Abstract
The aim of this study was to evaluate vascular and metabolic effects of chronic mild unpredictable stress (CMS) and hypercaloric diet (HD) without carbohydrate supplementation in rats. Male Sprague-Dawley rats were randomly assigned to four groups: Control, HD, CMS, and HD plus CMS. CMS consisted of the application of different stressors for 3 weeks. The rats were killed 15 days after CMS exposure. The HD group presented higher plasma lipid concentrations, without changes in fasting glucose concentration, glucose tolerance test, and vascular function and morphology, in comparison with the control group. Stressed rats presented higher fasting blood concentration of insulin, higher homeostasis model assessment index values and area under the curve in an oral glucose tolerance test, in comparison with non-stressed rats. CMS increased the plasma concentrations of corticosterone and lipids, and the atherogenic index values, without change in high-density lipoprotein level. CMS increased intima-media thickness and induced endothelium-dependent supersensitivity to phenylephrine, and lowered the relaxation response to acetylcholine in the thoracic aorta isolated from rats fed with control or HD, in comparison with non-stressed groups. CMS effects were independent of diet. In non-stressed rats, the HD induced dyslipidemia, but did not change glucose metabolism, vascular function, or morphology. The data from this study indicate that CMS promotes a set of events which together can contribute to impair function of the thoracic aorta.
Introduction
In Western societies, regular consumption of hypercaloric diets (HDs) and obesity are often associated with the occurrence of metabolic and cardiovascular diseases. Atherogenic dyslipidemia is a major underlying cause for the development of atherosclerosis (Sheril et al. Citation2009). However, a high-fat, high-sugar diet alone cannot account for the current epidemic of obesity (Ludwigh Citation2003) and coronary heart disease (Gu et al. Citation2009). Moreover, despite the association between atherosclerosis and increase in serum lipid concentration, many individuals develop severe atherosclerotic lesions while they have low serum lipid concentration, and others develop far more severe atherosclerosis than would be expected on the basis of a modest elevation of serum lipids (Kaplan et al. Citation1983).
As exposure to chronic stress also correlates with an increasing incidence of visceral obesity, insulin resistance, hypertension, and atherosclerosis, stress has been recognized as a risk factor for cardiovascular and metabolic diseases (Kyrou and Tsigos Citation2007; Shively et al. Citation2009). However, in response to stress, some people lose weight while others gain weight and the mechanisms involved in the relationships among chronic stress, visceral obesity, dyslipidemia, and cardiovascular diseases have not yet been completely clarified.
Animal experimentation is a useful tool to complement clinical research and epidemiological data. Rodents generally adapt to repeated application of stressors; however, this does not occur if the presentation of stimuli is unpredictable, as it is in chronic mild unpredictable stress (CMS). (Moreau et al. Citation1994; Moreau Citation1997). In a previous study (Neves et al. Citation2009), it was observed that CMS induced proatherogenic changes in rats and that this effect seems to be related to a decrease in the bioavailability of endothelial nitric oxide (NO) induced by stress. Here, we tested the hypothesis that CMS would adversely alter vascular function independently of high-fat diet.
Since insulin resistance and dyslipidemia are involved in atherogenesis and endothelium dysfunction, the aim of the present study was to evaluate the effects of CMS and high-fat HD on the reactivity of thoracic aorta to phenylephrine and acetylcholine, morphology of the thoracic aorta, blood lipid concentrations, and glucose metabolism.
Materials and methods
Animals and experimental design
Seventy-two male Sprague-Dawley rats (60 days old; 290–340 g) were randomly assigned to four groups: Control, HD, CMS, and HD plus CMS (HD+CMS). Rats were housed one per cage at 22 ± 2°C with lights on from 06:00 to 18:00 h, and received filtered water ad libitum. All procedures were approved by the UNICAMP Committee on Animal Research Ethics (Protocol number 900-1) and were in accordance with the guidelines of the National Council of Control of Animal Experimentation (CONCEA).
For 7 weeks, the control and CMS groups were fed a control diet based on AIN-93M, formulated for the maintenance of adult rodents (Reeves et al. Citation1993), containing 3.6 kcal/g (14.1% kcal protein, 75.9% kcal carbohydrate, 10.0% kcal fat). The HD and HD+CMS groups received a modified high-fat but not high-sugar HD, based on a purified moderately high fat (PMHF) diet (Lauterio et al. Citation1994) containing 4.41 kcal/g (16.8% kcal protein, 51.4% kcal carbohydrate, 31.8% kcal fat). In this study, all the butterfat (52 g) and corn oil (118 g) in the PMHF diet were replaced by coconut fat (44.2 g) and soybean oil (118 g), respectively. The soybean oil was used to replace corn oil because it was also used in the control diet, and because the literature has postulated that it may contribute to promoting insulin resistance in rodents (Bueno et al. Citation2008). The coconut fat replaced butterfat to increase the amount of saturated fatty acids (SFA). Coconut fat is a highly saturated fat (containing >76 g SFA/100 g fat), rich mainly in lauric, myristic and palmitic acids, which have been shown to be capable of promoting dyslipidemia in rats (Kamgang et al. Citation2005; Silva et al. Citation2006).
Stress protocol
The CMS protocol used in this study is based on the model described by Moreau (Citation1997) and modified according to Neves et al. (Citation2009). It consisted of the application of different stressors for 7 days per week for a period of three consecutive weeks (from the 3rd to 5th week) as described in . In this CMS model, a different stressor is presented every day and it does not allow the rodents to adapt to the stressors. The absence of adaptation was confirmed in a pilot study carried out in our laboratory; plasma corticosterone determinations showed that 1 and 15 days after the end of CMS, stressed rats had higher corticosterone concentrations (32.6 ± 2.4; 21.2 ± 0.9 ng/mL, respectively) in comparison with non-stressed rats (2.4 ± 0.4 ng/mL). As the aim of this study was to evaluate long-term effects of CMS, the metabolic and vascular evaluations were carried out 10 or 15 days after CMS. Control rats were subjected only to the procedures related to their normal care.
Table I. Chronic mild unpredictable stress procedure.
Oral glucose tolerance test and homeostasis model assessment (HOMA index)
Ten days after stress, the rats were fasted for 6 h prior to the collection of tail blood in heparin-coated tubes for subsequent determination of fasting glucose and insulin concentrations. Between 13:00 and 14:00 h, the rats were anaesthetized with Halothane, the tail was cut and blood samples were collected to determine the glucose and insulin fasting concentrations. Immediately afterwards, an oral glucose load (2 g/kg) solution was administered by oral gavage. Additional blood samples were collected without additional anesthesia at 30-, 60-, 90-, and 120-min time intervals. The blood collected for all analysis was obtained from the same site as the first bleeding, which was halted by applying a tourniquet. In each rat, only one tail cut was made. Plasma fasting insulin concentration was measured with a commercially available kit (LINCO Research®, St Charles, MO, USA) with a sensitivity of 3.4 pmol/L. Blood glucose was measured using the Prestige LX® glucosimeter. The homeostasis model assessment (HOMA) index was used to assess insulin resistance and it was calculated by the formula: [fasting plasma glucose (mmol/L) × fasting plasma insulin (μU/mL)]/22.5 (Søndergaard et al. Citation2006).
Analytic methods and tissue collection
All the rats were killed by decapitation 15 days after the end of stress, without previous anesthesia to avoid anesthetic-induced increase in plasma corticosterone concentrations (Vahl et al. Citation2005). Trunk blood was collected in heparin-coated tubes, and the plasma was used to determine corticosterone and lipids by enzymatic colorimetric assays. The thoracic aorta was isolated. Three depots of visceral white adipose tissue, which have been associated with insulin resistance and cardiovascular diseases, were carefully removed and weighed. The above-mentioned depots were the epididymal fat pad (around the testis and ductus deferens), retroperitoneal (located on the border between the spine and the posterior abdominal wall) and perirenal fat pad (along the posterior wall from the kidney to the hip region) (Cinti Citation2005; Tran and Kahn Citation2010). The ratio of total fat pad weight to final body weight was calculated (Levin et al. Citation2000).
Corticosterone was assayed using the Corticosterone Enzyme Immunoassay Kit (Assay Designs®, Ann Arbor, MI, USA), with sensitivity of 0.027 ng/mL, the intra- and inter-assay coefficients of variation being 7.7 and 9.7%, respectively. Total cholesterol (TC) was assayed using a commercially available kit (Laborlab®, Guarulhos, SP, Brazil), with sensitivity of 0.03 mmol/L; the intra- and inter-assay coefficients of variation were 3 and 2.7%, respectively. Triglycerides (TGL) were also assayed using the Laborlab® kit, with sensitivity of 0.02 mmol/L. High-density lipoprotein (HDL) was determined using the Laborlab® kit, with sensitivity of 0.01 mmol/L. The Friedewald's formula: low-density lipoprotein (LDL) = TC − HDL − (TGL × 0.2) was used to determine the plasma LDL concentrations (Friedewald et al. Citation1972). The atherogenic index (AI) was determined using the formula: AI = TC − HDL/TC (Kamgang et al. Citation2005).
Concentration-effect curves
The middle portion of thoracic aorta was excised free of fatty and connective tissue and then cut into two 3-mm-long to 5-mm-long rings. One ring was manipulated carefully to avoid damaging the endothelium, and the intimal surface of the second ring was scraped gently with a scalpel blade to remove the endothelial layer. The rings were suspended in a 20 mL organ bath containing Krebs–Henseleit solution. (Moura and Marcondes Citation2001). The aortic rings were connected to an isometric force transducer model 7004 (Ugo Basile, Varese, Italy), which was in turn connected to an Ugo Basile 2-channel Gemini recorder model 7070 (Ugo Basile) for registration of contractile responses. Concentration-effect curves (CEC) were obtained for aortic rings with and without endothelium to determine whether there were changes in the contractile activity of the thoracic aorta and whether the change was endothelium dependent, or was mediated directly by changes in the vascular smooth muscle (Cunha et al. Citation2005; Neves et al. Citation2009).
After 60 min of stabilization, the intactness of the endothelium was assessed by determining the vasodilating response to acetylcholine (1 μM) in rings pre-contracted with phenylephrine (0.1 μM). Only aortic rings that presented 100% relaxation by acetylcholine were considered to have an intact endothelium. The effectiveness of mechanical removal of the endothelium was confirmed by the complete absence of response to acetylcholine. The aortic rings were rinsed and allowed to re-equilibrate for 60 min. One cumulative CEC for phenylephrine (0.1 nM–0.1 mM) was obtained in each ring and the maximum response was evidenced when an increase in agonist concentration did not produce any additional response (Cunha et al. Citation2005; Neves et al. Citation2009). Changes in thoracic aorta sensitivity to phenylephrine were evaluated by determining the concentration that produced 50% of the maximum response and were expressed as the mean negative logarithm (pD2).
To evaluate the role of NO in modulating the thoracic aorta sensitivity to phenylephrine, aortic rings with intact endothelium, obtained from other rats subjected to the same treatments, were isolated as described above and were incubated with the NO synthesis inhibitor, NG-nitro-L-arginine methyl ester (l-NAME) (10 μM) for 40 min. A CEC for phenylephrine was obtained in the presence of l-NAME.
A CEC for acetylcholine was obtained in endothelium-intact thoracic aorta rings isolated from other rats subjected to the same treatments. In these experiments, the aortic rings with intact endothelium were pre-contracted with phenylephrine (1 μM). After stabilizing the contractile response to phenylephrine, acetylcholine (0.1 nM–0.1 mM) was cumulatively added into the bath medium to induce relaxation. The relaxation responses were expressed as percentages of the response to phenylephrine (1 μM).
The stock solutions of phenylephrine and acetylcholine (Sigma Chemical®, St Louis, MO, USA) were dissolved in 2% ascorbic acid and stored at − 10°C for 1 week. The dilutions for the CECs were prepared immediately before use and later discarded. For the Krebs–Henseleit solution, standard salts (Merk®, Darmstadt, Germany) were prepared in deionized water. The quality of the water was assessed weekly by measuring its conductivity and pH.
Morphometric study
To evaluate whether the functional changes in the aorta observed in the CEC were associated with morphological alterations, the inferior third of the thoracic aorta beginning 5 mm above the diaphragm muscle was placed in Karnovsky's fixative (Karnovsky Citation1965). In a previous analysis, we observed that the supersensitivity to phenylephrine observed in CEC obtained in the middle portion of thoracic aorta was also observed in its inferior third. Therefore, in order to decrease the number of rats used in the experiments, the morphometric study was carried out in the inferior third of the aorta isolated from the same rats from which aortas were used for obtaining the CEC to phenylephrine in the presence of l-NAME. The aorta fragments were washed in 1% osmium tetroxide in 0.1 M phosphate buffer, pH 7.3, for 2 h at room temperature and dehydrated with 50, 60, 70, 90, and 100% acetone. The fragments were embedded in Araldite-502 resin (Luft Citation1961), and polymerized for 48 h at 60°C.
Semi-thin (1 μm) transverse sections of the aorta were cut on a SORVALL® Porter-Blum MT2-B ultramicrotome with a glass knife, and collected at 10 μm intervals. The sections were stained with 0.5% toluidine blue in 1% sodium borate for 50 s followed by 1% basic fuchsin for 30 s. Five sections of each aorta in five rats per group were obtained, totaling 25 sections in each studied group for analysis by light microscopy.
The measurements of total intima-media thickness (IMT) were made using a photomicroscope (Carl Zeiss, Oberkochen, West Germany) connected to a millimeter eyepiece (Ernest leitz, Wetzlar, Germany, 12.5X), and using a millimeter ruler (Carl Zeiss 5+100/100 mm) for calibration. To calculate the IMT, 24 measurements were made in each section, using a 25X objective lens and 1.6X optovar, considering the IMT limits to be between the luminal surface and external elastic lamina. The IMT results are shown in micrometers (Neves et al. Citation2009).
Statistical analysis
Statistically significant differences were determined by two-way analysis of variance (ANOVA) followed by the Tukey test for multiple comparisons of means (p < 0.05). Stress and diet were considered the main factors, and their interaction was also analyzed. Values are presented as means ± SE of the means (SEM).
Results
Two-way ANOVA carried out on plasma corticosterone concentration evidenced a significant main effect of stress [F(1,44) = 60.14, p = 0.000, , without significant effect of diet (p = 0.883) or interaction (p = 0.634). Stressed rats showed a higher plasma corticosterone concentration in comparison with non-stressed groups (). There were no significant differences in initial body weight (p>0.05, ) among the four groups. However, 15 days after CMS, there was a significant main effect of stress [F(1,44) = 5.106, p = 0.029], and diet [F(1,44) = 30.198, p = 0.000] on final body weight, without significant interaction (p = 0.306, ). In the FP/BWf ratio, a significant stress/diet interaction [F(1,44) = 18.174, p = 0.000, was observed. The groups fed with a HD (HD and HD+CMS) presented a higher FP/BWf value when compared with the groups treated with the control diet (p = 0.000, Tukey's test, ). However, considering the effect of stress, stressed rats treated with a HD (HD+CMS) showed a higher FP/BWf when compared with non-stressed rats treated with the same diet (HD) (p = 0.000 with Tukey's test, ), without difference between stressed (CMS) and non-stressed (control) rats fed the control diet (p = 0.392 with Tukey's test, ).
Table II. Plasma concentrations of corticosterone and body weight of control and stressed rats, fed with control, or HD.
Two-way ANOVA carried out on TGL values evidenced significant effects of stress [F(1,44) = 10.102, p = 0.003, , and diet [F(1,44) = 7.251, p = 0.010], without significant interaction (p = 0.236). Significant main effects of stress [F(1.44) = 10.01, p = 0.003], and diet [F(1.44) = 9.86, p = 0.003, were also observed for TC plasma concentration, without significant effects for plasma concentration of HDL (p>0.05). With regard to LDL, there was a significant main effect of stress [F(1,44) = 5.881, p = 0.019, , and diet [F(1,44) = 8.777, p = 0.005, , but no interaction (p = 0.222). Similarly, for the AI, there was a significant effect of stress [F(1,44) = 10.782, p = 0.002, , and diet [F(1,44) = 16.746, p = 0.000, , without interaction (p = 0.526). Therefore, statistical analysis showed that the groups fed with a HD (HD and HD+CMS) presented higher plasma TGL concentrations, TC and LDL as well as higher AI values in comparison with the groups treated with the control diet (control and CMS). As regards the effects of stress, CMS increased the plasma concentrations of these lipids both in rats fed with the control and HD, and this effect was more pronounced in the HD+CMS group.
Figure 1. Effects of stress on plasma lipid concentrations of TGL, TC, HDL, and LDL in control rats, stressed rats subjected to CMS, non-stressed rats fed with HD, and rats fed with HD and subjected to CMS (HD+CMS), 15 days after the end of stress protocol (n = 12 rats per group). The AI for each of the four studied groups is presented on the right axis of graph (n = 12 rats per group). Data are mean ± SEM. *Significant difference vs. control (p < 0.05; two-way ANOVA + Tukey). †Significant vs. HD (p < 0.05; two-way ANOVA + Tukey). ‡Significant vs. CMS (p < 0.05; two-way ANOVA + Tukey).
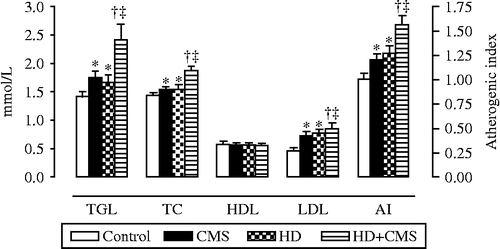
displays the mean fasting plasma glucose (Panel A) and insulin (Panel B) concentrations, area under the oral glucose tolerance test (OGTT) curve (AUC) (Panel C), and HOMA index (Panel D) of the four experimental groups. There was no significant difference in fasting glucose among the groups (p>0.05), but two-way ANOVA revealed a significant main effect of stress on fasting insulin [F(1,36) = 7.572, p = 0.009], AUC [F(1,28) = 38.065, p = 0.000], and on the HOMA index [F(1,36) = 9.169, p = 0.005], without significant effect of diet or interaction (p>0.05). These results showed that CMS increased the fasting insulin concentration, AUC and HOMA indices, but not the fasting glucose concentration in rats fed with the control or HD, in comparison with the non-stressed groups (control and HD) (). The HD did not change these parameters ().
Figure 2. Fasting plasma glucose (n = 10 rats/group), fasting plasma insulin (n = 10 rats/group) concentrations, area under the OGTT curve (AUC) (7–9 rats/group), and HOMA index (10 rats per group) carried out in control rats, stressed rats subjected to CMS, non-stressed rats fed with HD, and rats fed with HD and subjected to CMS (HD+CMS), 10 days after the end of stress protocol. Data are presented as mean ± SEM. *Significant difference vs. control (p < 0.05; two-way ANOVA + Tukey). †Significant vs. HD (p < 0.05; two-way ANOVA + Tukey).
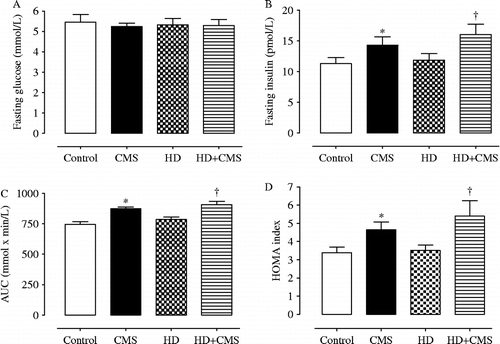
As the treatments did not modify the maximum response to phenylephrine and dry weight of aortic rings among the groups (p>0.05; ), the CEC were expressed as percentages of maximum response (). Considering pD2 values of phenylephrine of endothelium-intact rings (), two-way ANOVA revealed a significant main effect of stress [F(1,16) = 37.923, p = 0.000], without significant effect of diet or interaction (p>0.05). Therefore, stress induced supersensitivity to phenylephrine in the thoracic aorta with a 3.7-fold shift to the left in endothelium-intact aortic rings of the CMS group when compared with the control group (), and a 3.2-fold shift to the left of the HD+CMS group when compared with HD group (). Among the groups, there was no difference in CEC obtained in aortic rings without endothelium (p>0.05; ; ) or in CEC obtained in the presence of l-NAME (p>0.05; ; ). In the aorta isolated from the control and HD groups, pD2 values for phenylephrine obtained in the presence of l-NAME were higher in comparison with those pD2 values obtained in the endothelium-intact aortic rings of the same group. However, l-NAME did not change pD2 for phenylephrine in the aorta from the CMS and HD+CMS groups when compared with the endothelium-intact aorta (). Considering the CEC to acetylcholine, two-way ANOVA revealed a significant main effect of stress [F(1,19) = 8.522, p = 0.009] in the maximal acetylcholine-induced relaxation, with no effect of diet or interaction (p>0.05). Aortic rings isolated from stressed rats presented a markedly impaired maximal acetylcholine-induced relaxation, when compared with non-stressed groups (Control = 95.97 ± 8.22; CMS = 61.27 ± 6.14; HD = 94.21 ± 6.14; HD+CMS = 82.09 ± 7.29%) (p < 0.05, ).
Table III. Dry weight of aorta rings and maximum contractile response (MR) to phenylephrine in endothelium-intact, endothelium-denuded thoracic aortic rings and in the presence of l-NAME, isolated from control and stressed rats, fed with control or HD.
Figure 3. CEC for phenylephrine in endothelium-intact (A), endothelium-denuded (B), and in the presence of l-NAME in the endothelium-intact (C), and CEC to acetylcholine (D) obtained in the thoracic aorta isolated from control rats, stressed rats subjected to CMS, non-stressed rats fed with HD, and rats fed with HD and subjected to CMS (HD+CMS), obtained 15 days after stress protocol (N = 5–6 rats/group). Data are presented as mean ± SEM. *Significant difference vs. control (p < 0.05). †Significant vs. HD (p < 0.05).
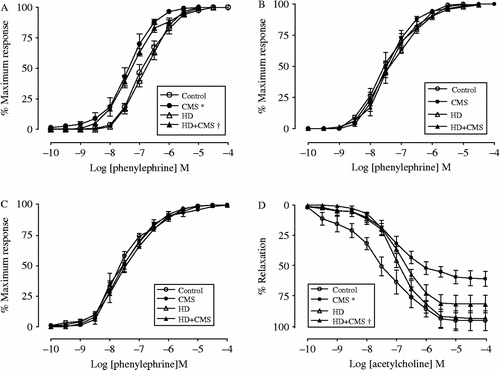
When considering aortic morphology, there was a significant main effect of stress on the aorta IMT [F(1,16) = 39.217, p = 0.000], without significant effect of diet or interaction (p>0.05). CMS and HD+CMS groups presented greater aorta IMT in comparison with the control and HD groups, respectively (Control = 91.3 ± 0.7 μm; CMS = 96.9 ± 1.1 μm; HD = 90.6 ± 1.2 μm; HD+CMS = 97.7 ± 1.0 μm, p < 0.05).
Discussion
This study showed that CMS induced insulin resistance, high concentration of lipids in the circulation, impaired the function and morphology of the thoracic aorta from the stressed groups, fed with control or high-fat but not high-sugar HD. The data indicate that CMS seems to be the determinant factor for these effects, since dyslipidemia induced by the high-fat HD alone was not sufficient to induce functional or morphological changes in the thoracic aorta.
As reported in other studies (Willner Citation1997; Marin et al. Citation2007), the high plasma corticosterone concentrations and lower final body weight observed after the end of the stress protocol in stressed rats in comparison with non-stressed rats, confirm the efficacy of the CMS protocol and support the absence of adaptation to stressors. The aim of this study was to analyze long-term effects of CMS. According to Moreau et al. (Citation1994), CMS promotes a state of anhedonia in rats, which can last for up to 20 days after stress. Hence, the purpose of waiting 15 days after the end of CMS was to analyze the level of corticosterone within this 20-day period of anhedonia. The sustained increase in the plasma corticosterone concentration 15 days after the end of stress confirms that CMS is an effective protocol to study the delayed effects of chronic stress in rats.
The higher values observed for final body weight, FP/BWf, and dyslipidemia with regard to the HD versus control diet indicate the effectiveness of HD treatment to disrupt the control of body weight. These effects observed in the HD and HD+CMS when compared with the control and CMS groups could be related to the high-fat content in the HD. The oleic acid present in soybean oil, and coconut fat in the presence of sucrose (Bueno et al. Citation2008) can readily be converted into acetate and then into cholesterol. The SFA contained in coconut fat, mainly lauric, myristic, and palmitic acids (Mendis et al. Citation2001), and in soybean oil (palmitic) (Bueno et al. Citation2008), could increase the production of TGL and cholesterol by the liver, and could also decrease LDL catabolism by repressing its receptors (Kamgang et al. Citation2005). In addition, when associated with oleic acid, palmitic acid (Black Citation2002) could increase the biosynthesis of chylomicrons (rich in TGL) (Silva et al. Citation2006).
Unlike the effects of a HD on lipids, in the present study, there were no differences in fasting plasma glucose and insulin concentrations between non-stressed rats fed with hypercaloric and control diets. Moreover, the HD alone did not induce insulin resistance. Although HD intake can be associated with hyperglycemia and insulin resistance, different authors have shown that these effects are often controversial, and seem to be dependent on a high supply of fat associated with high-sugar content. While a sucrose-rich diet impaired glucose homeostasis and induced insulin resistance before the increase in adiposity was detected (Soria et al. Citation2001), a diet rich in coconut oil and cholesterol without a significant increase in sugar content induced an increase in blood lipids accompanied by hypoglycemia and lower concentrations of serum insulin in rats (Zulet et al. Citation1999). In healthy women, replacing 4% of the energy in the form of palmitic or lauric acid by monounsaturated fatty acids had only minor effects on serum lipids and lipoproteins and caused no changes in glucose metabolism (Schwab et al. Citation1995). It has also been reported that the area under the curve during the intravenous glucose tolerance test was significantly lower, or did not change, after healthy women were treated with a diet enriched with monounsaturated fatty acid or SFA, respectively (Uusitupa et al. Citation1994). Therefore, the fact that the carbohydrate supply was not increased in the HD used in the present study could explain the absence of differences in glucose metabolism between the HD and control groups.
The duration of treatment also influences the metabolic effects of HDs. While the treatment of rats with a high-fat diet rich in saturated and unsaturated fatty acids for a period of 24 weeks increased the blood concentration of insulin and glucose, no differences were observed after 4 or 12 weeks of treatment in comparison with the group treated with low-fat diet (Song et al. Citation2006). Therefore, the results of the present study confirm that the effect of a HD on glucose metabolism is dependent on the association between high-fat and high-sucrose content, since all the rats in the present study were subjected to the same period of treatment and the HD had a higher lipid content but not a higher carbohydrate content in comparison with the control diet.
When considering the metabolic effects of chronic stress, in the present study, it was observed that CMS increased the blood lipid concentration, and the association between the HD and CMS potentiated the dyslipidemia as well as the increase in FP/BWf observed in the HD+CMS group in comparison with the control and HD groups. This effect could be related to a possible stress-induced desensitization or down-regulation of adrenoceptors in the fat cells (Black Citation2003), leading to lower catecholamine-induced lipolysis and increased adiposity in this group.
Another hypothesis is that the interaction between HD and CMS could have an additive effect on CMS-induced insulin resistance. In the present study, while the HD alone did not change glucose metabolism, CMS increased the fasting insulin concentrations, HOMA index values, and OGTT responses in stressed animals when compared with non-stressed rats. Therefore, the high TGL and TC concentrations in the circulation of the stressed groups may be a consequence of the insulin resistance promoted by the stress hormones. Under chronic stress conditions, corticosteroids may cause insulin resistance (Black Citation2002; Kyrou and Tsigos Citation2009), which facilitates TGL synthesis in the liver and may delay the clearance of lipoproteins, also resulting in hypercholesterolemia (Brindley et al. Citation1993). Moreover, the glucocorticoids may decrease the binding and degradation of LDL by liver cells (Brindley et al. Citation1993; Black Citation2002).
The metabolic effects of CMS observed in the present study are in agreement with the evidence that chronic stress interacts with dietary habits to determine the occurrence of metabolic diseases. In the chronic psychosocial stress model, it has been observed that subordination is associated with increased weight gain and dominance is associated with lower weight gain or weight loss in mice fed on a standard diet. However, opposite effects were observed in mice fed on a high-fat diet (Bartolomucci et al. Citation2009). It has been proposed that when chronic stress to which animals and humans cannot easily adapt is combined with high-fat high-sugar diets, it stimulates the sympathetic nerves to upregulate the expression of neuropeptide Y, which is an adrenergic co-transmitter and stress mediator. Stress and a HD also increase the glucocorticoid concentration in visceral fat, which in turn upregulates the expression of neuropeptide Y and its receptor Y2R, resulting in fat growth, hyperinsulinemia and hyperlipidemia (Kuo et al. Citation2008). In the present study, although there was a cumulative effect of HD plus CMS on the elevation of blood lipid concentration, it seems that this effect did not influence the increase in fasting insulin or lead to insulin resistance. This could be explained by the duration of treatment because in the present study the rats were treated for a period of 7 weeks.
Since dyslipidemia and insulin resistance are also associated with vascular diseases and high risk of atherogenesis, the function and morphology of the thoracic aorta were also evaluated in the present study. The use of adrenergic agonists, which are not substrates of the catecholamine uptake systems, are a tool for the study of the mechanisms involved in alterations of vascular reactivity. In experiments carried out in vitro, comparison among the responses of endothelium-intact and endothelium-denuded aortic rings, and CEC obtained in the presence and in the absence of inhibitors of NO synthesis, are used as pharmacological tools to investigate the role of the endothelium and NO, respectively, in the modulation of vascular activity. In addition, CEC to acetylcholine may provide a direct evaluation of NO involvement in vascular physiology. In the present study, CMS induced supersensitivity to phenylephrine in aortic rings with endothelium, and this effect was independent of diet. The absence of difference in the CEC obtained in the endothelium-denuded aortic rings among the groups indicates that CMS-induced supersensitivity to phenylephrine was endothelium dependent. The absence of difference between groups, in aortic rings incubated with l-NAME, which is an NO synthesis inhibitor, indicates that endothelial NO synthase (eNOS) seems to be inhibited in vivo, by some other factor not related to the addition of l-NAME in the incubation bath (Neves et al. Citation2009). This effect seems to stem from the exposure to CMS. The lower relaxation response to acetylcholine observed in the thoracic aorta isolated from stressed groups in comparison with control and HD groups indicates that CMS decreased NO bioavailability.
Considering that dyslipidemia induced by HD in non-stressed rats did not change the sensitivity to phenylephrine or acetylcholine in the thoracic aorta, it seems that the increase in corticosterone levels and insulin resistance induced by CMS could be the main factors involved in the vascular changes observed in the present study. Glucocorticoids reduce the expression of guanosine triphosphate cyclohydrolase 1 mRNA, which is necessary for the synthesis of the cofactor tetrahydrobiopterin, essential for stabilizing eNOS (Mitchell et al. Citation2004). Insulin stimulates endothelium-derived NO production (Muniyappa et al. Citation2008) and during insulin resistance, there is a decrease in endothelial eNOS activation and an increase in NO destruction by the reactive oxygen species. These effects result in diminished vasodilatation (Whaley-Connell and Sowers Citation2009) and increased endothelin-mediated vasoconstriction (Muniyappa and Quon Citation2007), and could also be involved in the effects of CMS.
Other authors have shown that the vascular effects of other stress protocols are dependent on the genetic background of the animals. Bernatova and Csizmadiova (Citation2006) showed that chronic crowding stress significantly reduced NO synthase activity in the aorta, and acetylcholine-induced relaxation of the femoral artery in borderline hypertensive adult rats with normotensive mothers. Bernatova et al. (Citation2007a) did not observe change in the activity of NO synthase in the aorta of Wistar normotensive rats subjected to crowding stress. In addition, Bernatova et al. (Citation2007b) showed that crowding stress increased the acetylcholine-induced relaxation in the femoral and mesenteric arteries from normotensive Wistar-Kyoto rats. While borderline hypertensive rats (BHR) subjected to crowding stress showed a reduction in NO synthase activity, suggesting a reduction in NO production, when compared with the control BHR, no changes were observed in Wistar normotensive rats (Okruhlicova et al. Citation2008). Cordellini et al. (Citation2006) studied the effect of acute and chronic stress by immobilization in Wistar and spontaneous hypertensive rats (SHR). These authors showed that the vascular adaptive response to stress involves hyperactivity of the endothelial NO system in normotensive rats. However, in the SHR strain, this adaptive response is impaired, independently of the hypertensive state.
In addition to the influence of genetic background, the differences observed between crowding and the vascular effects of CMS could also be related to stress intensity. Since crowding is a mild stress protocol with predictable stressors, and seems to be capable of eliciting adaptive mechanisms to prevent cardiovascular dysfunctions in normotensive rats, the repeated and unpredictable characteristics of the stressors used in the CMS protocol prevent the rodents from adapting, and seem to impair endothelial function. This hypothesis is supported by the high plasma corticosterone concentrations observed in stressed rats in the present study, and the absence of significant difference in plasma corticosterone between rats subjected to chronic crowding stress and control rats (Bernatova et al. Citation2007a).
Diminished NO availability could also be related to the increased aorta IMT in stressed groups (Okruhlicova et al. Citation2008). Morphological evaluation of IMT in the carotid artery is considered a marker of target organ damage in human hypertension (Sierra and de la Sierra Citation2008), and experimental studies (Razuvaev et al. Citation2008). Considering that decreased NO bioavailability may lead to the loss of mitogenic quiescence of vascular smooth muscle cells and consequent vessel wall hypertrophy (Costa and Assreuy Citation2005), the results of this study suggest that the aortic hypertrophy could be the result of the possible lack of NO elicited by the higher plasma corticosterone concentrations and insulin resistance induced by CMS.
Therefore, the proatherogenic and prohypertensive functional and morphological changes observed in the thoracic aorta from stressed rats are in agreement with the high AI values observed in both the CMS and HD+CMS groups. However, in spite of the increase in the AI value, non-stressed rats fed with the HD diet did not develop any alteration in the thoracic aorta. Another hypothesis to explain the absence of diet-induced deleterious vascular effects is that dyslipidemia has to be associated with high concentration of stress hormones or insulin resistance to be capable of generating vascular damages in rats. Further studies are needed to evaluate these hypotheses. These results indicate that rats, although resistant to the atherosclerosis induced only by diet (Moghadasian Citation2002), may present proatherogenic effects induced by CMS via insulin resistance.
In conclusion, the present study showed that the CMS protocol appears to be an appropriate model for the investigation of the cardiovascular and metabolic effects of stress. Investigation into the vascular damage associated with dyslipidemia induced by stress but not associated with dyslipidemia induced by the consumption of a high-fat, but not high-sugar, HD could help to clarify why some people present high cholesterol concentrations in the circulation in the absence of cardiovascular disease, while chronically stressed individuals could suffer heart attacks, for example. The association between glucocorticoids, insulin resistance, and dyslipidemia in rats may be one of the keys of association between chronic stress and cardiovascular diseases, and may be investigated in the CMS protocol providing insights for future clinical studies of the relationship between chronic stress and atherosclerosis.
Acknowledgements
The authors thank Margery Galbraith for English editing.
Declaration of interest: This work was supported by FAPESP and CNPq, Brazil. The authors report no conflicts of interest. The authors alone are responsible for the content and writing of the paper.
References
- Bartolomucci A, Cabassi A, Govoni P, Ceresini G, Cero C, Berra D, Dadomo H, Franceschini P, Dell'Omo G, Parmigiani S, Palanza P. 2009. Metabolic consequences and vulnerability to diet-induced obesity in male mice under chronic social stress. PLoS One. 4:e4331.
- Bernatova I, Csizmadiova Z. 2006. Effect of chronic social stress on nitric oxide synthesis and vascular function in rats with family history of hypertension. Life Sci. 78:1726–1732.
- Bernatova I, Csizmadiova Z, Kopincova J, Puzserova A. Vascular function and nitric oxide production in chronic social-stress-exposed rats with various family history of hypertension. J Physiol Pharmacol. 2007a; 58:487–501.
- Bernatova I, Puzserova A, Navarova J, Csizmadiova Z, Zeman M. Crowding-induced alterations in vascular system of Wistar-Kyoto rats: Role of nitric oxide. Physiol Res. 2007b; 56:667–669.
- Black PH. 2002. Stress and the inflammatory response: A review of neurogenic inflammation. Brain Behav Immun. 16:622–653.
- Black PH. 2003. The inflammatory response is an integral part of the stress response: Implications for atherosclerosis, insulin resistance, type II diabetes and metabolic syndrome X. Brain Behav Immun. 17:350–364.
- Brindley DN, McCann BS, Niaura R, Stoney CM, Suarez E. 1993. Stress and lipoprotein metabolism: Modulators and mechanisms. Metabolism. 42:3–15.
- Bueno AA, Oyama LM, de Oliveira C, Pisani LP, Ribeiro EB, Silveira VL, Oller do Nascimento CM. 2008. Effects of different fatty acids and dietary lipids on adiponectin gene expression in 3T3-L1 cells and C57BL/6J mice adipose tissue. Pflugers Arch. 455:701–709.
- Cinti S. 2005. The adipose organ. Prostaglandins Leukot Essent Fatty Acids. 73:9–15.
- Cordellini S, Novo R, Lanza UJr. 2006. Exposure to stress: Differential vascular adaptive response in spontaneously hypertensive and Wistar rats: Role of nitric oxide, and prohypertensive and hypertensive states. Life Sci. 79:646–653.
- Costa RS, Assreuy J. 2005. Multiple potassium channels mediate nitric oxide-induced inhibition of rat vascular smooth muscle cell proliferation. Nitric Oxide. 13:145–151.
- Cunha TS, Moura MJCS, Bernardes CF, Tanno AP, Marcondes FK. 2005. Vascular sensitivity to phenylephrine in rats submitted to anaerobic training and nandrolone treatment. Hypertension. 46:1010–1015.
- Friedewald WT, Levy RI, Fredrickson DS. 1972. Estimation of the concentration of low-density lipoprotein cholesterol in plasma without use of the preparative ultracentrifuge. Clin Chem. 18:499–502.
- Gu H, Tang C, Peng K, Sun H, Yang Y. 2009. Effects of chronic mild stress on the development of atherosclerosis and expression of toll-like receptor 4 signaling pathway in adolescent apolipoprotein E knockout mice. J Biomed Biotechnol. 613879:10–13.
- Kamgang R, Mboumi RY, N'dillé GP, Yonkeu JN. 2005. Cameroon local diet-induced glucose intolerance and dyslipidemia in adult Wistar rat. Diabetes Res Clin Pract. 69:224–230.
- Kaplan JR, Manuck SB, Clarkson TF, Lusso FM, Taub DM, Miller EW. 1983. Social stress and atherosclerosis in normocholesterolemic monkeys. Science. 220:733–735.
- Karnovsky MJ. 1965. A formaldehyde–glutaraldehyde fixative of high osmolarity for use in electron microscopy. J Cell Biol. 27:137A–138A.
- Kuo LE, Czarnecka M, Kitlinska JB, Tilan JU, Kvetnanský R, Zukowska Z. 2008. Chronic stress, combined with a high-fat/high-sugar diet, shifts sympathetic signaling toward neuropeptide Y and leads to obesity and the metabolic syndrome. Ann NY Acad Sci. 1148:232–237.
- Kyrou I, Tsigos C. 2007. Stress mechanisms and metabolic complications. Horm Metab Res. 39:430–438.
- Kyrou I, Tsigos C. 2009. Stress hormones: Physiological stress and regulation of metabolism. Curr Opin Pharmacol. 9:787–793.
- Lauterio TJ, Bond JP, Ulman EA. 1994. Development and characterization of a purified diet to identify obesity-susceptible and resistant rat populations. J Nutr. 124:2172–2178.
- Levin BE, Richard D, Michel C, Servatius R. 2000. Differential stress responsivity in diet-induced obese and resistant rats. Am J Physiol Regul Integr Comp Physiol. 279:R1357–R1364.
- Ludwigh DS. 2003. Novel treatments for obesity. Asia Pac J Clin Nutr. 12:S8.
- Luft JH. 1961. Improvements in epoxy resin embedding methods. J Biophys Biochem Cytol. 9:409–414.
- Marin MT, Cruz FC, Planeta CS. 2007. Chronic restraint or variable stresses differently affect the behavior, corticosterone secretion and body weight in rats. Physiol Behav. 90:29–35.
- Mendis S, Samarajeewa U, Thattil RO. 2001. Coconut fat and serum lipoproteins: Effects of partial replacement with unsaturated fats. Br J Nutr. 85:583–589.
- Mitchell BM, Dorrance AM, Mack EA, Webb RC. 2004. Glucocorticoids decrease GTP cyclohydrolase and tetrahydrobiopterin-dependent vasorelaxation through glucocorticoid receptors. J Cardiovasc Pharmacol. 43:8–13.
- Moghadasian MH. 2002. Experimental atherosclerosis: A historical overview. Life Sci. 70:855–865.
- Moreau JL. 1997. Validation of an animal model of anhedonia, a major symptom of depression. Encephale. 23:280–289.
- Moreau JL, Bourson A, Jenck F, Martin JR, Mortas P. 1994. Curative effects of the atypical antidepressant mianserin in the chronic mild stress-induced anhedonia model of depression. J Psychiatry Neurosci. 19:51–56.
- Moura MJCS, Marcondes FK. 2001. Influence of estradiol and progesterone on the sensitivity of rat thoracic aorta to noradrenaline. Life Sci. 68:881–888.
- Muniyappa R, Quon MJ. 2007. Insulin action and insulin resistance in vascular endothelium. Curr Opin Clin Nutr Metab Care. 10:523–530.
- Muniyappa R, Iantorno M, Quon MJ. 2008. An integrated view of insulin resistance and endothelial dysfunction. Endocrinol Metab Clin North Am. 37:685–711.
- Neves VJ, Moura MJCS, Tamascia ML, Ferreira R, Silva NS, Costa R, Montemor PL, Narvaes EAO, Bernardes CF, Novaes PD, Marcondes FK. 2009. Proatherosclerotic effects of chronic stress in male rats: Altered phenylephrine sensitivity and nitric oxide synthase activity of aorta and circulating lipids. Stress. 12:320–327.
- Okruhlicova L, Dlugosova K, Mitasikova M, Bernatova I. 2008. Ultrastructural characteristics of aortic endothelial cells in borderline hypertensive rats exposed to chronic social stress. Physiol Res. 57:S31–S37.
- Razuvaev A, Lund K, Roy J, Hedin U, Caidahl K. 2008. Noninvasive real-time imaging of intima thickness after rat carotid artery balloon injury using ultrasound biomicroscopy. Atherosclerosis. 199:310–316.
- Reeves PG, Nielsen FH, Fahey GCJr. 1993. AIN-93 purified diets for laboratory rodents: Final report of the American Institute of Nutrition ad hoc writing committee on the reformulation of the AIN-76A rodent diet. J Nutr. 123:1939–1951.
- Schwab US, Niskanen LK, Maliranta HM, Savolainen MJ, Kesäniemi YA, Uusitupa MI. 1995. Lauric and palmitic acid-enriched diets have minimal impact on serum lipid and lipoprotein concentrations and glucose metabolism in healthy young women. J Nutr. 125:466–473.
- Sheril A, Jeyakumar SM, Jayashree T, Giridharan NV, Vajreswari A. 2009. Impact of feeding polyunsaturated fatty acids on cholesterol metabolism of dyslipidemic obese rats of WNIN/GR-Ob strain. Atherosclerosis. 204:136–140.
- Shively CA, Register TC, Clarkson TB. 2009. Social stress, visceral obesity, and coronary artery atherosclerosis: Product of a primate adaptation. Am J Primatol. 71:742–751.
- Sierra C, de la Sierra S. 2008. Early detection and management of the high-risk patient with elevated blood pressure. Vasc Health Risk Manag. 4:289–296.
- Silva AP, Guimarães DE, Mizurini DM, Maia IC, Ortiz-Costa S, Sardinha FL, do Carmo MG. 2006. Dietary fatty acids early in life affect lipid metabolism and adiposity in young rats. Lipids. 41:535–541.
- Søndergaard LG, Stoltenberg M, Doering P, Flyvbjerg A, Rungby J. 2006. Zinc ions in the endocrine and exocrine pancreas of zinc deficient rats. Histol Histopathol. 21:619–625.
- Song GY, Gao Y, Di YW, Pan LL, Zhou Y, Ye JM. 2006. High-fat feeding reduces endothelium-dependent vasodilation in rats: Differential mechanisms for saturated and unsaturated fatty acids?. Clin Exp Pharmacol Physiol. 33:708–713.
- Soria A, D'Alessandro ME, Lombardo YB. 2001. Duration of feeding on a sucrose-rich diet determines metabolic and morphological changes in rat adipocytes. J Appl Physiol. 91:2109–2116.
- Tran TT, Kahn CR. 2010. Transplantation of adipose tissue and stem cells: Role in metabolism and disease. Nat Rev Endocrinol. 6:195–213.
- Uusitupa M, Schwab U, Sari Makimattila S, Karhapaa P, Sarkkinen E, Maliranta H, Agren J, Penttila I. 1994. Effects of two high-fat diets with different fatty acid compositions on glucose and lipid metabolism in healthy young women. Am J Clin Nutr. 59:1310–1316.
- Vahl TP, Ulrich-Lai YM, Ostrander MM, Dolgas CM, Elfers EE, Seeley RJ, D'Alessio DA, Herman JP. 2005. Comparative analysis of ACTH and corticosterone sampling methods in rats. Am J Physiol Endocrinol Metab. 289:E823–E828.
- Whaley-Connell A, Sowers JR. 2009. Hypertension and insulin resistance. Hypertension. 54:462–464.
- Willner P. 1997. Validity, reliability and utility of the chronic mild stress model of depression: A 10-year review and evaluation. Psychopharmacology (Berl). 134:319–329.
- Zulet MA, Barber A, Garcin H, Higueret P, Martínez JA. 1999. Alterations in carbohydrate and lipid metabolism induced by a diet rich in coconut oil and cholesterol in a rat model. J Am Coll Nutr. 18:36–42.