Abstract
Isolation of the lipid fraction from biological samples has been a crucial part of countless studies over the last century. This considerable research interest has led to the development of a number of methods for isolating a range of molecular species that fall under the umbrella term “lipid”. Such methods vary in popularity, complexity, specificity and even toxicity. In this review, we explore examples of published methods (1952–2014) for isolating lipids from biological samples and attempt to assess the limits of techniques both from a chemical and biological perspective. We also suggest how a suitable method might be chosen for a novel application.
Introduction
Recent progress in determining the molecular profile of biological samples, sometimes called the “omics” revolution, allows us to characterize biological systems in myriad ways. The range of proteins a given organism synthesises (proteome), all the RNA species produced (transcriptome) and at its broadest, the entire range of small molecule species present in a system (metabolome), can now be determined more accurately, quickly and extensively than techniques allowed ten or twenty years ago.
Recent detailed studies using mass spectrometry (Atilla-Gokcumen et al., Citation2014; Botté et al., Citation2013; Ejsing et al., Citation2009; Guan et al., Citation2010) and nuclear magnetic resonance (Bartz et al., Citation2007; Fernando et al., Citation2011; Furse et al., Citation2013; Kostara et al., Citation2014; Murgia et al., Citation2003) have shown how lipid profiles vary between organisms, cells and organelles. Profiling the lipid fraction (lipidome) is of increasing importance in generating understanding of how biological systems operate. Examples are studies about metabolic activity of healthy (Augert et al., Citation1989, Quehenberger et al., Citation2010) and diseased (Botté et al., Citation2013; Chan et al., Citation2012) cells and tissues, on changes in signalling (Cao et al., Citation2008; Wakelam et al., Citation2007), on physical properties of membranes (Atilla-Gokcumen et al., Citation2014; Hague et al., Citation2013), on age (Cronan, Citation1968, Wang & Cronan, Citation1994) and adaptation (Kulkarni et al., Citation2014, Sévin & Sauer, Citation2014; Zhou et al., Citation2012) of cells, and on the bioactivity of lipids in bacterial infection (Blaho et al., Citation2009; Tam, Citation2013). Lipid profiling studies also have led to the discovery of hitherto unknown lipid species, the role(s) of which are not yet understood (Garrett et al., Citation2009).
The variety of lipid species and the breadth of applications of lipidomics data means that techniques for isolating the lipid fraction are of considerable interest. In this review, we use examples of lipid isolation processes and put them in the context of the samples on which they have been used and which lipid fraction(s) were isolated. First, we discuss some relevant lipid chemistry as this shapes the approaches and methods used to date.
Lipid chemistry
The broadest definition of the word lipid includes species as chemically diverse as fatty alcohols, triglycerides, sterols, phosphatidylcholine, poly-phosphorylated inositides and lipopolysaccharides (). Most lipids comprise a hydrophilic part (head group), a lipophilic portion (tails), and a moiety that connects the two. Head groups may contain saccharide, amino acid and/or short-chain moieties. Phospholipids possess one or more phosphates, typically as phosphate mono- or di-esters. The connecting moiety is often a glycerol residue. Isoforms of lipids arise from a given head group being appended with a variety of aliphatic groups, usually fatty acids (FAs), on the connecting moiety. Lipophilic portions, e.g. diacylglycerides, often comprise more than one type of FA. The existence of lipid isoforms means that a considerable number of molecular species are present in the lipidome at any given moment (Muro et al., Citation2014). Isolates of phospholipids from natural sources therefore typically possess a range of fatty acids that may vary in a number of ways, including chain length and unsaturated bonds, and in most prokaryotes also branching and cyclopropylation.
Figure 1. Relative polarities of biological lipid species, (A) hexadecanol/cetyl alcohol, (B) trioleoyl glycerol, (C) cholesterol, (D) dioleoyl phosphatidylcholine, (E) phosphatidylinositol-3,4,5-tris-phosphate, (F) a generic lipopolysaccharide. The blue and red dashed lines show an approximate delineation between the head group region, the connecting region, and the hydrophobic fraction. The black dotted lines show an approximate delineation between lipophilic and intermediate (left) and intermediate and hydrophilic (right) lipid species.
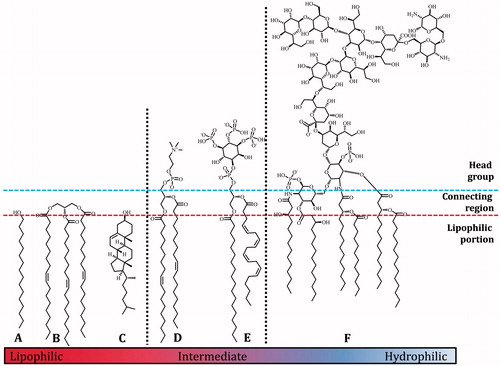
The balance between the size of the head group and the tails of a lipid has an important effect on its solvation properties. Species with a large tail region have relatively low polarity and are practically insoluble in water. They are also characterised as having a high solubility in organic solvents like hexane and diethyl ether and are thus relatively lipophilic. This group includes species such as fatty alcohols (A, ), triglycerides (B) and cholesterol (C) and are often referred to as fats rather than lipids. The lipids with the highest polarity, i.e. the most hydrophilic lipids (, e.g. F) have a head group region that can be considerably larger than its lipophilic part, and for practical purposes can dissolve in water. Lipids with intermediate polarity typically dissolve in mixtures of chloroform and methanol. This middle group includes most phospholipids, e.g. phosphatidylcholine (D) and phosphatidylinositol-3,4,5-tris-phosphate (PIP3, E).
The (lack of) interactions between lipids and solvents can make decisions about handling and isolation of the lipid fraction difficult. A convenient parameter for categorizing lipids may be the simple ratio of the sum of smaller heteroatoms (oxygen and nitrogen) to carbon. For fatty acids and triglycerides this ratio is around 1:7, while membrane lipids such as phosphatidylcholine tend to have a ratio of between 1:5 and 1:3, and lipopolysaccharides have a ratio of around 1:2. However, the validity of using this ratio has not yet been addressed experimentally and there is currently no absolute rule regarding lipid categorization with which to compare it. Indeed, a lipid’s chemistry is influenced by a number of factors and many lipids remain difficult to handle despite the plethora of solvents and solvent mixtures available.
A complicating factor in handling lipids is the variety of functional groups they possess, something that has both biological and chemical consequences. During sample preparation, endogenous lipases can shuffle fatty acids between hydroxyls (Akimoto et al., Citation2003) or remove them entirely (Egmond & de Vlieg, Citation2000; van Kampen et al., Citation2001). Lipid phosphatases (phospholipases) are capable of exchanging head groups (Furse et al., Citation2013) and removing them completely (Goldfine et al., Citation1993).
The rich assortment of functionality in lipids also gives scope for an array of possible forms of chemical degradation and modification. The most common is oxidation of unsaturated lipids by atmospheric oxygen, rancidification. Oxidation is undesirable because it prevents unambiguous identification of the endogenous fatty acid residues and because it can make the desired analyte(s) hydrophilic and thus more difficult to isolate. Acidic pH can effect hydrolysis of fatty acid residues (Schiller et al., Citation2003). When low pH is combined with mildly nucleophilic species such as short-chain alcohols, head group exchange can occur (Garrett et al., Citation2011) (Scheme 1, top). Re-arrangements such as phosphate and fatty acid migration can occur at high or low pH (Kuksis, Citation2003; Lubary et al., Citation2011), and thus are also a concern in the synthetic strategy behind the preparation of lipids (Furse et al., Citation2015; Gaffney & Reese, Citation2001; Panchal & Gaffney, Citation2009). Furthermore, there is some evidence that when solutions of chloroform and methanol are exposed to the ultra-violet light produced by strip lighting commonly used in laboratories, acylating agents may form. These can then react with nucleophilic functional groups such as the primary amine in PE (Cone et al., Citation1982, Garrett et al., Citation2011) (Scheme 1, bottom), meaning that both quantification of this lipid and identification of more minor isoforms may be compromised. Notably, several degradation reactions that are plausible on thermodynamic grounds have not yet been investigated in the context of handling lipids. For example, unsaturated fatty acids may undergo an addition reaction in the presence of hydrogen chloride that originates from the degradation of chloroform, giving lipids with chlorine substituent(s). Such reaction products may be identified by mass spectrometry, but it may become confusing in the context of profiling chlorinated lipids generated in vivo (see review Spickett, Citation2007). Like rancidification, it makes structural determination ambiguous. For these reasons, careful sample handling is an important requirement for reliable lipid profiling.
Scheme 1. Two types of chemical modification that may be observed in handling lipids (Garrett et al., Citation2011). Upper, acid-mediated electrophilic substitution of the phosphate di-ester that produces non-endogenous lipids such as phosphatidylmethanol. Lower, the degradation of chloroform on storage can produce phosgene that in turn may lead to carbamoylation of PE.
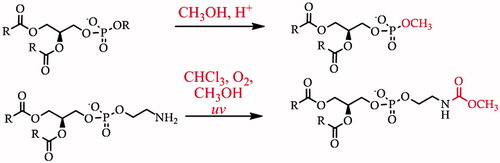
In the following sections we review the isolation methods that have been used for different lipid species, broadly defined by polarity. We will begin with isolation of the least polar species, fats.
Isolation of lipophilic species
The lipophilic fraction of a biological system comprises all fats and oils: triglycerides, fatty acids and alcohols, sterols, waxes and fat-soluble vitamins (carotenoids, tocopherols, etc.). One of the major research interests in this fraction is in fatty acids, for example in the milk of different mammals (Blasi et al., Citation2008; Ruiz-Sala et al., Citation1996) or in the metabolic response to changes in diet (DePeters et al., Citation2001). Profiling of the lipophilic fraction is also an important component of the analysis of lipid droplets (see section Isolating the different lipid fractions of complex samples).
The choice of isolation method may be informed by the requirements of the desired analyses. The traditional technique for identifying FAs is gas chromatography (GC), which has provided much data about the FAs from a variety of sources. Sample preparation requires separation of the FAs from the other material, followed by preparation of the methyl esters of the fatty acid, FAMEs (Caglayan et al., Citation2014; Dörr et al., Citation2014; Ichihara & Fukubayashi, Citation2010; Lipp, Citation1996).
Mass spectrometry (MS) has seen increased use in the last decade for identifying FAs, partly because it is convenient for high-throughput processing of samples and data. MS has another particular advantage over GC in that it can be used to identify which lipids comprise which FAs (Ejsing et al., Citation2009; Furse et al., Citation2013; Quehenberger et al., Citation2010). We discuss a variety of approaches here so that an appropriate procedure might be developed according to the type, number and format of sample(s), the data required, and the spectroscopic techniques available.
All lipid isolation methods require removal of non-lipidic material present in a biological sample. Some samples present a particular practical difficulty in isolating the triglyceride fraction, for example where there is a significant fibre fraction. In this case a continuous extraction with petroleum ether at elevated temperature (Soxhlet apparatus) can be used, as reported for ground seed material (Kraujalis et al., Citation2013; Niu et al., Citation2014) and dried fish meal (Xiao et al., Citation2012). After isolation of the triglycerides, their fatty acid composition can be investigated for example by GC (DePeters et al., Citation2001) after treatment of the sample with a methanolic solution of a mineral acid to produce the required fatty acid methyl esters.
In some cases it is possible to deal with removal of extraneous material present and isolation of the FAs in one step. An approach that is non-specific with respect to the lipid source of FAs has been used in the analysis of milk samples (DePeters et al., Citation2001). In this example, milk samples were acidified and heated, resulting in hydrolysis of the ester bonds that attached the FAs to glyceryl or ceramide moieties. The FAs can then be isolated using hexane or a similarly non-polar solvent before preparation of the FAMEs (Caglayan et al., Citation2014; Craig-Schmidt et al., Citation1984; DePeters et al., Citation2001; Dinius et al., Citation1975; Erickson & Dunkley, Citation1964). However, this practice is limited because of its lack of specificity; it also releases fatty acids from phospholipids, while species closely related to FAs (such as fatty alcohols in plasmalogen lipids) may not be isolated, even though they may represent an important part of the aliphatic residues present. Whether or not this method should be used therefore relies upon a clear understanding of which species are under study and to what extent the negative factors mediate the results.
Treatment of a concentrated sample of fatty acids, however they were isolated, with methanol and a mineral acid is a convenient method for preparing FAMEs that can then be profiled readily using GC (Caglayan et al., Citation2014; Dörr et al., Citation2014; Ichihara & Fukubayashi, Citation2010; Lipp, Citation1996). An alternative method for preparing FAMEs is to make the mixed anhydride of the fatty acids in situ with acetyl chloride, before methanolysis (Lepage & Roy, Citation1988). Methods based on mineral acids are desirable because they are the most reliable in driving the esterification reaction to completion. Although basic (high pH) conditions can also be used to prepare methyl esters, they can also lead to the formation of the carboxylate form of the fatty acid, that is not sensitive to nucleophiles and thus is not converted to the ester (details of ester chemistry, see e.g. (Clayden et al., Citation2001).
Triglycerides can also be isolated using mixtures of hexane with other solvents under mild conditions (Hara & Radin, Citation1978). This approach has been used not only for the overall fatty acid profile of samples (Guerra et al., Citation2014; Koçak et al., Citation2011; Moldoveanu & Chang, Citation2011), but also where the stereoisomeric position of the fatty acids on the glyceryl moiety was under study (Brockerhoff, Citation1965; Harp & Hammond, Citation1998; Koçak et al., Citation2011; Lísa & Holčapek, Citation2013). Solutions of hexane have the advantage that only the most hydrophobic small-and medium-sized molecules (and thus all triglycerides found in nature) dissolve in them, and typically, the more hydrophilic species do not.
Alternative solvents to hexane for isolating fats have been devised, including acetone (Deineka et al., Citation2003) and chloroform/methanol mixtures (Blasi et al., Citation2008; Christie & Moore, Citation1970; Wu et al., Citation1997). However, the latter should be used with caution; more polar solvent systems carry the risk of isolating some or all of the amphiphilic fraction (lipids of intermediate polarity, see next section) as well as the lipophilic one, and therefore the fatty acid profile acquired for such samples may include material from amphiphilic lipids as well as triglycerides.
Fortunately, there is little evidence for inadvertent chemical modification in isolating the lipophilic fraction and routine procedures avoid problems associated with microbial lipase activity and rancidification. This relative ease of handling is ascribed to the relatively small number of functional groups present in fats, where lipids typically contain many more. Isolation of such lipids, that includes bilayer-forming lipids, is discussed next.
Isolation of lipids of intermediate polarity
Lipids in this category typically include membrane lipids and thus most phospholipids. Biological studies of the intermediate fraction include research into lipid metabolism (Cao et al., Citation2008; da Silveira dos Santos et al., Citation2014), lipid-based signalling cascades (Wakelam et al., Citation2007) and the spatial arrangement of lipids (Ariotti et al., Citation2014; Yetukuri et al., Citation2010). Physical studies have shown that many lipids show remarkable polymorphism, and that they do not necessarily self-assemble into bilayers when hydrated (Cullis & de Kruijff, Citation1979; Seddon, Citation1990).
The two most widely used methods for isolating this lipid fraction are undoubtedly the Folch method (Folch et al., Citation1957) and the Bligh & Dyer (B&D) method (Bligh & Dyer, Citation1959), with the original publications together now approaching 80 000 citations between them. Both methods are based on mixtures of chloroform, methanol and water. In addition, there are countless examples of a simplified version of this solvent mixture, comprising chloroform/methanol (typically 2:1 or 1:1, v/v) (Ariotti et al., Citation2014; Chakravarthy et al., Citation2009; Kuge et al., Citation2014).
The Folch method consists of homogenizing the sample with a mixture of saline (NaCl (aq.), 0·9% w/v), methanol and chloroform, which is then left to separate into two phases. The lower, chloroform, phase contains most if not all lipids. The original B&D procedure differs in that water is used instead of saline, and that in the initial step an homogenous solution of chloroform, methanol and the water-based sample is produced. Phase separation is induced by the addition of more water and more chloroform (Bligh & Dyer, Citation1959).
Although the solvent ratios and extraction conditions were stipulated carefully in the original reports of these two methods, a considerable number of modifications have since been used. These include variations in the size of the water fraction (Hillery et al., Citation1996; Roose & Smedes, Citation1996), the use of acidified versions of both methods (Garrett et al., Citation2011; Mansbach et al., Citation1975), and the use of phosphate-buffered saline (PBS) instead of water in the B&D method (Garrett et al., Citation2011). These modifications were introduced mainly to facilitate separation of the lipid fraction from extraneous material such as proteins. Other tactics that may be useful for separating solutions include centrifugation of systems comprising both chloroform and water, and cooling to −20 °C in order to freeze the aqueous fraction.
The popularity of the Folch and B&D methods invites the question of how they compare. In a study that tested the two methods on several samples, no difference was detected at lower concentrations of lipid (< 2% lipid, w/w), but in some more concentrated samples the Folch method yielded a larger isolated mass of lipids than did the B&D method (Iverson et al., Citation2001). However, it is not clear how consistent such comparisons are, as there is clear evidence for considerable variation between experimentalists in terms of lipid extraction efficiency. For example, Roose and Smedes describe a study in which B&D isolations were carried out on portions of a nominated sample in several laboratories simultaneously and report that the lipid fraction isolated varied considerably (Roose & Smedes, Citation1996). Since profiling was not performed on any of the lipid fractions isolated, it is not possible to assess the efficiency of recovery with respect to lipid profile. In addition, it is not clear to what extent any degradation or modification may have occurred.
The lack of a fully comprehensive, controlled, examination of the B&D and Folch methods makes assessing their true value difficult. One comparison that can be made is with alternatives that have been developed for specified practical requirement(s). For example, several procedures have been designed to employ solvents that are less toxic than chloroform and methanol (Burton et al., Citation1985; Hara & Radin, Citation1978; Radin, Citation1981; Somersalo et al., Citation1986). Methods in wider use involve mixtures of SDS/ethanol/heptane mixtures (Burton et al., Citation1985) and petroleum ether/methanol mixtures (Somersalo et al., Citation1986).
The Burton method was originally developed in order to determine the ratio of phospholipids and tocopherols, fractionating the two groups in one step (Burton et al., Citation1985). It has since found use in studies of several mammalian tissues (Berger et al., Citation1992; Okabe et al., Citation2004). A relative comparison of the B&D and Burton methods with those using a hexane/ethanol mixture (Katayama et al., Citation1980), showed significant differences depending on the type of tissue used (Cabrini et al., Citation1992). However, no quantitative data of individual phospholipids was included.
Some applications, for example high-throughput systems, require a speedy separation of organic and aqueous phases in order to be experimentally useful. A convenient approach for such purposes may be the use of tertiary-butylmethyl ether (TBME) (Matyash et al., Citation2008), because mixtures partition into two phases more quickly than chloroform-based methods. Additionally, the TBME phase is less dense and thus forms the upper layer, facilitating automation of the process. Several variants of TBME-based system have been used (Gregory et al., Citation2013; Reis et al., Citation2013) indicating that it may be a useful alternative to chloroform/methanol based methods. However, TBME is somewhat less polar than chloroform, and therefore lipids with a higher polarity may not be able to dissolve in it.
Despite the dominance of traditional chloroform/methanol based methods (Bligh & Dyer, Citation1959; Folch et al., Citation1957), unfortunately not all glycero-phospholipids can be isolated using them. Lipids like phosphatidylinositol-3,4,5-tris-phosphate (PIP3) are difficult (Hetherington & Drøbak, Citation1992) or even impossible (König et al., Citation2008) to isolate using the original B&D method. A modified version in which the pH is much lower has been reported (Cho & Boss, Citation1995; König et al., Citation2008). In this method, methanol and chloroform (36% v/v each) are mixed with HCl to give a pH of no more than 0·4 (2·4 M, 18% v/v) in a uniphasic system. This protonates the phosphate mono- and di-esters and thus masks the anionic charge they carry in the region of physiological pH, making them more soluble in organic solvents. Although acidification does offer greater solubility in organic solvents, it comes with risk. Protonation of phosphates increases their electrophilicity, which increases the likelihood of structural re-arrangement in the presence of one or more nucleophiles (Kuksis, Citation2003). For purposes other than straightforward analysis only, this has led to a reliance on alternative sources of inositides that avoid any kind of lipid isolation from natural sources (Furse et al., Citation2015; Gaffney & Reese, Citation1998, Citation2001).
This difficulty in isolating such lipids raises the question of whether they could have been missed even in samples where they may have been relatively plentiful. One approach that may facilitate isolating such species is sequential isolation. This tactic for isolating lipid fractions that contain species of differing chemical properties is discussed next.
Isolating the different lipid fractions of complex samples
An emerging part of lipidomics research is the desire for profiling two or more lipid fractions from the same biological sample. For example, profiling both the fats and the membrane lipids has been crucial in understanding the metabolic activity of lipid droplets (Arrese et al., Citation2006) and lipoproteins (Sreckovic et al., Citation2014), the biophysics of oil droplets (Furse et al., Citation2013) and lipid metabolism (Ejsing et al., Citation2009).
It is often useful to separate the lipophilic and intermediate fractions of complex samples before profiling. In plant oil droplets, for example, the triglyceride fraction is about three orders of magnitude larger than the phospholipid one, making it difficult to profile both fractions reliably where separation is not used. A study in which the focus was the oil droplets of Helianthus annuus describes the use of hexane to isolate the lipophilic fraction, before using a mixture of organic solvents (chloroform, ethanol and triethylamine) to isolate the phospholipids (Furse et al., Citation2013). Notably, isolation conditions protonate a portion of the triethylamine, giving the triethylammonium cation. This cation is lipophilic, and so when associated with an anionic lipid species, it serves to increase the lipids' solubility in organic solvents such as chloroform in a similar way to the tetrabutylammonium ion (Pettitt et al., Citation2006). Triethylamine has the advantage that it also suppresses lipid modification caused by the HCl released during chloroform degradation. Thus, the use of this solvent system on de-fatted samples enables concentrated samples of the phospholipid fraction to be produced, facilitating the profiling of this fraction. The ability of the triethylammonium ion to mask the anionic charge of phosphate mono- and di-esters provides a possible alternative to using strong acid for isolating lipids with several anionic charges, such as inositides (see previous section).
A recent study that was focussed on the yeast lipid fraction made use of two chloroform/methanol mixtures with different solvent ratios (Ejsing et al., Citation2009) in order to divide the phospholipids into two fractions. PCs, PEs and PGs were isolated with the fats and sterols in the less polar solvent mixture. PIs, PSs, PAs, CLs and inositol ceramides were isolated together in the more polar solvent mixture. This enabled optimized MS conditions to be used for a narrowed range of target species and led to detailed profiling of the system. The lipid profile of individual organelles from yeast cells is also a research interest, which can be accomplished by careful fractionation of organelles (Schneiter et al., Citation1999). However it is not clear whether in this case a single solvent system is sufficient to isolate all lipid species.
A tactic that may expand the range of lipids isolated for global profiling (sometimes known as “shotgun lipidomics”) is to use two different solvent mixtures and combine the lipid-containing solutions in advance of profiling. Examples of solvent systems include chloroform/methanol solvent mixtures followed by aqueous butanol (Bodennec et al., Citation2003; Macmurray & Morrison, Citation1970; Ohnishi et al., Citation1990). It is not clear what the difference in fractionation is between these two solvent systems, i.e. whether the butanol-based system isolates more polar lipids only, or whether different sorts of lipid are measured. Either way, solvent systems comprising butanol have been used for lipid isolation of a very well defined more polar target, lyso-phosphatidic acid (LPA). In this work, a mixture of butanol and water-saturated butanol (2:1, v/v) were used to extract the lipid of interest (Baker et al., Citation2001). This was used to uncover a gender difference in the concentration of plasma LPA. Notably, an increase in the quantity of LPA was isolated from samples that were stored in a butanol/aqueous solvent mixture for longer periods. However, it is not clear whether the latter increase was the result of a more thorough extraction, or chemical or enzymatic hydrolysis of PA to LPA. More recent work shows that butanol mixed with methanol, followed by a less polar heptane/ethyl acetate mixture, provides a means for automating lipid isolation for analytical work; however, this was developed for fats and less hydrophilic charged lipids (Löfgren et al., Citation2012).
Sequential fractionation is one of the more sophisticated approaches yet developed for isolating the lipid fraction from biological samples. There is clearly more scope for tuning solvent systems for tissues as well as for groups of lipids. It seems likely that this approach will develop further, as the need for prompt and even automatic lipid profiling of an increasing number of lipid species becomes greater. In principle, the separation of two fractions of lipids from a given sample may also include isolation of the most hydrophilic lipid species. The latter present their own difficulties as a result of their chemistry, and are discussed in the next section.
Isolation of hydrophilic species
This category comprises the most polar lipid species, e.g. cerebrosides (Tan & Chen, Citation2003), lyso-lipids and lipids that contain several sugar residues (e.g. LPS, [F], ) and/or phosphates (Christie, Citation1993; Guan et al., Citation2010). These types of lipid can be difficult to isolate as some of their physical properties can be similar to extraneous material such as oligosaccharides.
However, various solvent-based approaches have been used. Several studies that focussed on phosphorylated sphingolipids in yeast used a complicated mixture of organic solvents at high pH [water/ethanol/diethyl ether/pyridine (Angus & Lester, Citation1972), sometimes also with ammonium hydroxide (Guan et al., Citation2010; Hanson & Lester, Citation1980; Jesch et al., Citation2010)], but a much simplified version of this solvent system (pyridine/water) has also been used successfully (Angus & Lester, Citation1972; Steiner et al., Citation1969). Mixtures of butanol and pyridine acetate at low pH (4·2) are effective in extracting Lipid II, which may then be observed chromatographically (Brötz et al., Citation1998; Breukink et al., Citation2003).
Isolating LPS from Gram-negative bacteria without proteinaceous material can be a particular problem, as these lipids are often soluble in water. Traditional methods such as hot phenol extractions (Hickman & Ashwell, Citation1966; Keleti et al., Citation1979; Mikheyskaya et al., Citation1977; Westphal et al., Citation1952) have largely been replaced by a milder, commercially-available mixture of guanidinium thiocyanate and phenol (“TRIzol” or “Tri-Reagent”) (Yi & Hackett, Citation2000), originally developed for isolating ribo-nucleic acids (Chomczynski & Sacchi, Citation1987). This reagent system has proved useful for isolating LPS and Lipid A from small samples. The solvent mixture is agitated with lyophilised bacterial cells and the system is partitioned by the addition of chloroform. The two phases are separated and the chloroform solution washed with water. The TRIzol and water fractions (the solutions containing hydrophilic lipids) are combined to give the hydrophilic lipid fraction. The original paper states that there is no need for cell disruption as this is achieved chemically by the guanidinium thiocyanate. However, it is not clear whether lipid modification occurs under these conditions, or under those of milder phenol-free alternatives to this procedure (Pieretti et al., Citation2014).
It seems clear that the hydrophilic lipids may be the most difficult fraction to isolate, and that methods for isolating this fraction are the least well-developed or tested when compared to those of lipophilic and intermediate species. It is therefore appealing to speculate that the greatest wealth of lipids yet to be discovered lies in this category.
Choosing a method
In order to isolate the lipids from a biological sample, the whole process, from the live cells to the interpreted data, should be considered. We suggest that planning the approach used requires three steps, as described in . The first step is a consideration of the sample form and size. A sample of concentrated, purified erythrocytes of a volume of 0.1 mL presents entirely different practical considerations than for example 1 kg of eucalyptus leaves. Second, is the lipid species of interest. Profiling the fatty acid residues present in the phospholipids of a bacterial membrane requires a quite different procedure to that for isolating the Lipid II. The third consideration is what the fate of the lipid fraction will be, once isolated. If it is for analysis, a consideration of which forms of spectroscopy or chromatography is required, as well as what sample size is required for them. Studies that use lipid(s) as a raw material, or physical characterization of lipids, typically require much more material than analytical techniques.
Table I. Planning steps and considerations for selecting a lipid extraction protocol.
It should also be noted that these parts of the process influence one another. If the species of interest is one that may easily be modified by endogenous lipases during isolation (Furse et al., Citation2013; Furse & Killian, Citation2013), the handling required in step one must be adapted accordingly. Equally, if a lipid isolate is required for purposes such as a physical study of the behaviour of a mixture of naturally-occurring membrane lipids, a procedure that isolates the lipid fraction in as comprehensive a way as possible must be selected. This may require a different approach to that for a project focussed on quantifying a single-species target such as LPA (Baker et al., Citation2001).
In order to optimize such a process for isolating the lipid fraction of interest, a certain amount of trial-and-error work is inevitable. This can be achieved by identifying the group into which the target lipid falls (lipophilic, intermediate, hydrophilic) and trying several of the methods mentioned above. An alternative approach, where little or no information on individual species is required, or for entirely novel samples, may be to separate the lipid fractions. For example, the first step may be to wash with hexane to isolate the most lipophilic species, then with chloroform/methanol to dissolve the lipids of intermediate polarity, and finally with TRIzol to fractionate the most polar species. The results of profiling of these fractions can be used to inform and optimize isolation of the species of interest.
Despite the probable necessity of optimizing an existing method for novel applications, sufficient work has now been done to give a good estimate of what may be required. As a result, current research interest in lipid isolation is on data acquisition and not the development of novel isolation methods.
Concluding remarks and perspectives for development in the future
Whatever the approach for isolating lipids used, a significant problem is that the available information from studies that compare methods is relative rather than absolute. That is to say, there has been no thorough investigation in which a known set of lipids has been used to test the efficacy and suitability of even well-known isolation procedures against one another. What is lacking therefore is a rigorous chemical test of the known methods. This may not be far away; it is conceivable that the use of internal standards for quantification in mass spectrometry studies (Ejsing et al., Citation2009; Schneiter et al., Citation1999) might well be adapted to investigate this.
This means that at present, many decisions about which method of isolation to use are based on estimates of the similarity of samples and the convenience of method(s) available. Typically, these are based on the given form of data acquisition (mass spectrometry, NMR) or other application, desired sample size and number of samples (comparative individuals, sample set, high through-put). The best available data in this area is probably in the determination of fatty acids and triglycerides as the methods for isolation are the best developed.
Despite the limits described, lipid species are still being discovered. As more novel lipid species are identified, our understanding of the chemistry of such species improves and the scope for sensitive handling of lipids increases. This persistent approach facilitated the isolation of poly-phosphorylated inositides, leading to the discovery of their role as signalling lipids in the 1980s (Berridge & Irvine, Citation1984). The progress of discovery in lipidology continues with the recent report of fatty acid esters of hydroxyl fatty acids that have an anti-diabetes and anti-inflammatory effect in mice and humans (Yore et al., Citation2014). Further work is required to establish whether the use of surfactants, a key part of an established isolation system (Burton et al., Citation1985), may yield lipids as yet undiscovered. It is also worth investigating to what extent modification caused by some solvents, lipolytic activity and hydrolysis of phosphate esters can be overcome or avoided by careful use of alternatives, doping and/or inhibitors, in order to ensure that the observed lipid fraction is a true reflection of the system in vivo.
Abbreviations | ||
CL | = | cardiolipin |
GC | = | Gas chromatography |
HRMS | = | High resolution mass spectrometry |
MS | = | Mass spectrometry |
MS/MS | = | tandem mass spectrometry |
NMR | = | nuclear magnetic resonance |
PA | = | phosphatidic acid |
PC | = | phosphatidylcholine |
PE | = | phosphatidylethanolamine |
PG | = | phosphatidylglycerol |
PI | = | phosphatidylinositol |
PIP2 | = | phosphatidylinositol-4,5-bis-phosphate |
PIP3 | = | phosphatidylinositol-3,4,5-tris-phosphate |
PS | = | phosphatidylserine |
TLC | = | thin layer chromatography. |
Acknowledgements
The authors would like to thank Dr Toon de Kroon for his helpful comments on the manuscript and the research programme of the bio-based ecologically balanced sustainable industrial chemistry (BE-BASIC) project for funding.
Declaration of interest
The authors report no conflicts of interest. The authors alone are responsible for the content and writing of the paper.
References
- Akimoto M, Izawa M, Hoshino K, Abe K-I, Takahashi H. 2003. Lipase-catalyzed interesterification of soybean oil with an ω-3 polyunsaturated fatty acid concentrate prepared from sardine oil. Appl Biochem Biotechnol 104:105–118
- Angus WW, Lester RL. 1972. Turnover of inositol and phosphorus containing lipids in Saccharomyces cerevisiae; extracellular accumulation of glycerophosphorylinositol derived from phosphatidylinositol. Arch Biochem Biophys 151:483–495
- Ariotti N, Fernández-Rojo MA, Zhou Y, Hill MM, Rodkey TL, Inder KL, et al. 2014. Caveolae regulate the nanoscale organization of the plasma membrane to remotely control Ras signaling. J Cell Biol 204:777–792
- Arrese EL, Patel RT, Soulages JL. 2006. The main triglyceride-lipase from the insect fat body is an active phospholipase A1: Identification and characterization. J Lipid Res 47:2656–2667
- Atilla-Gokcumen GE, Muro E, Relat-Goberna J, Sasse S, Bedigian A, Coughlin ML, et al. 2014. Dividing cells regulate their lipid composition and localization. Cell 156:428--439
- Augert G, Blackmore PF, Exton JH. 1989. Changes in the concentration and fatty acid composition of phosphoinositides induced by hormones in hepatocytes. J Biol Chem 264:2574–2580
- Baker DL, Desiderio DM, Miller DD, Tolley B, Tigyi GJ. 2001. Direct quantitative analysis of lysophosphatidic acid molecular species by stable isotope dilution electrospray ionization liquid chromatography-mass spectrometry. Analyt Biochem 292:287–295
- Bartz R, Li W-H, Venables B, Zehmer JK, Roth MR, Welti R, et al. 2007. Lipidomics reveals that adiposomes store ether lipids and mediate phospholipid traffic. J Lipid Res 48:837–847
- Berger A, Gershwin ME, German JB. 1992. Effects of various dietary fats on cardiolipin acyl composition during ontogeny of mice. Lipids 27:605–612
- Berridge MJ, Irvine RF. 1984. Inositol trisphosphate, a novel second messenger in cellular signal transduction. Nature 312:315–321
- Blaho VA, Buczynski MW, Brown CR, Dennis EA. 2009. Lipidomic analysis of dynamic eicosanoid responses during the induction and resolution of Lyme arthritis. J Biol Chem 284:21599–21612
- Blasi F, Montesano D, De Angelis M, Maurizi A, Ventura F, Cossignani L, et al. 2008. Results of stereospecific analysis of triacylglycerol fraction from donkey, cow, ewe, goat and buffalo milk. J Food Composit Anal 21:1–7
- Bligh EG, Dyer WJ. 1959. A rapid method of total lipid extraction and purification. Can J Biochem Physiol 37:911–917
- Bodennec J, Pelled D, Futerman AH. 2003. Aminopropyl solid phase extraction and 2 D TLC of neutral glycosphingolipids and neutral lysoglycosphingolipids. J Lipid Res 44:218–226
- Botté CY, Yamaryo-Botté Y, Rupasinghe TWT, Mullin KA, Macrae JI, Spurck TP, et al. 2013. Atypical lipid composition in the purified relict plastid (apicoplast) of malaria parasites. Proc Natl Acad Sci 110:7506–7511
- Breukink E, van Heusden HE, Vollmerhaus PJ, Swiezewska E, Brunner L, Walker S, et al. 2003. Lipid II is an intrinsic component of the pore induced by nisin in bacterial membranes. J Biol Chem 278:(1989)8–19903
- Brockerhoff H. 1965. Stereospecific analysis of triglycerides: An analysis of human depot fat. Arch Biochem Biophys 110:586–592
- Brötz H, Bierbaum G, Leopold K, Reynolds PE, Sahl H-G. 1998. The lantibiotic mersacidin inhibits peptidoglycan synthesis by targeting lipid II. Antimicrob Agents Chemother 42:154–160
- Burton GW, Webb A, Ingold KU. 1985. A mild, rapid, and efficient method of lipid extraction for use in determining vitamin E/lipid ratios. Lipids 20:29–39
- Cabrini L, Landi L, Stefanelli C, Barzanti V, Anna Maria S. 1992. Extraction of lipids and lipophilic antioxidants from fish tissues: A comparison among different methods. Comparat Biochem Physiol B: Comparat Biochem 101:383–386
- Caglayan O, Cakmak YS, Guler GO, Zengin G, Aktumsek A. 2014. Evaluation of fatty acid compositions of yogurts in Turkey. Asian J Chem 26:4871--4874
- Cao H, Gerhold K, Mayers JR, Wiest MM, Watkins SM, Hotamisligil GS. 2008. Identification of a lipokine, a lipid hormone linking adipose tissue to systemic metabolism. Cell 134:933–944
- Chakravarthy MV, Lodhi IJ, Yin L, Malapaka RRV, Xu HE, Turk J, Semenkovich CF. 2009. Identification of a physiologically relevant endogenous ligand for PPAR α in liver. Cell 138:476–488
- Chan RB, Oliveira TG, Cortes EP, Honig LS, Duff KE, Small SA, et al. 2012. Comparative lipidomic analysis of mouse and human brain with Alzheimer disease. J Biol Chem 287:2678–2688
- Cho MH, Boss WF. 1995. Transmembrane signaling and phosphoinositides (Chapt. 38). In: Galbraith DW, Bohnert HJ, Bourque DP, editors. Methods in cell biology. New York: Academic Press, 543–554
- Chomczynski P, Sacchi N. 1987. Single-step method of RNA isolation by acid guanidinium thiocyanate-phenol-chloroform extraction. Analyt Biochem 162:156–159
- Christie WW. 1993. Preparation of lipid extracts from tissues. In: Christie WW, editor. Advances in lipid methodology. Bridgwater, UK: Oily Press Ltd., 195–213
- Christie WW, Moore JH. 1970. The structure of egg yolk triglycerides. Biochim Biophys Acta (BBA) – Lipids Lipid Metabol 218:83–88
- Clayden J, Greeves N, Warren S, Wothers P. 2001. Organic chemistry. Oxford, UK: Oxford University Press
- Cone EJ, Buchwald WF, Darwin WD. 1982. Analytical controls in drug metabolic studies. II. Artifact formation during chloroform extraction of drugs and metabolites with amine substituents. Drug Metabol Disposition 10:561–567
- Craig-Schmidt MC, Weete JD, Faircloth SA, Wickwire MA, Livant EJ. 1984. The effect of hydrogenated fat in the diet of nursing mothers on lipid composition and prostaglandin content of human milk. Am J Clin Nutrit 39:778–786
- Cronan JE. 1968. Phospholipid alterations during growth of Escherichia coli. J Bacteriol 95:2054–2061
- Cullis PR, de Kruijff B. 1979. Lipid polymorphism and the functional roles of lipids in biological membranes. Biochim Biophys Acta (BBA) – Rev Biomembranes 559:399–420
- da Silveira dos Santos AX, Riezman I, Aguilera-Romero M-A, David F, Piccolis M, Loewith R, et al. 2014. Systematic lipidomic analysis of yeast protein kinase and phosphatase mutants reveals novel insights into regulation of lipid homeostasis. Mol Biol Cell 25:3234–3246
- Deineka VI, Maslov AN, Borzenko ON, Sirotin AA, Deineka LA. 2003. Change of triglyceride composition of cucurbitaceae and taraxacum officinale seed oil during ripening. Chem Nat Compounds 39:319–321
- DePeters EJ, German JB, Taylor SJ, Essex ST, Perez-Monti H. 2001. Fatty acid and triglyceride composition of milk fat from lactating holstein cows in response to supplemental canola oil. J Dairy Sci 84:929–936
- Dinius DA, Edmondson LF, Kimoto W, Oltjen RR. 1975. Growth, blood parameters and tissue lipids of finishing cattle fed a formaldehyde treated casein-safflower oil complex. J Anim Sci 40:58–365
- Dörr JM, Koorengevel MC, Schäfer M, Prokofyev AV, Scheidelaar S, van der Cruijsen EAW, et al. 2014. Detergent-free isolation, characterization, and functional reconstitution of a tetrameric K+ channel: The power of native nanodiscs. Proc Natl Acad Sci 111:18607–18612
- Egmond MR, de Vlieg J. 2000. Fusarium solani pisi cutinase. Biochimie 82:1015–1021
- Ejsing CS, Sampaio JL, Surendranath V, Duchoslav E, Ekroos K, Klemm RW, et al. 2009. Global analysis of the yeast lipidome by quantitative shotgun mass spectrometry. Proc Nat Acad Sci 106:2136–2141
- Erickson DR, Dunkley WL. 1964. Spectrophotometric determination of tocopherol in milk and milk lipides. Analyt Chem 36:1055–1058
- Fernando H, Bhopale KK, Kondraganti S, Kaphalia BS, Shakeel Ansari GA. 2011. Lipidomic changes in rat liver after long-term exposure to ethanol. Toxicol Appl Pharmacol 255:127–137
- Folch J, Lees M, Stanley GHS. 1957. A simple method for the isolation and purification of total lipides from animal tissues. J Biol Chem 226:497–509
- Furse S, Killian JA. 2013. Lipase activity in lipidomics – a hidden problem? Mol Memb Biol 30:347–349
- Furse S, Liddell S, Ortori CA, Williams H, Neylon DC, Scott DJ, et al. 2013. The lipidome and proteome of oil bodies from Helianthus annuus (common sunflower). J Chem Biol 6:63–76
- Furse S, Mak L, Tate EW, Templer RH, Ces O, Woscholski R, Gaffney PRJ. 2015. Synthesis of unsaturated phosphatidylinositol 4-phosphates and the effects of substrate unsaturation on SopB phosphatase activity. Organic Biomol Chem 13:2001--2011
- Gaffney PRJ, Reese CB. 1998. Synthesis of 1-O-stearoyl-2-O-arachidonoyl-sn-glycer-3-yl-D-myo-inositol 3,4,5-triphosphate and its stereoisomers (vol 7, pg 3171, (1997)). Bioorgan Med Chem Lett 8:202–202
- Gaffney PRJ, Reese CB. 2001. Synthesis of naturally occurring phosphatidylinositol 3,4,5-trisphosphate PtdIns(3,4,5)P-3 and its diastereoisomers. J Chem Soc-Perkin Transact 1:192–205
- Garrett TA, Raetz CRH, Richardson T, Kordestani R, Son JD, Rose RL. 2009. Identification of phosphatidylserylglutamate: A novel minor lipid in Escherichia coli. J Lipid Res 50:1589–1599
- Garrett TA, Raetz CRH, Son JD, Richardson TD, Bartling C, Guan Z. 2011. Non-enzymatically derived minor lipids found in Escherichia coli lipid extracts. Biochim Biophys Acta 1811:827–837
- Goldfine H, Johnston NC, Knob C. 1993. Nonspecific phospholipase C of Listeria monocytogenes: Activity on phospholipids in Triton X-100-mixed micelles and in biological membranes. J Bacteriol 175:4298–4306
- Gregory KE, Bird SS, Gross VS, Marur VR, Lazarev AV, Walker WA, Kristal BS. 2013. Method development for fecal lipidomics profiling. Analyt Chem 85:1114–1123
- Guan XL, Riezman I, Wenk MR, Riezman H. 2010. Yeast lipid analysis and quantification by mass spectrometry, chapt. 15. In: Weissman J, Guthrie C, Fink GR, editors. Methods in enzymology. New York: Academic Press, 369–391
- Guerra E, Gori A, Cevoli C, Losi G, Caboni MF. 2014. Lipid fraction of creams collected in the Parmigiano-Reggiano cheese production area in response to extruded linseed supplementation of dairy cows’ diets: GC-FID and FT-MIR evaluation. Int J Dairy Technol 67:510–520
- Hague CV, Postle AD, Attard GS, Dymond MK. 2013. Cell cycle dependent changes in membrane stored curvature elastic energy: Evidence from lipidomic studies. Faraday Discussions 161:481–497
- Hanson BA, Lester RL. 1980. The extraction of inositol-containing phospholipids and phosphatidylcholine from Saccharomyces cerevisiae and Neurospora crassa. J Lipid Res 21:309–315
- Hara A, Radin NS. 1978. Lipid extraction of tissues with a low-toxicity solvent. Analyt Biochem 90:420–426
- Harp T, Hammond E. 1998. Stereospecific analysis of soybean triacylglycerols. Lipids 33:209–216
- Hetherington AM, Drøbak BK. 1992. Inositol-containing lipids in higher plants. Progress Lipid Res 31:53–63
- Hickman J, Ashwell G. 1966. Isolation of a bacterial lipopolysaccharide from Xanthomonas campestris containing 3-acetamido-3,6-dideoxy-d-galactose and d-Rhamnose. J Biol Chem 241:1424–1428
- Hillery C, Du M, Montgomery R, Scott J. 1996. Increased adhesion of erythrocytes to components of the extracellular matrix: Isolation and characterization of a red blood cell lipid that binds thrombospondin and laminin. Blood 87:4879–4886
- Ichihara KI, Fukubayashi Y. 2010. Preparation of fatty acid methyl esters for gas-liquid chromatography. J Lipid Res 51:635–640
- Iverson S, Lang SC, Cooper M. 2001. Comparison of the bligh and dyer and folch methods for total lipid determination in a broad range of marine tissue. Lipids 36:1283–1287
- Jesch SA, Gaspar ML, Stefan CJ, Aregullin MA, Henry SA. 2010. Interruption of inositol sphingolipid synthesis triggers Stt4p-dependent protein kinase C signaling. J Biol Chem 285:41947–41960
- Katayama K, Takada M, Yuzuriha T, Abe K, Ikenoya S. 1980. Simultaneous determination of ubiquinone-10 and ubiquinol-10 in tissues and mitochondria by high performance liquid chromatography. Biochem Biophys Res Commun 95:971–977
- Keleti G, Sykora JL, Lippy EC, Shapiro MA. 1979. Composition and biological properties of lipopolysaccharides isolated from Schizothrix calcicola (Ag.) Gomont (Cyanobacteria). Appl Environ Microbiol 38:471–477
- Koçak D, Keskin H, Fadıloğlu S, Kowalski B, Göğöş F. 2011. Characterization of terebinth fruit oil and optimization of acidolysis reaction with caprylic and stearic acids. J Am Oil Chem Soc 88:1531–1538
- König S, Hoffmann M, Mosblech A, Heilmann I. 2008. Determination of content and fatty acid composition of unlabeled phosphoinositide species by thin-layer chromatography and gas chromatography. Analyt Biochem 378:197–201
- Kostara CE, Papathanasiou A, Psychogios N, Cung MT, Elisaf MS, Goudevenos J, Bairaktari ET. 2014. NMR-based lipidomic analysis of blood lipoproteins differentiates the progression of coronary heart disease. J Proteome Res 13:2585–2598
- Kraujalis P, Venskutonis PR, Pukalskas A, Kazernavičiūtė R. 2013. Accelerated solvent extraction of lipids from Amaranthus spp. seeds and characterization of their composition. LWT -- Food Sci Technol 54:528–534
- Kuge H, Akahori K, Yagyu KI, Honke K. 2014. Functional compartmentalization of the plasma membrane of neurons by a unique acyl chain composition of phospholipids. J Biol Chem 289:26783–26793
- Kuksis A. 2003. Inositol phospholipid metabolism and phosphatidyl inositol kinases. In: van der Vliet PC, Pillai S, editors. Laboratory techniques in biochemistry and molecular biology. Amsterdam: Elsevier, 1–971
- Kulkarni HM, Swamy CVB, Jagannadham MV. 2014. Molecular characterization and functional analysis of outer membrane vesicles from the antarctic bacterium Pseudomonas syringae suggest a possible response to environmental conditions. J Proteome Res 13:1345–1358
- Lepage G, Roy CC. 1988. Specific methylation of plasma nonesterified fatty acids in a one-step reaction. J Lipid Res 29:227–235
- Lipp M. 1996. Determination of the adulteration of butter fat by its triglyceride composition obtained by GC. A comparison of the suitability of PLS and neural networks. Food Chem 55:389–395
- Lísa M, Holčapek M. 2013. Characterization of triacylglycerol enantiomers using chiral HPLC/APCI-MS and synthesis of enantiomeric triacylglycerols. Analyt Chem 85:1852–1859
- Löfgren L, Ståhlman M, Forsberg G-B, Saarinen S, Nilsson R, Hansson GI. 2012. The BUME method: A novel automated chloroform-free 96-well total lipid extraction method for blood plasma. J Lipid Res 53:1690–1700
- Lubary M, Hofland GW, ter Horst JH. 2011. A process for the production of a diacylglycerol-based milk fat analogue. Eur J Lipid Sci Technol 113:459–468
- Macmurray TA, Morrison WR. 1970. Composition of wheat-flour lipids. J Sci Food Agricul 21:520–528
- Mansbach CM 2nd, Cohen RS, Leff PB. 1975. Isolation and properties of the mixed lipid micelles present in intestinal content during fat digestion in man. J Clin Invest 56:781–791
- Matyash V, Liebisch G, Kurzchalia TV, Shevchenko A, Schwudke D. 2008. Lipid extraction by methyl-tert-butyl ether for high-throughput lipidomics. J Lipid Res 49:1137–1146
- Mikheyskaya LV, Ovodova RG, Ovodov YS. 1977. Isolation and characterization of lipopolysaccharides from cell walls of blue-green algae of the genus Phormidium. J Bacteriol 130:1–3
- Moldoveanu SC, Chang Y. 2011. Dual analysis of triglycerides from certain common lipids and seed extracts. J Agricult Food Chem 59:2137–2147
- Murgia S, Mele S, Monduzzi M. 2003. Quantitative characterization of phospholipids in milk fat via P-31 NMR using a monophasic solvent mixture. Lipids 38:585–591
- Muro E, Atilla-Gokcumen GE, Eggert US. 2014. Lipids in cell biology: How can we understand them better? Mol Biol Cell 25:1819–1823
- Niu L, Li J, Chen M-S, Xu Z-F. 2014. Determination of oil contents in Sacha inchi (Plukenetia volubilis) seeds at different developmental stages by two methods: Soxhlet extraction and time-domain nuclear magnetic resonance. Industrial Crops Prod 56:187–190
- Ohnishi M, Hirose S, Kawaguchi M, Ito S, Fujino Y. 1990. Chemical composition of lipids, especially triacylglycerol, in grape seeds. Agricult Biol Chem 54:1035–1042
- Okabe M, Sakai M, Maeda N, Miyamoto Y, Tachibana H, Yamada K. 2004. Effects of dietary flaxseeds on lipid metabolism of Sprague-Dawley rats. J Japan Soc Food Sci Technol – Nippon Shokuhin Kagaku Kogaku Kaishi 51:352–357
- Panchal N, Gaffney PRJ. 2009. Synthesis of unsaturated phosphatidylinositol 4,5-bisphosphate and analogues. Organ Biomol Chem 7:4832–4841
- Pettitt TR, Dove SK, Lubben A, Calaminus SDJ, Wakelam MJO. 2006. Analysis of intact phosphoinositides in biological samples. J Lipid Res 47:1588–1596
- Pieretti G, Cipolletti M, D’Alonzo D, Alfano A, Cimini D, Cammarota M, et al. 2014. A combined fermentative-chemical approach for the scalable production of pure E. coli monophosphoryl lipid A. Appl Microbiol Biotechnol 98:7781–7791
- Quehenberger O, Armando AM, Brown AH, Milne SB, Myers DS, Merrill AH, et al. 2010. Lipidomics reveals a remarkable diversity of lipids in human plasma. J Lipid Res 51:3299–3305
- Radin NS. 1981. Extraction of tissue lipids with a solvent of low toxicity. In: John ML, editor. Methods in enzymology. Salt Lake City, UT: Academic Press, 5–7
- Reis A, Rudnitskaya A, Blackburn GJ, Fauzi NM, Pitt AR, Spickett CM. 2013. A comparison of five lipid extraction solvent systems for lipidomic studies of human LDL. J Lipid Res 54:1812–1824
- Roose P, Smedes F. 1996. Evaluation of the results of the QUASIMEME lipid intercomparison: The Bligh and Dyer total lipid extraction method. Marine Pollut Bull 32:674–680
- Ruiz-Sala P, Hierro MTG, Martínez-Castro I, Santa-María G. 1996. Triglyceride composition of ewe, cow, and goat milk fat. J Am Oil Chem Soc 73:283–293
- Schiller J, Müller K, Süß R, Arnhold J, Gey C, Herrmann A, et al. 2003. Analysis of the lipid composition of bull spermatozoa by MALDI-TOF mass spectrometry – a cautionary note. Chem Phys Lipids 126:85–94
- Schneiter R, Brügger B, Sandhoff R, Zellnig G, Leber A, Lampl M, et al. 1999. Electrospray ionization tandem mass spectrometry (ESI-MS/MS) analysis of the lipid molecular species composition of yeast subcellular membranes reveals acyl chain-based sorting/remodeling of distinct molecular species en route to the plasma membrane. J Cell Biol 146:741–754
- Seddon JM. 1990. Structure of the inverted hexagonal (Hii) phase, and non-lamellar phase-transitions of lipids. Biochim Biophys Acta 1031:1–69
- Sévin DC, Sauer U. 2014. Ubiquinone accumulation improves osmotic-stress tolerance in Escherichia coli. Nat Chem Biol 10:266–272
- Somersalo S, Karunen P, Aro E-M. 1986. The acyl lipid composition of wheat leaves and moss protonemata using a new, non-carcinogenic extraction solvent system. Physiologia Plantarum 68:467–470
- Spickett CM. 2007. Chlorinated lipids and fatty acids: An emerging role in pathology. Pharmacol Therapeut 115:400–409
- Sreckovic I, Birner-Gruenberger R, Besenboeck C, Miljkovic M, Stojakovic T, Scharnagl H, et al. 2014. Gestational diabetes mellitus modulates neonatal high-density lipoprotein composition and its functional heterogeneity. Biochim Biophys Acta (BBA) – Molec Cell Biol Lipids 1841:1619–1627
- Steiner S, Sharron S, Waechter CJ, Lester RL. 1969. Isolation and partial characterization of a major inositol-containing lipid in baker’s yeast, mannosyl-diinositol, diphosphoryl-ceramide. Proc Natl Acad Sci USA 64:1042–1048
- Tam VC. 2013. Lipidomic profiling of bioactive lipids by mass spectrometry during microbial infections. Semin Immunol 25:240–248
- Tan RX, Chen JH. 2003. The cerebrosides. Nat Prod Rep 20:509–534
- van Kampen MD, Rosenstein R, Götz F, Egmond MR. 2001. Cloning, purification and characterisation of Staphylococcus warneri lipase 2. Biochim BiophysActa (BBA) – Protein Struct Molec Enzymol 1544:229–241
- Wakelam MJO, Pettitt TR, Postle AD. 2007. Lipidomic analysis of signaling pathways. In: Brown HA, editor. Methods in Enzymology. San Diego, VA: Academic Press, 233–246
- Wang A-Y, Cronan JE. 1994. The growth phase-dependent synthesis of cyclopropane fatty acids in Escherichia coli is the result of an RpoS(KatF)-dependent promoter plus enzyme instability. Molec Microbiol 11:1009–1017
- Westphal O, Lüderitz O, Bister F. 1952. Über die Extraktion von Bakterien mit Phenol/Wasser [On the extraction of bacteria using phenol/water]. Z Naturforschg Tiel B 7:148–155
- Wu SSH, Platt KA, Ratnayake C, Wang T-W, Ting JTL, Huang AHC. 1997. Isolation and characterization of neutral-lipid-containing organelles and globuli-filled plastids from Brassica napus Tapetum. Proc Natl Acad Sci USA 94:12711–12716
- Xiao L, Mjos SA, Haugsgjerd BO. 2012. Efficiencies of three common lipid extraction methods evaluated by calculating mass balances of the fatty acids. J Food Composit Analysis 25:198–207
- Yetukuri L, Söderlund S, Koivuniemi A, Seppänen-Laakso T, Niemelä PS, Hyvönen M, et al. 2010. Composition and lipid spatial distribution of HDL particles in subjects with low and high HDL-cholesterol. J Lipid Res 51:2341–2351
- Yi EC, Hackett M. 2000. Rapid isolation method for lipopolysaccharide and lipid A from Gram-negative bacteria. Analyst 125:651–656
- Yore MM, Syed I, Moraes-Vieira PM, Zhang T, Herman MA, Homan EA. 2014. Discovery of a class of endogenous mammalian lipids with anti-diabetic and anti-inflammatory effects. Cell 159:318–332
- Zhou P, Hu R, Chandan V, Kuolee R, Liu X, Chen W, et al. 2012. Simultaneous analysis of cardiolipin and lipid A from Helicobacter pylori by matrix-assisted laser desorption/ionization time-of-flight mass spectrometry. Molec BioSyst 8:720–725