Abstract
Recent prospective studies have shown that gestational stress in humans is more likely to cause cognitive and emotional problems in the offspring if it occurs during weeks 12–20 of pregnancy. There are also suggestions that such problems may be gender dependent. This review describes recent studies that found sex differences in the behaviour and brain morphology of rats stressed prenatally during the equivalent period of neuronal development in humans. Learning deficits are more prevalent in males and anxious behaviour in females but their appearance depends also on the timing and intensity of the stress and the age when the offspring were tested. Cognitive deficits and anxiety are linked to a sex-dependent reduction in neurogenesis and in measures of dendritic morphology in the prefrontal cortex and hippocampal formation. Maternal adrenalectomy prior to the stress prevents the anxiety in both sexes and learning deficits in males. Corticosterone administration to the dam to mimic levels induced by stress reinstates only the anxiety, indicating that it arises from foetal exposure to corticosterone from the maternal circulation. Learning deficits in males may result from a combination of a reduction in testosterone and in aromatase activity, together with the action of other adrenal hormones.
Introduction
Until the last 5 years, our information about the possible effects of prenatal stress on offspring behaviour in humans has mainly been obtained from retrospective studies (reviewed in Weinstock Citation2001, Citation2008). Recently, data published from prospective clinical studies have suggested that the behavioural outcome in the offspring of maternal stress depends on the time during gestation that it occurs and the gender of the offspring, with some changes being more prevalent in males and others in females. This review will describe the recent studies in humans and will detail experiments in rat models that support the role of the time of the stress in relation to neuronal development and the presence of sex differences in behaviour. The underlying brain morphology and hormonal changes that may mediate them will be discussed.
Recent findings of behavioural changes induced by prenatal stress in humans
In prospective studies in humans, the presence of stress has been implied either from reports of chronic anxiety arising from psychosocial problems (Talge et al. Citation2007; Van den Bergh et al. Citation2008; Beijers et al. Citation2010; Martini et al. Citation2010) or from objective indications of stress arising from well-documented natural disasters, like a freezing ice storm or hurricane (Kinney et al. Citation2008; Laplante et al. Citation2008). In addition to emotional problems, anxiety, depressive states and schizophrenia described in earlier retrospective studies (reviewed in Weinstock Citation2001, Citation2008; Charil et al. Citation2010), recent prospective studies indicate an incidence of attention-deficit hyperactivity disorder (ADHD; Grizenko et al. Citation2008; Kim et al. Citation2009; Li et al. Citation2010), autism (Kinney et al. Citation2008), impaired learning and language ability (King and Laplante Citation2005; Laplante et al. Citation2008) in children or adolescents in whom prenatal stress has been documented. Some of the new findings also suggest that gestational stress may have a different impact on male and female offspring (de Bruijn et al. Citation2009; Li et al. Citation2010; Van Lieshout and Boylan Citation2010). ADHD (Rodriguez and Bohlin Citation2005; Li et al. Citation2010) and possibly schizophrenia of early onset (Thorup et al. Citation2007) appear to be more prevalent in males, whereas depression and anxiety states are more commonly seen in females (Van den Bergh et al. Citation2008; Van Lieshout and Boylan Citation2010).
Attempts have been made to link offspring behaviour to the timing and severity of the maternal stress. Two-year-old offspring of mothers exposed to a moderate degree of stress in the ice storm showed some enhancement of the development of cognitive ability, whereas those whose mothers were exposed to more severe stress showed a degree of cognitive retardation (DiPietro et al. Citation2006). Delayed cognitive development after more severe objective stress during the first trimester, indicated by lower full-scale IQ's and language abilities, was also seen in a follow-up study at 5.6 years of age (Laplante et al. Citation2008) and by others after perceived pregnancy-related anxiety (Davis and Sandman Citation2010). In 15-year-old adolescents of both sexes from mothers experiencing a high level of anxiety during weeks 12–22 of gestation, a faster reaction but with more errors was reported in a matching-to-sample task (Van den Bergh et al. Citation2005). In another study from this group, high maternal antenatal anxiety, but not that of low to moderate levels, was associated with a decreased performance when the cognitive load of the task was increased (Mennes et al. Citation2006). Although examination of the data suggests that the decrement may have been greater in boys than in girls, no statistical evaluation for sex was included. This group also reported that maternal perceived stress at weeks 12–22 of gestation proved to be a better predictor of anxiety in 8–9-year-old children than perceived stress at weeks 32–40 (Van den Bergh and Marcoen Citation2004). Early maternal stress also increased the incidence of ADHD (Van den Bergh and Marcoen Citation2004) and schizophrenia (Khashan et al. Citation2008). By contrast, autism has been associated with maternal stress during 25–28 weeks of gestation (Beversdorf et al. Citation2005). A reduction in grey matter volume was found in several cortical areas of children whose mothers were stressed at week 19 but not at week 25 or 32 (Buss et al. Citation2010).
Since there are clear sex differences in normal brain morphology both during development (Munoz-Cueto et al. Citation1990; Konkle and McCarthy Citation2010) and in adulthood (Madeira and Lieberman Citation1995; Andrade et al. Citation2000; Garcia-Falgueras et al. Citation2005), it is reasonable to expect that maternal stress may also induce different behavioural and structural changes in males and females according to the timing and severity of the stress. However, there is insufficient information from the existing studies to enable us to link the timing of the maternal stress to differences in the behavioural outcomes in boys and girls. We await the planned MRI measurement on the children from the Ice Storm project (Charil et al. Citation2010) and hope that they will provide information about any differences between boys and girls. In order to be able to compare changes induced by prenatal stress in humans and rats and the possibility of sex differences, the following sections describe brain development and sex differences in the structure and function of the areas of the limbic system.
Development of the brain in humans and rats
Although gross development of brain regions is similar in rats and humans, with the hindbrain developing before the forebrain areas, the timeline in rats is measured in days (length of gestation 21 days) and in humans in weeks to months (Bayer et al. Citation1993). In humans, neurogenesis begins in the 5th week of pregnancy in the amygdala and several cortical areas and continues until about week 20. Cell migration is followed by proliferation, differentiation, synaptogenesis, apoptosis and myelination.
In the rat, neurogenesis begins in the amygdala and hypothalamus on day 12, in various cortical regions on day 14, in hippocampal fields CA1–3 on day 15, the dentate gyrus (DG) granule cells on day 19, and continues until birth on day 21 or, in the DG, until post-natal day 19 (Rice and Barone Citation2000). Myelination in the rat brain begins before birth and continues up to 45 days of age; gliogenesis starts in the embryonic period and also continues until adolescence (Rice and Barone Citation2000).
The number of synapses, comprising pre- and post-synaptic elements, continues to increase during the first weeks of life in rats and during childhood through adolescence in humans. This information is important for those attempting to model in rats the changes found in humans and will help us to understand how a potential disturbance induced by maternal stress and/or inadequate maternal care can induce particular behavioural changes by affecting specific various brain regions. In most, but not all rat studies, the dams were stressed during the period of day 14–21 of gestation, which is roughly equivalent to the 6th–15th week in humans.
Functional and anatomical sex differences in the limbic system
The sex differences that underlie the performance of spatial learning in humans and rodents are related to different strategies used by each sex in the performance of these tasks (Sandstrom et al. Citation1998; Newhouse et al. Citation2007). Several brain regions may participate in the execution of these strategies, including the medial prefrontal cortex (de Bruin et al. Citation2001) and the hippocampal formation which is particularly involved in spatial navigation guided by distal cues to which females tend to respond better (Blokland et al. Citation2006). Fear-related behaviour, spatial learning and the control of the hypothalamic–pituitary–adrenal (HPA) axis are dependent on the appropriate neural connections between cortical areas, the hippocampal formation, amygdala and hypothalamus (Madeira and Lieberman Citation1995), the structure and function of which differ in males and females.
Hippocampal formation and prefrontal cortex
The hippocampal formation comprises the DG, CA1, CA2, CA3, CA4, subicular complex and entorhinal cortex. The DG is larger in adult males than in females, because it contains a higher number of granule cells (Madeira and Lieberman Citation1995). This sex difference becomes apparent in rats on post-natal day 27 and results from the action of testosterone during the perinatal period (Wimer and Wimer Citation1989), since it is abolished by neonatal castration of males (Juraska et al. Citation1985). The total number of CA1 neurons is also smaller in females than in males but there appears to be no differences in the size of the dendritic trees of CA1 pyramidal cells. Adult female rats have a larger number of dendritic spines and more complex branching than males in the apical dendrites in the CA3 pyramidal region (Gould et al. Citation1990). The attainment of a normal number of dendritic spines is dependent on adequate levels of testosterone in males (Meyer et al. Citation1978) and of oestradiol and progesterone in females (Woolley and McEwen Citation1992). Oestradiol can increase the density of spines of both apical and basal trees of CA1 pyramidal cells and mediates the fluctuations in synapse density during the oestrous cycle (Woolley and McEwen Citation1992). This is how oestradiol can increase activity-dependent synaptic processes known as long-term potentiation (LTP; Warren et al. Citation1995) that are critical for spatial learning and memory (Bliss and Collingridge Citation1993). Furthermore, oestradiol increases NMDA receptor binding in parallel with increased synaptic spine density (Woolley et al. Citation1997). Interestingly, external factors like environmental enrichment have a larger influence on dendritic complexity in females than in males (Juraska et al. Citation1985).
The majority of comparative studies on dendritic morphology in male and female rats have been conducted on the hippocampal formation. Nevertheless, sex differences have also been found in dendritic spine morphology in the layer V pyramidal neurones in the cerebral cortex. At 20–40 days of age, the number of spines on apical dendrites was found to be higher in females than in males. In females, the number of spines reached a peak at 20 days of age and then declined, whereas those of males increased to achieve the same numbers as of females at 60 days of age (Munoz-Cueto et al. Citation1990). The cortex in the male rat develops more slowly than that in the female (Gregory Citation1975). The delay in male cortical development could be due to the formation of oestradiol from testosterone at a time when the capacity of cortical cells is enhanced to accumulate oestradiol that can inhibit cortical growth (Munoz-Cueto et al. Citation1990).
Role of testosterone and aromatase in mediating sexual dimorphic behaviour
The developing male brain is exposed to testosterone from its own testis. In the rat, this hormone reaches peak levels on day 18 of gestation and then declines, except for about 2 h after birth. Oestradiol concentrations are similar in males and females in the foetal hippocampus on day 19 of gestation. So also are concentrations of testosterone after which they rapidly fall to their basal levels on post-natal days 10–12 (Konkle and McCarthy Citation2010). The activity of CYP450 aromatase during critical periods of development in the rat is essential for the defeminisation and masculinisation of the male brain (Sakuma Citation2009). Aromatase converts testosterone to oestradiol which is responsible for sexual dimorphism in behaviour (Johnston and File Citation1991). Since it was found that the removal of the adrenals and gonads at birth did not further reduce the oestradiol or testosterone content of these brain areas on post-natal day 3, it appears that local steroidogenesis can maintain brain levels of these hormones (Konkle and McCarthy Citation2010).
Some forms of prenatal stress like thrice daily maternal restraint decrease brain aromatase activity in the brain of male and female rat foetuses (Weisz et al. Citation1982) and plasma levels of testosterone in males (Gerardin et al. Citation2005; Richardson et al. Citation2006; Rodriguez et al. Citation2007). These and other hormonal changes could, therefore, contribute to the alterations in brain structure and behaviour induced by prenatal stress.
Changes in behaviour induced by prenatal stress in rats
The majority of studies on the effect of prenatal stress in rats performed over the past 40 years have examined only the male offspring (see reviews by Archer and Blackman Citation1971; Weinstock Citation1997, Citation2007, Citation2008; Kofman Citation2002; Maccari et al. Citation2003; Lazinski et al. Citation2008; Markham and Koenig Citation2011). Most of these have used thrice daily immobilisation or restraint during the last week of gestation, since this was originally used in the pioneering work of Ward and her colleagues (Ward Citation1972). More recent studies have used shorter periods of restraint or varied forms of maternal stress during different periods of time in the last week of gestation and have found clear sex-related effects. The following sections will describe recent experimental data from our group and others that have attempted to elucidate such structural and hormonal changes in rats that may explain the behavioural alterations in the offspring.
Learning and memory
Like those in humans, the behavioural changes most frequently reported in prenatally stressed (PS) rats are learning and memory impairment and heightened anxiety in a novel or stressful environment (Weinstock Citation2007, Citation2008). Experiments designed to detect changes in spatial learning and memory in the Morris water maze (MWM) in adult rats have produced inconsistent results, even when the same strain of rat (Sprague-Dawley) and stress paradigm (thrice daily restraint during the last week of gestation) were used. For example, in two studies in which control males learned the task more quickly than females, prenatal stress improved spatial learning in females but had no effect in males thereby abolishing the sex difference (Bowman et al. Citation2004; Zuena et al. Citation2008). In another study, learning was impaired by prenatal stress in juvenile rats of both sexes, but in adulthood, only in females (Wu et al. Citation2007). There is no obvious explanation for the inconsistency in the data on spatial learning after the use of this maternal stress paradigm but it may arise from a difference in the design of the spatial memory test, including the size of the pool, 1.6–2.5 m (Szuran et al. Citation2000; Yang et al. Citation2006), number of trials the rats were given, from two daily (Zagron and Weinstock Citation2006) to two sessions of five daily trials (Wu et al. Citation2007) and the temperature of the water, ranging from 20 to 25°C (Szuran et al. Citation2000; Zuena et al. Citation2008), which has been shown to affect the rate of learning (Salehi et al. Citation2010).
Sex differences in the effects of prenatal stress were obtained when pregnant rats were restrained only once daily during the last week of gestation in Wistar rats (Zagron and Weinstock Citation2006), or after varied forms of stress of short duration applied once daily (Salomon et al. Citation2011), and rats were given two daily trials in the MWM test. These maternal stressors decreased the rate of spatial learning in males but not in females. Learning decrements were also seen when assessed only in the male offspring after different forms of maternal stress applied once daily (Fujioka et al. Citation2006; Yang et al. Citation2006; Hosseini-Sharifabad and Hadinedoushan Citation2007; Yaka et al. Citation2007). Varied maternal stress started later in gestation (days 17–20) had no effect on the rate of acquisition of spatial learning in juvenile Sprague-Dawley offspring of either gender (Meunier et al. Citation2004). Stress may have been initiated too late to have an impact on the appropriate stage of neural development. The detection of an effect of prenatal stress may also depend on how and when learning was assessed, because male and female rats use different learning strategies during pre-pubertal and adult periods (Kanit et al. Citation2000). This was shown in the following experiments in which we subjected pregnant Wistar rats to daily periods of short varied stress from days 14 to 21 of gestation and examined spatial learning in pre-pubertal (aged 25 days) and adult (aged 65–70 days) male and female offspring. We found that pre-pubertal female rats performed better than males in a spatial learning task in the MWM and that prenatal stress selectively impaired learning in females, while tending to improve it in males (). By contrast, adult males learned to find a hidden escape platform more quickly than females, and prenatal stress slowed the rate of learning only in males (), in agreement with reports of other studies in male rats (Fujioka et al. Citation2006; Yang et al. Citation2006; Hosseini-Sharifabad and Hadinedoushan Citation2007; Yaka et al. Citation2007). These findings show that the same maternal stress paradigm can have a different outcome on spatial learning both according to the sex of the rat and age of testing.
Figure 1. Changes in spatial learning induced by prenatal stress in pre-pubertal and adult male and female rats. Pregnant rats were stressed from days 14–21 by varied forms of stress consisting of restraint for 45 min, elevated platform exposure, crowding, forced swim to which they did not adapt. Offspring were tested either at day 25 or 3 months of age. Latency to find escape platform in MWM is depicted as the average of two daily trials. Values represent mean ± SEM in groups of 10 rats. (a) Pre-pubertal rats. All groups of rats were able to learn the task on consecutive days of testing. There was a significant group × day interaction (F3,36 = 9.7, P < 0.0001). Control females showed significantly shorter latencies over the five days than control males (P < 0.01). Prenatal stress improved learning in males (P < 0.05) and impaired it in females (P < 0.01). (b) Adult rats. All groups of rats were able to learn the task on consecutive days of testing. There was a significant group × day interaction (F3,36 = 10.38, P < 0.0001). Control males showed significantly shorter latencies over the 5 days than control females (P < 0.05). Prenatal stress selectively impaired learning in males (P < 0.05) but had no effect in females.
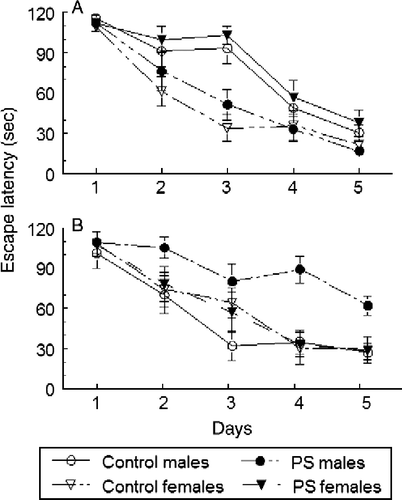
Anxious behaviour
As described above for spatial learning, the majority of studies on the effect of thrice daily maternal restraint stress were performed only in male offspring. Some, but not others, found that prenatal stress heightened their anxiety when tested in the elevated plus maze (EPM) (reviewed in Weinstock Citation2008). When other forms of maternal stress were applied and the offspring of both sexes examined, an increase in anxiety was seen in males and females (Fride and Weinstock Citation1988; Salomon et al. Citation2011) or only in females (Zagron and Weinstock Citation2006; CitationBiala et al. 2010). In recent experiments in which we applied varied stress to rats housed in a reversed light cycle and tested the offspring during the time of peak activity, we were able to detect greater anxious behaviour in the EPM in PS than in control males (Zohar and Weinstock Citation2011). Induction of anxious behaviour selectively in males was seen when pregnant rats were exposed to an aggressive resident lactating rat only from day 16 of gestation (Brunton and Russell Citation2010), but in both sexes if the same stress was applied from day 5 (Bosch et al. Citation2007). The selective effect of late prenatal stress in males may result from slower development of neuronal systems involved in mediation of fear-related behaviour in the cortex of males (Munoz-Cueto et al. Citation1990). These findings indicate that the severity of the stress, the time period during which it occurs and conditions under which behaviour is assessed determine whether anxious behaviour is seen only in female or in male rats or in both sexes.
Morphological changes induced by prenatal stress
Hippocampal and cortical dendritic spines
The establishment and efficient maturation of synaptic circuits is essential for the development of normal brain function. The majority of excitatory synapses are formed at dendritic spines (Bourne and Harris Citation2008), and changes in spine density and morphology account for functional differences at the synaptic level (Segal Citation2005, Citation2010). Together with the prefrontal cortex and amygdala, the hippocampal formation is an essential component of the neural pathways that mediate stress responses and which are essential for learning and memory formation (Madeira and Lieberman Citation1995). Therefore, it is logical to seek a relation between altered dendrite morphology in the prefrontal cortex and hippocampal formation and spatial learning following prenatal stress. In studies conducted by means of Golgi analysis in the hippocampal formation only in adult male rats, a decrease was found in spine length and complexity in the CA1 (Martinez-Tellez et al. Citation2009), CA1 and CA3 (Jia et al. Citation2010) and in CA3 pyramidal neurones (Hosseini-Sharifabad and Hadinedoushan Citation2007). The link between the reduction in spine complexity and length in the CA1 region to learning impairment was seen in the reduction in hippocampal LTP and that of the NMDA receptor subunits (Son et al. Citation2006; Yaka et al. Citation2007). Others found a reduction in the length of neuronal processes in the DG and CA1 region, together with a decrease in dendritic complexity in the DG, CA1 and CA3 regions of neonatal PS males. Learning deficits were only assessed and found in adult PS males (Fujioka et al. Citation2006). However, maternal stress of shorter duration improved learning in their adult male offspring and caused an increase in the length of neuronal processes in the DG and CA1 region and an increase in dendritic complexity in the CA3 region of neonates (Fujioka et al. Citation2006). The findings clearly show that changes in dendritic morphology are established prenatally and contribute to learning deficits induced by prenatal stress. The differential effects of severe and milder stress on spatial learning in rats support those of deficits or improvement in measures of cognition in children of women who experienced severe stress or milder stress during the ice storm (DiPietro et al. Citation2006).
Since the majority of reports of learning deficits in humans have been confined to young children or adolescents, we examined the effect in rats of varied prenatal stress that impaired learning in females and slightly improved that in males, based on dendritic morphology in pre-pubertal male and female offspring. We found that prenatal stress caused a reduction in spine densities and dendritic length in the DG, CA1 and CA3 regions of females but increased spine densities and dendrite length in males (). These changes paralleled their respective learning performance in the water maze (). As the same prenatal stress paradigm impaired learning in adult males, but not in females, it is likely that the reduction in hippocampal LTP shown in PS males in a previous study (Yaka et al. Citation2007) resulted from a decrease in dendritic morphology in the hippocampal sub-regions previously described (Hosseini-Sharifabad and Hadinedoushan Citation2007; Martinez-Tellez et al. Citation2009; Jia et al. Citation2010).
Figure 2. Sex-specific alterations in pre-pubertal rats induced by varied forms of prenatal stress in the morphology of DG granular neurons. Values represent mean ± SEM from 4 to 6 rats/group. ‡ Significantly different from males, P < 0.05; significantly different from controls, P < 0.05. Spine density, length and the number of intersections were significantly higher in females than in males in this hippocampal region. Prenatal stress abolished the sex difference by increasing these measures of dendritic morphology in males and decreasing them in females.
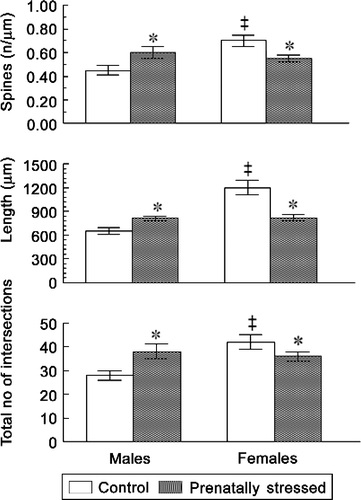
There appear to be only two studies on the effect of prenatal stress on dendritic spine morphology in the prefrontal cortex. In pre-pubertal rats, prenatal stress induced heightened anxiety in both sexes, reduced spine densities on the apical and basal dendrites in layers II and III (orbitofrontal cortex and anterior cingulate cortex of their naïve male and female litter mates (Murmu et al. Citation2006). In a later study performed in adult PS males which had been subjected to a variety of behavioural tasks (not specified) prior to the histological examination, no significant changes in spine length or dendritic complexity were seen in the same cortical areas (Michelsen et al. Citation2007).
Hippocampal neurogenesis
The subgranular zone (SG) of the DG of the hippocampal formation is an important site for neurogenesis during adulthood (Abrous et al. Citation2005). Three major steps govern the production of new neurons: the rate of cell production, their survival and differentiation to a neuronal phenotype. Hippocampal neurogenesis differs in males and females (Perfilieva et al. Citation2001), with dioestrous females showing lower levels than males (Mandyam et al. Citation2008). There is also a differential sex sensitivity to stress in adulthood (Westenbroek et al. Citation2004) and to early post-natal manipulations (Oomen et al. Citation2009). Maternal thrice daily restraint stress that heightened anxiety selectively in females (Richardson et al. Citation2006), reduced proliferation of precursor cells in the SG layer of the DG in both sexes and increased cell death in males, thereby upsetting the balance between neurogenesis and cell death in a sex-specific manner (Mandyam et al. Citation2008). In rats in which maternal stress induced anxiety selectively in males and improved spatial learning in females, a reduction in newly formed cells was seen in the ventral hippocampus of males with a trend towards an increase in the dorsal hippocampus of females (Zuena et al. Citation2008). It was also found that neonatal handling during the period when the DG develops prevents the development of heightened anxiety (Wakshlak and Weinstock Citation1990; Bogoch et al. Citation2007) and the deficits in hippocampal neurogenesis (Lemaire et al. Citation2006). A decrease in neurogenesis was found in the dorsal hippocampus of 30-day-old male and female offspring of mothers who had received “psychological stress” of proximity to other pregnant rats while they were exposed to electric foot shocks. No difference was detected in the open-field exploratory behaviour of the offspring, but these were not focused on measures of anxiety (Odagiri et al. Citation2008).
Although we have no information about the effect of prenatal stress on neurogenesis in humans, acute disturbance 5 days a week either during days 50–92 or during days 105–147 of gestation in monkeys resulted in a decrease of some 32% in newly formed cells in the SG zone of the DG in the offspring before puberty (Coe et al. Citation2003). The data were not analysed separately in males and females. The reduction in neurogenesis was associated with a decrease in exploratory activity and elevated basal levels of cortisol which were not suppressed by dexamethasone. This indicated that maternal stress in non-human primates altered the feedback regulation of the HPA axis in the offspring as had been reported in PS rats (Weinstock et al. Citation1992; McCormick et al. Citation1995; Maccari et al. Citation2003; Poltyrev et al. Citation2005; Bosch et al. Citation2007). The consistent finding of a reduction in neurogenesis indicates that gestational stress has a long-lasting influence of the formation of new neurones in the hippocampal formation. This effect, coupled with the changes in dendritic morphology in the hippocampus and prefrontal cortex, can form the basis of the anxious behaviour and learning deficits induced by prenatal stress.
Hormonal mediation of the morphological and behavioural effects of prenatal stress
Considerations of the possible aetiology of the morphological and behavioural alterations induced by gestational stress have led to the hypothesis of altered brain programming with a major role played by maternal glucocorticoids (Seckl Citation2008). In normal pregnancies, the foetus is protected from excess levels of cortisol or corticosterone (COR) by an enzyme 11β-dehydrogenase (11β-HSD-2) that converts them to relatively inactive products. The enzyme is found in the placenta and CNS, but its expression is greatly reduced in mid-gestation in the rat (Diaz et al. Citation1998) and between gestational weeks 19 and 26 in humans (Stewart et al. Citation1994). This increases the vulnerability of the brain to glucocorticoids that could reach it in excess amounts when rats or humans are stressed during this time. In the rat, glucocorticoid receptors (GR) appear in the hippocampus on day 13 and mineralocorticoid receptors (MR) on day 16 of gestation. The observation that the density of GR and MR was reduced in the hippocampus of rats whose mothers were stressed from day 11–20 in gestation (Maccari et al. Citation2003) and which also show impaired regulation of the HPA axis response to stress (Poltyrev et al. Citation2005) supports the suggestion of an effect of excess COR on the developing foetal brain. This suggestion was further strengthened by the finding that maternal adrenalectomy prior to the initiation of stress prevented the reduction in hippocampal MR and the increased response to stress in their male offspring, and both were reinstated by daily administration of COR together with the stress (Barbazanges et al. Citation1996).
We conducted experiments to determine whether altered brain programming by COR was also responsible for the heightened anxiety seen in female rats and learning deficits in males. Maternal adrenalectomy with the administration of the steroid to maintain basal circulating levels completely prevented the rise in plasma COR in response to stress. The offspring of adrenalectomised dams no longer showed anxiety or learning deficits in response to restraint stress, indicating that adrenal hormones were responsible for their aetiology (Zagron and Weinstock Citation2006). In another study, we determined the extent and duration of the elevation of circulating COR by each of the varied stressors used on different days of gestation to induce anxiety and learning deficits in male and female offspring. We then injected COR to replicate the pattern of its elevation in adrenalectomised rat dams. Anxious behaviour, but not the learning deficits, was reinstated by COR injections (Salomon et al. Citation2011). The finding supports that of Nagano et al. (Citation2008) who induced anxiety in the male offspring (females were not tested) by injecting on days 16–21 of pregnancy dexamethasone, a steroid that is not metabolised by 11β-HSD-2.
The varied maternal stress used in our experiments reduced the anogenital distance in neonatal males but did not affect their birth weight. Anogenital distance was still smaller than in controls on days 7 and 14 but not on day 21. This suggests that neonatal and probably foetal (Ward and Weisz Citation1984) testosterone levels were decreased compared to that in control pups, as shown in studies using different prenatal stressors (Gerardin et al. Citation2005; Richardson et al. Citation2006; Rodriguez et al. Citation2007). The decrease in testosterone or exposure to excess maternal COR (Takahashi et al. Citation1998) occurring at a critical time in the development of the foetal hippocampal formation could have caused the reductions in dendritic morphology and neurogenesis in the DG. Although maternal adrenalectomy prevented the reduction in anogenital distance, this was not reinstated by maternal COR injections, making it unlikely that excess maternal COR was the primary cause of diminished testosterone levels. It is probable that some effects of prenatal stress are mediated by other adrenal hormones such as adrenaline, which can reach the foetus from the placental circulation and activate β-receptors in the brain. Such activation that could contribute to the learning impairment in PS males was suggested by McGivern et al. (Citation1986) since it was prevented by administration of propranolol to the stressed mothers. Further studies are in progress to elucidate these and other possibilities.
The possible role played by a reduction in maternal attention to the pups given by a stressed mother has been discussed in previous articles (Weinstock Citation2008; Salomon et al. Citation2011). The deficit in such maternal behaviour reported in some, but not other studies, may be responsible for the altered regulation of the HPA axis and heightened anxiety because these can be significantly reduced in PS pups reared by foster mothers (Maccari et al. Citation1995; Barros et al. Citation2006). Since administration of COR to stressed, adrenalectomised mothers reinstated in the offspring both the alteration in the HPA axis and the anxiety, these may be mediated by raised levels of COR present in their milk (Pfister and Muir Citation1989) as PS pups fostered onto control mothers would not be exposed to higher levels of COR through this vehicle. This suggestion is also compatible with our finding that the learning impairment in PS males was not reinstated in offspring of adrenalectomised mothers injected with COR, neither was it affected by a fostering procedure (Yang et al. Citation2006). It is more likely that the effect on learning results from processes occurring prenatally, because significant reductions in dendritic morphology were seen in the hippocampus of 1-day-old rats (Fujioka et al. Citation2006) which presaged the appearance of learning deficits in adulthood.
Conclusions
Recent prospective studies indicate that stress occurring during weeks 12–20 of pregnancy increases the likelihood of cognitive and emotional problems in the offspring. There is also a suggestion that cognitive deficits may be more prevalent in males, and anxiety in females. Experiments in rats that were stressed, during the equivalent period of neuronal development in the foetus indicated from human studies, found that heightened anxiety can appear in both sexes or selectively in either sex depending on the severity of stress and its timing. They also found that spatial learning deficits occur in pre-pubertal females but are more prevalent in the male offspring in adulthood. Like in humans, the outcome on cognition depends on the severity of the stress and the age at which the offspring are examined. The behavioural changes in rats are preceded by a sex-dependent reduction in neurogenesis and in measures of dendritic morphology in the hippocampal formation and in the prefrontal cortex. The morphological changes in males may result from a reduction in foetal levels of testosterone and brain aromatase activity at a critical time during development. Anxious behaviour, but not learning deficits, probably results from altered brain programming by COR as it can be prevented by maternal adrenalectomy prior to the stress and reinstated by COR administration. Further studies are needed in humans to determine whether there are also sex-dependent structural changes due to prenatal stress and which hormones are responsible for them.
Declaration of interest: The authors report no conflicts of interest. The authors alone are responsible for the content and writing of the paper.
References
- Abrous DN, Koehl M, Le Moal M. 2005. Adult neurogenesis: From precursors to network and physiology. Physiol Rev. 85:523–569.
- Andrade JP, Madeira MD, Paula-Barbosa MM. 2000. Sexual dimorphism in the subiculum of the rat hippocampal formation. Brain Res. 875:125–137.
- Archer JE, Blackman DE. 1971. Prenatal psychological stress and offspring behavior in rats and mice. Dev Psychobiol. 4:193–248.
- Barbazanges A, Piazza PV, Le Moal M, Maccari S. 1996. Maternal glucocorticoid secretion mediates long-term effects of prenatal stress. J Neurosci. 16:3943–3949.
- Barros VG, Rodriguez P, Martijena ID, Perez A, Molina VA, Antonelli MC. 2006. Prenatal stress and early adoption effects on benzodiazepine receptors and anxiogenic behavior in the adult rat brain. Synapse. 60:609–618.
- Bayer SA, Altman J, Russo RJ, Zhang X. 1993. Timetables of neurogenesis in the human brain based on experimentally determined patterns in the rat. Neurotoxicology. 14:83–144.
- Beijers R, Jansen J, Riksen-Walraven M, de Weerth C. 2010. Maternal prenatal anxiety and stress predict infant illnesses and health complaints. Pediatrics. 126:e401–e409.
- Beversdorf DQ, Manning SE, Hillier A, Anderson SL, Nordgren RE, Walters SE, Nagaraja HN, Cooley WC, Gaelic SE, Bauman ML. 2005. Timing of prenatal stressors and autism. J Autism Dev Disord. 35:471–478.
- Biala YN, Bogoch Y, Bejar C, Linial M, Weinstock M. 2010. Prenatal stress diminishes gender differences in behavior and in expression of hippocampal synaptic genes and proteins in rats Hippocampus (available online).
- Bliss TV, Collingridge GL. 1993. A synaptic model of memory: Long-term potentiation in the hippocampus. Nature. 361:31–39.
- Blokland A, Rutten K, Prickaerts J. 2006. Analysis of spatial orientation strategies of male and female Wistar rats in a Morris water escape task. Behav Brain Res. 171:216–224.
- Bogoch Y, Biala YN, Linial M, Weinstock M. 2007. Anxiety induced by prenatal stress is associated with suppression of hippocampal genes involved in synaptic function. J Neurochem. 101:1018–1030.
- Bosch OJ, Musch W, Bredewold R, Slattery DA, Neumann ID. 2007. Prenatal stress increases HPA axis activity and impairs maternal care in lactating female offspring: Implications for postpartum mood disorder. Psychoneuroendocrinology. 32:267–278.
- Bourne JN, Harris KM. 2008. Balancing structure and function at hippocampal dendritic spines. Annu Rev Neurosci. 31:47–67.
- Bowman RE, MacLusky NJ, Sarmiento Y, Frankfurt M, Gordon M, Luine VN. 2004. Sexually dimorphic effects of prenatal stress on cognition, hormonal responses, and central neurotransmitters. Endocrinology. 145:3778–3787.
- Brunton PJ, Russell JA. 2010. Prenatal social stress in the rat programmes neuroendocrine and behavioural responses to stress in the adult offspring: Sex-specific effects. J Neuroendocrinol. 22:258–271.
- Buss C, Davis EP, Muftuler LT, Head K, Sandman CA. 2010. High pregnancy anxiety during mid-gestation is associated with decreased gray matter density in 6–9-year-old children. Psychoneuroendocrinology. 35:141–153.
- Charil A, Laplante DP, Vaillancourt C, King S. 2010. Prenatal stress and brain development. Brain Res Rev. 65:56–79.
- Coe CL, Kramer M, Czeh B, Gould E, Reeves AJ, Kirschbaum C, Fuchs E. 2003. Prenatal stress diminishes neurogenesis in the dentate gyrus of juvenile rhesus monkeys. Biol Psychiatry. 54:1025–1034.
- Davis EP, Sandman CA. 2010. The timing of prenatal exposure to maternal cortisol and psychosocial stress is associated with human infant cognitive development. Child Dev. 81:131–148.
- de Bruijn AT, van Bakel HJ, van Baar AL. 2009. Sex differences in the relation between prenatal maternal emotional complaints and child outcome. Early Hum Dev. 85:319–324.
- de Bruin JP, Moita MP, de Brabander HM, Joosten RN. 2001. Place and response learning of rats in a Morris water maze: Differential effects of fimbria fornix and medial prefrontal cortex lesions. Neurobiol Learn Mem. 75:164–178.
- Diaz R, Brown RW, Seckl JR. 1998. Distinct ontogeny of glucocorticoid and mineralocorticoid receptor and 11beta-hydroxysteroid dehydrogenase types I and II mRNAs in the fetal rat brain suggest a complex control of glucocorticoid actions. J Neurosci. 18:2570–2580.
- DiPietro JA, Novak MF, Costigan KA, Atella LD, Reusing SP. 2006. Maternal psychological distress during pregnancy in relation to child development at age two. Child Dev. 77:573–587.
- Fride E, Weinstock M. 1988. Prenatal stress increases anxiety related behavior and alters cerebral lateralization of dopamine activity. Life Sci. 42:1059–1065.
- Fujioka A, Fujioka T, Ishida Y, Maekawa T, Nakamura S. 2006. Differential effects of prenatal stress on the morphological maturation of hippocampal neurons. Neuroscience. 141:907–915.
- Garcia-Falgueras A, Pinos H, Collado P, Pasaro E, Fernandez R, Segovia S, Guillamon A. 2005. The expression of brain sexual dimorphism in artificial selection of rat strains. Brain Res. 1052:130–138.
- Gerardin DC, Pereira OC, Kempinas WG, Florio JC, Moreira EG, Bernardi MM. 2005. Sexual behavior, neuroendocrine, and neurochemical aspects in male rats exposed prenatally to stress. Physiol Behav. 84:97–104.
- Gould E, Westlind-Danielsson A, Frankfurt M, McEwen BS. 1990. Sex differences and thyroid hormone sensitivity of hippocampal pyramidal cells. J Neurosci. 10:996–1003.
- Gregory E. 1975. Comparison of postnatal CNS development between male and female rats. Brain Res. 99:152–156.
- Grizenko N, Shayan YR, Polotskaia A, Ter-Stepanian M, Joober R. 2008. Relation of maternal stress during pregnancy to symptom severity and response to treatment in children with ADHD. J Psychiatry Neurosci. 33:10–16.
- Hosseini-Sharifabad M, Hadinedoushan H. 2007. Prenatal stress induces learning deficits and is associated with a decrease in granules and CA3 cell dendritic tree size in rat hippocampus. Anat Sci Int. 82:211–217.
- Jia N, Yang K, Sun Q, Cai Q, Li H, Cheng D, Fan X, Zhu Z. 2010. Prenatal stress causes dendritic atrophy of pyramidal neurons in hippocampal CA3 region by glutamate in offspring rats. Dev Neurobiol. 70:114–125.
- Johnston AL, File SE. 1991. Sex differences in animal tests of anxiety. Physiol Behav. 49:245–250.
- Juraska JM, Fitch JM, Henderson C, Rivers N. 1985. Sex differences in the dendritic branching of dentate granule cells following differential experience. Brain Res. 333:73–80.
- Kanit L, Taskiran D, Yilmaz OA, Balkan B, Demirgoren S, Furedy JJ, Pogun S. 2000. Sexually dimorphic cognitive style in rats emerges after puberty. Brain Res Bull. 52:243–248.
- Khashan AS, Abel KM, McNamee R, Pedersen MG, Webb RT, Baker PN, Kenny LC, Mortensen PB. 2008. Higher risk of offspring schizophrenia following antenatal maternal exposure to severe adverse life events. Arch Gen Psychiatry. 65:146–152.
- Kim HW, Cho SC, Kim BN, Kim JW, Shin MS, Kim Y. 2009. Perinatal and familial risk factors are associated with full syndrome and subthreshold attention-deficit hyperactivity disorder in a korean community sample. Psychiatry Investig. 6:278–285.
- King S, Laplante DP. 2005. The effects of prenatal maternal stress on children's cognitive development: Project Ice Storm. Stress. 8:35–45.
- Kinney DK, Miller AM, Crowley DJ, Huang E, Gerber E. 2008. Autism prevalence following prenatal exposure to hurricanes and tropical storms in Louisiana. J Autism Dev Disord. 38:481–488.
- Kofman O. 2002. The role of prenatal stress in the etiology of developmental behavioural disorders. Neurosci Biobehav Rev. 26:457–470.
- Konkle AT, McCarthy MM. 2010. Developmental time course of estradiol, testosterone, and dihydrotestosterone levels in discrete regions of male and female rat brain. Endocrinology. 152 1: 223–235.
- Laplante DP, Brunet A, Schmitz N, Ciampi A, King S. 2008. Project Ice Storm: Prenatal maternal stress affects cognitive and linguistic functioning in 5 1/2-year-old children. J Am Acad Child Adolesc Psychiatry. 47:1063–1072.
- Lazinski MJ, Shea AK, Steiner M. 2008. Effects of maternal prenatal stress on offspring development: A commentary. Arch Womens Ment Health. 11:363–375.
- Lemaire V, Lamarque S, Le Moal M, Piazza PV, Abrous DN. 2006. Postnatal stimulation of the pups counteracts prenatal stress-induced deficits in hippocampal neurogenesis. Biol Psychiatry. 59:786–792.
- Li J, Olsen J, Vestergaard M, Obel C. 2010. Attention-deficit/hyperactivity disorder in the offspring following prenatal maternal bereavement: A nationwide follow-up study in Denmark. Eur Child Adolesc Psychiatry. 19:747–753.
- Maccari S, Piazza PV, Kabbaj M, Barbazanges A, Simon H, Le Moal M. 1995. Adoption reverses the long-term impairment in glucocorticoid feedback induced by prenatal stress. J Neurosci. 15:110–116.
- Maccari S, Darnaudery M, Morley-Fletcher S, Zuena AR, Cinque C, Van Reeth O. 2003. Prenatal stress and long-term consequences: Implications of glucocorticoid hormones. Neurosci Biobehav Rev. 27:119–127.
- Madeira MD, Lieberman AR. 1995. Sexual dimorphism in the mammalian limbic system. Prog Neurobiol. 45:275–333.
- Mandyam CD, Crawford EF, Eisch AJ, Rivier CL, Richardson HN. 2008. Stress experienced in utero reduces sexual dichotomies in neurogenesis, microenvironment, and cell death in the adult rat hippocampus. Dev Neurobiol. 68:575–589.
- Markham JA, Koenig JI. 2011. Prenatal stress: Role in psychotic and depressive diseases. Psychopharmacology (Berl). 214 1: 89–106.
- Martinez-Tellez RI, Hernandez-Torres E, Gamboa C, Flores G. 2009. Prenatal stress alters spine density and dendritic length of nucleus accumbens and hippocampus neurons in rat offspring. Synapse. 63:794–804.
- Martini J, Knappe S, Beesdo-Baum K, Lieb R, Wittchen HU. 2010. Anxiety disorders before birth and self-perceived distress during pregnancy: Associations with maternal depression and obstetric, neonatal and early childhood outcomes. Early Hum Dev. 86:305–310.
- McCormick CM, Smythe JW, Sharma S, Meaney MJ. 1995. Sex-specific effects of prenatal stress on hypothalamic–pituitary–adrenal responses to stress and brain glucocorticoid receptor density in adult rats. Brain Res Dev Brain Res. 84:55–61.
- McGivern RF, Poland RE, Taylor AN, Branch BJ, Raum WJ. 1986. Prenatal stress feminizes adult male saccharin preference and maze learning: Antagonism by propranolol. Monogr Neural Sci. 12:172–178.
- Mennes M, Stiers P, Lagae L, Van den Bergh B. 2006. Long-term cognitive sequelae of antenatal maternal anxiety: Involvement of the orbitofrontal cortex. Neurosci Biobehav Rev. 30:1078–1086.
- Meunier J, Gue M, Recasens M, Maurice T. 2004. Attenuation by a sigma1 (sigma1) receptor agonist of the learning and memory deficits induced by a prenatal restraint stress in juvenile rats. Br J Pharmacol. 142:689–700.
- Meyer G, Ferres-Torres R, Mas M. 1978. The effects of puberty and castration on hippocampal dendritic spines of mice. A Golgi study. Brain Res. 155:108–112.
- Michelsen KA, van den Hove DL, Schmitz C, Segers O, Prickaerts J, Steinbusch HW. 2007. Prenatal stress and subsequent exposure to chronic mild stress influence dendritic spine density and morphology in the rat medial prefrontal cortex. BMC Neurosci. 8:107.
- Munoz-Cueto JA, Garcia-Segura LM, Ruiz-Marcos A. 1990. Developmental sex differences and effect of ovariectomy on the number of cortical pyramidal cell dendritic spines. Brain Res. 515:64–68.
- Murmu MS, Salomon S, Biala Y, Weinstock M, Braun K, Bock J. 2006. Changes of spine density and dendritic complexity in the prefrontal cortex in offspring of mothers exposed to stress during pregnancy. Eur J Neurosci. 24:1477–1487.
- Nagano M, Ozawa H, Suzuki H. 2008. Prenatal dexamethasone exposure affects anxiety-like behaviour and neuroendocrine systems in an age-dependent manner. Neurosci Res. 60:364–371.
- Newhouse P, Newhouse C, Astur RS. 2007. Sex differences in visual-spatial learning using a virtual water maze in pre-pubertal children. Behav Brain Res. 183:1–7.
- Odagiri K, Abe H, Kawagoe C, Takeda R, Ikeda T, Matsuo H, Nonaka H, Ebihara K, Nishimori T, Ishizuka Y, Hashiguchi H, Ishida Y. 2008. Psychological prenatal stress reduced the number of BrdU immunopositive cells in the dorsal hippocampus without affecting the open field behavior of male and female rats at one month of age. Neurosci Lett. 446:25–29.
- Oomen CA, Girardi CE, Cahyadi R, Verbeek EC, Krugers H, Joels M, Lucassen PJ. 2009. Opposite effects of early maternal deprivation on neurogenesis in male versus female rats. PLoS One. 4:e3675.
- Perfilieva E, Risedal A, Nyberg J, Johansson BB, Eriksson PS. 2001. Gender and strain influence on neurogenesis in dentate gyrus of young rats. J Cereb Blood Flow Metab. 21:211–217.
- Pfister HP, Muir JL. 1989. Psychological stress and administered oxytocin during pregnancy: Effect corticosterone and prolactin response in lactating rats. Int J Neurosci. 45:91–99.
- Poltyrev T, Gorodetsky E, Bejar C, Schorer-Apelbaum D, Weinstock M. 2005. Effect of chronic treatment with ladostigil (TV-3326) on anxiogenic and depressive-like behaviour and on activity of the hypothalamic–pituitary–adrenal axis in male and female prenatally stressed rats. Psychopharmacology (Berl). 181:118–125.
- Rice D, Barone SJr. 2000. Critical periods of vulnerability for the developing nervous system: Evidence from humans and animal models. Environ Health Perspect. 108 Suppl 3: 511–533.
- Richardson HN, Zorrilla EP, Mandyam CD, Rivier CL. 2006. Exposure to repetitive versus varied stress during prenatal development generates two distinct anxiogenic and neuroendocrine profiles in adulthood. Endocrinology. 147:2506–2517.
- Rodriguez A, Bohlin G. 2005. Are maternal smoking and stress during pregnancy related to ADHD symptoms in children?. J Child Psychol Psychiatry. 46:246–254.
- Rodriguez N, Mayer N, Gauna HF. 2007. Effects of prenatal stress on male offspring sexual maturity. Biocell. 31:67–74.
- Sakuma Y. 2009. Gonadal steroid action and brain sex differentiation in the rat. J Neuroendocrinol. 21:410–414.
- Salehi B, Cordero MI, Sandi C. 2010. Learning under stress: The inverted-U-shape function revisited. Learn Mem. 17:522–530.
- Salomon S, Bejar C, Schorer-Apelbaum D, Weinstock M. 2011. Corticosterone mediates some but not other behavioural changes induced by prenatal stress in rats. J Neuroendocrinol. 23:118–128.
- Sandstrom NJ, Kaufman J, Huettel SA. 1998. Males and females use different distal cues in a virtual environment navigation task. Brain Res Cogn Brain Res. 6:351–360.
- Seckl JR. 2008. Glucocorticoids, developmental “programming” and the risk of affective dysfunction. Prog Brain Res. 167:17–34.
- Segal M. 2005. Dendritic spines and long-term plasticity. Nat Rev Neurosci. 6:277–284.
- Segal M. 2010. Dendritic spines, synaptic plasticity and neuronal survival: Activity shapes dendritic spines to enhance neuronal viability. Eur J Neurosci. 31:2178–2184.
- Son GH, Geum D, Chung S, Kim EJ, Jo JH, Kim CM, Lee KH, Kim H, Choi S, Kim HT, Lee CJ, Kim K. 2006. Maternal stress produces learning deficits associated with impairment of NMDA receptor-mediated synaptic plasticity. J Neurosci. 26:3309–3318.
- Stewart PM, Murry BA, Mason JI. 1994. Type 2 11 beta-hydroxysteroid dehydrogenase in human fetal tissues. J Clin Endocrinol Metab. 78:1529–1532.
- Szuran TF, Pliska V, Pokorny J, Welzl H. 2000. Prenatal stress in rats: Effects on plasma corticosterone, hippocampal glucocorticoid receptors, and maze performance. Physiol Behav. 71:353–362.
- Takahashi LK, Turner JG, Kalin NH. 1998. Prolonged stress-induced elevation in plasma corticosterone during pregnancy in the rat: Implications for prenatal stress studies. Psychoneuroendocrinology. 23:571–581.
- Talge NM, Neal C, Glover V. 2007. Antenatal maternal stress and long-term effects on child neurodevelopment: How and why?. J Child Psychol Psychiatry. 48:245–261.
- Thorup A, Petersen L, Jeppesen P, Ohlenschlaeger J, Christensen T, Krarup G, Jorgensen P, Nordentoft M. 2007. Gender differences in young adults with first-episode schizophrenia spectrum disorders at baseline in the Danish OPUS study. J Nerv Ment Dis. 195:396–405.
- Van den Bergh BR, Marcoen A. 2004. High antenatal maternal anxiety is related to ADHD symptoms, externalizing problems, and anxiety in 8- and 9-year-olds. Child Dev. 75:1085–1097.
- Van den Bergh BR, Mennes M, Oosterlaan J, Stevens V, Stiers P, Lagae MA. 2005. High antenatal maternal anxiety is related to impulsivity during performance on cognitive tasks in 14- and 15-year-olds. Neurosci Biobehav Revs. 29:259–269.
- Van den Bergh BR, Van Calster B, Smits T, Van Huffel S, Lagae L. 2008. Antenatal maternal anxiety is related to HPA-axis dysregulation and self-reported depressive symptoms in adolescence: A prospective study on the fetal origins of depressed mood. Neuropsychopharmacology. 33:536–545.
- Van Lieshout RJ, Boylan K. 2010. Increased depressive symptoms in female but not male adolescents born at low birth weight in the offspring of a national cohort. Can J Psychiatry. 55:422–430.
- Wakshlak A, Weinstock M. 1990. Neonatal handling reverses behavioral abnormalities induced in rats by prenatal stress. Physiol Behav. 48:289–292.
- Ward IL. 1972. Prenatal stress feminizes and demasculinizes the behavior of males. Science. 175:82–84.
- Ward IL, Weisz J. 1984. Differential effects of maternal stress on circulating levels of corticosterone, progesterone, and testosterone in male and female rat fetuses and their mothers. Endocrinology. 114:1635–1644.
- Warren SG, Humphreys AG, Juraska JM, Greenough WT. 1995. LTP varies across the estrous cycle: Enhanced synaptic plasticity in proestrus rats. Brain Res. 703:26–30.
- Weinstock M. 1997. Does prenatal stress impair coping and regulation of hypothalamic–pituitary–adrenal axis?. Neurosci Biobehav Rev. 21:1–10.
- Weinstock M. 2001. Alterations induced by gestational stress in brain morphology and behaviour of the offspring. Prog Neurobiol. 65:427–451.
- Weinstock M. 2007. Gender differences in the effects of prenatal stress on brain development and behaviour. Neurochem Res. 32:1730–1740.
- Weinstock M. 2008. The long-term behavioural consequences of prenatal stress. Neurosci Biobehav Rev. 32:1073–1086.
- Weinstock M, Matlina E, Maor GI, Rosen H, McEwen BS. 1992. Prenatal stress selectively alters the reactivity of the hypothalamic–pituitary adrenal system in the female rat. Brain Res. 595:195–200.
- Weisz J, Brown BL, Ward IL. 1982. Maternal stress decreases steroid aromatase activity in brains of male and female rat fetuses. Neuroendocrinology. 35:374–379.
- Westenbroek C, Den Boer JA, Veenhuis M, Ter Horst GJ. 2004. Chronic stress and social housing differentially affect neurogenesis in male and female rats. Brain Res Bull. 64:303–308.
- Wimer CC, Wimer RE. 1989. On the sources of strain and sex differences in granule cell number in the dentate area of house mice. Brain Res Dev Brain Res. 48:167–176.
- Woolley CS, McEwen BS. 1992. Estradiol mediates fluctuation in hippocampal synapse density during the estrous cycle in the adult rat. J Neurosci. 12:2549–2554.
- Woolley CS, Weiland NG, McEwen BS, Schwartzkroin PA. 1997. Estradiol increases the sensitivity of hippocampal CA1 pyramidal cells to NMDA receptor-mediated synaptic input: Correlation with dendritic spine density. J Neurosci. 17:1848–1859.
- Wu J, Song TB, Li YJ, He KS, Ge L, Wang LR. 2007. Prenatal restraint stress impairs learning and memory and hippocampal PKCbeta1 expression and translocation in offspring rats. Brain Res. 1141:205–213.
- Yaka R, Salomon S, Matzner H, Weinstock M. 2007. Effect of varied gestational stress on acquisition of spatial memory, hippocampal LTP and synaptic proteins in juvenile male rats. Behav Brain Res. 179:126–132.
- Yang J, Han H, Cao J, Li L, Xu L. 2006. Prenatal stress modifies hippocampal synaptic plasticity and spatial learning in young rat offspring. Hippocampus. 16:431–436.
- Zagron G, Weinstock M. 2006. Maternal adrenal hormone secretion mediates behavioural alterations induced by prenatal stress in male and female rats. Behav Brain Res. 175:323–328.
- Zohar I, Weinstock M. 2011. Differential effect of prenatal stress on the expression of cortiocotropin releasing hormone and its receptors in the hypothalamus and amygdala in male and female rats. J Neuroendocrinol. 23:320–328.
- Zuena AR, Mairesse J, Casolini P, Cinque C, Alema GS, Morley-Fletcher S, Chiodi V, Spagnoli LG, Gradini R, Catalani A, Nicoletti F, Maccari S. 2008. Prenatal restraint stress generates two distinct behavioral and neurochemical profiles in male and female rats. PLoS One. 3:e2170.