Abstract
In previous work we described six point mutations that thermostabilised the turkey β1-adrenergic receptor (tβ1AR). The thermostable mutant, tβ1AR-m23, had an apparent Tm 21°C higher than the native protein when solubilized in dodecylmaltoside (DDM) and, in addition, was significantly more stable in short chain detergents, which allowed its crystallization and structure determination. Identification of thermostabilizing mutations in tβ1AR was performed by systematic mutagenesis followed by expressing and assaying each of the 318 mutants for their thermostability. This is time-consuming, so to facilitate studies on related receptors, we have studied the transferability of these mutations to the human adrenergic receptors, hβ1AR and hβ2AR, which have, respectively, 76% and 59% sequence identity to tβ2AR, excluding the N- and C-termini. Thermostability assays revealed that hβ1AR was much more unstable than tβ2AR, whereas hβ2AR was more stable than tβ1AR. Addition of the 6 thermostabilizing mutations in tβ2AR-m23 into both hβ2AR and hβ2AR increased their apparent Tms by 17°C and 11°C, respectively. In addition, the mutations affected the global conformation of the human receptors so that they were predominantly in the antagonist bound form, as was originally observed for tβ2AR-m23. Thus, once thermostabilizing mutations have been identified in one G protein-coupled receptor, stabilization of close members within the subfamily is rapidly obtainable.
Introduction
Thermostability of integral membrane proteins in detergent solution has proven to be an important parameter in the successful production of diffraction-quality crystals for structure determination by X-ray crystallography. Although rigorous comparative studies are unavailable, circumstantial evidence suggests that the most stable membrane proteins are the most likely to form crystals that diffract to high resolution and are therefore the most amenable to structure determination. The corollary that membrane proteins that are unstable in detergents are unlikely to form well-diffracting crystals is self-evident, but often the situation is even worse, because many membrane proteins are inactivated immediately upon solubilization, which precludes their purification in a functional form. Therefore, methods that can stabilise membrane proteins in detergent solution have been sought for many years and numerous solutions have been described, including mutagenesis [Citation1–5], new detergents [Citation6–11] and additives [Citation2,Citation12,Citation13]. A key component in maintaining the stability of many membrane proteins is the presence of lipids. Some membrane proteins bind lipids relatively tightly, which means that during purification the lipids are not removed and, if sufficiently ordered, may be observed in the crystal structure [Citation14,Citation15]. In contrast, other membrane proteins are readily inactivated during purification, but this can be prevented by the addition of either polar phospholipids [Citation16,Citation17] or lipidic additives such as cholesteryl hemisuccinate [Citation12,Citation18] and, in some instances, crystals can then be grown from the purified protein [Citation13,Citation16,Citation19,Citation20]. In this regard, crystallization using lipidic mesophases seems a choice strategy [Citation21], because the crystallization occurs from proteins incorporated into the mesophase [Citation22,Citation23], thus circumventing problems of large lipid-rich micelles preventing crystallization due to the occlusion of hydrophilic surfaces of small membrane proteins such as G protein-coupled receptors (GPCRs) [Citation24]. Mutagenesis to identify point mutations that improve thermostability [Citation2-4] is another strategy that has yielded the structure of a ligand-binding GPCR [Citation25]. A number of studies have demonstrated that point mutations can thermostabilise membrane proteins in detergent solution and that, when combined, the thermostability of the protein can be enhanced dramatically [Citation1,Citation5,Citation26]. The improvement in thermostability correlates with a general increase in stability such as increased half-life and, especially, increased tolerance to short chain detergents that are ideal for crystallization.
Engineering a GPCR for improved thermostability can be achieved by either random mutagenesis coupled to a screening or selection procedure [Citation5,Citation27] or by systematic scanning mutagenesis and optimal combination of thermostabilizing mutations identified from the screen [Citation1–4]. We have thermostabilized three GPCRs using the latter strategy; this entailed mutating every amino acid residue in the receptor to either alanine or leucine, expressing every mutant and determining its thermostability and finally finding the best combination of 4–6 thermostabilizing mutations to construct the optimally stable receptor [Citation2–4]. Thermostable mutants were identified by measuring the amount of functional detergent-solubilized receptor after incubation at elevated temperatures and, as a consequence of this, the receptors, normally in equilibrium between a number of conformations, were preferentially stabilized in a single conformation related to the state conferred upon the receptor when binding the radioligand used in the selection procedure. Thus the turkey β1-adrenergic receptor mutant tβ1AR-m23 was stabilized in an antagonist-bound conformation [Citation3] whilst the neurotensin receptor (NTS1) was stabilized in an agonist-bound conformation [Citation4]. The adenosine A2a receptor was stabilized in both agonist- and antagonist-bound conformations, and it was noticeable that of the 17 thermostabilizing mutations for the antagonist conformation, only three were identical to mutations that stabilized the agonist conformation [Citation2]. We have therefore called this procedure conformational thermostabilization. The structure of tβ1AR-m23 was subsequently determined to 2.7 Å resolution [Citation25].
Conformational thermostabilization of GPCRs is ideally performed after assaying all the mutants from a complete mutagenic scan throughout the protein, which means constructing, expressing and assaying 315–330 mutants for a typical GPCR. This is quite time consuming. It would, therefore, be advantageous to transfer thermostabilizing mutations between receptors to save on the amount of work required. Out of about 90 thermostabilizing mutations identified in GPCRs by Ala scanning so far [Citation2–4], only two are predicted to be in the identical position from an alignment of the amino acid sequences, so it is unlikely that there is good transferability of thermostability between distantly related receptors. We have, therefore, focussed our attention on the transferability of thermostabilizing mutations between closely related receptors in the same family, using the mutations in tβ1AR-m23 as our starting point. Turkey β1AR is 76% and 59% identical with human β1AR and β2AR respectively (N- and C-termini removed in the alignments; ), so it was possible that the mutations that stabilized tβ1AR could also stabilise hβ1AR and hβ2AR. We therefore transferred all six thermostabilizing mutations en bloc into the human βARs and tested their thermostability.
Figure 1. (a) Sequence alignment between the turkey β2AR (adrb_melga) and human βARs (adrb1/2/3_human). The N- and C-termini of the sequences were removed in the alignment, because there is no significant homology between them. Amino acid residues coloured red in the turkey sequence correspond with mutations present in tβ2AR-m23 (R68S, M90V, Y227A, A282L, F327A and F338M), whereas those coloured in blue correspond with other thermostabilizing mutations. Letters above these residues correspond to the position of the residues in (b). Pink rectangles below the sequence alignment indicate the positions of transmembrane domains and the green rectangle corresponds with the position of helix 8. (b) Structure of the turkey β2AR (PDB accession number 2VT4) with the side chains of thermostabilizing mutations represented as space filling models. Side chains in grey are thermostabilizing when mutated to alanine. The five magenta side chains correspond to the five thermostabilizing mutations found in tβ2AR-m23, i.e., R68S, M90V, Y227A, F327A and F338M; the mutation A282L is not shown because this region of the receptor was disordered in this crystal form. The N- and C-termini are labelled, along with the transmembrane α-helices. The model is in rainbow coloration with the N terminus in blue and the C terminus in red. The black sphere is a Na+ ion. This Figure is reproduced in colour in Molecular Membrane Biology online.
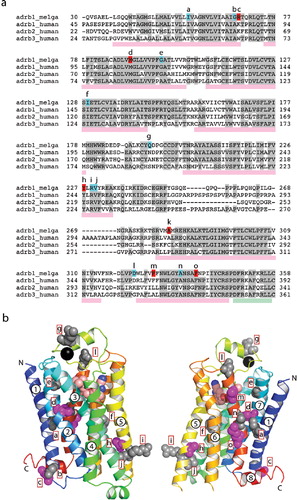
Materials and methods
Clones encoding the human β1, β2 and β3 adrenergic receptors were supplied in pcDNA3.1+ by Missouri S&T cDNA Resource centre (www.cdna.org). Turkey β2AR (tβAR34-424, residues indicated as subscripts) [Citation28] and the mutated version β2AR-m23 [Citation3] were both subcloned into pcDNA3. 1-[4,6-propyl-3H]dihydroalprenolol ([3H]DHA) was supplied by Amersham Bioscience. (-)-isoprenaline hydrochloride, (-)-adrenaline, and (-)-cyanopindolol hemifumarate salt were from Sigma.
Site-directed mutagenesis and receptor expression
Human receptors were mutated by PCR using primers containing the desired mutations as previously described [Citation3]. The C-terminal region of human β1AR was also truncated by PCR using a primer that contained a stop codon after Leu463. PCR reactions were carried out using KOD polymerase (Novagen), transformed into XL-Gold ultra competent cells (Stratagene) and mutants checked by sequencing mini-prepped plasmid DNA. Large-scale plasmid preps for transient transfection into mammalian cells was obtained using the EndoFree Plasmid Maxi Kit (Qiagen). Transient transfection of HEK293 cells was performed using GeneJuice (Novagen). After transfection, cells were incubated for 48 h and then harvested; 1 ml aliquots (2 million cells/ml) were centrifuged, cell medium removed and the cell pellets stored at −20°C. For the assays, cells were resuspended in 500 μl of buffer [75 mM Tris, pH 8, 12.5 mM MgCl2, 5 mM EDTA, protease inhibitors (Complete; Roche)].
Ligand binding and thermostability assays
Solubilization was performed by incubating cells (final concentration 1 million cells per ml) in buffer containing the desired detergent at the required concentration for 1 hour on ice. The solubilized receptors were immediately used in binding assays without centrifugation. Binding assays were performed by incubating 40 μl of solubilized receptor with 80 nM [3H]DHA in a final volume of 120 μl buffer [75 mM Tris, pH8, 12.5 mM MgCl2, 5 mM EDTA, 0.01% DDM or alternative detergent]. Equilibration was for 1 h at 4°C. Receptor-bound and free radioligand were separated by gel filtration as described previously [Citation29]. The thermostability was determined from solubilized receptors heated in the absence of ligand for 30 min at different temperatures.
Saturation binding curves were determined using a concentration range of [3H]DHA from 0.3–100 nM. Non-specific binding was determined in the presence of 8 μM of (-)-alprenolol. Competition assays were performed using a concentration of [3H]DHA of 22.5 nM for human β2AR1-463 and human β2AR1-463-m23, and 9 nM for human β2AR and human β2AR-m23 (i.e., three times the KD) and increasing concentrations of agonists (isoprenaline and adrenaline) and antagonist (cyanopindolol). Radioactivity was counted on a Beckman LS6000 liquid scintillation counter and data were analyzed by non-linear regression using Prism software (GraphPad).
Results
Expression and thermostability of human β1AR and β2AR
In the original studies on the thermostabilization of GPCRs, we expressed the receptors as maltose binding protein fusions in Escherichia coli [Citation2–4]. However, initial trials using a similar strategy for hβ1AR and hβ2AR gave poor expression levels. We therefore decided to express the receptors in HEK293 cells by transient transfection. Expression levels were calculated by binding assays with the radioligand 1-[4,6-propyl-3H]dihydroalprenolol ([3H]DHA). Expression of hβ1AR was very low so the C-terminus was truncated after Leu463 in order to increase the expression. The truncated receptor hβ2AR1-463 showed a 5-fold increase in expression compared to wild-type hβ2AR (data not shown). Similar truncations in the C-terminus of the tβ2AR did not affect either stability or expression levels. As a result, hβ2AR1-463 and β2AR had similar expression levels when assayed in membranes, although still lower than tβ2AR (). However, upon solubilization with 2% DDM, the activity of hβ2AR1-463 decreased dramatically ().
Figure 2. Expression and binding assays of turkey β2AR and human βARs expressed in HEK293 cells. The number of receptors per cell was calculated from single-point ligand binding assays using [3H]DHA at a final concentration of 80 nM performed either on membranes (a) or on cells solubilized with 2% DDM (b). Cells were transfected either with empty vector (V), turkey β2AR (tβ2), human β2AR1-463 (hβ2) or human β2AR (hβ2). Experiments were performed in duplicate and the error bars represent the standard error of the mean (SEM). This Figure is reproduced in colour in Molecular Membrane Biology online.
![Figure 2. Expression and binding assays of turkey β2AR and human βARs expressed in HEK293 cells. The number of receptors per cell was calculated from single-point ligand binding assays using [3H]DHA at a final concentration of 80 nM performed either on membranes (a) or on cells solubilized with 2% DDM (b). Cells were transfected either with empty vector (V), turkey β2AR (tβ2), human β2AR1-463 (hβ2) or human β2AR (hβ2). Experiments were performed in duplicate and the error bars represent the standard error of the mean (SEM). This Figure is reproduced in colour in Molecular Membrane Biology online.](/cms/asset/90384ae9-b1f9-420d-aca0-337e81cb1e36/imbc_a_420996_f0002_b.jpg)
This destabilization by DDM was not apparent for tβ1AR or hβ2AR, and was the first sign that hβ2AR was significantly less stable than the other two β receptors. The instability of detergent-solubilized hβ2AR at 4°C was demonstrated by solubilizing membranes in increasing amounts of DDM from 0.01–1% (). Both tβ1AR and hβ2AR showed similar activity between 0.05–1% DDM, but human β–AR1-463 showed decreased binding of [3H]DHA between 0.01–1% DDM, which was also observed in the wild type hβ2AR (data not shown). This effect is most likely due to the decreased stability of hβ2AR upon detergent-solubilization rather than a direct competition by detergent for the radioligand because the binding sites for hβ2AR and tβ2AR are virtually identical () and the monomeric DDM concentration (which would be most likely to affect ligand binding) does not increase over this detergent range as the critical micellar concentration (cmc) of DDM is 0.007%. The low amount of activity seen upon solubilization of tβ2AR and hβ2AR with 0.01% DDM is probably a reflection of poor solubilization of the receptors. The lowest concentration of DDM tested was deliberately chosen as just above the cmc so that receptors are only partially solubilized and probably have associated with them large amounts of lipid that will stabilise the receptors; this is corroborated by the observation that receptors partially solubilized in 0.01% DDM could be sedimented by centrifugation for 5 min at 13,000 g, but were still capable of passing through the gel filtration column used to separate receptor-bound [3H]DHA from free [3H]DHA.
Figure 3. The effect of increasing detergent concentrations on the [3H]DHA binding activity of βARs. Receptors were transiently expressed in HEK293 cells, which were subsequently solubilized at 4°C in different concentrations of DDM (0.01%, 0.05%, 0.1%, 0.5% and 1%); the amount of solubilized functional receptor was determined from a single-point binding assay performed at 4°C using 80 nM [3H]DHA; (a) turkey β2AR, (b) human β2AR1-463 and (c) human β2AR. Experiments were performed in duplicate and the error bars represent the SEM.
![Figure 3. The effect of increasing detergent concentrations on the [3H]DHA binding activity of βARs. Receptors were transiently expressed in HEK293 cells, which were subsequently solubilized at 4°C in different concentrations of DDM (0.01%, 0.05%, 0.1%, 0.5% and 1%); the amount of solubilized functional receptor was determined from a single-point binding assay performed at 4°C using 80 nM [3H]DHA; (a) turkey β2AR, (b) human β2AR1-463 and (c) human β2AR. Experiments were performed in duplicate and the error bars represent the SEM.](/cms/asset/baf73ffb-ddcd-4061-b93b-ef68ef88a107/imbc_a_420996_f0003_b.jpg)
The thermostability of all the solubilized receptors was determined by calculating the apparent Tm in the presence of either 0.1% or 0.01% of DDM (; ). In the presence of low amounts of detergent, the receptors had similar apparent Tms of 31°C, 21°C and 30°C for tβ2AR, hβ2AR1-463 and hβ2AR, respectively. However, tβ1AR and hβ2AR1-463 were less stable when solubilized in 0.1% DDM than in 0.01% DDM, with hβ2AR1-463 being particularly detergent-sensitive. This revealed that both β1 receptors prefer a lipid-rich environment rather than just DDM, whereas hβ2AR is largely insensitive to different DDM concentrations. For this reason, working with hβ2AR is especially advantageous for purification [Citation30] and crystallization [Citation19,Citation31,Citation32].
Figure 4. The stability of βARs with and without thermostabilizing mutations. Receptors were solubilized from transiently transfected cells using either 0.1% (red squares) or 0.01% DDM (blue triangles). Samples were then heated at the specified temperature for 30 minutes, quenched on ice and the amount of receptor remaining determined by a single-point ligand binding assay using 80 nM [3H]DHA performed in duplicate; (a) turkey β2AR, (b) human β2AR1-463, (c) human β2AR, (d) turkey β2AR-m23, (e) human β2AR1-463-m23, (f) human β2AR-m23. Stability curves were analyzed by non-linear regression using Prism (GraphPad) to determine the Tms (). This Figure is reproduced in colour in Molecular Membrane Biology online.
![Figure 4. The stability of βARs with and without thermostabilizing mutations. Receptors were solubilized from transiently transfected cells using either 0.1% (red squares) or 0.01% DDM (blue triangles). Samples were then heated at the specified temperature for 30 minutes, quenched on ice and the amount of receptor remaining determined by a single-point ligand binding assay using 80 nM [3H]DHA performed in duplicate; (a) turkey β2AR, (b) human β2AR1-463, (c) human β2AR, (d) turkey β2AR-m23, (e) human β2AR1-463-m23, (f) human β2AR-m23. Stability curves were analyzed by non-linear regression using Prism (GraphPad) to determine the Tms (Table I). This Figure is reproduced in colour in Molecular Membrane Biology online.](/cms/asset/3a2307c2-f667-442b-b04f-1b341c849d8a/imbc_a_420996_f0004_b.jpg)
Table I. Apparent Tms of β receptors solubilized in different concentrations of dodecylmaltoside (DDM) and decylmaltoside (DM).
Table II. Affinity of agonist and antagonist binding to detergent solubilized βARs.
The effect of turkey β2AR-m23 stabilizing mutations in human β receptors
Human β2AR1-463 and β2AR were mutated to incorporate the corresponding thermostabilizing mutations present in tβ2AR-m23 (R68S, M90V, Y227A, A282L, F327A and F338M; these six mutations are referred to as the m23 mutations). The apparent Tm of all the mutants carrying the m23 mutations was calculated to compare the stability with and without mutations (). For the human β receptors, the apparent Tm was increased regardless of the detergent concentration in the assay conditions, whereas the increase in apparent Tm for tβ2AR was more apparent in the presence of 0.1% DDM than in 0.01% DDM (). In the case of tβ2AR-m23 the mutations made the receptor more detergent resistant since the apparent Tm for the receptor solubilized in 0.1% or 0.01% DDM were similar (45°C). However, although the m23 mutations in hβ2AR1-463 increased its thermostability in both 0.01% and 0.1% DDM, with apparent Tms of 27°C and 38°C respectively, there is still an 11°C difference in the apparent Tm of the receptors when solubilized in the two different DDM concentrations. For hβ2AR-m23, the Tm was 41°C both in 0.1% and 0.01% DDM, respectively, which is an increase of 11°C over the wild-type receptor.
The stability of the human mutants was also measured when solubilized in decylmaltoside (DM), which is more denaturing than DDM. Human β receptors that contain the m23 thermostabilizing mutations are more resistant to higher detergent concentrations, as seen when comparing β–AR1-463 to β–AR1-463-m23 solubilized in DM (). The apparent Tms of the receptors with the m23 mutations improved about 10°C for hβ2AR1-463 and 4°C for hβ2AR in 0.1% DM. This is a smaller improvement than when the experiment was performed in DDM and is probably a reflection of the stabilization of the wild type receptors by the high amount of lipids in the DDM micelles.
Figure 5. Resistance of the human β2AR1-463 and β2AR to decylmaltoside. (a) Receptors were solubilized with different amounts of decylmaltoside (DM) and the ligand binding activity measured using 80 nM [3H]DHA. Red bars, hβ2AR1-463; pink bars, hβ2AR1-463-m23; dark blue bars, hβ2AR and light blue bars, hβ2AR-m23. The DM concentrations used are as follows: a, 0.1%; b, 0.3%; c, 1%. (b) The stability of the hβ2AR1-463 and hβ2AR solubilized in decylmaltoside (DM) was determined by incubating the sample for 30 minutes at different temperatures, quenching on ice and determining the amount of remaining receptors by assaying with 80 nM [3H]DHA: red, hβ2AR1-463; pink, hβ2AR1-463-m23; dark blue, hβ2AR and light blue, hβ2AR-m23. Stability curves were analysed by non-linear regression using Prism (GraphPad) to determine the Tms (). This Figure is reproduced in colour in Molecular Membrane Biology online.
![Figure 5. Resistance of the human β2AR1-463 and β2AR to decylmaltoside. (a) Receptors were solubilized with different amounts of decylmaltoside (DM) and the ligand binding activity measured using 80 nM [3H]DHA. Red bars, hβ2AR1-463; pink bars, hβ2AR1-463-m23; dark blue bars, hβ2AR and light blue bars, hβ2AR-m23. The DM concentrations used are as follows: a, 0.1%; b, 0.3%; c, 1%. (b) The stability of the hβ2AR1-463 and hβ2AR solubilized in decylmaltoside (DM) was determined by incubating the sample for 30 minutes at different temperatures, quenching on ice and determining the amount of remaining receptors by assaying with 80 nM [3H]DHA: red, hβ2AR1-463; pink, hβ2AR1-463-m23; dark blue, hβ2AR and light blue, hβ2AR-m23. Stability curves were analysed by non-linear regression using Prism (GraphPad) to determine the Tms (Table I). This Figure is reproduced in colour in Molecular Membrane Biology online.](/cms/asset/775c28c5-b7d8-4f86-af8a-f6295884399a/imbc_a_420996_f0005_b.jpg)
The six thermostabilizing m23 mutations were originally selected to stabilize tβ2AR, but subtle differences between the turkey and human receptors could mean that the optimal amino acid residue required to stabilize the human receptors could be different from those that stabilized tβ2AR. Consequently, new amino acid residue changes were made in hβ2AR1-463-m23 and hβ2AR-m23 at the positions of the m23 mutations. The stability of these mutants was tested by heating the receptors at a single temperature, but the majority of the mutants showed similar or worse thermostability compared to the original m23 mutants (Supplementary Tables S1 and 2, online version only). The exceptions in hβ1AR-m23 were K85L and K85N that were marginally more stable than K85S. In hβ2AR-m23, the mutation C265I appeared significantly more stable than C265L, and there were also slight improvements in thermostability for C265V and C265Q. These results suggest that the original combination of mutations in m23 is also good for the human βARs, but further optimization may increase slightly the thermostability of the mutant receptors.
The m23 thermostabilizing mutations had a dramatic effect on the preferred conformation of the receptor in the absence of ligands [Citation3]. Competition binding assays showed that tβ2AR-m23 was preferentially in the antagonist bound conformation, because the antagonist affinities were largely unchanged, whilst the affinities for agonists was weakened by up to 3 log units. We therefore tested the effect of the m23 mutations on the conformation of the human βARs by measuring the KD for the antagonist [3H]DHA (Supplementary Table S3, online version only) and by performing competition assays between [3H]DHA and increasing concentrations of the agonists isoprenaline and adrenaline and the antagonist cyanopindolol (). We observed that the KD for the antagonist [3H]DHA did not vary with the mutations, even in the presence of different amounts of detergent. In addition, competition assays using another antagonist, cyanopindolol, also showed an unchanged affinity. In contrast, the affinities for both agonists were reduced upon inclusion of the m23 mutations by up to 2 orders of magnitude. Thus the m23 mutations are affecting the conformation of the human βARs in a similar manner to how they affected the conformation of tβ2AR.
Figure 6. Competition binding assays of human βARs with and without the m23 thermostabilizing mutations. Increasing amounts of the agonists, isoprenaline (a) and adrenaline (b), and the antagonist cyanopindolol (c), were used to compete with binding of [3H]DHA present at a concentration three times greater than the KD for each receptor (Supplementary Table S3, online version only): red squares, hβ2AR1-463; pink circles, hβ2AR1-463-m23; dark blue triangles, hβ2AR; pale blue inverted triangles, hβ2AR-m23. Data were analyzed with GraphPad Prism and the Kis determined (). This Figure is reproduced in colour in Molecular Membrane Biology online.
![Figure 6. Competition binding assays of human βARs with and without the m23 thermostabilizing mutations. Increasing amounts of the agonists, isoprenaline (a) and adrenaline (b), and the antagonist cyanopindolol (c), were used to compete with binding of [3H]DHA present at a concentration three times greater than the KD for each receptor (Supplementary Table S3, online version only): red squares, hβ2AR1-463; pink circles, hβ2AR1-463-m23; dark blue triangles, hβ2AR; pale blue inverted triangles, hβ2AR-m23. Data were analyzed with GraphPad Prism and the Kis determined (Table II). This Figure is reproduced in colour in Molecular Membrane Biology online.](/cms/asset/191388c5-187f-40e2-80ce-35ff76313956/imbc_a_420996_f0006_b.jpg)
Discussion
The stability of detergent-solubilized GPCRs cannot be predicted from inspection of the primary amino acid sequence and there can be great variability between the thermostability of receptors from different species. The advantage of purifying the turkey β1AR compared to the human β1AR is clear from the thermostability assay, which indicates that the turkey receptor is significantly more stable than its human counterpart. This demonstrates the utility of thermostability assays in assessing the potential of a given membrane protein for structural studies. The method used here relies on having a radiolabelled ligand that can be used to rapidly determine the amount of functional receptor in detergent solution, without having to purify the receptor. The fluorescence-detection size exclusion chromatography (FSEC) assay has also been used to assess the stability of unpurified detergent-solubilized membrane proteins by monitoring the shape and mobility of a GFP fusion during size exclusion column chromatography [Citation33,Citation34]. The success of this strategy is based on the observation that misfolded membrane proteins usually migrate anomalously on a size exclusion column [Citation35]. The sensitivity of a receptor to high concentrations of detergent during solubilization, either in terms of ligand-binding or in terms of mobility, is also a clear indicator of instability. It is noticeable that the hβ2AR is insensitive to the concentration of DDM used for solubilization, but both the human and turkey β2ARs had higher apparent Tms when partially solubilized with low concentrations of DDM. To maintain receptor stability under these conditions during purification, it is probable that mixtures of DDM and lipid would have to be employed. As crystallization of small membrane proteins is best attempted when the micelle size is small, and additional lipid will increase the micelle size, this method of receptor stabilization may lead to problems in the formation of highly-diffracting crystals. This was why the thermostabilized tβ2AR receptor, tβ2AR-m23, was developed for crystallization, because it is insensitive to detergent concentration and can tolerate even denaturing short chain detergents that form small micelles [Citation3]. The structure of tβ2AR-m23 was determined to 2.7 Å resolution after crystallization using the detergent octylthioglucoside [Citation25].
The creation of the thermostabilized receptor tβ2AR-m23 took two years, including assay development and expression optimization. Although this process is now faster, it is still a lot of work, so if the mutations can be used to thermostabilise other receptors, there will be a considerable saving in time. We therefore transferred the six thermostabilizing mutations en bloc to the human β2 and β2 receptors, which improved their thermostability by 17°C and 11°C, respectively. Human β2AR and β2AR are 76% and 59% identical compared to tβ2AR (excluding the N and C termini), so it appears that the degree of thermostabilization is approximately proportional to the degree of amino acid similarity between the receptors. Thus, although transferring thermostabilizing mutations between receptors in the same family worked well, it may prove less successful if the mutations were transferred to distantly related receptors. This conclusion is substantiated by comparing the thermostabilizing mutations in the tβ2AR [Citation3], human adenosine A2a receptor [Citation2] and the rat neurotensin receptor [Citation4]. All these receptors were subjected to systematic mutagenesis and thermostabilizing mutations identified, but there is no clear homology between the positions of thermostabilizing mutations. Thus distantly related receptors will need to be stabilized by undergoing a complete Ala/Leu scan or, alternatively, other mutagenic strategies could be employed [Citation36].
An interesting question to consider is whether a complete Ala/Leu scan of human β1 and β2 adrenergic receptors would have identified the six thermostabilizing mutations we used here and whether this represents the best combination of mutations to stabilise these receptors. The fact that only one out of the six mutated amino acids in each human receptor could be altered to significantly improve thermostability suggests that the transferability is remarkably good, but it also suggests that further improvements in stability could be obtained. This is further substantiated by the comparisons in affinity of the agonists adrenaline and isoprenaline. The thermostabilized turkey receptor β1AR-m23 bound the agonists noradrenaline and isoprenaline 2470 and 650 times, respectively, more weakly than the wild-type receptor [Citation3]. In contrast, hβ1AR-m23 bound the agonists adrenaline and isoprenaline 365 and 175 times, respectively, more weakly than wild-type, and the equivalent figures for hβ2AR-m23 are 200 and four times more weakly, respectively. This indicates that the shift in equilibrium between R and R* is less pronounced in the thermostabilized human receptors than in the thermostabilized turkey receptor, which suggests that the mutations are less optimal for conformational selection and thermostabilization of the human receptors. However, the substantial improvement in thermostability of hβ1AR will allow its purification in a functional state. It remains to be seen whether the improvements in the thermostability or hβ2AR will correlate with improved resolution of the x-ray structure.
Acknowledgements
This work was supported by a joint grant from Pfizer Global Research and Development and from the MRCT Development Gap Fund A853-0031, in addition to core funding from MRC. We would like to thank R. Henderson and G. Schertler for many helpful discussions throughout this work and the preparation of the manuscript.
Declaration of Interest: C.G. Tate is on the scientific advisory board of Heptares Therapeutics Ltd. M.J. Serrano-Vega declares no conflicts of interest.
References
- Lau FW, Nauli S, Zhou Y, Bowie JU. 1999. Changing single side-chains can greatly enhance the resistance of a membrane protein to irreversible inactivation. J Mol Biol 290:559–564.
- Magnani F, Shibata Y, Serrano-Vega MJ, Tate CG. 2008. Co-evolving stability and conformational homogeneity of the human adenosine A2a receptor. Proc Natl Acad Sci USA 105:10744–10749.
- Serrano-Vega MJ, Magnani F, Shibata Y, Tate CG. 2008. Conformational thermostabilization of the beta1-adrenergic receptor in a detergent-resistant form. Proc Natl Acad Sci USA 105:877–882.
- Shibata Y, White JF, Magnani F, Serrano-Vega MJ, Aloia AA, Grisshammer R, Tate CG. 2009. Thermostabilisation of the neurotensin receptor NTS1. J Mol Biol 390:262–277.
- Zhou Y, Bowie JU. 2000. Building a thermostable membrane protein. J Biol Chem 275:6975–6979.
- Gohon Y, Dahmane T, Ruigrok RW, Schuck P, Charvolin D, Rappaport F, Timmins P, Engelman DM, Tribet C, Popot JL, Ebel C. 2008. Bacteriorhodopsin/amphipol complexes: Structural and functional properties. Biophys J 94:3523–3537.
- Picard M, Dahmane T, Garrigos M, Gauron C, Giusti F, le Maire M, Popot JL, Champeil P. 2006. Protective and inhibitory effects of various types of amphipols on the Ca2+-ATPase from sarcoplasmic reticulum: A comparative study. Biochemistry 45:1861–1869.
- McGregor CL, Chen L, Pomroy NC, Hwang P, Go S, Chakrabartty A, Prive GG. 2003. Lipopeptide detergents designed for the structural study of membrane proteins. Nat Biotechnol 21:171–176.
- Yeh JI, Du S, Tortajada A, Paulo J, Zhang S. 2005. Peptergents: Peptide detergents that improve stability and functionality of a membrane protein, glycerol-3-phosphate dehydrogenase. Biochemistry 44:16912–16919.
- Zhao X, Nagai Y, Reeves PJ, Kiley P, Khorana HG, Zhang S. 2006. Designer short peptide surfactants stabilize G protein-coupled receptor bovine rhodopsin. Proc Natl Acad Sci USA 103:17707–17712.
- Polidori A, Presset M, Lebaupain F, Ameduri B, Popot JL, Breyton C, Pucci B. 2006. Fluorinated and hemifluorinated surfactants derived from maltose: Synthesis and application to handling membrane proteins in aqueous solution. Bioorg Med Chem Lett 16:5827–5831.
- Tucker J, Grisshammer R. 1996. Purification of a rat neurotensin receptor expressed in Escherichia coli. Biochem J 317(Pt 3):891–899.
- Jaakola VP, Griffith MT, Hanson MA, Cherezov V, Chien EY, Lane JR, Ijzerman AP, Stevens RC. 2008. The 2.6 angstrom crystal structure of a human A2A adenosine receptor bound to an antagonist. Science 322:1211–1217.
- Fyfe PK, McAuley KE, Roszak AW, Isaacs NW, Cogdell RJ, Jones MR. 2001. Probing the interface between membrane proteins and membrane lipids by X-ray crystallography. Trends Biochem Sci 26:106–112.
- Palsdottir H, Hunte C. 2004. Lipids in membrane protein structures. Biochim Biophys Acta 1666:2–18.
- Guan L, Smirnova IN, Verner G, Nagamori S, Kaback HR. 2006. Manipulating phospholipids for crystallization of a membrane transport protein. Proc Natl Acad Sci USA 103:1723–1726.
- Lemieux MJ, Reithmeier RA, Wang DN. 2002. Importance of detergent and phospholipid in the crystallization of the human erythrocyte anion-exchanger membrane domain. J Struct Biol 137:322–332.
- Weiss HM, Grisshammer R. 2002. Purification and characterization of the human adenosine A(2a) receptor functionally expressed in Escherichia coli. Eur J Biochem 269:82–92.
- Cherezov V, Rosenbaum DM, Hanson MA, Rasmussen SG, Thian FS, Kobilka TS, Choi HJ, Kuhn P, Weis WI, Kobilka BK, Stevens RC. 2007. High-resolution crystal structure of an engineered human beta2-adrenergic G protein-coupled receptor. Science 318:1258–1265.
- Lee SY, Lee A, Chen J, MacKinnon R. 2005. Structure of the KvAP voltage-dependent K+ channel and its dependence on the lipid membrane. Proc Natl Acad Sci USA 102:15441–15446.
- Caffrey M, Cherezov V. 2009. Crystallizing membrane proteins using lipidic mesophases. Nat Protoc 4:706–731.
- Landau EM, Rosenbusch JP. 1996. Lipidic cubic phases: A novel concept for the crystallization of membrane proteins. Proc Natl Acad Sci USA 93:14532–14535.
- Caffrey M. 2008. On the mechanism of membrane protein crystallization in lipidic mesophases. Crystal Growth Design 8:4244–4254.
- Tate CG2009 Practical considerations of membrane protein instability for purification and crystallisation. In: Mus-Veteau I, editor. Heterologous expression of membrane proteins: Methods and protocols. Methods in molecular biology. New York: Humana Press.
- Warne T, Serrano-Vega MJ, Baker JG, Moukhametzianov R, Edwards PC, Henderson R, Leslie AG, Tate CG, Schertler GF. 2008. Structure of a beta1-adrenergic G-protein-coupled receptor. Nature 454:486–491.
- Bowie JU. 2001. Stabilizing membrane proteins. Curr Opin Struct Biol 11:397–402.
- Sarkar CA, Dodevski I, Kenig M, Dudli S, Mohr A, Hermans E, Plückthun A. 2008. Directed evolution of a G protein-coupled receptor for expression, stability, and binding selectivity. Proc Natl Acad Sci USA 105:14808–14813.
- Warne T, Serrano-Vega MJ, Tate CG, Schertler GF. 2009. Development and crystallization of a minimal thermostabilized G protein-coupled receptor. Protein Exp Purif 65:204–213.
- Warne T, Chirnside J, Schertler GF. 2003. Expression and purification of truncated, non-glycosylated turkey beta-adrenergic receptors for crystallization. Biochim Biophys Acta 1610:133–140.
- Kobilka BK. 1995. Amino and carboxyl terminal modifications to facilitate the production and purification of a G protein-coupled receptor. Anal Biochem 231:269–271.
- Rasmussen SG, Choi HJ, Rosenbaum DM, Kobilka TS, Thian FS, Edwards PC, Burghammer M, Ratnala VR, Sanishvili R, Fischetti RF, Schertler GF, Weis WI, Kobilka BK. 2007. Crystal structure of the human beta2 adrenergic G-protein-coupled receptor. Nature 450:383–387.
- Rosenbaum DM, Cherezov V, Hanson MA, Rasmussen SG, Thian FS, Kobilka TS, Choi HJ, Yao XJ, Weis WI, Stevens RC, Kobilka BK. 2007. GPCR engineering yields high-resolution structural insights into beta2-adrenergic receptor function. Science 318:1266–1273.
- Drew D, Newstead S, Sonoda Y, Kim H, von Heijne G, Iwata S. 2008. GFP-based optimization scheme for the overexpression and purification of eukaryotic membrane proteins in Saccharomyces cerevisiae. Nat Protoc 3:784–798.
- Kawate T, Gouaux E. 2006. Fluorescence-detection size-exclusion chromatography for precrystallization screening of integral membrane proteins. Structure 14:673–681.
- Lemieux MJ, Song J, Kim MJ, Huang Y, Villa A, Auer M, Li XD, Wang DN. 2003. Three-dimensional crystallization of the Escherichia coli glycerol-3-phosphate transporter: A member of the major facilitator superfamily. Protein Sci 12:2748–2756.
- Martin A, Damian M, Laguerre M, Parello J, Pucci B, Serre L, Mary S, Marie J, Baneres JL. 2009. Engineering a G protein-coupled receptor for structural studies: Stabilization of the BLT1 receptor ground state. Protein Sci 18:727–734.