemical reactions of formulation components and/or from the aerosol generation process itself (which involves heating and aerosolization of the formulation). Others have reported on these contaminants and inclusions in commercial e-cigaret products (Etter et al., Citation2013; Goniewicz et al., Citation2014; Kim & Shin, Citation2013; Williams et al., Citation2013).
As stated previously, the use of GRAS ingredients is not wholly sufficient to mitigate the safety of e-cigaret formulations. Therefore, to assess the safety profile for the prototype e-cigaret and three formulations, we conducted an inhalation exposure study using rats to evaluate the potential biological impact of vehicle, nicotine and a commercial flavor formulation at relevant levels of daily aerosol exposure as well as elevated levels up to 10-fold higher. Daily aerosol exposures in the low-, mid- and high-dose exposure groups were equivalent to human exposure doses of approximately 160, 480 and 1600 mg/day of aerosol mass, respectively.
The no observed effect level (NOEL) established in this study was the mid-dose level of approximately 9.6 mg/kg/day for each formulation based upon decreased body weights. The greatest attenuation was found for males and females exposed at the high-dose level, with body weight differences exceeding the 10% necessary to define the maximum tolerated dose (MTD). The vehicle control groups had the smallest attenuation in body weights. The greater decreases in the mean body weights of the other exposure groups might be attributed to effects of exposure to make the animals unwell and unwilling to eat and drink, or may be related to the well-known and described appetite suppressant effects of nicotine. In general, body weights in males and females tracked food consumption on a weekly basis, which was lowest for the males in the high-dose Formulation 1 and Formulation 2 groups up to study day 90. After day 90, differences in food consumption were less across all groups, but males did not match the vehicle control groups’ food consumption. This observation may be due to the already smaller body mass from which the animals did not fully recover by day 132 (end of recovery). In females, food consumption trends were similar to males but with smaller differences in food consumption in females from the Formulation 1 group compared with the vehicle control groups up to day 90. However, in females after the 90-day time point when animals were no longer exposed to the aerosols, the Formulation 1 groups’ food consumption values exceeded food consumption values compared with the vehicle control groups until the end of the recovery period. In general, males were more affected by exposure to the three formulations, with the largest impact upon body weight and food consumption in high-dose exposed, and nicotine treatment groups. Females were less affected with smaller, albeit similar, trends in body weights and food consumption values compared with males. These findings (males showing a greater response than females) are not uncommon in inhalation toxicity studies and may be associated with an irritation response to which females appear to be somewhat more resistant.
In general, the organ and tissue weight decreases that suggest exposure-related effects are correlated with body weight declines in mid- and high-dose groups, particularly in those exposed to Formulation 1 and Formulation 2. The organ and tissue weight decreases lack histopathological correlates except for the lungs. All the organ weight differences appear to be secondary to body weight effects for exposure to these formulations and all these findings are not considered adverse. Lung weight increases were observed in all treatment groups after 90 days of exposure to any aerosol formulation. These lung weight differences are associated with histopathology changes (described later), that were nearly resolved at day 132, after the 42-day recovery period.
Perturbations in total protein and albumin are likely associated with decreased nutritional status of the animals that sometimes simultaneously showed body weight declines and lower food consumption as was observed in this case. In this study, this could be due to the appetite suppressant effects of nicotine or due to the irritant effects of inhalation exposure to the test formulations. All males from high-dose groups had slightly elevated phosphorus levels at day 90, and this persisted through day 132. Plasma phosphorus changes are usually associated with aging in rats, and toxic responses sometimes result in reduced plasma phosphorus correlated with organ or tissue changes. Sie et al. (Citation1974) reported that feeding with high levels of phosphorus increased plasma phosphorus and decreased calcium with corresponding effects upon the bone. These effects were not observed in this study. All the BALF chemistry and cytology findings were considered to be vehicle related. BALF analysis of biochemical markers and cytology showed lung inflammatory responses in males and females at day 28 in the high-dose vehicle group and by day 90, the lung inflammatory response was noted in all treatment groups at the high dose. The inflammatory responses were generally higher for vehicle control groups, compared with Formulation 1 and Formulation 2 groups at day 90, and resolved at day 132. Lung cytology at days 28 and 132 showed elevated neutrophils, slightly elevated lymphocytes and correspondingly lower percentages of large mononuclear cells (alveolar macrophages) in the high-dose groups, with vehicle control groups showing the most marked response. At day 90, the high dose had increased numbers of lysed cells, some possibly identified as ciliated columnar epithelium cilia. The observed alveolar macrophages had increased vacuolization, with blue-gray material in the cytoplasm presumed to be engulfed test article. By day 132, the cytology differences had partially resolved. These data suggest that exposure to any of the formulations caused a lung inflammatory response with recruitment of neutrophils and alveolar macrophages, which phagocytized the test material during the slow process of clearing the lung. There is evidence of subclinical tissue damage related to the presence of biochemical markers that are hallmarks for general cytotoxicity, alveolar Type II cell cytotoxicity and microvascular leakage (i.e. LDH, ALP and total protein, respectively). Minimal tissue damage might be indicated by the possible unconfirmed presence of ciliated columnar epithelial debris. However, in spite of these changes described above, there were no correlated histopathological changes indicating morphological tissue changes or damage associated with exposure to any of the test materials (vehicle control, Formulation 1 and Formulation 2). At day 132, all the biochemical differences had resolved, and persistent populations of alveolar macrophages continued to clear the tissue of inhaled test material. There was no indication of further morphological tissue changes as commonly observed with inhaled insoluble particles, poorly soluble drugs and nanoparticles (Byrne & Baugh, Citation2008; Oberdörster et al., Citation2007). Indeed, even the presence of alveolar macrophages at day 132 is not unusual or unexpected considering that, except for absorption, the only removal mechanism in the deep lung is via alveolar macrophage activity, which is relatively slow (Oberdörster et al., Citation2007). Even for small amounts of respirable particulates that do not overload macrophages, the pulmonary clearance half-life is approximately 100 days in the rat lung (Oberdörster et al., Citation1992). According to the criteria established by the Society of Toxicologic Pathology Working Group and published by Nikula et al. (Citation2014), such a response should be considered a normal adaptive response of the lung since “findings suggest that even in susceptible populations, there is no evidence for any functional adverse effect due to increased alveolar macrophages”.
At 90 days, the only morphologic changes in the tissues included effects upon the nasal epithelium, larynx and lungs. A similar product exposure for comparison, which has been studied extensively and reported in the literature, is combustible tobacco products such as cigarets. In general, smoking-related effects upon the tissues in the respiratory tract are more adverse than those observed in our study, with increased incidence and severity, although a side-by-side comparison with tobacco smoke was not used in this study. Refer to Coggins (Citation2007) for a thorough review of tobacco smoke-related effects in inhalation studies in animals. These three vehicle-related microscopic findings, mucous cell hyperplasia in the nasal cavity and lungs and laryngeal exudates, were generally observed with no dose response, including at the recovery necropsy, and were of unlikely or uncertain toxicological significance. Mucous cell hyperplasia in the nasal cavity was considered non-adverse, and believed to represent an adaptive response to long-term tissue irritation. Dose-related increased macrophages in the lungs, were persistent and correlated with increased lung weights but not clearly formulation related. Vehicle-exposed groups had the highest exposure-related pulmonary effects. In general, long-term macrophage infiltration to the lung without underlying tissue damage is considered an adaptive response to toxic insult.
There were no microscopic changes specifically associated with exposure to Formulation 1, Formulation 2 or Reference product at the interim, primary or recovery necropsies. The prevalent changes were attributed to vehicle exposure and noted in the nose, lung and larynx. These vehicle-related changes were similar among the different formulation groups including vehicle and occasionally showed a dose-related increase in incidence (lung only).
The NOEL established in this study was the mid-dose level of approximately 9.6 mg/kg/day for each formulation based upon decreased body weights. The greatest attenuation was found for males and females exposed at the high exposure concentration, with body weight differences approaching or greater than 10% necessary to define the MTD. The vehicle exposure groups had the smallest attenuation in body weights, while greater decreases in the other groups might be attributed to nicotine in the formulations since it is well known that nicotine acts to suppress appetite (Mineur et al., Citation2011) acting either alone or in combination with the formulations.
Supplementary material available online
IIHT_1130758_Supp_File.pdf
Download PDF (410.3 KB)Acknowledgments
The authors acknowledge the statistical contribution of Les Freshwater, the veterinary pathology evaluation by Gail Walter, MT (ASCP), DVM, DACVP, DABT and inhalation exposure technical assistance of Mike Cockburn. The authors greatly appreciate the technical writing and editorial assistance provided by Eileen Y. Ivasauskas (Accuwrit Inc).
Declaration of interest
Dr. Daniel Kirkpatrick is employed by the contract laboratory that conducted these studies.
References
- Bahl V, Lin S, Xu N, et al. (2012). Comparison of electronic cigarette refill fluid cytotoxicity using embryonic and adult models. Reprod Toxicol 34:529–37
- Behar RZ, Davis B, Wang Y, et al. (2014). Identification of toxicants in cinnamon-flavored electronic cigarette refill fluids. Toxicol In Vitro 28:198–208
- Bullen C, Williman J, Howe C, et al. (2013). Study protocol for a randomised controlled trial of electronic cigarettes versus nicotine patch for smoking cessation. BMC Public Health 13:210
- Burstyn I. (2014). Peering through the mist: systematic review of what the chemistry of contaminants in electronic cigarettes tells us about health risks. BMC Public Health 14:18
- Byrne JD, Baugh JA. (2008). The significance of nanoparticles in particle-induced pulmonary fibrosis. McGill J Med 11:43–50
- CDC. (2015). Comments of the National Institute of Safety and Health to the Food and Drug Administration (FDA) in response to establishment of public docket; Electronic cigarettes and the public health workshop, Docket no. FDA-2014-N-1936, May 8
- Coggins CR. (2007). An updated review of inhalation studies with cigarette smoke in laboratory animals. Int J Toxicol 26:331–8
- Costigan S, Meredith C. (2015). An approach to ingredient screening and toxicological risk assessment of flavours in e-liquids. Regul Toxicol Pharmacol 72:361–9
- Ellis SP, Underwood MD, Arango V, Mann JJ. (2000). Mixed models and multiple comparisons in analysis of human neurochemical maps. Psychiatry Res 99:111–19
- Etter JF, Bullen C. (2014). A longitudinal study of electronic cigarette users. Addict Behav 39:491–4
- Etter JF, Zather E, Svensson S. (2013). Analysis of refill liquids for electronic cigarettes. Addiction 108:1671–9
- Fagerström K. (2014). Nicotine: pharmacology, toxicity and therapeutic use. J Smok Cessat 9:53–9
- Farsalinos KE, Polosa R. (2014). Safety evaluation and risk assessment of electronic cigarettes as tobacco cigarette substitutes: a systematic review. Ther Adv Drug Saf 5:67–86
- Goniewicz ML, Knysak J, Gawron M, et al. (2014). Levels of selected carcinogens and toxicants in vapour from electronic cigarettes. Tob Control 23:133–9
- Jensen RP, Luo W, Pankow JF, et al. (2015). [Letter] Hidden formaldehyde in e-cigarette aerosols. N Engl J Med 372:392–3
- Kim HJ, Shin HS. (2013). Determination of tobacco-specific nitrosamines in replacement liquids of electronic cigarettes by liquid chromatography-tandem mass spectrometry. J Chromatogr A 1291:48–55
- Klimisch HJ, Meissner K, Wernicke H. (1974). Quantitative determination of carbon monoxide in blood by gaschromatography. Z Klin Chem Klin Biochem 12:535–8
- Kosmider L, Sobczak A, Fik M, et al. (2014). Carbonyl compounds in electronic cigarette vapors-effects of nicotine solvent and battery output voltage. Nicotine Tob Res 16:1319–26
- Laugesen M. (2008). Safety Report on the Ruyan® E-Cigarette Cartridge and Inhaled Aerosol. Available from: http://www.healthnz.co.nz/RuyanCartridgeReport30-Oct-08.pdf. [Last accessed: 10 Aug 2015]
- Laugesen M. (2009). Ruyan® E-Cigarette Bench-Top Tests. Society for Research on Nicotine and Tobacco (SRNT) Dublin, Poster 5-11. Available from: http://www.healthnz.co.nz/DublinEcigBenchtopHandout.pdf. [Last accessed: 10 Aug 2015]
- Lerner CA, Sundar IKHY, Gerloff J, et al. (2015). Vapors produced by electronic cigarettes and e-juices with flavorings induce toxicity, oxidative stress, and inflammatory response in lung epithelial cells and in mouse lung. PLoS One 10:e0116732
- Losco PE. (1995). Dental dysplasia in rats and mice. Toxicol Pathol 23:677–88
- Matsuzawa T, Inoue H, eds. CD(SD)IGS Study Group. (1998). Biological Reference Data on CD(SD)IGS Rats – 1998. Yokohama, Japan: CD(SD)IGS Study Group
- Mery S, Gross EA, Joyner DR, et al. (1994). Nasal diagrams: a tool for recording the distribution of nasal lesions in rats and mice. Toxicol Pathol 22:353–72
- Mineur YS, Abizald A, Rao Y, et al. (2011). Nicotine decreases food intake through activation of POMC neurons. Science 332:1330–2
- Morgan KT. (1991). Approaches to the identification and recording of nasal lesions in toxicology studies. Toxicol Pathol 19:337–51
- Nikula KJ, McCartney JE, McGovern T, et al. (2014). STP position paper: interpreting the significance of increased alveolar macrophages in rodents following inhalation of pharmaceutical materials. Toxicol Pathol 42:472–86
- Norwood DL, Paskiet D, Ruberto M, et al. (2008). Best practices for extractables and leachables in orally inhaled and nasal drug products: an overview of the PQRI recommendations. Pharm Res 25:727–39
- Oberdörster G, Ferin J, Morrow PE. (1992). Volumetric loading of alveolar macrophages (AM): a possible basis for diminished AM-mediated particle clearance. Exp Lung Res 18:87–104
- Oberdörster G, Stone V, Donaldson K. (2007). Toxicology of nanoparticles: a historical perspective. Nanotoxicology 1:2–25
- OECD. 2009. OECD guideline for the testing of chemicals. No. 413 Subchronic inhalation toxicity, 90-day study. Available from: http://www.oecd-ilibrary.org/docserver/download/9741301e.pdf?expires=1439839455&id=id&accname=guest&checksum=E75DDFFD7B5D748E1B9FCBC8E4D37C09. [Last accessed: 10 Aug 2015]
- Petterino C, Argentino-Storino A. (2006). Clinical chemistry and haematology historical data in control Sprague-Dawley rats from pre-clinical toxicity studies. Exp Toxicol Pathol 57:213–19
- Renne RA, Wehner AP, Greenspan BJ, et al. (1992). 2-Week and 13-week inhalation studies of aerosolized glycerol in rats. Inhal Toxicol 4:95–111
- Renne RA, Gideon KM. (2006). Types and patterns of response in the larynx following inhalation. Toxicol Pathol 34:281–5
- RJ Reynolds Tobacco Company. (1988). Chemical and biological studies, new cigarette prototypes that heat instead of burn tobacco. Winston-Salem, NC: RJ Reynolds Tobacco Company
- Robehmed N. (2013). Forbes Magazine. Available from: http://www.forbes.com/sites/natalierobehmed/2013/09/17/e-cigarette-sales-surpass-1-billion-as-big-tobacco-moves-in/. [Last accessed: 10 Aug 2015]
- Robertson OH, Loosli CG, Puck TT, et al. (1947). Tests for the chronic toxicity of propylene glycol and triethylene glycol on monkeys and rats by vapor inhalation and oral administration. J Pharmacol Exp Ther 91:52–76
- Romagna G, Allifranchini E, Bocchietto E, et al. (2013). Cytotoxicity evaluation of electronic cigarette vapor extract on cultured mammalian fibroblasts (ClearStream-LIFE): comparison with tobacco cigarette smoke extract. Inhal Toxicol 25:354–61
- Sie TL, Draper HH, Bell RR. (1974). Hypocalcemia, hyperparathyroidism and bone resorption in rats induced by dietary phosphate. J Nutr 104:1195–201
- Suber RL, Deskin R, Nikiforov I, et al. (1989). Subchronic nose-only inhalation study of propylene glycol in Sprague–Dawley rats. Food Chem Toxicol 27:573–83
- US DHHS. (2012). US Department of Health and Human Services, Food and Drug Administration, Center for Tobacco Products, Guidance for Industry. Modified Risk Tobacco Product Applications. Draft Guidance. Available from: http://www.fda.gov/downloads/TobaccoProducts/Labeling/UCM297751.pdf. [Last accessed: 10 Aug 2015]
- VanVunakis H, Gjika HB, Langone JJ. (1987) Method 16 – Radioimmunoassay for nicotine and cotinine. In: International Agency for Research on Cancer, ed. Environmental carcinogens, methods of analysis and exposure measurement, Vol. 9. Passive smoking. International Agency for Research on Cancer, Scientific Publications No. 81. Oxford, UK: Oxford University Press
- Waldum HL, Nilsen OG, Nilsen T, et al. (1996). Long-term effects of inhaled nicotine. Life Sci 58:1339–46
- Werley MS, McDonald P, Lilly P, et al. (2011). Non-clinical safety and pharmacokinetic evaluations of propylene glycol aerosol in Sprague-Dawley rats and Beagle dogs. Toxicology 287:76–90
- Westenberger B. (2009). Evaluation of e-cigarettes. Available from: http://www.fda.gov/downloads/drugs/Scienceresearch/UCM173250.pdf. [Last accessed: 10 Aug 2015]
- Williams M, Villarreal A, Bozhilov K, et al. (2013). Metal and silicate particles including nanoparticles are present in electronic cigarette cartomizer fluid and aerosol. PLoS One 8:e57987