Abstract
Stress and depression may share common neural plasticity mechanisms. Importantly, the development and reversal of stress-induced plasticity requires time. These temporal aspects, however, are not captured fully in the forced-swim test (FST), a behavioural model for testing antidepressant efficacy, used originally in naïve animals. The present study probed whether and how a rodent model of stress affects behaviour in the FST over time. We found that the intensity and duration of stress are critical in the development of depressive symptoms in male Wistar rats (n = 37) as tested in the FST. Chronic immobilization stress (2 h/day for 10 days) elicited a range of responses, from low to high values of immobility in the FST on day 1, and subsequent immobility on day 2 was inversely related to individual day 1 values. As a whole, chronically stressed rats did not exhibit any significant change in immobility either on day 1 or day 2 compared to control rats. However, climbing behaviour was reduced uniformly from day 1 to day 2, despite the differences in immobility. In contrast, a separate group of rats (n = 30) subjected to the same chronic stressor displayed a significant reduction in open-arm exploration in the elevated plus maze, indicative of a robust increase in anxiety-like behaviour. Furthermore, when the 10-day chronic stress paradigm was reduced to a single 2-h episode of immobilization stress, it triggered a uniform day 1 to day 2 increase in immobility, which was not persistent 10 days later. These results highlight a need for closer examination of the ways in which stress-induced modulation of behaviour in the FST may be used and interpreted in future studies aimed at exploring connections between stress and depression.
Introduction
Research on the neurobiology of depression and antidepressant action is faced with the challenge of elucidating the roles of various genetic and environmental factors underlying the pathophysiology of the disorder (Nestler et al. Citation2002a, Krishnan and Nestler Citation2008). This has impacted on the development of animal models that attempt to capture specific facets of the complex range of symptoms seen in the human condition. Despite these limitations, two lines of investigation have been particularly fruitful. First, studies relying on animal models have focused on the striking parallels between key features of severe depression and dysregulation of the stress response (Pittenger and Duman Citation2008). These studies highlight the pivotal role played by stress-induced plasticity mechanisms in corticolimbic structures that are considered to underlie key symptoms of depression (Calabrese et al. Citation2009). Importantly, neuroimaging studies in humans suffering from depression also implicate many of the same brain areas, thereby providing convergence between clinical data and animal models. Second, animal studies have also made use of antidepressant treatments that are effective in alleviating depressive symptoms in humans. Antidepressants, however, take several weeks to yield benefits (Nestler et al. Citation2002b). There is a growing consensus that this temporal dimension stems from the underlying structural plasticity caused by stress, and its reversal by antidepressant treatment—both of which take a long time (Duman et al. Citation1997, Brown et al. Citation1999, McEwen Citation1999).
To predict the antidepressant efficacy of a drug, the Porsolt forced-swim test (FST) is a widely used and effective behavioural assay for rodent models of depression (Porsolt et al. Citation1977, Cryan et al. Citation2002). In this test, rodents placed in an inescapable container of water display an immobile posture after initial escape-oriented behaviour. This immobility increases during subsequent replacement in the same container. This enhanced immobility, which can be blocked by antidepressant treatment, is a key measure of depression-like behaviour in this animal model. Previous studies using the FST have given rise to a comprehensive framework for interpreting behaviour on day 1 vs. day 2 wherein rodents learn on the first exposure to the FST that escape is not possible and thereby adopt a passive strategy that is manifested as greater immobility during the second exposure. Antidepressants provide beneficial effects by enhancing active coping strategies that, in turn, reduce the immobility on day 2 of the FST (Armario et al. Citation1988, Citation1991, Marti and Armario Citation1993, Citation1996). However, more recently, even immobility on a 1-day version of this test has been shown to be modified by stress hormones (Johnson et al. Citation2006). One major weakness of the FST lies in its time scales of action. First, antidepressant drugs reverse immobility in rodents in hours, whereas their action in humans takes several weeks. Second, another concern lies in the use of naïve or normal animals, rather than animals in a “diseased state”, such as that triggered by chronic stress (Schmidt et al. Citation2010). Stress or glucocorticoids have been implicated in the aetiology and development of depression in human patients (Sachar et al. Citation1973, Dinan Citation1994). This link between stress and depression has been studied with particular focus on the role of glucocorticoids in the development of depression-like behaviour (Johnson et al. Citation2006, Marks et al. Citation2009). However, another study has not found a relation between immobility and corticosterone levels achieved in the FST (Marti and Armario Citation1996). Third, a relatively brief placement in an inescapable container of water triggers the increase in immobility upon re-exposure the next day. By contrast, robust structural plasticity in corticolimbic structures, implicated in depression, is triggered by chronic stress. Finally, the increase in immobility in the FST happens on a timescale that is considerably shorter than those leading to stress-induced plasticity (Cryan et al. Citation2002).
Therefore, the present study addressed these issues by exploring the impact of different rodent models of stress on behaviour in the FST. The choice of stress models was guided by previous studies of how the duration and severity of stress modulates anxiety-like behaviour over different timescales (Vyas et al. Citation2002, Mitra et al. Citation2005). Specifically, we examined whether paradigms of chronic immobilization stress (CIS) and acute immobilization stress (AIS), which elicit morphological changes in corticolimbic structures implicated in depression and are also reversed by antidepressants, amplify depressive symptoms in the FST in a manner consistent with the implicit rationale of this behavioural assay. Thus, our goal was to test the hypothesis that chronic stress would increase depression-like behaviour in the FST. In addition, noting earlier reports on differences in the short- and long-term effects of acute stress on anxiety-like behaviour, we hypothesized that acute stress may also elicit time-dependent effects on depression-like behaviour in the FST. In view of the current interpretation (Johnson et al. Citation2006) of how behaviour on the 2 days of the FST manifest depression-like behaviour, we analyzed the effects of prior stress on behavioural parameters on both day 1 and day 2 of the FST.
Methods
Animals
Two- to three-month-old male Wistar rats, weighing 240–320 g, were used in this study. They were housed with siblings with a 14 -h day-10-h night cycle and ad libitum access to food and water. Lights were switched on at 06:00 h. All rats were housed in cages containing either two or three siblings. Ambient temperature in the animal house was 22 ± 2°C. All procedures related to maintenance and experimental protocols were in accordance with National Institutes of Health guidelines and approved by the Institutional Animal Ethics Committee. The number of rats for the FST was control = 8, chronic immobilization stress (CIS) = 14, AIS = 7, AIS +10 days = 8. For the Elevated Plus Maze (EPM), the number of rats was control = 15 and CIS = 15. All groups in an experiment were studied together.
Stress
CIS consisted of containment in rodent immobilization bags for 2 h/day (10:00 h–12:00 h) for 10 consecutive days (Vyas et al. Citation2002). Rodent immobilization bags are cone-shaped polyethene bags with the apex of the cone cut open. Each rat was placed within the bag with the nose emerging from the cut end, allowing unrestricted breathing. The open rear end was then closed with tape, such that the rat was immobilized within the bag. Experimenters ensured that the rat's legs were not folded and the neck was not bent abnormally. AIS was a single episode of 2 h of such immobilization (Vyas et al. Citation2002, Mitra et al. Citation2005). Four treatment conditions were compared: control, CIS, AIS+1 day and AIS+10 days.
Forced-swim test
On day 1, rats were placed singly in a cylinder (63-cm high) filled with water to a depth of 50 cm (28°C) for 15 min. At this depth, the rats cannot touch the base of the cylinder with their tail. After the test, rats were dried and returned to their home cages. Twenty-four hours later, rats were placed in the same cylinder again for 5 min. The experimental setup was placed in a cubicle surrounded by black curtains. Sessions were videotaped, and the first 5 min on each day was analyzed, as previously described (Cryan et al. Citation2002, Shirayama et al. Citation2002). Behavioural testing for depression-like behaviour was done 24 h after the end of the stress protocol. For comparisons among the CIS, AIS and control groups, age-matched rats were tested on the same day.
Elevated plus maze
The elevated plus maze consisted of two opposite open arms (70 × 15 cm, surrounded by 1-cm high transparent walls) and two enclosed arms (70 × 15 cm, surrounded by 20-cm high opaque walls). The maze was elevated 75 cm above the ground. Individual trials lasted for 5 min each and the behaviour was recorded with a video camera for off-line analysis. At the beginning of each trial, rats were placed at the centre of the maze (15 × 15 cm), facing an enclosed arm. All trials were conducted between 10:00 and 14:00 h, and the maze was cleaned with 20% ethanol solution (v/v) after each trial.
Behavioural analysis
Behavioural analysis for immobility was done using the Noldus Behaviour analysis software (Ethovision 3.0, Noldus Information Technology, Wageningen, Netherlands). Immobility was defined as the rat making no movements except those essential for staying afloat (Shirayama et al. Citation2002). FST behaviour was initially analyzed manually for immobility values. This manual calibration was then used as the basis for defining thresholds of immobility in the automated analysis using Noldus, which ensured the absence of experimenter bias. As Noldus analysis works by subtraction of pixel areas over user-defined time bins, we calibrated (a) these bins and (b) percentage of movement thresholds, until we obtained results for immobility times that were most similar to those obtained manually. The background contrast being critical for identification of the rat in Noldus 3.0, the camera was placed lateral to the cylinder, such that the white rat was viewed against a black background. At this angle, the forepaws of the rat were clearly visible, and escape-oriented climbing against the walls of the cylinder was distinguishable. Analysis of time spent on climbing was done by the experimenter by considering the active climbing movement of the forepaws against the glass wall of the cylinder. The first 5 min on each day was used for behavioural analysis, and the total time spent immobile was measured. Analysis of elevated plus maze data was done manually, and in all cases the analysis was done blind to group.
Statistical analysis
Within a behavioural group, comparisons on day 1 to day 2 used the Student's t-test, with Levene's test to make appropriate assumptions of equality of variance. Across group comparisons, with comparisons to control, were done with a one-way ANOVA, with post hoc pairwise Tukey's tests. F values and p values are given in Results. An analysis of variance was done using group as the between-subjects variable and day of FST as the within-subjects repeated measures variable. All tests were performed using Statistical Package for the Social Sciences (Statistical Package for the Social Sciences, Inc., Chicago, IL, USA). All values are stated as mean ± SEM.
Results
Chronic immobilization stress
In the first experiment, we tested the hypothesis that if prior exposure to chronic stress enhances depressive symptoms in the FST, then the increase in immobility from day 1 to day 2 in the FST, i.e. the ratio of immobility on day 2 to day 1, would be greater in stressed rats compared to that in unstressed rats. Control rats exhibited a significant increase in immobility from day 1 to day 2 (; Student's t-test, p = 0.036; assuming equal variances as tested by Levene's test, n = 8 rats), which was quantified as an index of immobility on day 2 normalized to that of day 1 (). Having confirmed that control rats indeed exhibit the expected behavioural index of enhanced immobility, we analyzed immobility levels for a second group of rats which had been subjected to CIS (2 h/day for 10 days). Twenty-four hours after the end of CIS, stressed rats were exposed to day 1 of the FST, followed by re-exposure (5 min) on day 2. Unlike control rats, the CIS-treated rats did not show any increase in immobility from day 1 to day 2 (). The normalized immobility for rats subjected to CIS was not different from that of control rats (normality of distributions tested with single sample Kolmogorov-Smirnov test, one-way ANOVA across all groups followed by post hoc Tukey's test; ). However, on day 2, individual rats responded to chronic stress in a divergent manner: half of the CIS rats (n = 7) showed lower immobility on day 2 than on day 1, and half showed greater immobility on day 2 ().
Figure 1. Chronic stress results in a differential response to the FST. (a) Control rats show an increase in immobility from day 1 to day 2 of the Porsolt FST, (*p = 0.036, n = 8). (b) After 10 days of CIS, rats no longer show an increase in immobility. Inset: schematics of experimental design. (c) This increase was measured as normalized immobility = day 2 immobility/day 1 immobility. (d) Some rats showed high levels of immobility on day 1, which decreased on day 2; (**p = 0.004, n = 7) and (e) other rats began at lower immobility levels on day 1, which increased on day 2; (**p = 0.001, n = 7).
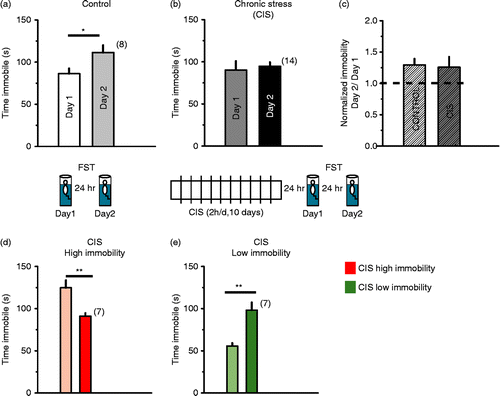
Inspection of the behaviour of the rats on day 1 indicated that the rats with higher immobility on day 1 (mean (124.8 ± 8.8 s, n = 7; ) showed reduced immobility on day 2, and rats that showed lower immobility on day 1 (55.7 ± 3.5 s, n = 7; ) showed increased immobility on day 2. Hence, the CIS rats were categorized post hoc into two subgroups, along the median of the population, by their behaviour on day 2 with respect to their behaviour on day 1. Nonetheless, on day 2 these subgroups showed comparable levels of immobility, i.e. the two subgroups that had exhibited higher or lower immobility on day 1 no longer showed a difference on day 2. The subdivision into two behavioural subgroups in terms of time spent immobile on day 1, as well as a contrasting coping behaviour on day 2, was not explained by genotype, cage assignment or body weight differences. In several cases, siblings housed in the same cage fell into different behavioural subsets, and this assignment did not correlate consistently with any differences in body weight between the siblings. In order to take into consideration the within-group effect of the repeated measurement of immobility on the same rats on two consecutive days, as well as the between-group effects, we used a mixed-model ANOVA considering all behavioural groups, as discussed below.
This differential effect on immobility led us to examine the impact of CIS on the FST in greater detail. To this end, we next focused on another key behavioural parameter in the FST—active escape-oriented behaviour manifested as “climbing” (Detke et al. Citation1995). We found that there was the expected decrease in climbing behaviour from day 1 to day 2 of the FST in control rats (, p < 0.01, Student's t-test). Furthermore, rats subjected to CIS also show a significant decrease in climbing (, p < 0.001, Student's t-test). Importantly, the two CIS subgroups that exhibited differential responses in immobility showed no difference in climbing either on day 1 or on day 2 (). Hence, unlike immobility, the response to CIS was uniform in terms of climbing behaviour. Normalized values of climbing, measured as a ratio of time spent climbing on day 2 and on day 1, when compared with those of control showed only a non-significant trend towards reduction (). Nor was there any difference between control and the CIS subgroups on day 1 (p = 0.4, F2,20 = 0.95, one-way ANOVA) or on day 2 (p = 0.32, F2,20 = 1.2, one-way ANOVA).
Figure 2. CIS effects on climbing behaviour in the FST. Note that in contrast to immobility (Figure 1), climbing behaviour was not affected by CIS and was not dependent on the previous levels of climbing on day 1. Nor was there any change in the day 2/day 1 ratio of climbing. (a) Climbing was reduced from day 1 to day 2 of the FST in control rats. (p < 0.01, n = 8) (b) A similar decrease in climbing from day 1 to day 2 is seen in rats subjected to CIS (p < 0.001, n = 14). On day 1, time spent climbing was the same for control and CIS rats. (c, d) Rats are colour coded to indicate high immobility (red) and low immobility (green) subgroups. These two subgroups did not show any difference in climbing behaviour. (e) Normalized climbing is not different between control and CIS rats (p>0.05). Rats subjected to CIS show a reduction in (f) open-arm time (**p < 0.01) and (g) open-arm entries (*p < 0.05) on the elevated-plus maze. (h) Locomotor activity, as indicated by an equal number of closed arm entries, was not different. (i) CIS rats also showed reduced body-weight gain. (** p < 0.001, n = 15 in each group).
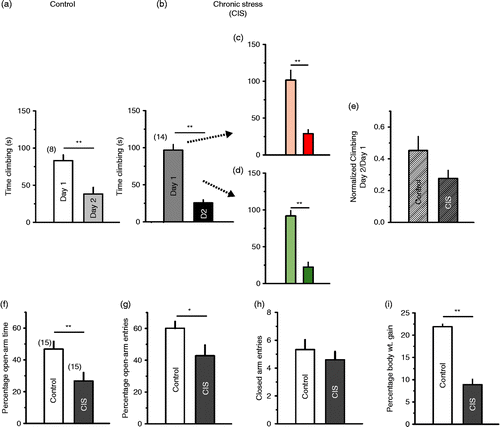
As CIS evidently differed in its impact on different behavioural parameters in the FST, in a separate group of rats we tested anxiety-like behaviour in the elevated plus-maze at 24 h after the end of CIS. As expected (Vyas et al. Citation2002), anxiety-like behaviour was increased 24 h after CIS, compared to that of control rats. Specifically, the percentage of open-arm time and open arm entries was reduced (; p < 0.01, p < 0.05 respectively, Student's t-test, n = 15 rats each in CIS and control groups). CIS had no significant effect on locomotor activity as measured by the number of closed arm entries in the plus-maze (, p = 0.43, Student's t-test).
The decrease in body weight gain when rats were subjected to chronic stress was uniform across all rats (; p < 0.001, Student's t-test).
Acute immobilization stress
We tested whether AIS would have a different effect from CIS on behaviour in the FST. When tested 24 h after AIS, there was no difference in day 1 levels of immobility between controls and AIS rats (see below). Moreover, unlike after CIS, 24 h after AIS all rats showed initial low immobility on day 1, followed by a significant increase on day 2 (, p < 0.05, Student's t-test).
Figure 3. The response to AIS was different from that elicited by chronic stress. (a) AIS rats showed an increase in immobility from day 1 to day 2 of the FST: (**p < 0.01, n = 7). Inset: schematic of experimental design (b) This effect of AIS was no longer present after 10 days of stress-free recovery. Day 1 immobility was different between AIS+1 day and AIS+10 days groups (p < 0.05, post hoc Tukey's test). (c) Normalized immobility was significantly lower after 10 days (p < 0.001, one-way ANOVA, post hoc Tukey's test **p < 0.01, n = 7, 8 rats). (d, e) Effects of AIS on climbing 1 and 10 days later (p < 0.01, Student's t-test).
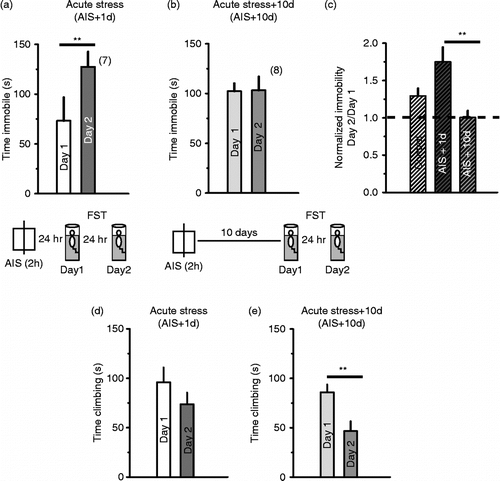
As depressive behaviour in the FST was already high 1 day after AIS, we tested AIS effects on behaviour in the FST 10 days later. AIS did not increase immobility in the FST 10 days later: normalized immobility was significantly lower compared to that observed 1 day after AIS (; p < 0.01, F4,33 = 12.8, one-way ANOVA, post hoc Tukey's test p < 0.01). There was also a difference in day 1 immobility between the AIS+1 day and AIS+10 days groups (; p < 0.001, F4,33, one-way ANOVA, post hoc Tukey's test p < 0.05). Comparing across groups, there was a significant within-subject day 1 to day 2 change in immobility (p < 0.001, F1,36 = 18.1, General Linear Model repeated measures analysis of variance) as well as a significant interaction between day and the between-subject variable of group (p < 0.001, F4,33 = 13.5), with no between-subject effect of group alone (p = 0.08, F4,33 = 2.3). The AIS+10 days group did not show the day 1 to day 2 increase in immobility seen in control rats or the AIS+1 day group (). This finding was similar to that of the CIS rats overall, but in contrast to the CIS rats, there was no pattern of rats with higher immobility on day 1 decreasing time spent immobile on day 2 and vice versa.
Climbing behaviour in the FST was also measured for the AIS groups (). Within-subject effects from day 1 to day 2 were significant (p < 0.001, F1,36 = 82.1, mixed model ANOVA), with decrease in climbing from day 1 to day 2 in (). Across-group comparison of climbing (between-subject effects) was not significantly different (p = 0.123, F3,34 = 2.7).
Comparison of the ratio of climbing (day 2/ day 1) showed that there was a significant difference in the effects of CIS and AIS (one-way ANOVA; p < 0.01, F3,34 = 6.3, post hoc Tukey's test, p < 0.05).
Discussion
Here, we investigated how different durations of immobilization stress, which are known to activate cellular and molecular correlates of depression in rat models, affect behaviour in the FST over time (Porsolt et al. Citation1977, Anisman and Zacharko Citation1990, Citation1992, Armario et al. Citation1991, Gil et al. Citation1992, Nestler et al. Citation2002a). We first analyzed the impact of 10 days of CIS. Unexpectedly, in contrast to unstressed control rats, CIS rats no longer showed the characteristic increase in immobility from day 1 to day 2 of the FST. We found that the apparent lack of effect of chronic stress was caused by individual differences in rats that exhibited opposite responses in the FST. The changes in immobility from day 1 to day 2 in CIS-treated rats were dependent on their immobility scores on day 1. Rats with greater immobility on day 1 showed a reduction in immobility on day 2, whereas the opposite was found with those showing lower immobility on day 1.
Depressive symptoms have been reported earlier with repeated corticosterone treatment (Kalynchuk et al. Citation2004, Gregus et al. Citation2005, Johnson et al. Citation2006, Marks et al. Citation2009). Our results indicate that CIS shows no effect on day 2 immobility, but does result in individual variability in the day 1 response., highlighting the relevance of day 1 immobility as an effective measure of depression-like behaviour in rats subjected to prior stress. This brings into focus the important question of how to interpret immobility on day 1 alone vs. a change in immobility from day 1 to day 2 in the FST, issues that have also been addressed in earlier studies (Overstreet and Griebel Citation2004, Johnson et al. Citation2006). As immobility on day 2 has been widely used as a direct measure of a depressogenic effect, a decrease in immobility on day 2 could be viewed as an “antidepressant effect”. Interestingly, chronic restraint stress for 11 days has also been found to actually result in antidepressant-like effects in the FST (Platt and Stone Citation1982).
Within the traditional framework of the FST, several previous studies have used immobility on day 2 of a two-day FST as a measure of depression (Siuciak et al. Citation1997), with day 1 serving as the inescapable stress that causes it. More recently, a single exposure to swim stress has been studied as a measure of depression as well as an effective test of antidepressant efficacy (Armario et al. Citation1988, Overstreet and Griebel Citation2004, Johnson et al. Citation2006). Our study considers both measures of depression-like behaviour—that on day 1 alone, as well as the increase from day 1 to day 2. Although differences that we found in day 1 effects are a direct consequence of prior stress on immobility or climbing behaviour, the subsequent effects on day 2 also implicate the capacity of the rat to learn to cope with the stress induced by forced swim (but see Marks et al. Citation2009). Clearly, the prolonged immobilization stress was a more aversive experience compared to that of day 1 in the FST, which itself is known to affect subsequent FST behaviour (Dal-Zotto et al. Citation2000). Overall, these divergent effects of chronic stress on immobility highlight the ways in which behaviour on the 2 days of the FST can be interpreted, based on immediate response to stress as well as on subsequent adaptive or learning behaviour. Furthermore, these effects could also be a manifestation of the ability of stress to alter learning capabilities, which is consistent with a large body of evidence on the impact of stress on learning and memory in both animal models and stress-related psychiatric disorders (Marti and Armario Citation1993, McEwen Citation1999, Vasterling et al. Citation2002, Swiergiel et al. Citation2008, Conrad Citation2009, Bangasser and Shors Citation2010). Finally, the inter-individual differences in response to stressors (Anisman and Zacharko Citation1990) are relevant to our observations on the high variability in the immobility response after chronic stress. However, in the present study, this variability is specific to one parameter of depression-like behaviour, that of immobility. Climbing behaviour, known to be selectively and specifically modified by a subset of antidepressants (Cryan and Lucki Citation2000, Cryan et al. Citation2002), is not different between the same animals that show a divergence in immobility.
Results presented here also suggest that a significantly shorter duration of stress did not elicit the divergent immobility behaviour seen after chronic stress. Unlike CIS, AIS caused a uniform increase in immobility in all the rats tested in the 2-day FST. This AIS-induced increase in immobility, however, was not long-lasting—it was evident 1 day, but not 10 days after the AIS (). This is in striking contrast to earlier reports showing that the same AIS that fails to have any anxiogenic effects 1 day later gives rise to enhanced anxiety 10 days later (Mitra et al. Citation2005, Mitra and Sapolsky Citation2008, Roozendaal et al. Citation2009). Thus, the temporal profile of both the short- and long-term effects of acute stress on behavioural measures of anxiety and depression appears to be quite different. A previous study, aimed at characterizing the effects of acute vs. chronic stress on performance in the FST, has shown that repeated exposure to uncontrollable footshock does not cause adaptation to the behavioural changes ordinarily elicited by acute shock stress (Prince and Anisman Citation1984). Taken together, these findings also suggest that the intensity and duration of the stressor may play a pivotal role in the temporal development and persistence of affective symptoms triggered by stress. Therefore, future studies aimed at dissecting out the role of stress as a causative factor in how and why mood disorders such as anxiety and depression develop are likely to provide a useful framework for developing more effective antidepressants.
Acknowledgements
This work was supported by an International Senior Research Fellowship from the Wellcome Trust, UK, to SC. We thank Supriya Ghosh for useful comments and suggestions.
Declaration of interest: The authors report no conflicts of interest. The authors alone are responsible for the content and writing of the paper.
References
- Anisman H, Zacharko RM. 1990. Multiple neurochemical and behavioral consequences of stressors: Implications for depression. Pharmacol Ther. 46:119–136.
- Anisman H, Zacharko RM. Depression as a consequence of inadequate neurochemical adaptation in response to stressors. Br J Psychiatry Suppl. 199236–43.
- Armario A, Gavalda A, Marti O. 1988. Forced swimming test in rats: Effect of desipramine administration and the period of exposure to the test on struggling behavior, swimming, immobility and defecation rate. Eur J Pharmacol. 158:207–212.
- Armario A, Gil M, Marti J, Pol O, Balasch J. 1991. Influence of various acute stressors on the activity of adult male rats in a holeboard and in the forced swim test. Pharmacol Biochem Behav. 39:373–377.
- Bangasser DA, Shors TJ. Critical brain circuits at the intersection between stress and learning. Neurosci Biobehav Rev. 2010 (in press).
- Brown ES, Rush AJ, McEwen BS. 1999. Hippocampal remodeling and damage by corticosteroids: Implications for mood disorders. Neuropsychopharmacology. 21:474–484.
- Calabrese F, Molteni R, Racagni G, Riva MA. 2009. Neuronal plasticity: A link between stress and mood disorders. Psychoneuroendocrinology. 34 Suppl 1: S208–S216.
- Conrad CD. A critical review of chronic stress effects on spatial learning and memory. Prog Neuropsychopharmacol Biol Psychiatry. 2009 November 10 [Epub ahead of print].
- Cryan JF, Lucki I. 2000. Antidepressant-like behavioral effects mediated by 5-Hydroxytryptamine(2C) receptors. J Pharmacol Exp Ther. 295:1120–1126.
- Cryan JF, Markou A, Lucki I. 2002. Assessing antidepressant activity in rodents: Recent developments and future needs. Trends Pharmacol Sci. 23:238–245.
- Dal-Zotto S, Marti O, Armario A. 2000. Influence of single or repeated experience of rats with forced swimming on behavioural and physiological responses to the stressor. Behav Brain Res. 114:175–181.
- Detke MJ, Rickels M, Lucki I. 1995. Active behaviors in the rat forced swimming test differentially produced by serotonergic and noradrenergic antidepressants. Psychopharmacology (Berl). 121:66–72.
- Dinan TG. 1994. Glucocorticoids and the genesis of depressive illness. A psychobiological model. Br J Psychiatry. 164:365–371.
- Duman RS, Heninger GR, Nestler EJ. 1997. A molecular and cellular theory of depression. Arch Gen Psychiatry. 54:597–606.
- Gil M, Marti J, Armario A. 1992. Inhibition of catecholamine synthesis depresses behavior of rats in the holeboard and forced swim tests: Influence of previous chronic stress. Pharmacol Biochem Behav. 43:597–601.
- Gregus A, Wintink AJ, Davis AC, Kalynchuk LE. 2005. Effect of repeated corticosterone injections and restraint stress on anxiety and depression-like behavior in male rats. Behav Brain Res. 156:105–114.
- Johnson SA, Fournier NM, Kalynchuk LE. 2006. Effect of different doses of corticosterone on depression-like behavior and HPA axis responses to a novel stressor. Behav Brain Res. 168:280–288.
- Kalynchuk LE, Gregus A, Boudreau D, Perrot-Sinal TS. 2004. Corticosterone increases depression-like behavior, with some effects on predator odor-induced defensive behavior, in male and female rats. Behav Neurosci. 118:1365–1377.
- Krishnan V, Nestler EJ. 2008. The molecular neurobiology of depression. Nature. 455:894–902.
- Marks W, Fournier NM, Kalynchuk LE. 2009. Repeated exposure to corticosterone increases depression-like behavior in two different versions of the forced swim test without altering nonspecific locomotor activity or muscle strength. Physiol Behav. 98:67–72.
- Marti J, Armario A. 1993. Effects of diazepam and desipramine in the forced swimming test: Influence of previous experience with the situation. Eur J Pharmacol. 236:295–299.
- Marti J, Armario A. 1996. Forced swimming behavior is not related to the corticosterone levels achieved in the test: A study with four inbred rat strains. Physiol Behav. 59:369–373.
- McEwen BS. 1999. Stress and hippocampal plasticity. Annu Rev Neurosci. 22:105–122.
- Mitra R, Sapolsky RM. 2008. Acute corticosterone treatment is sufficient to induce anxiety and amygdaloid dendritic hypertrophy. Proc Natl Acad Sci USA. 105:5573–5578.
- Mitra R, Jadhav S, McEwen BS, Vyas A, Chattarji S. 2005. Stress duration modulates the spatiotemporal patterns of spine formation in the basolateral amygdala. Proc Natl Acad Sci USA. 102:9371–9376.
- Nestler EJ, Barrot M, DiLeone RJ, Eisch AJ, Gold SJ, Monteggia LM. Neurobiology of depression. Neuron. 2002a; 34:13–25.
- Nestler EJ, Gould E, Manji H, Buncan M, Duman RS, Greshenfeld HK, Hen R, Koester S, Lederhendler I, Meaney M, Robbins T, Winsky L, Zalcman S. Preclinical models: Status of basic research in depression. Biol Psychiatry. 2002b; 52:503–528.
- Overstreet DH, Griebel G. 2004. Antidepressant-like effects of CRF1 receptor antagonist SSR125543 in an animal model of depression. Eur J Pharmacol. 497:49–53.
- Pittenger C, Duman RS. 2008. Stress, depression, and neuroplasticity: A convergence of mechanisms. Neuropsychopharmacology. 33:88–109.
- Platt JE, Stone EA. 1982. Chronic restraint stress elicits a positive antidepressant response on the forced swim test. Eur J Pharmacol. 82:179–181.
- Porsolt RD, Le Pichon M, Jalfre M. 1977. Depression: A new animal model sensitive to antidepressant treatments. Nature. 266:730–732.
- Prince CR, Anisman H. 1984. Acute and chronic stress effects on performance in a forced-swim task. Behav Neural Biol. 42:99–119.
- Roozendaal B, McEwen BS, Chattarji S. 2009. Stress, memory and the amygdala. Nat Rev Neurosci. 10:423–433.
- Sachar EJ, Hellman L, Roffwarg HP, Halpern FS, Fukushima DK, Gallagher TF. 1973. Disrupted 24-hour patterns of cortisol secretion in psychotic depression. Arch Gen Psychiatry. 28:19–24.
- Schmidt MV, Scharf SH, Sterlemann V, Ganea K, Liebl C, Holsboer F, Muller MB. High susceptibility to chronic social stress is associated with a depression-like phenotype. Psychoneuroendocrinology. 2010635–643.
- Shirayama Y, Chen AC, Nakagawa S, Russell DS, Duman RS. 2002. Brain-derived neurotrophic factor produces antidepressant effects in behavioral models of depression. J Neurosci. 22:3251–3261.
- Siuciak JA, Lewis DR, Wiegand SJ, Lindsay RM. 1997. Antidepressant-like effect of brain-derived neurotrophic factor (BDNF). Pharmacol Biochem Behav. 56:131–137.
- Swiergiel AH, Leskov IL, Dunn AJ. 2008. Effects of chronic and acute stressors and CRF on depression-like behavior in mice. Behav Brain Res. 186:32–40.
- Vasterling JJ, Duke LM, Brailey K, Constans JI, Allain ANJr, Sutker PB. 2002. Attention, learning, and memory performances and intellectual resources in Vietnam veterans: PTSD and no disorder comparisons. Neuropsychology. 16:5–14.
- Vyas A, Mitra R, Shankaranarayana Rao BS, Chattarji S. 2002. Chronic stress induces contrasting patterns of dendritic remodeling in hippocampal and amygdaloid neurons. J Neurosci. 22:6810–6818.