Abstract
Dehydroepiandrosterone (DHEA) and DHEA sulfate (DHEAS) are anabolic prehormones involved in the synthesis of testosterone. Both have been shown to exert neuroprotective effects during stress. In this randomized, controlled, double-blind field study, we examined the effects of a 12-day DHEA regimen on stress indices in military men undergoing survival training. Forty-eight men were randomized to either a DHEA treatment group or placebo control group. The treatment group received 50 mg of oral DHEA supplementation daily for 5 days during classroom training followed by 7 days of 75 mg during stressful field operations. Control subjects received identical placebo pills. Salivary assays (DHEA[S], testosterone, and cortisol) were conducted at four time points: distal pre-stress (T1), proximal pre-stress (T2), mock-captivity stress (T3), and 24 h recovery (T4). Subjective distress was also assessed at T1, T3, and T4. As expected, DHEA treatment resulted in higher salivary concentrations of DHEA and DHEAS during daily living, mock-captivity stress, and recovery. Similar patterns were observed for salivary markers of anabolic balance: DHEA/cortisol, DHEAS/cortisol, and testosterone/cortisol concentration ratios. Despite notable time effects, no group differences emerged for subjective distress. A brief, low dose DHEA regimen yielded large increases in salivary DHEA(S) concentrations and enhanced anabolic balance throughout sustained military stress. These physiological changes did not extrapolate to subjective distress.
Introduction
It is well known that stress leads to distinct physiological changes. Acute exposure to a stressor leads to autonomic nervous system activation in particular altering heart rate and blood vessel tone via sympathetic innervation, and the activation of the hypothalamic–pituitary–adrenal (HPA) axis (Mason Citation1968). The stress hormone cortisol is a primary end product of HPA stimulation and is responsible for mobilizing blood glucose for energy and increasing blood pressure in support of the “fight or flight” response. Chronically high cortisol levels, however, can have adverse neural consequences including hippocampal atrophy (Sapolsky Citation2000, Citation2003), decreased neurogenesis, and impaired memory (Bremner Citation2006a,Citationb).
Dehydroepiandrosterone (DHEA) and its sulfated version, DHEA sulfate (DHEAS), hereafter referred to collectively as DHEA(S), are co-secreted with cortisol from the adrenal gland (Baileau and Robel Citation1998) and serve as precursors for anabolic steroids (Friess et al. Citation2000). Although their respective mechanisms of action are not fully understood (Maninger et al. Citation2009), plausible biological effects include neuroprotection (Kaasik et al. Citation2001; Fiore et al. Citation2004), stimulation of neurite growth (Compagnone and Mellon Citation1998) and neurogenesis (Karishma and Herbert Citation2002). In addition, it is believed that DHEA(S) has antagonistic effects on oxidants (Bastianetto et al. Citation1999), inflammatory markers (Chen and Parker Citation2004), and glucocorticoids (e.g. cortisol) (Hu et al. Citation2000; Karishma and Herbert Citation2002). In light of the antiglucocorticoid relationship, the DHEA(S)–cortisol ratio is a widely accepted indicator of “anabolic balance”. This term reflects the ratio of anabolic to catabolic hormones which may, in turn, indicate susceptibility to disease, stress, or aging (Goodyer et al. Citation1998; Morgan et al. Citation2004). That is, although DHEA(S) concentrations tend to decline under such conditions, cortisol concentrations generally either increase or do not change. Since anabolic and catabolic hormones regulate each other and may produce “net effects”, the ratio of their levels represents an important addition to measures of either one independently (Carvalhaes-Neto et al. Citation2003).
Current research also explores clinical applications of DHEA(S) (Kaminska et al. Citation2000; Eser et al. Citation2006), inoculation effects of DHEA(S) during sustained stress (Morgan et al. Citation2004; Taylor et al. Citation2007), as well as possible relationships to posttraumatic stress disorder (PTSD; Rasmusson et al. Citation2004; Butterfield et al. Citation2005). For example, several studies have found inverse associations between DHEA(S) and depression (Barrett-Connor et al. Citation1999; Morsink et al. Citation2007), whereas another work has shown that the DHEA–cortisol ratio discriminates depressed from non-depressed individuals better than the level of either hormone alone (Assies et al. Citation2004). By contrast, higher DHEA(S) concentrations and DHEAS–cortisol ratios are associated with panic disorders (Fava et al. Citation1989; Eser et al. Citation2006). Inconsistently, DHEA(S) values have been linked to schizophrenia (Ritsner et al. Citation2006), dementia (Bernardi et al. Citation2000) as well as functional abilities in the elderly. Interestingly, consistent positive relationships have been observed between DHEA(S) (or DHEAS–cortisol) level and PTSD (Pico-Alfonso et al. Citation2004; Butterfield et al. Citation2005). However, several researchers have suggested that higher levels of DHEAS in PTSD patients may actually reflect healthy responses to treatment (i.e. salutatory effects) (Rasmusson et al. Citation2004; Yehuda et al. Citation2006). Other work has documented increased DHEAS concentrations under sustained military stress along with buffering of acute stress symptoms and performance maintenance (Morgan et al. Citation2004; Taylor et al. Citation2007). Morgan and colleagues (Citation2004), for instance, examined DHEAS responses to military survival training and showed that plasma DHEAS–salivary cortisol ratios were higher in participants who reported fewer symptoms of peritraumatic dissociation and demonstrated superior military performance. Subsequently, Taylor et al. (Citation2007) showed that salivary DHEAS concentrations associated with better performance in a low-intensity mock captivity challenge during survival training, but not a high-intensity challenge. More recently, Morgan et al. (Citation2009) have shown that plasma DHEA(S) was positively correlated with improved performance and fewer dissociative symptoms in a stressful underwater navigation examination in military members enrolled in a combat diver qualification course.
Not surprisingly, this correlative literature has inspired a series of studies exploring the effects of exogenous DHEA. In brief, clinical trials have shown varying beneficial effects of DHEA supplementation on depressive symptoms (Wolkowitz et al. Citation1999), PTSD (Sageman and Brown Citation2006), dementia (Wolkowitz et al. Citation2003), and neuropsychiatric comorbidities in a variety of medical conditions (Arlt et al. Citation1999, Citation2000; Maninger et al. Citation2009). Depending on the endpoint and population in question, various behavioral effects have been demonstrated with doses as low as 25 mg and as high as 450 mg. Likewise, effects have been shown in some studies within a few days (Sageman and Brown Citation2006), yet in others effects have been evident only after several months of treatment (Balieu et al. Citation2000) or not at all (Barnhart et al. Citation1999; Nair et al. Citation2006). A few studies have also shown beneficial effects in healthy individuals (Morales et al. Citation1994; Kudielka et al. Citation1998), typically with dose ranges of 50–150 mg/day. Morales et al. (Citation1994), for example, administered a 3-month DHEA regimen (50 mg/day) to healthy middle-aged and elderly subjects in a randomized, controlled, double-blind crossover design. They observed significant increase in plasma DHEA(S) concentrations within 2 weeks, as well as multiple behavioral improvements including better sleep, improved mood, and improved ability to handle stressful events. In another double-blind study (Kudielka et al. Citation1998), a 2-week DHEA regimen (50 mg/day) resulted in improved well-being in elderly women but not men. However, a double-blind, controlled crossover study of healthy young men receiving a 7-day course of oral DHEA (150 mg) led to improvements in episodic memory and mood—as well as reductions in evening cortisol levels (Alhaj et al. Citation2006). Other controlled studies have shown no discernible benefits of DHEA supplementation, even when the interventions are long term (Barnhart et al. Citation1999; Nair et al. Citation2006).
Altogether, the current literature suggests several clinical applications of DHEA(S), a protective role of DHEA(S) during intense stress, as well as relationships with PTSD. This work, however, is restricted by discrepancies owing possibly to methodological inconsistencies (e.g. dosing and length of treatment), small sample sizes, as well as a persistent focus on phenomenological outcomes. Maninger et al. (Citation2009) suggest that a strict phenomenological approach fails to fully capitalize on recent advances in understanding DHEA(S)' mechanisms of action. They recommend instead that future DHEA studies include biomarkers of neuroprotection, neurogenesis, oxidative stress, or neurophysiology. In the present study, we assessed the effects of DHEA supplementation on stress indices in military men undergoing intense survival training. Key endpoints included physiologic markers of neuroprotection and anabolic balance, as well as subjective distress. We hypothesized that DHEA supplementation would enhance salivary concentrations of DHEA(S) and anabolic balance both during daily living and in response to intense stress. We also hypothesized that DHEA supplementation would buffer subjective distress in response to survival training.
Materials and methods
Military survival training
Survival, Evasion, Resistance, and Escape (SERE) training is described in earlier reports (Taylor et al. Citation2007, Citation2009) and some portions of the curriculum are classified. In brief, US military members who are deemed to be at “high risk of capture” are required to attend this course, which includes a period of mock-captivity. After an initial phase of classroom-based didactic training (5 days), students were taken to a field training site where they were trained in SERE techniques (7 days). Training tasks include evasion from a simulated enemy and, upon eventual “capture”, students must practice resistance to various forms of simulated exploitation in stressful mock-captivity training challenges. The entire course lasts 12 days. Because SERE is designed in part to simulate a captivity experience, it offers an unusual medium to prospectively evaluate the effects of highly realistic stress on human functioning. Recent studies confirm its validity as a sustained stressor, as evidenced by substantial alterations in both physiological and self-report indices (Morgan et al. Citation2000; Taylor et al. Citation2007). Moreover, the SERE environment boasts a high degree of ecological validity with respect to military operations.
Participants
Subjects included 48 healthy, male active duty Navy and Marine Corps personnel (13 officers and 35 enlisted men). All subjects underwent medical and psychological screening by the SERE medical officer prior to enrollment in survival training. Examples of criteria for exclusion from SERE training include endocrine, renal, cardiovascular, psychological, or musculoskeletal disorders. Subjects who were deemed medically fit to undergo SERE training and who were enrolled in the SERE course were thus considered eligible for the current study, with two exceptions: females were excluded owing to slightly increased health risks associated with DHEA supplementation, as were individuals who had been taking DHEA or any anabolic supplement within the past 3 months. Those who expressed an interest in participating attended an in-person meeting to review the details of the study and provided written informed consent. This protocol was approved by the Naval Aerospace Medical Research Laboratory Institutional Review Board.
Overview of protocol
As shown in , subjects completed baseline salivary hormone assessments and a self-report measure of dissociative symptoms on the first day of the academic phase of SERE training (T1; distal pre-stress) and were then randomized to either a DHEA supplementation group (n = 24) or control group (n = 24). Only the principal investigator and the medical monitor were aware of group assignments. DHEA and control groups did not differ with respect to age, education, body mass index, years of military service, baseline subjective distress, or baseline salivary hormone concentrations (p>0.05). Salivary hormone assessments and the measure of dissociative symptoms were completed again on the last (fifth) day of academic training (T2; proximal pre-stress). Subsequently, all subjects experienced a rigorous evasion exercise, after which they participated in a highly realistic mock-captivity scenario. Salivary hormones and dissociative symptoms were assessed again directly after a stressful mock-captivity event (T3; mock-captivity stress). Finally, approximately 24 h after release from mock-captivity (which marked completion of training), all subjects completed the hormone assessment and measure of dissociative symptoms again, as well as a measure of psychological impact of mock-captivity (T4; recovery).
DHEA and placebo regimen
The first DHEA and placebo regimens began on Day 1 of SERE training. Subjects were given 50 mg capsules, sufficient for 5 days, in a small plastic container, to be self-administered once per day through the end of the classroom phase (Day 5). The pill containers consisted of five separate mini-containers labeled by day of week (i.e. Monday, Tuesday, etc.) for all 5 days. Each daily regimen was pre-placed in the daily mini-containers. Research staff oversaw the first self-administration. Subjects were then instructed to self-administer subsequent daily capsules (supplements or placebo) independently. Research personnel made one daily telephone call and/or sent one daily text message to each participant's cell phone requesting confirmation that he had taken the capsule. If applicable, the participant was asked to return the phone call or text message providing confirmation once the daily regimen was consumed. The daily phone call or text message was placed no later than 11:00 h each day.
Upon transition to the field on Day 5, subjects were given a pill container with 75 mg capsules (DHEA or placebo) for 7 days: sufficient to complete the regimen through Day 12 (the final day of SERE training). Participants were instructed to self-administer according to the same daily regimen while in the field. At some point during the evasion exercise, each subject was “captured”. At this point, the supplement container was taken from the subject prior to entering the mock-captivity scenario. During mock-captivity, a member of the research staff assumed responsibility for administering the daily regimen through Day 12. On the basis of a count of remaining pills, mean ± SEM adherence to the DHEA regimen was estimated at 97.8 ± 0.01% which did not differ from compliance of subjects in the placebo group (99.7 ± 0.01%; p>0.05).
Distal pre-stress (T1) assessments
Subjects provided an initial salivary sample using the passive drool technique (Granger et al. Citation2007) between 11:45 and 12:47 h on the first day of the classroom phase of SERE training (prior to beginning the DHEA or placebo regimen). Each participant was asked to rinse his mouth with water approximately 10 min prior to sample collection and to avoid the following: brushing teeth prior to collection, using salivary stimulants (e.g. chewing gum, lemon drops), and consuming acidic or high-sugar foods within 20 min prior to collection. After data collection, all samples were immediately placed on dry ice and transferred to Salimetrics, LLC (State College, PA, USA) for storage and processing.
Dhea And Dheas
All samples were assayed for salivary DHEA in duplicate using a highly sensitive enzyme immunoassay (Salimetrics, LLC). The test used 50 μl of saliva per determination, lower limit of sensitivity was 5 pg/ml, standard curve ranged from 10.2 to 1000 pg/ml, average intra-assay coefficient of variation was 5.6% and average inter-assay coefficient was 8.2%. Method accuracy determined by spike recovery averaged 102.2% and linearity determined by serial dilution averaged 106.9%. The serum–saliva correlation for DHEA in a combined male/female normative database (Salimetrics, LLC) is high (r = 0.86, p < 0.0001, n = 39).
Similarly, samples were assayed for salivary DHEAS in duplicate using a highly sensitive enzyme immunoassay (Salimetrics, LLC). The test used 100 μl of saliva per determination, lower limit of sensitivity was 43 pg/ml, standard curve range from 189 to 15,300 pg/ml, average intra-assay coefficient of variation was 7.3% and an inter-assay coefficient of variation was 7.6%. Method accuracy determined by spike recovery averaged 105.9% and linearity determined by serial dilution averaged 98.2%.
Testosterone
Since DHEA is an anabolic precursor, all samples were also assayed for salivary testosterone. This was performed in duplicate using a highly sensitive enzyme immunoassay (Salimetrics, LLC). The test used 25 μl of saliva per determination, had a lower limit of sensitivity of 1.0 pg/ml, standard curve range from 6.1 to 600 pg/ml, an average intra-assay coefficient of variation of 4.6%, and an average inter-assay coefficient of variation of 9.8%. Method accuracy determined by spike recovery averaged 104.3% and linearity determined by serial dilution averaged 102.4%. Serum–saliva correlations from a normative database (Salimetrics, LLC) of male subjects are high (r = 0.91, p < 0.001, n = 26).
Cortisol
All samples were assayed for salivary cortisol in duplicate using a highly sensitive enzyme immunoassay (Salimetrics, LLC). The test used 25 μl of saliva per determination, had a lower limit of sensitivity of 0.003 μg/dl, standard curve range from 0.012 to 3.0 μg/dl, an average intra-assay coefficient of variation of 3.5%, and an average inter-assay coefficient of variation of 5.1%. Method accuracy determined by spike recovery averaged 100.8% and linearity determined by serial dilution averaged 91.7%. Serum–saliva correlations from a normative database (Salimetrics, LLC) show the expected strong linear relationship, (r = 0.91, p < 0.0001, n = 47).
Anabolic balance
As noted earlier, anabolic balance reflects the ratio of anabolic to catabolic hormones which may indicate susceptibility to disease, stress, or aging. In this study, we assessed three biomarkers of anabolic balance: DHEA–cortisol, DHEAS–cortisol, and testosterone–cortisol ratios.
Background questionnaire
This questionnaire assesses basic background and demographic information (e.g. age, ethnicity, military occupational specialty) as well as current use of prescription or over-the-counter drugs.
Subjective distress
The 19 self-report items from the Clinician-Administered Dissociative States Scale (CADSS; Bremner et al. Citation1998) were used to assess the frequency and intensity of state symptoms of dissociation. Although the CADSS includes additional items used for clinical observation, the set of 19 self-report items is a valid, reliable, and independent indicator of dissociative state symptoms (Morgan et al. Citation2004; Taylor et al. Citation2009). This scale is designed to assess how perceptually connected or disconnected an individual is relative to his or her environment. Examples of items include: “Did you feel as if you were watching the situation as an observer or spectator?” and “Did you space out or in some way lose track of what is going on?” The self-report items are rated on a Likert scale of 0 (not at all) to 4 (extremely), with a maximum possible score of 76. The mean CADSS scores are listed in . During T1, each subject was asked to respond with respect to what he is experiencing “right now”. Cronbach's α reliability for the CADSS at T1 was 0.91.
Table I. Participant* characteristics.
Proximal pre-stress assessments (T2)
A second saliva sample was collected between 11:25 and 12:09 h on Day 5, immediately prior to the subjects' transition from academic to field training. The purpose of this sample was to monitor changes in DHEA(S) resulting from the initial supplementation regimen and, in turn, to establish a new pre-stress “baseline”. Identical salivary collection procedures as described in T1 were performed.
Mock-captivity stress (T3) assessments
During the mock-captivity phase of training (after the evasion exercise) subjects were escorted to a secure area by the SERE staff. A third saliva sample was collected at this time using identical saliva collection procedures as described in T1. Also, the CADSS was administered a second time, in which subjects were asked to respond to each question with respect to a stressful mock-captivity event. Cronbach's α reliability for the CADSS at T2 was 0.93. Although logistical constraints associated with the SERE curriculum prevented our ability to match T3 data collection times to T1 and T2, all T3 data were collected across a 5-h time frame on the same day.
Recovery (T4) assessments
Approximately 24 h after the conclusion of SERE training, a final salivary hormone assessment was performed and a final CADSS was administered, again asking subjects to respond with respect to the mock-captivity event. Cronbach's α reliability for the CADSS at T4 was 0.87. At this time, the Impact of Event Scale—Revised (IESR) (Weiss and Marmar Citation1997) was also administered.
Impact of Event Scale: Revised
The IESR is a self-report measure designed to assess current subjective distress for any specific life event. It has three subscales comprising 22 items: avoidance (IESR-avoid; mean of eight items measuring the extent to which the respondent avoids situations that remind him or her of the stressful or traumatic event), intrusion (IESR-intrusion; mean of eight items assessing the extent to which one experiences intrusive thoughts), and hyperarousal (IESR-arousal; mean of six items measuring anger, irritability, heightened startle response, and hyperarousal). The total impact of events score (IESR-total) is the mean of all 22 items. We have also provided the sum of all items as well as the sum of subscales, which are two other common data reporting methods (Taylor et al. Citation2009). In the current study, respondents completed the IESR 24 h after the conclusion of survival training (T4). The IESR directions were modified to ask the participant to indicate how distressing each difficulty has been relative to mock-captivity on a scale of 0 (not at all) to 4 (extremely). Adequate reliability and predictive validity have been shown for this scale (Weiss and Marmar Citation1997), and Cronbach's α reliabilities in the present sample were 0.83, 0.78, and 0.90 for IESR-arousal, IESR-avoid, and IESR-intrusion, respectively. Cronbach's α reliability for IESR-total was 0.94. Mean IESR scores are provided in .
Data analysis
Data were analyzed using SPSS software Version 16 (SPSS, Inc., Chicago, IL, USA). Characteristics of the distributions for all dependent variables were examined to determine whether assumptions of normality were met (Leech et al. Citation2001). All hormonal and subjective distress variables were slightly to moderately positively skewed, upon which logarithmic transformations were performed to approximate normal distributions (Stuart-Hamilton Citation2007). Descriptive analyses were conducted (), and repeated-measures analyses of variance with post hoc paired t-tests were then used to examine differences across time and conditions for hormonal responses and dissociative symptoms, respectively. Lastly, independent samples t-tests compared DHEA and control groups relative to psychological impact of mock-captivity. All hypothesis tests were based on log-transformed data; untransformed means are reported for ease of interpretation, as were all percent change values [(value 2 − value 1/value 1) × 100%]. All hypothesis tests were two-sided and the probability of committing a type I error was set at 0.05, although p is reported when more stringent probabilities were achieved (p < 0.01 or p < 0.001). Bonferroni corrections were made for time-series comparisons (0.05/4 = 0.0125 for four comparisons; 0.05/3 = 0.017 for three comparisons). Overall effects sizes were estimated via partial η2 (O'Keefe Citation2007).
Results
Participant characteristics are shown in . Group effects for hormonal responses and dissociative symptoms are shown in , respectively.
Figure 2. Effects of DHEA supplementation on salivary concentrations of: (A) DHEA, (B) DHEAS, (C) testosterone, and (D) cortisol. DHEA-treated group (from T1: 50 mg/day; from T2: 75 mg/day; n = 24) and CONTROL group (placebo; n = 24). T1, distal pre-stress; T2, proximal pre-stress; T3, mock-captivity stress and T4, recovery. Data are mean ± SEM. Group effects are denoted above time series: *Bonferroni-corrected independent t-test p < 0.0125. Time effects are denoted below time series: #different from T1, Bonferroni-corrected dependent t-test p < 0.0125), + different from T3, Bonferroni-corrected dependent t-test p < 0.0125.
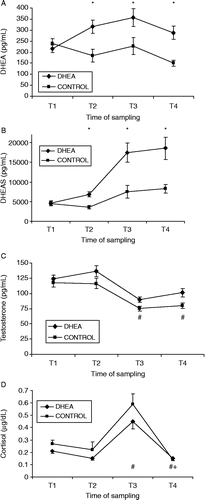
Figure 3. Effect of DHEA supplementation on dissociative symptoms during survival training. Dissociative symptoms (mean ± SEM) for DHEA-treated (DHEA; n = 24) and CONTROL (placebo; n = 24) groups. T1, distal pre-stress; T3, mock-captivity stress and T4, recovery. No significant group effects. Time effects are denoted below time series, (different from T1, Bonferroni-corrected dependent t-test p < 0.017, + different from T3, Bonferroni-corrected dependent t-test p < 0.017.
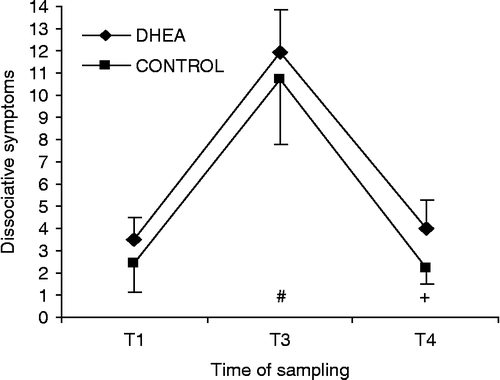
Salivary DHEA(S), testosterone, and cortisol concentrations
Salivary hormone responses to DHEA supplementation during survival training are shown in . A group × time interaction was demonstrated for salivary DHEA concentration (F = 8.6, p < 0.001, partial η2 = 0.16). Although DHEA values were not different at T1, the supplemented subjects possessed an average 72.1% higher concentration after 5 days of 50 mg supplementation than control subjects (T2; p < 0.0125), a 59.4% higher concentration during mock-captivity (T3; p < 0.0125), and a 92.0% higher concentration during recovery from SERE training (T4; p < 0.0125) (). A similar group × time interaction was observed for salivary DHEAS concentration (F = 6.9, p < 0.001, partial η2 = 0.13). DHEAS values did not differ between groups at T1, but DHEA subjects possessed an average 122.0% higher concentration after 5 days of 50 mg supplementation than control subjects (T2; p < 0.0125), a 132.5% higher concentration during mock-captivity (T3; p < 0.0125), and a 123.7% higher concentration during recovery from SERE training (T4; p < 0.0125) (). Stress-induced decrease in salivary testosterone concentration were evident (time main effect, F = 39.0, p < 0.001, partial η2 = 0.47). That is, irrespective of group assignment, testosterone values decreased during mock-captivity (T1 vs. T3; mean ± SEM percent Δ = − 27.1 ± 3.8% [p < 0.0125]) and remained suppressed at 24-h recovery (T1 vs. T4; mean ± SEM percent Δ = − 21.6 ± 3.9% [p < 0.0125]). In general, DHEA supplementation tended to buffer the testosterone stress response and enhance recovery (group main effect, F = 6.2, p < 0.05, partial η2 = 0.12). Post hoc analyses yielded noteworthy group differences at T2–T4, although these findings were rendered nonsignificant with Bonferroni correction (p > 0.0125; see ). Finally, profound stress-induced increases were noted in salivary cortisol concentration (time main effect, F = 67.9, p < 0.001, partial η2 = 0.60). Hence, irrespective of group assignment, cortisol values increased during mock-captivity (T1 vs. T3; mean ± SEM percent Δ = 156.7 ± 29.9% [p < 0.0125]) and then returned to pre-stress values at 24-h recovery (T3 vs. T4; mean ± SEM percent Δ = − 61.9 ± 3.8% [p < 0.0125]). No group differences, however, were observed in this analyte (p > 0.05) ().
Anabolic balance
A group × time interaction emerged for salivary DHEA/cortisol concentration (F = 5.0, p < 0.01, partial η2 = 0.10). That is, although DHEA/cortisol values did not differ at T1, the DHEA subjects possessed 118.4% higher ratios after 5 days of 50 mg supplementation than control subjects (T2; p < 0.0125), a 112.7% higher ratio during mock-captivity (T3; p < 0.0125), and a 89.1% higher ratio during recovery from SERE training (T4; p < 0.0125). A nearly identical group × time interaction was shown for salivary DHEAS/cortisol concentration (F = 3.8, p < 0.01, partial η2 = 0.08). Specifically, although DHEAS values did not differ at T1, the DHEA subjects showed 104.4% higher ratios after 5 days of 50 mg supplementation than control subjects (T2; p < 0.0125), a 203.2% higher ratio during mock-captivity (T3; p < 0.0125), and a 167.4% higher ratio during recovery from SERE training (T4; p < 0.0125). Finally, stress-induced decreases in salivary testosterone/cortisol concentration were demonstrated (time main effect, F = 102.4, p < 0.001, partial η2 = 0.70). Irrespective of group assignment, testosterone/cortisol concentration decreased during mock-captivity (T1 vs. T3 mean ± SEM percent Δ = − 57.1% ± 3.6 [p < 0.0125]). Overall, DHEA supplementation tended to mitigate this stress response (group main effect, F = 9.0, p < 0.01, partial η2 = 0.17). In post hoc comparisons, DHEA demonstrated 38.1% higher testosterone/cortisol concentration than control during mock-captivity (T3; p < 0.0125). Notable group differences were also observed at T2 although this finding was rendered nonsignificant with Bonferroni correction (p>0.0125).
Subjective distress
Despite considerable time effects for dissociative symptoms (F = 53.5, p < 0.001, partial η2 = 0.55) no discernible group effects emerged. Not considering group assignment, dissociative symptoms increased during mock-captivity stress (T1 vs. T3, p < 0.017) and then returned to pre-stress values at 24-h recovery (T3 vs. T4, p < 0.017) (). This analysis was then repeated after excluding subjects reporting no dissociative symptoms during mock-captivity stress (CADSS = 0, n = 3 [6.25%]), and again after excluding subjects with CADSS scores ≤ 5 (n = 17 [35.4%]). Essentially identical results prevailed in both cases. Percent change scores were not calculated on this variable because several subjects' T1 and T4 CADSS scores were zero (i.e. no dissociative symptoms), an expected finding under non-stressful conditions. Psychological impact scores (as measured by the IESR) are presented in . No group differences prevailed relative to IESR-total or for any of the subscales.
Discussion
In this study, we examined the effects of a DHEA manipulation on stress indices in military men undergoing rigorous survival training. As expected, DHEA treatment resulted in higher salivary concentrations of DHEA(S) during daily living, mock-captivity stress, and at 24 h recovery. Protective effects were also observed for key markers of anabolic balance, namely salivary concentration ratios for DHEA(S)/cortisol and testosterone/cortisol. Although survival training intensified subjective distress, DHEA supplementation did not appear to influence this effect.
DHEA supplementation led to large increases in salivary DHEA(S) concentrations. Supplementation substantially increased both analytes after only 5 days of treatment, and it enhanced their concentrations during mock-captivity and recovery. To our knowledge, this is the first exogenous DHEA intervention study in a realistic military context, although previous work has documented increases in endogenous DHEA(S) values during intense military training (Gomez-Merino et al. Citation2005; Taylor et al. Citation2007). Also, recent primate research suggests that DHEAS increases in response to both acute and chronic stress with less adaptation (desensitization) across time than for cortisol levels (Maninger et al. Citation2010). Finally, a double-blind, crossover, controlled clinical trial has examined effects of DHEA supplementation at rest and during exercise stress (a known activator of the HPA axis) (Deuster et al. Citation2005). In that study, Deuster and colleagues observed higher resting plasma DHEA concentrations in healthy men after 2 weeks of DHEA treatment (100 mg/day) as well as enhanced DHEA responses to a 20-min treadmill exercise protocol. Clearly, DHEA supplementation appreciably enhances endogenous levels within days and augments the DHEA stress response even with modest dosing.
Consistent with prior research in the military setting (Morgan et al. Citation2000; Gomez-Merino et al. Citation2005; Nindl et al. Citation2007), salivary testosterone concentrations decreased during mock-captivity. DHEA supplementation tended to buffer this stress effect and enhance recovery, although small effect sizes were noted and follow-up comparisons were rendered nonsignificant upon correction for multiple comparisons. With some notable exceptions, most studies from the human and animal literature describe a suppressive effect of various stressors on testosterone (Chichinadze and Chichinadze Citation2008). Mechanistically, it is believed that glucocorticoid (e.g. cortisol) elevations during stress inhibit testosterone production, particularly during chronic stress (Hardy et al. Citation2005). By extension, purported antiglucocorticoid effects of DHEA (Cardounel et al. Citation1999) may counter this effect, thereby preserving testosterone concentrations during stress. DHEA supplementation, then, may not boost testosterone concentrations exclusively by virtue of its role as an anabolic precursor. Indeed, previous studies have documented increases in endogenous DHEA(S) concentrations due to supplementation in the absence of discernible alterations in testosterone levels (Deuster et al. Citation2005). These relationships almost certainly depend on moderating factors such as dosing and length of treatment. Also, certain variants of DHEA (e.g. 7-keto DHEA) are not aromatized to testosterone and may also possess superior antiglucocorticoid properties (Sageman and Brown Citation2006). Such treatment options may be worth considering in light of possible health risks of high testosterone levels across the lifespan, particularly in older age (Pierorazio et al. Citation2010). The current data do raise the possibility that DHEA administration may buffer operational stress reactions in military personnel by preventing declines in testosterone levels. This effect may be larger with a larger dose of DHEA, which is a distinct possibility that future research will clarify.
As an end product of HPA stimulation underlying the so-called fight-or-flight response, cortisol is a well-studied, gold standard indicator of physiological stress. Profound cortisol responses to SERE training were observed, thus validating the stressful nature of this context. This coincides with prior studies conducted in nearly identical military settings (Morgan et al. Citation2000; Taylor et al. Citation2007). Here, DHEA supplementation did not modulate cortisol concentrations during daily living or the cortisol response to sustained stress. This resonates with previous research suggesting that DHEA may not alter cortisol concentrations per se (Labrie et al. Citation1997) but rather may counteract glucocorticoid function at the receptor level (Karishma and Herbert Citation2002). In contrast, other research suggests that DHEA directly alters cortisol concentrations. For example, one study revealed an effect of 7 days of DHEA treatment (300 mg per day) on reduced evening (trough) cortisol levels in healthy young men (Alhaj et al. Citation2006). Another study of perimenopausal women showed decreases in cortisol concentrations after 3 months of daily 50 mg DHEA treatment (Barnhart et al. Citation1999). Evidently, a full understanding of the DHEA–cortisol interface and resultant net effects awaits further study.
The present findings show that DHEA supplementation augments anabolic balance and thus may enhance neuroprotection during intense stress. Specifically, DHEA supplementation led to higher DHEA(S)–cortisol ratios after 5 days, during mock-captivity and recovery, as well as higher testosterone–cortisol ratios during mock-captivity. Cross-sectional research has shown relationships between DHEAS–cortisol and metabolic syndrome in veterans from the war in Vietnam such that higher DHEAS level relative to cortisol appears to be protective against the disease. In addition, a prospective study in this same cohort found that DHEAS–cortisol ratios were protective against all-cause disease mortality (Boscarino Citation2008). Clearly, anabolic balance is an important biomarker of stress, illness, and disease. These data suggest that DHEA supplementation fortifies anabolic balance not only during daily living but also in response to intense stress in healthy young men.
Military stress resulted in notable increases in dissociative symptoms and most subjects still experienced some psychological effects of mock-captivity 24 h after the conclusion of SERE training. This corroborates prior findings in similar contexts (Morgan et al. Citation2004; Eid and Morgan Citation2006; Taylor et al. Citation2007). Contrary to expectation, however, DHEA treatment did not alter these effects. These findings were essentially unchanged when the analyses were repeated excluding nonresponders, which indicate robustness of the result. In previous work, Morgan et al. (Citation2004) studied endogenous DHEA concentrations during SERE training and found inverse relationships to dissociative symptoms. Taylor et al. (Citation2007) observed no link between salivary DHEAS concentrations and dissociative symptoms, but did show an inverse relationship between DHEAS and psychological impact of mock-captivity. It is important to recognize that neither the salivary DHEAS level nor the dissociative response to military stress in the current study was as pronounced as that shown in Morgan et al. (Citation2004) or Taylor et al. (Citation2007). Therefore, subjects in this current study may not have been exposed to an identical stress load as that observed in prior work. Also, some authors have suggested that overt subjective/psychological benefits of DHEA administration may take up to several months to develop (Balieu et al. Citation2000). However, in a double-blind, controlled crossover study of healthy young men receiving a 7-day course of oral DHEA (at a relatively high dose of 300 mg), improvements were observed in episodic memory and mood (Alhaj et al. Citation2006). Other controlled studies, though, have shown no discernible benefits of DHEA supplementation even when the interventions are long term (Barnhart et al. Citation1999; Nair et al. Citation2006). It is quite possible that the low dose and/or brief regimen utilized in the present study were inadequate to appreciably affect subjective distress. Future studies are warranted to examine the effects of higher DHEA doses on subjective stress indices both during daily living and in response to stress.
This study has several limitations which should be considered. As noted above, the DHEA dose was relatively low (50–75 mg) and the treatment regimen was brief. However, these data assist in the quest to deduce effective dose ranges that may elicit behavioral effects in this unique setting. For example, if an inverted-U or catastrophe model is hypothesized, the current findings provide support for the left (low dose) side of the models. Also, the study was conducted in a field setting which precludes the experimental control typically conferred by laboratory settings. This is counter-balanced to some degree by the ecological validity afforded by the military survival training context. In addition, direct oversight of DHEA administration was not provided by research staff for some portions of the DHEA regimen. Finally, logistical constraints associated with the SERE curriculum prevented our ability to perfectly match T3 data collection times to T1, T2, and T4. However, all T3 data were collected across a 5-h time frame on the same day. Although hormonal concentrations may normally be sensitive to discrepant sampling times during free living, current research suggests that sustained military stress tends to extinguish the normal diurnal rhythm (Opstad Citation1992). More research is needed to better understand the effects of DHEA supplementation on the human stress response, ideally in ecologically valid settings with dependent measures that capitalize on advances in basic science (Maninger et al. Citation2009). Undoubtedly, dosing is a critical issue given that inverted-U relationships of DHEA supplementation to neuroprotection have been observed. Although low concentrations can be neuroprotective, high concentrations have been shown to be ineffective or neurotoxic (Bologa et al. Citation1987; Kimonides et al. Citation1999). Similarly, more work is needed to determine whether there is a threshold at which subjective stress effects of DHEA supplementation appear. Also, it would be of interest to examine 7-keto DHEA as well as other compounds which do not convert to testosterone and thus may not carry the health risks associated with increased concentrations of that steroid.
In this randomized controlled field study, DHEA treatment resulted in higher salivary concentrations of DHEA(S) during daily living, mock-captivity stress and at 24 h recovery in military men undergoing survival training. Protective effects were also observed for key biomarkers of anabolic balance. Although survival training led to subjective distress, DHEA supplementation did not influence this effect. It may well be that the dose was too low to permit an impact on subjective reports. Future studies, then, are needed to fully characterize the effects of DHEA on both physiological and subjective stress indices in this unique setting, along with the moderating effects of dose and length of intervention.
Acknowledgements
This study was supported by a grant from the Office of Naval Research Force Health Protection Program. Appreciation is extended to students and staff at Center for Security Forces—Detachment North Island for “training the best for the worst”. We appreciate Mrs. Renee Kotch for editorial assistance, as well as Drs. Nicole Maninger and Owen Wolkowitz for so graciously sharing their subject matter expertise. Finally, we wish to thank Dr. Charles “Andy” Morgan III for his pioneering military stress research and his expert guidance.
Declaration of interest: The authors report no conflicts of interest. The authors alone are responsible for the content and writing of the paper.
Sources of support: This study was supported by a grant from the Office of Naval Research Force Health Protection Program. This work was performed under work unit number PB401.
Disclaimer: The views expressed in this article are those of the authors and do not necessarily reflect the official policy or position of the Department of the Navy, Department of Defense, nor the US Government.
Human Research Protections/IRB statement: The study protocol was approved by the Naval Aerospace Medical Research Laboratory Institutional Review Board in compliance with all applicable Federal regulations governing the protection of human subjects.
Copyright statement: I am a military service member. This work was prepared as part of my official duties. Title 17 U.S.C. §105 provides that ‘Copyright protection under this title is not available for any work of the United States Government.’ Title 17 U.S.C. §101 defines a US Government work as a work prepared by a military service member or employee of the US Government as part of that person's official duties.
Distribution statement: Approved for public release; distribution is unlimited.
References
- Alhaj HA, Massey AE, McAllistair-Williams RH. 2006. Effects of DHEA administration on episodic memory, cortisol and mood in healthy young men: A double-blind, placebo controlled study. Psychopharmacology. 188:541–551.
- Arlt W, Callies F, van Vlijmen JC, Koehler I, Reincke M, Bidlingmaier M, Huebler D, Oettel M, Ernst M, Schulte HM, Allolio B. 1999. Dehydroepiandrosterone replacement in women with adrenal insufficiency. N Engl J Med. 341:1013–1020.
- Arlt W, Callies F, Allolio B. 2000. DHEA replacement in women with adrenal insufficiency: Pharmocokinetics, bioconversion and clinical effects on well-being, sexuality, and cognition. Endocr Res. 26:505–511.
- Assies J, Visser I, Nicolson NA, Eggelte TA, Wekking EM, Huyser J, Lieverse R, Shene AH. 2004. Elevated salivary dehydroepiandrosterone-sulfate but normal cortisol levels in medicated depressed patients: Preliminary findings. Psychiatry Res. 128:117–122.
- Barnhart KT, Freeman E, Grisso JA, Rader DJ, Sammel M, Kapoor S, Nestler JE. 1999. The effect of dehydroepiandrosterone supplementation to symptomatic perimenopausal women on serum endocrine profiles, lipid parameters and health-related quality of life. J Clin Endocrinol Metab. 84:3896–3902.
- Barrett-Connor E, von Muhlen D, Laughlin GA, Kripke A. 1999. Endogenous levels of dehydroepiandrosterone sulfate, but not other sex hormones are associated with depressed mood in older women: The Rancho Bernardo Story. J Am Geriatr Soc. 47:685–691.
- Bastianetto S, Ramassamy C, Poirier J, Quiron R. 1999. Dehydroepiandronsterone (DHEA) protects hippocampal cells from oxidative stress-induced damage. Brain Res Mol Brain Res. 66:35–41.
- Baulieu EE, Robel P. 1998. Dehydroepiandrosterone (DHEA) and dehydroepiandrosterone sulfate (DHEAS) as neuroactive neurosteroids. Proc Natl Acad Sci U S A. 95:4089–4091.
- Baulieu EE, Thomas G, Legrain S, Lahlou N, Roger M, Debuire B, Faucounau V, Girard L, Hervy MP, Latour F, Leaud MC, Mokrane A, Pitti-Ferrandi H, Trivalle C, de Lacharriere O, Nouveau S, Rakoto-Arison B, Souberbielle JC, Raison J, Le Bouc Y, Raynaud A, Giered X, Forette F. 2000. Dehydroepiandrosterone (DHEA), DHEA sulfate, and aging: Contribution of the DHEAge study to a sociobiomedical issue. Proc Natl Acad Sci U S A. 97:4279–4284.
- Bernardi F, Lanzone A, Cento RM, Spada RS, Pezzani I, Genazzani AD, Luisi S, Luisi M, Petraglia G, Genazzani AR. 2000. Allopregnenolone and dehydroepiandrosterone response to corticotropin-releasing factor in patients suffering from Alzheimer's disease and vascular dementia. Eur J Endocrinol. 142:466–471.
- Bologa L, Sharma J, Roberts E. 1987. Dehydroepiandrosterone and its sulfated derivative reduce neuronal death and enhance astrocytic differentiation in brain cell cultures. J Neurosci Res. 17:225–234.
- Boscarino JA. 2008. A prospective study of PTSD and early-age heart disease mortality among Vietnam veterans: Implications for surveillance and prevention. Psychosom Med. 70:668–676.
- Bremner JD. Stress and brain atrophy. CNS Neurol Disord Drug Targets. 2006a; 5:503–512.
- Bremner JD. Traumatic stress: Effects on the brain. Dialogues Clin Neurosci. 2006b; 8:445–461.
- Bremner JD, Krystal JH, Putman FW, Southwick SM, Marmar C, Charney DS, Mazure CM. 1998. Measurement of dissociative states with the Clinician-Administered Dissociative States Scale (CADSS). J Trauma Stress. 11:125–136.
- Butterfield MI, Stechuchak KM, Connor KM, Davidson JR, Wang C, MacKuen CL, Pearlstein AM, Marx CE. 2005. Neuroactive steroids and suicidality in posttraumatic stress disorder. Am J Psychiatry. 162:380–382.
- Cardounel A, Regelson W, Kalimi M. 1999. Dehydroepiandrosterone protects hippocampal neurons against neurotoxin-induced cell death: Mechanism of action. Proc Soc Exp Biol Med. 222:145–149.
- Carvalhaes-Neto N, Huayllas MK, Ramos LR, Cendoroglo MS, Kater CE. 2003. Cortisol, DHEAS, and aging: Resistance to cortisol suppression in frail institutionalized elderly. J Endocrinol Invest. 26:17–22.
- Chen CC, Parker CRJr. 2004. Adrenal androgens and the immune system. Semin Reprod Med. 22:369–377.
- Chichinadze K, Chichinadze N. 2008. Stress-induced increase of testosterone: Contributions of social status and sympathetic reactivity. Physiol Behav. 94:595–603.
- Compagnone NA, Mellon SH. 1998. Dehydroepiandronsterone: A potential signaling molecule for neurocortical organization during development. Proc Natl Acad Sci U S A. 95:4678–4683.
- Deuster PA, Faraday MM, Chrousos GP, Poth MA. 2005. Effects of dehydroepiandrosterone and alprazolam on hypothalamic–pituitary responses to exercise. J Clin Endocrinol Metab. 90:4777–4783.
- Eid J, Morgan C. 2006. Dissociation, hardiness, and performance in military cadets participating in survival training. Mil Med. 171:436–442.
- Eser D, Schule C, Romeo E, Baghai TC, di Michele F, Pasini A, Zwanzger P, Padberg F, Rupprecht R. 2006. Neuropsychopharmacological properties of neuroactive steroids in depression and anxiety disorders. Psychopharmacology (Berl). 186:373–387.
- Fava M, Rosenbaum JF, MacLaughlin RA, Tesar GE, Pollack MH, Cohen LS, Hirsch M. 1989. Dehydroepiandrosterone-sulfate/cortisol ratio in panic disorder. Psychiatry Res. 28:345–350.
- Fiore C, Inman DM, Hirose S, Noble LJ, Igarashi T, Compagnone NA. 2004. Treatment with the neurosteroid dehydroepiandrosterone promotes recovery of motor behavior after moderate contusive spinal cord injury in the mouse. J Neurosci Res. 75:391–400.
- Friess E, Schiffelholz T, Steckler T, Steiger A. 2000. Dehydroepiandrosterone: A neurosteroid. Eur J Clin Invest. 30:46–50.
- Gomez-Merino D, Drogou C, Chennaoui M, Tiollier E, Mathieu J, Guezzennec CY. 2005. Effects of combined stress during intense training on cellular immunity, hormones and respiratory infections. Neuroimmunomodulation. 12:164–172.
- Goodyer IM, Herbert J, Altham PM. 1998. Adrenal steroid secretion and major depression in 8- to 16-year-olds, III. Influence of cortisol/DHEA ratio at presentation on subsequent rates of disappointing life events and persistent major depression. Psychol Med. 28:265–273.
- Granger DA, Kivlighan KT, Fortunato C, Harmon AG, Hibel LC, Schwartz EB, Whembolua GL. 2007. Integration of salivary biomarkers into developmental and behaviorally-oriented research: Problems and solutions for collecting specimens. Physiol Behav. 92 4: 583–590.
- Hardy MP, Gao HB, Dong Q, Wang Q, Chai WR, Feng X, Sottas C. 2005. Stress hormone and male reproductive function. Cell Tissue Res. 322:147–153.
- Hu Y, Cardounel A, Gursoy E, Anderson P, Kalimi M. 2000. Anti-stress effects of dehydroepiandrosterone: Protection of rats against repeated immobilization stress-induced weight loss, glucocorticoid receptor production, and lipid peroxidation. Biochem Pharmacol. 59:753–762.
- Kaasik A, Kalda A, Jaako K, Zharkovsky A. 2001. Dehydroepiandrosterone sulphate prevents oxygen–glucose deprivation-induced injury in cerebellar granule cell culture. Neuroscience. 102:427–432.
- Kaminska M, Harris J, Gijsbers K, Dubrovsky B. 2000. Dehydroepiandrosterone sulfate (DHEAS) counteracts decremental effects of corticosterone on dentate gyrus LTP. Implications for depression. Brain Res Bull. 52:229–234.
- Karishma KK, Herbert J. 2002. Dehydroepiandrosterone (DHEA) stimulates neurogenesis in the hippocampus of the rat, promotes survival of newly formed neurons and prevents corticosterone-induced suppression. Eur J Neurosci. 16:445–453.
- Kimonides VG, Spillantini MG, Sofroniew MV, Fawcett JW, Herbert J. 1999. Dehydroepiandrosterone antagonizes the neurotoxic effects of corticosterone and translocation of stress-activated protein kinase 3 in hippocampal primary cultures. Neuroscience. 89:429–436.
- Kudielka BM, Hellhammer J, Hellhammer DH, Wolf OT, Pirke KM, Varadi E, Pilz J, Kirschbaum C. 1998. Sex differences in endocrine and psychological responses to psychosocial stress in healthy elderly subjects and the impact of a 2-week dehydroepiandrosterone treatment. J Clin Endocrinol Metab. 83:1756–1761.
- Labrie F, Diamond P, Cusan L, Gomez JL, Belanger A, Candas B. 1997. Effect of 12-month dehydroepiandrosterone replacement therapy on bone, vagina, and endometrium in postmenopausal women. J Clin Endocrinol Metab. 82:3498–3505.
- Leech NL, Barrett KC, Morgan GA. 2001. SPSS for intermediate statistics. New York: Routledge.
- Maninger N, Wolkowitz OM, Reus VI, Epel ES, Mellon SH. 2009. Neurobiological and neuropsychiatric effects of dehydroepiandrosterone (DHEA) and dehydroepiandrosterone sulfate (DHEAS). Front Neuroendocrinol. 30:65–91.
- Maninger N, Capitanio JP, Mason WA, Ruys JD, Mendoza SP. 2010. Acute and chronic stress increase DHEAS concentrations in rhesus monkeys. Psychoneuroendocrinology. 35:1055–1062.
- Mason JW. 1968. Organization of psychoendocrine mechanisms. Psychosom Med. 30:565–808.
- Morales AJ, Nolan JJ, Nelson JC, Yen SS. 1994. Effects of replacement dose of dehydroepiandrosterone in men and women of advancing age. J Clin Endocrinol Metab. 78:1360–1367.
- Morgan CAIII, Wang S, Mason J, Southwick SM, Fox P, Hazlett G, Charney DS, Greenfield G. 2000. Hormone profiles in humans experiencing military survival training. Biol Psychiatry. 47:891–901.
- Morgan CAIII, Southwick S, Hazlett G, Rasmusson A, Hoyt G, Zimolo Z, Charney D. 2004. Relationships among plasma dehydroepiandrosterone sulfate and cortisol levels, symptoms of dissociation, and objective performance in humans exposed to acute stress. Arch Gen Psychiatry. 61:819–825.
- Morgan CAIII, Rasmusson A, Pietrzak RH, Coric V, Southwick SM. 2009. Relationships among plasma dehydroepiandrosterone and dehydroepiandrosterone sulfate, cortisol, symptoms of dissociation, and objective performance in humans exposed to underwater navigation stress. Biol Psychiatry. 66:334–340.
- Morsink LF, Vogelzangs N, Nicklas BJ, Beekman AT, Satterfield S, Rubin SM, Yaffe K, Simonsick E, Newman AB, Kritchevsky SB, Penninx BW, Health ABC Study. 2007. Associations between sex steroid hormone levels and depressive symptoms in elderly men and women: Results from the Health ABC study. Psychoneuroendocrinology. 32:874–883.
- Nair KS, Rizza RA, O'Brien P, Dhatariya K, Short KR, Nehra A, Vittone JL, Klee GG, Basu A, Basu R, Cobelli C, Toffolo G, Dalla Man C, Tindall DJ, Melton LJ 3rd, Smith GE, Khosla S, Jensen MD. 2006. DHEA in elderly women and DHEA or testosterone in elderly men. N Engl J Med. 355:1647–1659.
- Nindl BC, Barnes BR, Alemany JA, Frykman PN, Shippee RL, Friedl KE. 2007. Physiological consequences of US army ranger training. Med Sci Sports Exerc. 39:1380–1387.
- O'Keefe DJ. 2007. Post hoc power, observed power, a priori power, retrospective power, prospective power, achieved power: Sorting out appropriate uses of statistical power analyses. Commun Methods Meas. 1:291–299.
- Opstad PK. 1992. Androgenic hormones during prolonged physical stress, sleep, and energy deficiency. J Clin Endocrinol Metab. 74:1176–1183.
- Pico-Alfonso MA, Garcia-Linares MI, Celda-Navarro N, Herbert J, Martinez M. 2004. Changes in cortisol and dehydroepiandrosterone in women victims of physical and psychological intimate partner violence. Biol Psychiatry. 56:233–240.
- Pierorazio PM, Ferruci L, Kettermann A, Longo DL, Metter EJ, Carter HB. 2010. Serum testosterone is associated with aggressive prostate cancer in older men: Results from the Baltimore Longitudinal Study of Aging. BJU Int. 105:824–829.
- Rasmusson AM, Vasek J, Lipschitz DS, Vojvoda D, Mustone ME, Shi Q, Gudmundsen G, Morgan CA, Wolfe J, Charney DS. 2004. An increased capacity for adrenal DHEA release is associated with decreased avoidance and negative mood in women with PTSD. Neuropsychopharmacology. 29:1546–1557.
- Ritsner MS, Gibel A, Ratner Y, Tsinovoy G, Strous RD. 2006. Improvement of sustained attention and visual and movement skills, but not clinical symptoms, after dehydroepiandrosterone augmentation in schizophrenia: A randomized, double-blind, placebo controlled, crossover trial. J Clin Psychopharmacol. 26:495–499.
- Sageman S, Brown RP. 2006. 3-acetyl-7-oxo-dehydroepiandrosterone for healing treatment-resistant posttraumatic stress disorder in women: 5 case reports. J Clin Psychiatry. 67:493–496.
- Sapolsky RM. 2000. Glucocorticoids and hippocampal atrophy in neuropsychiatric disorders. Arch Gen Psychiatry. 57:925–935.
- Sapolsky RM. 2003. Stress and plasticity in the limbic system. Neurochem Res. 28:1735–1742.
- Stuart-Hamilton I. 2007. Dictionary of psychological testing, assessment and treatment. London: Jessica Kingsley Publishers.
- Taylor MK, Sausen KP, Potterat EG, Mujica-Parodi LR, Reis JR, Markham AE, Padilla GA, Taylor DL. 2007. Stressful military training: Endocrine reactivity, performance and psychological impact. Aviat Space Environ Med. 78:1143–1149.
- Taylor MK, Mujica-Parodi LR, Padilla GA, Markham AE, Potterat EG, Momen N, Sander TC, Larson GE. 2009. Behavioral predictors of acute stress symptoms during intense military training. J Trauma Stress. 22:212–217.
- Weiss D, Marmar C. 1997. The impact of event scale: Revised. In: Wilson J, Keane T. editors. Assessing psychological trauma and PTSD. New York: Guilford.
- Wolkowitz OM, Reus VI, Keebler A, Nelson N, Friedland M, Brizendine L, Roberts E. 1999. Double-blind treatment of major depression with dehydroepiandrosterone (DHEA). Am J Psychiatry. 156:646–649.
- Wolkowitz OM, Kramer JH, Reus VI, Costa MM, Yaffe K, Walton P, Raskind M, Peskind E, Newhouse P, Sack D, De Souza E, Sadowsky C, Roberts E, Alzheimer's Disease Collaborative Research. 2003. DHEA treatment of Alzheimer's disease: A randomized, double-blind, placebo-controlled study. Neurology. 60:1071–1076.
- Yehuda R, Brand SR, Golier JA, Yang RK. 2006. Clinical correlates of DHEA associated with posttraumatic stress disorder. Acta Psychiatr Scand. 114:187–193.