Abstract
Nociceptin (N/OFQ) peptide has regulatory roles in neuroendocrine responses to stress. We sought to clarify the roles of nociceptin and its receptors (NOP receptors) in the regulation of rat bed nucleus of the stria terminalis (BNST) neurones in vitro. The effect of nociceptin (75 nM) across subregions of the anterior BNST was determined using extracellular single-unit recordings in rat brain slices. Firing patterns of the neurones were recorded in the presence of N-methyl-D-aspartate (NMDA, 10 μm) for the classification of putative cell types. Based on the firing patterns, four cell types were identified. The distribution of cell types differed between the dorsal and ventral BNST. Nociceptin inhibited the activity of 53.2% of all the neurones tested (n = 47), regardless of the cell type or subregion. The duration of nociceptin-mediated inhibition of cell firing was significantly attenuated by pre-treatment with the NOP receptor antagonist, UFP-101 (750 nM), indicating that nociceptin-induced suppression of firing rate involves NOP receptor activation in the BNST. Pre-treatment of slices with 100-nM corticosterone (CORT) vs. dimethylsulphoxide (DMSO) for 20 min significantly abolished the nociceptin-induced inhibition of firing rate (P < 0.001) when tested 2 h later. We did not, however, observe a significant effect of CORT on baseline firing rate or pattern in BNST neurones. We suggest that the interaction between nociceptin and glucocorticoids in the BNST may be essential for normal adaptive stress responses.
Introduction
In the context of integrated responses to stress and anxiety, much interest has centred on the function of the bed nucleus of the stria terminalis (BNST). The BNST is a limbic region comprising a complex of subnuclei that can be segregated in terms of their cytoarchitecture, neurochemistry and major communicating pathways (Ju and Swanson Citation1989; Ju et al. Citation1989; Shimada et al. Citation1989). The BNST is situated ventral to the lateral septum and dorsal to the preoptic regions and is therefore anatomically well positioned to act as a functional relay between limbic structures and hypothalamus. In terms of neuroendocrine stress responses and HPA axis regulation, the BNST appears to be topographically organised, with recent evidence suggesting that the anterior compartment of the BNST sends projections to the PVN region providing excitatory input, with the posterior BNST effecting tonic inhibition (Choi et al. Citation2007). Given that there is little, if any, direct PVN afferent input originating from the limbic sites (amygdala, hippocampus), the BNST subserves a vital role in the coordination and control of hypophysiotropic parvocellular PVN (pPVN) neurones in the signature response to stress. Indeed, there is a tight connection between the BNST and hypothalamus, and electrical stimulation of the former elicits changes in both cardiovascular and HPA axis parameters.
The BNST relays inputs arising in the stress-responsive noradrenergic A1 and A2 cell groups of the brainstem, in addition to amygdaloid and hippocampal afferents, denoting extensive multisynaptic pathways converging on BNST neurones (Forray and Gysling Citation2004). The BNST is a primary site of noradrenergic neurotransmission (Woulfe et al. Citation1990), and BNST α1-adrenoceptor activation apparently mediates attenuation of the acute restraint stress-evoked pressor response (Crestani et al. Citation2009). The BNST receives diverse limbic inputs and is involved in the processing of ventral subicular inputs that serve to restrain hypophysiotrophic pPVN neurones (Cullinan et al. Citation1993). In contrast, pPVN stimulatory inputs originating in the medial amygdala are relayed via the medial BNST (Feldman et al. Citation1990). In terms of the phenotypic characterisation of BNST subregions, evidence indicates that the anterior BNST contains populations of corticotrophin releasing factor (CRF)-expressing neurones, with the posterior BNST being characterised by dense glutamic acid decarboxylase expression consistent with a GABAergic neuronal phenotype (Choi et al. Citation2007). Such segregation is consistent with the BNST role as an integrative information processor in the prioritisation of excitatory or inhibitory drives, and further study of the functional organisation of the BNST complex is warranted in terms of enhanced understanding of the regulation of stress-induced HPA axis activity, cardiovascular stimulation and behavioural anxiety.
BNST CRF is of interest, given that i.c.v. CRF acutely stimulates HPA axis activity, and populations of anterior BNST neurones express CRF mRNA, including the dorsomedial and fusiform subnuclei that have dense connections with the central amygdala (Sakanaka et al. Citation1986). Indeed, CRF co-expression in glutamatergic BNST neurones may provide a positive influence over the medial pPVN. In addition to CRF, studies indicate that there are a number of neuropeptides expressed in the BNST that may be important regulators of neurotransmission within the BNST, including neuropeptide Y, galanin, somatostatin and substance P (Ju et al. Citation1989; Shimada et al. Citation1989; Walter et al. Citation1991). In recent years, the opioid-related peptide, nociceptin (N/OFQ), has emerged as a prominent peptide with significance for understanding the regulation of anxiety and stress. Particularly, dense expression of the N/OFQ precursor and NOP receptor mRNA transcripts and N/OFQ peptide expression throughout the subnuclei of the BNST (Neal et al. Citation1999a,Citationb) is potentially highly significant, given that functional interaction between N/OFQ and CRF in the BNST has been proposed on the basis of behavioural and HPA axis studies. We have recently shown that N/OFQ regulates the hypophysiotropic CRF neurones in rats, and potently regulates the basal HPA axis activity (Leggett et al. 2006). In response to the predominantly psychological stress of restraint, our recent evidence suggests that central NOP receptors are necessary for the normal termination of HPA axis activity (Leggett et al. Citation2007). N/OFQ-mediated activation of NOP receptors in the BNST is important for the acute activation of the HPA axis in response to intracranial N/OFQ peptide infusion (Green et al. Citation2007) and N/OFQ infusion i.c.v., or intra-BNST attenuates CRF-induced anxiety in the elevated plus maze test in rats (Rodi et al. Citation2008). In addition, intra-BNST infusion of N/OFQ peptide antagonises CRF-induced anorexia in rats. Evidently, interaction between these two neuropeptide systems is bi-directional, given that CRF injection i.c.v (1.0 μg/rat) increases the expression of NOP mRNA in the BNST (Rodi et al. Citation2008). Taken together, this research strongly suggests that CRFergic and N/OFQergic systems interact in the regulation of stress-related behaviour.
There is a relatively good understanding of how N/OFQ peptide regulates neuronal function in the CNS, in terms of its well-known inhibitory effects on neuronal activity. N/OFQ reduces synaptic transmission in the dentate gyrus, NMDA currents in dentate gyrus and long-term potentiation (LTP) in the hippocampal formation (Yu and Xie Citation1998). NOP receptor agonist binding activates K+ currents; in particular, G-protein-activated K+ (Kir3) channels (see Meis Citation2003). Despite the growing evidence for a role of the BNST in stress, there is less understanding about the electrophysiological and subregional characteristics of this complex. Although behavioural pharmacological studies have shown effects of N/OFQ restricted to the BNST, the characteristics of N/OFQ actions in terms of electrophysiology and subregional heterogeneity are not known. Thus, the first objective of the current study was to investigate the electrophysiological actions of N/OFQ peptide and NOP antagonist ligands within the BNST using single-unit recordings from in vitro slices of rat brain. A second objective was to investigate the effect of glucocorticoids on the response of BNST neurones to N/OFQ. As the ventral region of the anterior BNST sends significant projections to pPVN CRF cells and evidence suggests that anteroventral BNST efferents are sensitive to corticosterone (CORT) levels (Mulders et al. Citation1997), we focussed our attention on CORT-N/OFQ interactions in this subregion.
Materials and methods
Animals
Adult male Sprague Dawley rats weighing 150–200 g (Harlan, UK) were housed in a temperature- and humidity-controlled environment on a 12 hour light:12 hour dark cycle. Food and water was available ad libitum and all experiments were carried out in strict accordance with UKs Home Office regulations (Animals (Scientific Procedures) Act 1986).
Brain slice preparation
The rats were decapitated under sodium phenobarbitone (40 mg/kg i.p) anaesthesia. Brains were then quickly removed, trimmed and mounted on a chuck for sectioning using a Vibratome. Slices (400 μm) containing the anterior BNST were cut and collected in ice-cold artificial cerebrospinal fluid (aCSF; composition in mM: NaCl 124.0, MgSO4 2.4, KH2PO4 1.25, KCl 3.25, NaHCO3 26.0, D-glucose 10.0 and CaCl2 1.0, gassed with 95% O2/5% CO2).
Single-unit recordings of BNST neurones
Recordings were made in the anterior BNST, either in the dorsal or ventral compartment, with respect to the crossing of fibres of the anterior commissure (Bregma − 0.26 mm, according to the atlas of Paxinos and Watson Citation1997). After a 90-min equilibration period, extracellular recordings were made from the BNST using glass microelectrodes filled with aCSF (impedance 5–10 mΩ). The action potentials were fed to a window discriminator and the resultant pulses were recorded using the Spike2 software (CED, Cambridge, UK). Application of NMDA (10 μm) to the perfusion medium was used to stimulate spontaneous discharges of BNST neurones at rest in order to mimic endogenous glutamate input to this region. Following a minimum of 5 min of stable baseline recording, N/OFQ peptide (Tocris, Bristol, UK) was applied to the slice via the incubation medium. N/OFQ peptide was applied for 4 min at concentrations between 50 and 100 nM. The choice of concentrations of applied peptides was based on previous in vitro electrophysiological studies (Emmerson and Miller Citation1999; Shirasaka et al. Citation2001). Some neurones were also tested in the presence of selective peptidic NOP receptor antagonist, UFP-101 (750 nM). In the antagonist study, half of the cells were treated with nociceptin (75 nM) followed by a nociceptin+UFP-101 test and in the other half, the order of testing was reversed. In antagonist studies, the first peptide was applied for 4 min before second peptide exposure.
Effect of CORT pre-treatment
Anterior BNST slices subjected to CORT pre-treatment were exposed to 100-nM CORT in 0.005% DMSO (made to a final concentration in aCSF supplemented with 10 μm NMDA) for 20 min. Where possible, the ventral BNST cells were located and recordings were made prior to commencement of CORT exposure. The recordings were continued for the duration of the CORT exposure and for up to 2 h following withdrawal of CORT so as to monitor the typical response of BNST neurones to glucocorticoid treatment. Control slices were exposed to 0.005% DMSO (vehicle) only. With regard to CORT pre-treatment, recordings were made before, during and after exposure to vehicle. Following exposure to the assigned pre-treatment, the slices were left for 2 h to allow for genomic CORT action. This time testing of the response to nociceptin proceeded as described earlier. The rats were randomly assigned to either the CORT pre-treatment (n = 10) or vehicle (n = 5) condition.
Electrophysiological data analysis
Neurones displaying a firing rate above 0.1 spikes/s were considered to be suitable for analysis. Baseline firing rates were calculated from 300 s prior to the drug application until 300 s following treatment. The data were stored for offline analysis using the Spike2 software to generate sequential histograms of neuronal firing rates. The basal firing rates were determined from a minimum period of 300 s, and interspike interval histograms were calculated from the same period (300 s) for each cell. The interspike interval histograms were calculated with 150 bins each of 50 ms duration. To allow direct comparison of the changes in the firing rates relative to baseline, the spike rates were standardised between the cells by calculating the mean number of spikes per 10 s bin over a period of 250 s for each cell and subtracting the mean from all the values for that cell. This brought the baseline firing rate close to zero for each cell. For presentation purposes, an arbitrary value of 30 was then added to all values for each cell, which ensured that the spike rates did not fall into negative values during an inhibitory response.
Drugs
All drugs were made up to the specified final concentration in aCSF containing 10 μm NMDA. NMDA, nociceptin and UFP-101 (a NOP receptor antagonist) were purchased from Tocris. All drug applications were for 4 min (240 s) duration.
Statistical analysis
For electrophysiological data analysis, correlations were analysed using Pearson's correlation coefficient, except non-parametric correlations that used Spearman's Rho statistic. For treatment comparisons, the mean values were analysed using two-way ANOVA followed by post hoc multiple comparison tests. The frequencies were analysed using likelihood ratios.
Results
Classification of BNST cell types according to neuronal firing pattern
Forty-seven firing neurones were recorded from 39 slice preparations in the presence of NMDA (10 μm). Of these, 13 neurones were located in the medio-dorsal BNST, 20 in the lateral-dorsal BNST, 8 in the medio-ventral regions and 6 in the lateral-ventral region (see for the representation of anterior BNST architecture). All recordings were made within 5 h of slice preparation. The mean firing rate of these neurones was 2.9 ± 0.4 spikes/s and, based on their firing pattern, they were classified into four different categories (Types A–D; ). Type A neurones (14/47, i.e. 29.8%) displayed a continuous and regular pattern of firing with a near-constant interspike interval. The mean firing rate of these neurones was 4.2 ± 0.8 spikes/s. A typical interspike interval histogram of a continuous (regular) firing neurone displayed a normal distribution (see ). Type B neurones (21/5, i.e. 44.7%) oscillated between periods of high- and low-frequency firings and had a mean firing rate of 1.3 ± 0.2 spikes/s. Interspike interval histograms showed a positively skewed distribution (). Type C neurones (3/47, i.e. 6.4%) were characterised by periods of discontinuous activity with periods of well-defined activity and quiescence (). These bursting neurones (regular cells) had consistent patterns of activity. The mean firing rate of these cells was 2.2 ± 0.2 spikes/s. These cells had bimodal interspike interval histograms, with the first peak representing the interspike intervals within a burst and the second smaller peak representing the inter-burst intervals (see ). Type D neurones included 17.0% (8/47) of tested cells that displayed an irregular pattern of discontinuous activity. These cells had a mean firing rate of 2.1 ± 0.4 spikes/s. Interspike interval histograms were characterised by a sharp peak of short interspike intervals representing intra-burst firings and were positively skewed reflecting the interspike intervals between the bursts (). A small number (2.1%) of neurones were deemed “non-classified” as they displayed a range of irregular firing patterns (see ).
Figure 1. (a) Schematic diagram illustrating placement of electrodes for recording of rat anterior BNST neurones. Slices were sectioned at the level of the anterior commissure crossing which divides the BNST broadly into dorsal (supra-commissure) and ventral (sub-commissure) compartments. In this plane (Bregma − 0.26 mm), anterodorsal and anteroventral BNST can be further divided into medial and lateral parts. In this study, different cell types are indicated by different shaped symbols. A non-random distribution between subregions of the BNST was suggested when the cell types were plotted in this way. Key: BSTMA, medial dorsal BNST; BSTLP, lateral dorsal BNST; BSTMV, medial ventral; BSTLV, lateral ventral BNST (see atlas of Paxinos and Watson for reference map). (b) The distribution of cell types between the dorsal and ventral BNST regions (bars represent medial/lateral combined). BNST neurones could be subclassified on the basis of their essential firing characteristics (see descriptive results), and major BNST regions were differentiated in terms of the percentage of cells falling into each category.
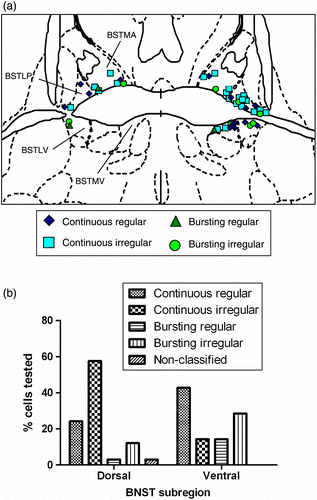
Figure 2. (a–d) Representative firing patterns and interspike interval histograms from BNST neurones recorded in the dorsal (n = 33) and ventral (n = 14) subregions. Neurones could be subclassified in terms of their basic electrophysiological properties into cells firing continuously or in bursts and their baseline firing rates (P < 0.001). In addition, cells were subdivided on the basis of regular or irregular firing characteristics. A few cells tested in the dorsal BNST did not fall into any of these four groups (unclassified). Most of the dorsal BNST neurones were classified as continuous irregular firing neurones, with most ventral BNST neurones displaying a continuous regular firing pattern (P = 0.040).
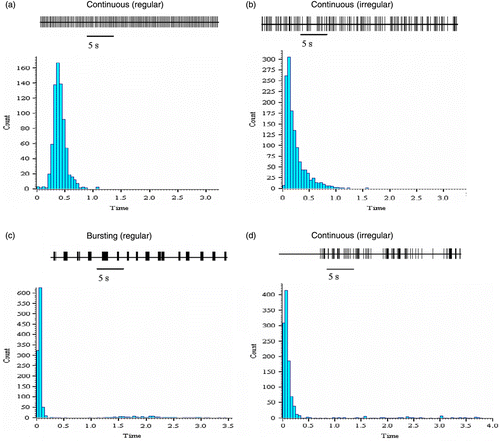
Analysis of baseline firing rates revealed significant differences between dorsal and ventral subregions (1.8 ± 0.2 spikes/s in the dorsal BNST, n = 33, vs. 3.8 ± 0.8 spikes/s in the ventral BNST, n = 14; t = 2.49, P = 0.025, equal variances not assumed). Further subdivision of the BNST into medio-dorsal (n = 13), lateral-dorsal (n = 20), medio-ventral (n = 8) and lateral ventral (n = 6) revealed that these subdivisions differed in their baseline firing rates (F = 7.95, P < 0.001). Bonferroni post hoc tests confirmed that the mean firing rate of cells recorded from the medio-ventral region (5.1 ± 1.2 spikes/s) of the BNST was significantly higher than the basal firing rates of the neurones in the medio-dorsal (2.1 ± 0.4 spikes/s, P = 0.003), lateral-dorsal (1.5 ± 0.3 spikes/s, P < 0.001) and lateral-ventral (2.1 ± 0.4 spikes/s, P = 0.019) subregions.
Effect of N/OFQ peptide and NOP antagonist on neuronal activity in the bed nucleus
A 240 s application of 75 nM N/OFQ was found to result in a clear inhibition of limited duration in a proportion of BNST neurones (see for representative example). Forty-seven neurones were tested with 75-nM N/OFQ. N/OFQ inhibited the spontaneous activity of 25 (53.2%) neurones. There was a significant correlation between the magnitude of N/OFQ-induced inhibition (firing reduced to a mean of 73.9 ± 5% of the basal firing rate) and the duration (average 403.9 ± 82.1 s) of N/OFQ-induced inhibition (Pearson's correlation of − 0.686, P < 0.0001). There was no relationship between either the magnitude or duration of N/OFQ-induced inhibition and the basal firing rate of neurones. Co-application of the NOP antagonist, UFP-101, was found to significantly limit the duration of N/OFQ-induced inhibition (1068.8 ± 159.9 s vs. 495 ± 18.9 s, repeated measures t = 3.67, P = 0.035, n = 4, see ). UFP-101 also reduced the magnitude of N/OFQ-induced inhibition, although this did not reach significance (19.4 ± 5.7% vs. 26.7 ± 6.1% basal firing rate, repeated measures t = 2.33, P = 0.10, n = 4, see ). Application of NOP antagonist UFP-101 alone had no effect on the spontaneous firing of BNST neurones (, n = 6).
Figure 3. Representative frequency histograms showing inhibitory response to (a) 75 nM N/OFQ over BNST neuronal firing activity. BNST subregions did not differ significantly in terms of the mean duration of inhibition induced by N/OFQ (P = 0.188). (b) Effect of NOP antagonist application (UFP101, 750 nM) on the response of BNST neurones to N/OFQ (75 nM). UFP-101 treatment significantly attenuated the duration of the N/OFQ-induced inhibitions but did not significantly affect the magnitude of the response (n = 4).
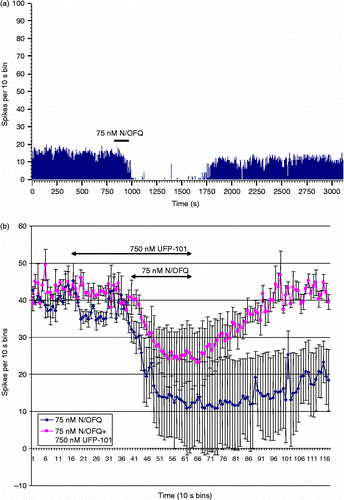
Figure 4. Histograms representing the response to combined application of N/OFQ (75 nM, N/OFQ) and UFP-101 (750 nM) on N/OFQ-induced inhibition. (a) Magnitude of firing rate did not differ significantly between UFP-101 co-treated vs. N/OFQ alone treatment, however, (b) Duration of N/OFQ-induced inhibition was significantly limited in the UFP-101 co-treated group. The values are presented as mean ± S.E.M. (n = 4/group, *P < 0.05). (c) Application of antagonist UFP-101 alone on bed nucleus neuronal firing activity. UFP-101 (750 nM) was added to cells to determine whether NOP receptors exert tonic regulation in the BNST slice preparation in vitro; however, UFP-101 application for 240 s was without effect (n = 6).
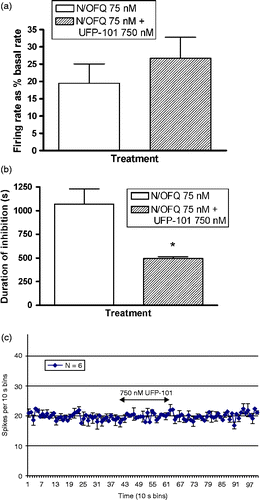
Dorsal and ventral BNST subregions and neuronal response to N/OFQ
Changes in the activity in response to N/OFQ were observed in all cell types, across all subregions of the BNST. BNST cell type did not influence the number of cells that responded to the application of N/OFQ (likelihood ratio = 3.25, P = 0.52). Similarly, there was no effect of cell type on the magnitude of N/OFQ-induced inhibition (F = 0.236, P = 0.92) or the duration of N/OFQ-induced inhibition (F = 0.806, P = 0.53). We examined the sensitivity of ventral vs. dorsal BNST neurones to N/OFQ peptide and found equivalent effects of N/OFQ in terms of firing-rate suppression. In the dorsal BNST, 16 cells (48.5% of the cells in that region) were inhibited by N/OFQ. In the ventral BNST, 9 cells (64.3% of the cells in that region) were inhibited by N/OFQ. The subregion from which each cell was recorded had no effect on the number of cells inhibited by N/OFQ (likelihood ratio = 0.998, P = 0.32, n = 47). Similarly, there was no effect of subregion on the magnitude of N/OFQ-induced inhibition. In the dorsal BNST, the mean percentage of N/OFQ-induced inhibition was 70.8% (n = 33) and in the ventral region, it was 81.1% (n = 14) (t = 1.028, P = 0.312). Similarly, there was no effect of BNST region on the duration of N/OFQ-induced inhibition. In the dorsal BNST, the mean duration of inhibition was 336.1 s (n = 31) and in the ventral region, it was 579.1 s (n = 12) (t = 1.339, P = 0.188).
NMDA responsiveness and N/OFQ-induced inhibition
Within a subset of 23 cells, the effect of withdrawing NMDA supplementation was examined and the magnitude and duration of the subsequent reduction in firing was measured. On an average, withdrawal of NMDA for 240 s resulted in a reduction in firing to 41.2 ± 25.5% of the basal rate for an average of 347.2 ± 16.8 s. A non-parametric analysis of the correlation between the percent N/OFQ-induced inhibition of individual neurones and the percent NMDA withdrawal-induced inhibition revealed a positive correlation (Spearman's rho = 0.68, P < 0.001). However, there was no relationship between the duration of N/OFQ- and NMDA-withdrawal-induced inhibition.
Effect of CORT on the neuronal activity in the BNST and response to N/OFQ
In experiments involving 33 cells from 27 slice preparations, prior exposure of the slice to CORT (100 nM) or DMSO (0.005%) vehicle was found to have no significant effect on the firing frequency of neurones recorded in the ventral or dorsal compartment of the anterior BNST (). In a proportion of cells (7 exposed to CORT and 5 to DMSO), the spike activity was recorded during the period of drug exposure. As it can be seen in the representative trace (), the firing rates remained stable during CORT application suggesting a lack of rapid CORT-mediated effect on electrical activity. Treatment with either CORT (100 nM) or vehicle (0.005% DMSO) had no effect on the mean firing rate of the neurones during the application of treatment (CORT = 100 nM, 92.5 ± 6.9 spikes/s vs. 0.005% DMSO vehicle, 88.5 ± 8.3 spikes/s; t = 0.376, P = 0.714, n = 12, ).
Figure 5. (a) Representative frequency histogram of ventral BNST cellular activity during 20-min CORT application (100 nM). Firing rates of cells recorded during application of 100-nm CORT tended to remain stable throughout the 20-min treatment period. This was also the case for cells treated with 0.005% DMSO vehicle for 20 min. (b) The mean firing rate, expressed as the % of basal firing rate, of ventral BNST neurones during a 20-min application of CORT (n = 7) did not differ from neurones exposed to vehicle (0.005% DMSO in aCSF) alone (n = 5). (c) The effect of 20 min of pre-treatment with 100-nm CORT (n = 4) or vehicle (0.005% DMSO in aCSF, n = 4) on the firing rate of BNST neurones 1 h post-treatment onset. Although a trend towards a reduced firing rate in cells in the CORT pre-treatment condition was observed, the difference was not significant. In both the histograms, values represent mean ± SEM.
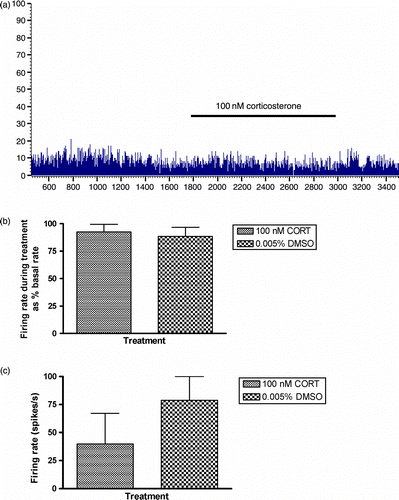
In 8 cells (4 pre-treated with CORT, 4 control slices pre-treated with vehicle), it was possible to measure the firing rate at 1 h post-treatment. Cells that had been pre-treated with CORT tended to have reduced firing rate at 1 h post-treatment (CORT = 100 nM, 39.9 ± 27.1 spikes/s vs. 0.005% DMSO vehicle, 78.8 ± 21.2 spikes/s); however, this was not a significant effect (t = 1.13, P = 0.30, n.s, ).
The effect of CORT pre-treatment on N/OFQ-induced inhibition
Ten BNST slice preparations were pre-treated with 100-nM CORT in vehicle for 20 min, while 5 control slices were pre-treated with vehicle only (0.005% DMSO) for 20 min. The cells were then tested with 75-nM N/OFQ, as per previous experiments. Representative traces from the cells tested with N/OFQ that had been treated with vehicle only (A) and 100 nM of CORT (B) are shown in . It was observed that cells recorded from slices that had been exposed to 20 min of 100-nM CORT were less likely to be inhibited by N/OFQ than those in the control condition (likelihood ratio = 10.72, P = 0.001, ). illustrates the mean response of the neurones tested with 75-nM N/OFQ. Firing rates were standardised to allow direct comparison. The analysis revealed that the post-N/OFQ firing rate of the cells that had been pre-treated with CORT was significantly higher than those that had been pre-treated with DMSO only (Mann-Whitney U = 6.00, P = 0.019), indicating that N/OFQ effect had been attenuated in CORT pre-treated slices. Multivariate analysis of the change in the firing rate revealed a significant interaction between N/OFQ application and pre-treatment condition (F = 15.50, P = 0.002, ). There was no difference in the cell-type response to drug pre-treatment (likelihood ratio = 11.2, P = 0.26) and CORT and vehicle conditions did not differ in terms of mean (t = 0.97, P = 0.34) or mode (t = 0.17, P = 0.87) interspike interval.
Figure 6. Representative frequency histograms illustrating the effect of nociceptin (75 nM, N/OFQ) on neuronal activity in the ventral BNST following pre-treatment with either (a) Vehicle (0.005% DMSO in aCSF) or (b) 100 nM CORT. Vehicle or CORT were perfused for a 20 min time period and N/OFQ responses were tested 2 h later. (c) Pre-treatment of ventral BNST neurones with 100-nm CORT fully attenuated the inhibitory effect of nociceptin (75 nM) on the mean firing rate of cells when compared with those pre-treated with vehicle (0.005% DMSO in aCSF), (n = 5–10/group) *P < 0.05.
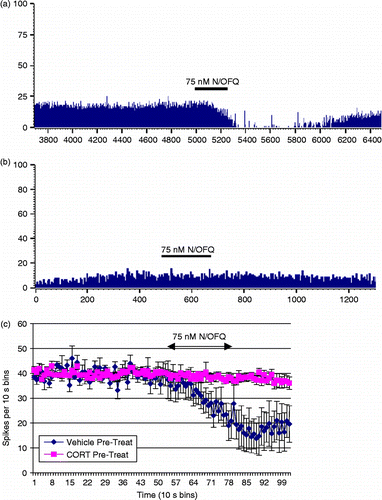
Discussion
Stress responsive neurotransmitter and neuropeptide circuits converge in the BNST, a limbic structure considered to be pivotal in the integration and processing of salient inputs. N/OFQ has been demonstrated to attenuate CRF function in the BNST, and N/OFQ-CRF interactions may be important for normal adaptive responses to stress and anxiety. This is the first electrophysiological study to directly examine the response to N/OFQ in the BNST, and we have demonstrated that N/OFQ elicits strong inhibition of BNST neuronal firing mediated via activation of NOP receptors. In addition, we have shown that the major stress hormone, CORT, attenuates N/OFQ-mediated inhibition. This has relevance in understanding the mechanism of action of N/OFQ in the BNST, a highly organised and prominent region within the extended amygdala. Our results provide important new evidence for this peptide's role in BNST neuromodulation, which has relevance in understanding the adaptive responses to stress.
Neuronal recordings were made in the BNST following application of NMDA (10 μm) to the aCSF perfusion fluid to elicit background firing in our slice preparation. Previous research confirmed that NMDA-receptor-mediated excitatory postsynaptic potentials (EPSPs) can be recorded in BNST cells (Egli and Winder Citation2003). Although we did not directly investigate the plasticity mechanisms (LTP or long-term depression, LTD) in this study, our ability to record neuronal firing under long-lasting and stable conditions in the presence of NMDA was necessary for the purposes of experimentation. There was no evidence, however, that N/OFQ-mediated responses were influenced by the effects of NMDA on synaptic potentiation. EPSPs recorded from cells in the dorsal BNST and ventral BNST do show paired pulse facilitation, a type of short-term plasticity (Egil and Winder Citation2003). However, this group suggests that the extent of facilitation at more extended interpulse intervals is not considerable. In addition, under our experimental conditions involving recording of spike activity in the presence of continuous NMDA (10 μm), the requirements for synaptic potentiation are unlikely to be met, given that the latter requires very precisely patterned input and stimulation parameters across time. Further research would be required to directly investigate whether there is an interaction between N/OFQ and synaptic plasticity mechanisms in BNST. This could potentially involve a role for both NMDA and non-NMDA glutamate receptors in BNST subregions.
In our single-unit recording experiments, we identified four different types of cells that could be distinguished by their firing pattern characteristics, whereas other studies in rats conducted using whole cell patch clamp recordings discerned three physiological types of cells in the dorsal anterolateral BNST (Hammack et al. Citation2007). Although the majority of cells that we recorded in the BNST were continuously firing cells, it is significant that we also showed evidence of bursting characteristics of neurones in the BNST, with such cells being found in both dorsal and ventral compartments. Given the high concentration of several major neuropeptides in the BNST, a distribution of bursting-type cells would be consistent with a prominent role for peptidergic neurotransmission within this complex. Although they share many similar basic electrophysiological characteristics, dorsal vs. ventral BNST neurones in male mice have been shown to differ in terms of certain properties of excitability, e.g. inward rectification (Egli and Winder Citation2003). BNST cells have also been differentiated on the basis of a low-threshold spike that is conspicuous in the ventral BNST, and has been suggested to underpin bursting properties of the cells (Egli and Winder Citation2003). Furthermore, dorsal vs. ventrally located cells differ in tonic GABAergic inputs, with dorsal BNST displaying significant GABAA-dependent regulation (Egli and Winder Citation2003).
The dense distribution of NOP expression (Neal et al. Citation1999b) in the BNST is consistent with our observation that 53% of neurones recorded were responsive to N/OFQ peptide. In response to N/OFQ application in vitro, a large proportion of cells recorded in either the dorsal (64%) or ventral (49%) anterolateral BNST were robustly inhibited by N/OFQ peptide (75 nM), although there was no significant difference in the duration or magnitude of the inhibition between the BNST subregions. N/OFQ had similarly robust inhibitory effects on each of the type of cell recorded in the present study. Further investigations would be required to exactly determine how N/OFQ may regulate the intrinsic currents and other biophysical properties in the subdivisions of BNST. It is noteworthy that following NMDA withdrawal, the majority of recorded BNST neurones displayed a lower firing rate. There was a significant correlation between the degree of loss of excitation owing to NMDA withdrawal and N/OFQ-induced inhibition, perhaps suggesting that NOP receptors may be present on NMDA responsive interneurones in the BNST.
In the ventromedial and arcuate nuclei of the hypothalamus, N/OFQ and NOP-receptor activation have been shown to exert inhibitory effects over neuronal excitability in vitro, involving presynaptic suppression of glutamatergic mechanisms in addition to membrane hyperpolarisation and activation of inwardly rectifying K+ conductances (Emmerson and Miller Citation1999). Assuming a similar mechanism in the BNST, functional inhibition of anterior BNST glutamatergic neurones by N/OFQ has a potential significance for stress responses in vivo, given that there is evidence for a role of BNST glutamate receptors in fear-elicited behaviours (Walker and Davis Citation1997) and synaptic plasticity (Weitlauf et al. Citation2004). N/OFQ-induced anxiolysis or stress-adaptive responses could be dependent on the suppression of glutamatergic neurotransmission. Nociceptin-mediated suppression of glutamatergic function in vivo could provide a critical endogenous restraint over excitatory neurotransmission in limbic-hypothalamic circuits.
In our studies, we determined that the duration of N/OFQ-dependent inhibition of neuronal firing rate was attenuated in the presence of the selective NOP antagonist, UFP-101, despite no difference in the magnitude of inhibition. Longer-acting, non-peptidic NOP antagonists may be useful in the further characterisation of the N/OFQ-dependent effects on BNST neurones to confirm the extent of involvement of NOP receptors. Interestingly, although we saw significant attenuation of N/OFQ-dependent actions using NOP antagonist treatment, we found no evidence for UFP-101-dependent effects per se on BNST neuronal firing. This suggests that under the experimental conditions employed in the present study, NOP receptors are not endogenously active in the BNST, potentially ruling out a major role for N/OFQ in tonic inhibition of BNST cells, although in vivo studies would be required to further address this possibility. In keeping with the latter premise, our recent evidence from stress-induced regulation of HPA axis activity suggests that following acute stressors, central nociceptinergic tone in vivo is enhanced (Leggett et al. Citation2007, Citation2009).
Glucocorticoid regulation in the anteroventral BNST has significance in understanding the effects of glucocorticoids in vivo. The BNST has been shown to regulate conditioned fear responses in rats, and our evidence for CORT-mediated attenuation of N/OFQ action in vitro is one mechanism by which fear-conditioned memory consolidation could be modulated. N/OFQ is known to inhibit memory functions in a range of learning and memory tasks, and therefore, glucocorticoid-mediated attenuation of N/OFQ function could be important for the maintenance of memory during emotionally arousing situations. We can only speculate on the neurones subject to regulation by glucocorticoids, and it is perhaps important to note that CRF neurones in the lateral BNST possess glucocorticoid receptors (Cintra et al. Citation1987; Lechner and Valentino Citation1999). Therefore, it is possible that the population of anteroventral neurones subject to inhibition by N/OFQ may include a CRF-containing/glucocorticoid-receptor-expressing subpopulation. Although we did not determine whether genomic or non-genomic mechanisms subserve the effect of glucocorticoids in our in vitro system, the brief application of glucocorticoids (20 min), followed by a 2 h delay, could engage genomic mechanisms of steroid action. The application of 100 nM CORT was used to mimic the effect of a high level of glucocorticoid, consistent with acute stress and associated occupation of glucocorticoid receptors. In future experiments, it will be important to investigate the potential for short-latency effects of glucocorticoid on N/OFQ responses in BNST neurones. Interestingly, we did not observe a significant effect of CORT application on baseline firing rate or firing pattern in BNST neurones. This contrasts with reports of modestly increased intrinsic excitability of principal neurones in the basolateral amygdala (BLA) in response to a relatively low (1 nM) or high (100 nM) CORT concentration (Duvarci and Pare Citation2007). Glucocorticoids also increase calcium-channel conductances in principal BLA neurones in vitro (Karst et al. Citation2002). The application of NMDA (10 μm) to our slice preparations may have masked the potential for enhanced intrinsic excitability by CORT in the present experiment, or perhaps, this result represents an inherent difference in the electrophysiological properties of BLA vs. BNST neurones in vitro. Stress hormones are implicated in profound changes in synaptic remodelling and dendritic organisation in the amygdala and hippocampus, invoking site-specific changes that may be associated with comparative differences in the expression of glucocorticoid receptors (van Eekelen et al. Citation1991; see Duvarci and Pare Citation2007). The emergent findings clearly indicate that further research is needed to fully discriminate the limbic regulatory roles of glucocorticoids in complex regions, such as the BNST and extended amygdala.
Previous research has highlighted the subregional differences in phenotypic characterisation and cytoarchitecture of neurones within the BNST. The complex afferent and efferent connections of the BNST region are reflective of its importance in the information processing of visceromotor and neuroendocrine responses. The central amygdala sends dense fibres via the ventral stria terminalis precommissural pathway to the lateral and medial compartments of the anterior BNST (Dong et al. Citation2001). In addition to the widespread innervation to BNST from the central amygdala, the medial amygdala also innervates many BNST cellular groups, and to a significant extent, the terminal fields of the central and medial amygdala axons overlap within the BNST (Dong et al. Citation2001). A rich supply of PVN afferents originating in the lateral BNST provides a tonic excitatory influence as evidenced from bilateral BNST lesions using neurotoxic ibotenic acid that suppress ACTH and CORT secretion in association with a context-conditioned emotional response (Gray et al. Citation1993). Both the central and medial amygdaloid nuclei contain N/OFQergic perikarya (Neal et al. Citation1999a) that release N/OFQ peptide from their terminals in the BNST, providing a source of N/OFQ able to activate local NOP receptors and potentially inhibit function of BNST neurones. In response to stress, increased firing of central amygdala neurones would elicit peptide release in the BNST, including N/OFQ. In addition, in association with immobilisation stress, extracellular levels of noradrenaline and its major metabolite, dihydroxyphenylglycol, are significantly increased in the BNST, implicating stress-induced activation of BNST noradrenergic afferents arising in the brainstem (Pacak et al. Citation1995). As N/OFQ suppressed the neuronal activity in dorsal and ventral compartments of anterior BNST, this suggests that this neuropeptide serves an important neuromodulatory role over BNST functions. This is consistent with the behavioural data indicating a close interaction between CRF and nociceptin in this region (Ciccocioppo et al. Citation2003; Rodi et al. Citation2008). Taken together, our evidence strengthens the neuroanatomical basis for a stress-integrative role of N/OFQ and we propose that the interaction between N/OFQ and glucocorticoids in the BNST, in particular, is essential for normal adaptive neuroendocrine and behavioural stress responses.
Acknowledgements
This work was supported by the Wellcome Trust, UK. Karen Dawe (KLD) was in receipt of BBSRC Animal Sciences Committee Studentship. KLD's current address: Department of Social Medicine, University of Bristol, Canynge Hall, 39 Whatley Road, Bristol BS8 2PS, U.K.
Declaration of interest: The authors report no conflicts of interest. The authors alone are responsible for the content and writing of the paper.
References
- Choi DC, Furay AR, Evanson NK, Ostrander MM, Ulrich-Lai YM, Herman JP. 2007. Bed nucleus of the stria terminalis subregions differentially regulate hypothalamo-pituitary-adrenal axis activity: Implications for the integration of limbic inputs. J Neurosci. 27:2025–2034.
- Cintra A, Fuxe K, Härfstrand A, Agnati LF, Wikström AC, Okret S, Vale W, Gustafsson JA. 1987. Presence of glucocorticoid receptor immunoreactivity in corticotrophin releasing factor and in growth hormone releasing factor immunoreactive neurones of the rat di- and telencephalon. Neurosci Lett. 77:25–30.
- Ciccocioppo R, Fedeli A, Economidou D, Policani F, Weiss F, Massi M. 2003. The bed nucleus is a neuroanatomical substrate for the anorectic effect of corticotropin-releasing factor and for its reversal by nociceptin/orphanin FQ. J Neurosci. 23:9445–9451.
- Crestani CC, Alves FHF, Tavares RF, Correa FMA. 2009. Roles of the bed nucleus of the stria terminalis in the cardiovascular response to acute restraint stress in rats. Stress. 12:268–278.
- Cullinan WE, Herman JP, Watson SJ. 1993. Ventral subicular interaction with the hypothalamic paraventricular nucleus: Evidence for a relay in the bed nucleus of the stria terminalis. J Comp Neurol. 332:1–20.
- Dong HW, Petrovich GD, Swanson L. 2001. Topography of projections from amygdala to bed nuclei of the stria terminalis. Brain Res Rev. 38:192–246.
- Duvarci S, Pare D. 2007. Glucocorticoids enhance the excitability of principal basolateral amygdala neurones. J Neurosci. 27:4482–4491.
- Egli RE, Winder DG. 2003. Dorsal and ventral distribution of excitable and synaptic properties of neurons of the bed nucleus of the stria terminalis. J Neurophysiol. 90:405–414.
- Emmerson PJ, Miller RJ. 1999. Pre- and postsynaptic actions of opioid and orphan opioid agonists in the rat arculate nucleus and ventromedial hypothalamus in vitro. J Physiol. 517:431–445.
- Feldman S, Conforti N, Saphier D. 1990. The preoptic area and bed nucleus of the stria terminalis are involved in the effects of the amygdala on adrenocortical secretion. Neuroscience. 37:775–779.
- Forray MI, Gysling K. 2004. Role of noradrenergic projections to the bed nucleus of the stria terminalis in the regulation of the hypothalamo-pituitary-adrenal axis. Brain Res Rev. 47:145–160.
- Gray TS, Piechowski RA, Yracheta JM, Rittenhouse PA, Bethea CL, Van de Kar LD. 1993. Ibotenic acid lesions in the bed nucleus of stria terminalis attenuates conditioned stress-induced increases in prolactin, ACTH and corticosterone. Neuroendocrinology. 57:517–524.
- Hammack SE, Mania I, Rainnie DG. 2007. Differential expression of intrinsic membrane currents in defined cell types of the anterolateral bed nucleus of the stria terminalis. J Neurophysiol. 98:638–656.
- Green MK, Barbieri EV, Brown BD, Chen KW, Devine DP. 2007. Roles of the bed nucleus of stria terminalis and of the amygdala in N/OFQ-mediated anxiety and HPA axis activation. Neuropeptides. 41:399–410.
- Ju G, Swanson LW. 1989. Studies on the cellular architecture of the bed nuclei of the stria terminalis in the rat: I. Cytoarchitecture. J Comp Neurol. 280:587–602.
- Ju G, Swanson LW, Simerly RB. 1989. Studies on the cellular architecture of the bed nuclei of the stria terminalis in the rat: II. Chemoarchitecture. J Comp Neurol. 280:603–621.
- Karst H, Nair S, Velzing E, Rumpff-van Essen L, Slagter E, Shinnick-Gallagher P, Joëls M. 2002. Glucocorticoids alter calcium conductances and calcium channel subunit expression in basolateral amygdala neurons. Eur J Neurosci. 16:1083–1089.
- Lechner SM, Valentino RJ. 1999. Glucocorticoid receptor-immunoreactivity in corticotrophin-releasing factor afferents to the locus coeruleus. Brain Res. 816:17–28.
- Leggett JD, Harbuz MS, Jessop DS, Fulford AJ. 2006. The nociceptin receptor antagonist UFP-101 blocks the stimulatory effects of nociceptin on the HPA axis. Neurosci. 141:2051–2057.
- Leggett JD, Jessop DS, Fulford AJ. 2007. The nociceptin/orphanin FQ antagonist UFP-101 differentially modulates the glucocorticoid response to restraint stress in rats during the peak and nadir phases of the HPA axis circadian rhythm. Neuroscience. 147:757–764.
- Leggett JD, Dawe KL, Jessop DS, Fulford AJ. 2009. Endogenous Nociceptin/Orphanin FQ system involvement in HPA axis responses: Relevance to models of inflammation. J Neuroendocrinology. 21:888–897.
- Meis S. 2003. Nociceptin/orphanin FQ: Actions within the brain. Neuroscientist. 9:158–168.
- Mulders WH, Meek J, Hafmans TG, Cools AR. 1997. Plasticity in the stress-regulating circuit; decreased input from the bed nucleus of the stria terminalis to the hypothalamic paraventricular nucleus in Wistar rats following adrenalectomy. Eur J Neurosci. 9:2462–2471.
- Neal CR, Akil H, Watson SJ. Expression of orphanin FQ and the opioid receptor-like (ORL1) receptor in the developing human and rat brain. J Chem Neuroanat. 1999a; 22:219–249.
- Neal CR, Mansour A, Reinscheid R, Nothacker H-P, Civelli O, Watson SJ. Localisation of orphanin FQ (nociceptin) peptide and messenger RNA in the central nervous system of the rat. J Comp Neurol. 1999b; 406:503–547.
- Pacak K, McCarty R, Palkovits M, Kopin IJ, Goldstein DS. 1995. Effects of immobilisation on in vivo release of norepinephrine in the bed nucleus of the stria terminalis in conscious rats. Brain Res. 688:242–246.
- Paxinos G, Watson C. 1997. The rat brain in stereotaxic coordinates. San Diego, USA: Academic Press Inc.
- Rodi D, Zucchini S, Simonato M, Cifani C, Massi M, Polidori C. 2008. Functional antagonism between nociceptin/orphanin FQ (N/OFQ) and corticotrophin-releasing factor (CRF) in the rat brain: Evidence for involvement of the bed nucleus of the stria terminalis. Psychopharmacology. 196:523–531.
- Sakanaka M, Shibasaki T, Lederis K. 1986. Distribution and efferent projections of corticotrophin-releasing factor-like immunoreactivity in the rat amygdaloid complex. Brain Res. 382:213–238.
- Shimada S, Inagaki S, Kubota Y, Ogawa N, Shibasaki T, Takagi H. 1989. Coexistence of peptides (corticotropin releasing factor/neurotensin and substance P/somatostatin) in the bed nucleus of the stria terminalis and central amygdaloid nucleus of the rat. Neuroscience. 30:377–383.
- Shirasaka T, Miyahara S, Takasaki M, Kannan H. 2001. Nociceptin/orphanin FQ and [Phe(1)psi(CH2-NH)Gly2]nociceptin(1-13)NH2 modulates the activity of hypothalamic paraventricular nucleus neurones in vitro. Brain Res. 890:147–153.
- van Eekelen JA, Bohn MC, de Kloet ER. 1991. Postnatal ontogeny of mineralocorticoid and glucocorticoid receptor gene expression in regions of the rat tel- and diencephalon. Brain Res Dev Brain Res. 61:33–43.
- Walker DL, Davis M. 1997. Double dissociation between the involvement of the bed nucleus of the stria terminalis and the central nucleus of the amygdala in startle increases produced by conditioned versus unconditioned fear. J Neurosci. 17:9375–9383.
- Walter A, Mai JK, Lanta L, Gorcs T. 1991. Differential distribution of immunohistochemical markers in the bed nucleus of the stria terminalis in the human brain. J Chem Neuroanat. 4:281–298.
- Weitlauf C, Egli RE, Grueter BA, Winder DG. 2004. High-frequency stimulation induces ethanol-sensitive long-term potentiation at glutamatergic synapses in the dorsolateral bed nucleus of the stria terminalis. J Neurosci. 24:5741–5747.
- Woulfe JM, Flumerfelt BA, Hrycyshyn AW. 1990. Efferent connections of the A1 noradrenergic cell group: A DBH immunohistochemical and PHA-L anterograde tracing study. Exp Neurol. 109:308–322.
- Yu T-P, Xie C-W. 1998. Orphanin FQ/nociceptin inhibits synaptic transmission and long-term potentiation in rat dentate gyrus through postsynaptic mechanisms. J Neurophysiol. 80:1277–1284.