Abstract
Annual variation in day length (photoperiod) triggers changes in the immune system of seasonal breeders. The rationale behind this study was to delineate any sex differences in immune responses of photoperiodically entrained animals challenged against lipopolysaccharide (LPS)-induced inflammatory stress. We observed that photoperiodically entrained [short day, SD, 10 h light (L):14 h dark (D); long day, LD, 16 h L:8 h D; and natural day length, NDL, 12 h L:12 h D] male and female Indian palm squirrels, Funambulus pennanti, presented sexual dimorphism in immune status after LPS-induced stress. Females presented high humoral (anti-keyhole limpet hemocyanin immunoglobulin) and cellular immunity (lymphocyte proliferation) compared with the males of all photoperiodic conditions. Female squirrels showed reduced pro-inflammatory cytokine levels (interleukin-1β, interleukin-6, and tumor necrosis factor-α) than the males suggesting their high efficiency to recover from LPS-induced inflammatory stress. Increased duration of melatonin secretion and corticosterone concentration in squirrels experiencing SD evidently supported survival of squirrels as compared with control (NDL) and LD squirrels of both sexes. Decreased immune status in both sexes under LD condition might be due to a short melatonin signal mimicking the LDs of summer. Thus, we infer that photoperiodic entrainment via the levels of melatonin and corticosterone synergistically supported more the survival of female squirrels under LPS-induced stress.
Introduction
Exposure to lipopolysaccharide (LPS), a structural component of the cell wall of gram-negative bacteria, activates the innate immune system of terrestrial vertebrates and triggers several non-specific sickness responses including fever, lethargy, and reduced food intake (Exton Citation1997). The changes in normal physiology produce a stressful and challenging condition for the animal. Immune tissues respond to LPS challenge by producing cytokines that enter the circulation to trigger a cascade of immunological responses. Previous results suggest a direct relationship between cytokine production in the hypothalamus and sickness responses (Dantzer Citation2001). There are neuroendocrine responses such as suppression of the hypothalamo–pituitary–gonadal axis and a rapid and sustained activation of the hypothalamo–pituitary–adrenal (HPA) axis following stress induced by LPS (Dhabhar Citation2002). Normally, inflammatory stress acts via a feedback loop to the HPA axis, which stimulates synthesis and secretion of glucocorticoids by the adrenal cortex, which elicits pleiotropic responses that affect a number of different signaling pathways and eventually counter inflammation. Activation of the HPA axis associated with stress not only affects the central nervous system but also affects the immune system. Further, activation of the HPA axis results in elevation of circulating concentration of corticosteroids which then drives the release of cytokines from the thymus and spleen into the periphery to inhibit various immune activities (Dunn Citation2007).
In seasonal breeders, it is known that the annual cycle of changing photoperiod provides an accurate indication of time of year and thus allows immunological adjustments prior to the deterioration of conditions (Nelson and Demas Citation1996; Ahmad and Haldar Citation2009). Short day (SD) lengths enhance several aspects of immune function in laboratory studies, and melatonin appears to mediate many of the enhanced immunological effects of photoperiod (Nelson and Drazen Citation1999; Ahmad and Haldar Citation2009). Photoperiod affects the stress-induced immunological adjustments, suggesting that glucocorticoids, melatonin, and sex steroids may influence seasonal changes in immune activity in wild animals. Seasonal changes in the immune system are evident in many vertebrate species and have been hypothesized to have evolved to facilitate survival in terms of annual cycles in pathogen prevalence and seasonal stressors (Nelson et al. Citation1998). Further, sex differences in stress responses, including HPA axis regulation and glucocorticoid secretion, have been demonstrated in several rodent species (Shanks et al. Citation1994). Females often exhibit higher titers of immunoglobulins (Igs; e.g. IgG, IgM, and IgA) than males (Eidinger and Garrett Citation1972; Zuk and McKean Citation1996) and display a higher splenocyte blastogenic response to T and B cell mitogen than males (Krzych et al. Citation1981). To our knowledge, immune adjustment as a result of inflammatory stress due to LPS has not been systematically examined under different photoperiodic conditions in any tropical seasonally breeding rodent, and sexual dimorphism in this regard has not been reported. Hence, we planned to test immune adjustment and its efficiency in photoperiodically entrained male and female squirrels following endotoxin (LPS)-induced stress.
The tropical seasonal breeder Funambulus pennanti uses photoperiodic information and adjusts reproduction and the immune system accordingly to cope with the stress of seasonal fluctuations (Ahmad and Haldar Citation2009). Thus, enhanced immune function during the winter serves to counteract immunosuppressive stressors and thus increases the chances of survival. In this study, we hypothesized that immunocompetence of photoperiodically entrained F. pennanti against LPS-induced inflammatory stress would present sex-dependent variation and SD-exposed females would present higher immune responses than males and females exposed to long days (LDs).
For this purpose, both male and female squirrels were exposed to different photoperiods [natural day length (NDL), LDs, and SDs] and challenged with the endotoxin LPS. Following LPS stress under LD or SD conditions, cellular and humoral immunity was measured. To analyze the effect of inflammatory stress following LPS injection, we measured levels of pro-inflammatory cytokines [interleukin-1β (IL-1β), interleukin-6 (IL-6), and tumor necrosis factor-α (TNF-α)]. Thus, the aim of this study was (i) to evaluate any sex differences in humoral and cell-mediated immune responses in photoperiodically entrained male and female squirrels against inflammatory stress induced by LPS, and (ii) to explore the role played by glucocorticoid and melatonin in immune adjustment of photoperiodically entrained male and female squirrels against LPS-induced stress.
Materials and methods
Maintenance of animals
All the experiments were conducted in accordance with Institutional practice and within the framework of the revised Animal (specific procedure) Act of 2007 of the Government of India on animal welfare. The Indian Palm squirrel F. pennanti is an indigenous diurnal seasonal (LD) breeder, and is semi-domesticated (Haldar and Saxena Citation1988). The squirrels were collected from the vicinity of Varanasi (latitude 25° 18′N and longitude 83° 1′E). After two weeks of acclimatization to laboratory conditions (equivalent to ambient conditions), healthy young adult male and female squirrels of mean ( ± SEM) body weight 100 ± 10 g were randomly selected and divided into three major groups each of 28 squirrels (male/female squirrels). During the experiments, seven squirrels were maintained in separate wire net cages (25″ × 25″ × 30″ in size) in a well-ventilated room exposed to ambient conditions (ambient photoperiod, ∼12L:12D; average day/night temperature, ∼35/30°C; and humidity, ∼50%). Squirrels were fed with soaked gram seeds (Cicer arietinum), nuts, seasonal fruits/vegetables, and water ad libitum.
Experimental plan
One hundred and twelve young adult male and 112 female squirrels were randomly selected during August (ambient photoperiod, ∼12L:12D) from a large number of squirrels acclimatized to captivity. The squirrels were then exposed to different photoperiodic regimens, i.e. NDL (taken as control), 12L:12D (lights on 08:00 h and off 20:00 h), 10L:14D (SD; equivalent to the day length observed in December–January; lights on at 10:00 h and off at 20:00 h), and 16L:8D (LD; equivalent to the day length observed in May–June; lights on at 04:00 h and off at 20:00 h) in specially designed photoperiodic chambers. After 9 weeks of photoperiodic exposure, all squirrels were injected with endotoxin LPS during their respective photoperiodic schedule. At the end of the experiment (10 weeks exposure), the four groups of squirrels were divided further into three sets. Set I was killed at 22:00 h under ether anesthesia for collection of spleen and thymus for splenocyte and thymocyte culture and other immunological parameters. Set II was tested for delayed-type hypersensitivity (DTH) by application of the antigen oxazolone (Sigma Aldrich, St Louis, MO, USA). Set III was divided into two subsets (A and B) of seven squirrels in each for anti-keyhole limpet hemocyanin (KLH)-IgG assay (after KLH administration for 5 and 10 days). The experimental design is presented in . Another set of experiments was performed with the same number of male and female squirrels, and these were killed after 10 weeks of different photoperiodic exposure regimes to evaluate immune parameters without LPS challenge.
LPS treatment
All experimental groups of male and female squirrels experiencing different photoperiodic regimes (for 9 weeks) were given LPS injection (50 μg/100 μl in 0.9% saline, Sigma Aldrich, 026:B6) intraperitoneally (i.p.) for three consecutive days (24 h apart) in the 9th week. After LPS treatment, the squirrels were returned back to their respective photoperiodic regimens. At the end of the 10th week of exposure, Set I male and female squirrels were weighed and killed by decapitation under ether anesthesia. Lymphoid organs (spleen and thymus) and testes or ovaries were weighed. The spleen and thymus were processed for blastogenic response (percent stimulation ratio, % SR). Squirrels of Set II experiment were used for the DTH response study; Set III, subsets A and B, was given KLH (Sigma Aldrich) injection, to evaluate humoral immunity from serum anti-KLH-IgG titer. At the end of the experiment, animals were killed (at 22:00 h) by decapitation as described above. Trunk blood was collected in a sterile 15 ml tube, centrifuged at 4°C at 700g and serum was collected. Serum was stored at − 20°C for melatonin radioimmunoassay (RIA) and enzyme-linked immunosorbent assay (ELISA) of cytokines (IL-1β, IL-6, and TNF-α) and corticosterone. The diurnal peak of corticosterone secretion occurs in Indian palm squirrels at 12:00 h under NDL (Haldar et al. Citation2006).
Total leukocyte and percent lymphocyte count
Trunk blood was aspirated quickly before clotting along with Turk solution into the hemacytometer for the counting of leukocytes in a Neubauer's chamber slide under a microscope; lymphocytes (number/mm3) were counted in Leishman-stained preparations (Haldar and Singh Citation2001).
Blastogenic response (% SR)
The blastogenic response of splenocytes and thymocytes was evaluated as % SR from the incorporation of tritiated thymidine [3H] by untreated viable cells versus cells treated with Concanavalin-A (Con-A, Sigma-Aldrich, St. Louis, MO). Thymidine [3H] was counted as counts per minute (CPM) in a liquid scintillation β-counter (Beckman, Miami, FL). (Pauly and Soakal Citation1972; Haldar and Singh Citation2001) and % SR calculated as (CPM with Con-A/CPM without Con-A) × 100.
Delayed-type hypersensitivity
Squirrels were initially immunized by applying 100 μl of oxazolone [5% (wt/vol.), Sigma] to a shaved abdominal skin area for two consecutive days. On day 6, baseline ear thickness was measured with a Vernier caliper. DTH was tested by applying 50 μl of oxazolone [0.5% (wt/vol.) in 4:1, acetone: olive oil] to the skin of the dorsal surface of the right pinna; the left ear was treated with vehicle alone. Ear swellings were measured after every 24 h for three consecutive days to record the maximum DTH response, which was observed on the 2nd day. The DTH response was expressed as difference in thickness from the control pinna as percent of control pinna thickness (Dhabhar and McEwen Citation1999; Ahmad and Haldar Citation2009).
Anti-KLH-IgG estimation for humoral immunity
LPS injection on day 5 of the 9th week of the different photoperiod exposures (24 h after the last LPS injection), the squirrels received a subcutaneous injection of 150 μg of KLH (Sigma-Aldrich) in 0.1 ml sterile 0.9% saline (day 0). KLH was used because it generates a robust antigenic response in rodents, with minimal side effects (Dixon et al. Citation1966). Trunk blood was collected on day 5 or 10 to capture peak IgG production in response to KLH (Demas et al. Citation1997). Squirrels were anesthetized with i.p. injection of sodium pentobarbital (1.75 mg/kg body wt), killed, and trunk blood collected and allowed to clot; after 1 h, the clots were removed and the samples were centrifuged (at 4°C) for 30 min at 700g; serum aliquots were aspirated and stored at − 20°C until assayed for IgG.
Elisa For Anti-klh-igg
Humoral immunity was assessed by measuring serum anti-KLH-IgG concentrations using an ELISA (Demas et al. Citation1997). Microtiter plates were coated with antigen by overnight incubation at 4°C with 0.5 mg/ml KLH in sodium bicarbonate buffer, washed with phosphate-buffered saline containing 0.05% Tween 20 (PBST), blocked with 0.5% non-fat dry milk in PBST overnight at 4°C, and washed again with PBST. Thawed serum samples were diluted 1:100, 1:200, 1:400, and 1:800 with PBST, and 150 μl of each serum dilution was added in duplicate to the wells of the antigen-coated plates. Positive control samples (pooled serum from squirrels previously determined to have high levels of anti-KLH antibodies, similarly diluted with PBST) and negative control samples (pooled serum from squirrels never immunized with KLH) were also added in duplicate to each plate. The plates were sealed, incubated at 37°C for 3 h, and then washed with PBST. Secondary antibody (alkaline phosphatase-conjugated anti-mouse IgG diluted 1:500; SC-2320; Santa Cruz Biotech, Santa Cruz, CA) was added to the wells, and plates were sealed and incubated at 37°C for 1 h. Plates were again washed with PBST and 150 μl of the enzyme–substrate p-nitrophenyl phosphate (SRL Chemicals, Mumbai, India) at a concentration of 1 mg/ml dissolved in diethanolamine buffer (Sigma-Aldrich) was added to each well. Plates were protected from light during the enzyme–substrate reaction, which was terminated after 15 min by adding 50 μl of 1.5 M NaOH to each well. The optical density (OD) of each well was determined using a plate reader equipped with a 405-nm wavelength filter (ELx-800, Biotek Instruments, Winooski, VT, USA), and the average OD for each set of duplicate wells was calculated. To minimize intra- and inter-assay variability, the average OD for each sample was expressed as a percentage of its plate-positive control OD for statistical analyses. The mean absorbance value for the positive control was 1.24 nm. Intra- and inter-assay variation was < 6%.
Elisa For Cytokines (Il-1β, Il-6, And Tnf-α)
IL-6 and IL-1β were measured by ELISA (EIA Kit, Immunotech, Marseille, France) according to the manufacturer's instructions. Serum samples and standards were thawed, centrifuged at room temperature, and added in duplicate to a microplate coated with a monoclonal antibody against IL-1β and IL-6. OD of each microplate well was read at 450 nm using a microplate reader (ELx-800, Biotek Instruments). Sample values were determined by extrapolation from a standard curve. The IL-1β ELISA sensitivity was 1.5 pg/ml, intra-assay coefficient of variation (CV) was 2.5–9.4%, inter-assay CV was 3.7–11.5%, and recovery was 104–122%. IL-6 ELISA sensitivity was 5 pg/ml; intra-assay CV was 3.3–7.2%, inter-assay CV was 6.2–8.2%; recovery was 80–132%. TNF-α was assayed with an EIA kit from Immunotech, France, by a one immunological step sandwich-type assay method. Sample and standard were incubated in a microtiter plate coated with anti-TNF-α as first monoclonal antibody with the second anti-TNF-α monoclonal antibody linked to alkaline phosphatase. After washing the wells, the bound enzymatic activity was detected by addition of chromogenic substrate. Finally, the OD of each microplate well was read at 405 nm using a microplate reader (ELx-800, Biotek Instruments). Sample values were determined by extrapolation from a standard curve. The TNF-α ELISA has a sensitivity of 5 pg/ml, an intra-assay CV was 1.6–10%, inter-assay CV was 5.4–12.8%, and recovery was 80–113%. All samples, standards, and replicates were assayed in duplicate.
Corticosterone Elisa
Corticosterone was measured using an ELISA kit (provided by Prof. T. G. Srivastava, National Institute for Health and Family Welfare, New Delhi, India) according to the manufacturer's instructions. Serum samples were thawed at room temperature, and applied to a microplate. Following incubation, the plates were read on a microplate reader at 405 nm, and values were determined by extrapolation from a standard curve. The recovery, accuracy, and sensitivity of the corticosterone assay were 95%, 99.1%, and 0.27 μg/dl, respectively. Inter- and intra-assay variation was 3.38–5.56% and 5.69–7.84%, respectively. All samples were assayed in a single lot and samples, standards, and replicates were assayed in duplicate.
Melatonin RIA
Blood samples were taken and allowed to clot for 1 h, then centrifuged at 4°C for 30 min at 700g. Serum was collected and frozen at − 20°C until assayed for melatonin concentrations. The melatonin RIA was performed following the method of Rollag and Niswender (Citation1976) using Guildhey antisera (Guildhey, Surrey, UK). Details of the method and RIA validation have been published (Haldar and Dubey Citation1996). The intra- and inter-assay variation for melatonin was 9% and 15%, respectively; assay sensitivity was 18–20 pg/ml, and recovery was 92%.
Statistical analysis
To assess differences between means for all parameters, paired t-tests were used in Microsoft-Excel. Differences were considered statistically significant if p < 0.05. One-way ANOVA was performed in Microsoft-Excel to analyze the difference between individual groups of males with and without LPS treatment of control NDL and the three photoperiodic exposed groups (12L, 10L, and 16L). Data for female squirrels were analyzed similarly. Following significant F values, post-hoc tests (Tukey's honestly significant difference) were used to further distinguish between groups. All differences were considered statistically significant if p < 0.05. All statistical analysis was performed according to Bruning and Knitz (Citation1977).
To analyze the individual as well interactive effect of different factors, Factorial ANOVA 2 × 2 × 4 (α = 0.05) was also performed with three factors (sex, LPS, and photoperiod): (i) sex with two levels, i.e. male and female, (ii) LPS with two levels, i.e. LPS treated and non-LPS treated, and (iii) photoperiod with four levels, i.e. NDL, 12L, SD, and LD, for lymphoid organ weight, white blood cell counts, DTH response, RIA of hormones, and ELISA of cytokines. Further, for anti-KLH-IgG another factor, i.e. days (with two levels; 5 and 10 days), was included, and factorial ANOVA was carried out with other two factors, i.e. sex and photoperiod. For factorial ANOVA, SPSS-statistics software was used. Following a significant F-score, post-hoc tests (Tukey's as above) were also carried out for photoperiod factor to distinguish between different photoperiod groups. All differences were considered statistically significant if p < 0.05.
Results
Body weight and reproductive tissue weight
Significant difference was observed following paired t-test analysis in body weights of both male and female squirrels experiencing ambient NDL (Control-NDL), SD (10L:14D), and LD (16L:8D) photoperiodic regimens with or without LPS treatment (data not shown). Male and female squirrels with or without LPS treatment showed significant decrease in body weight under SD condition, whereas significant increase was observed under LD condition when compared with NDL male and female squirrels with or without LPS injection, respectively (data not shown). Testis and ovary weights were decreased significantly in SD squirrels with or without LPS treatment, whereas increased significantly after LD exposure with or without LPS treatment when compared with control NDL squirrels (data not shown).
Lymphoid organ weights
Females showed greater spleen and thymus weight than males for all the photoperiodic exposed groups with or without LPS treatment (t-tests; data not shown). Both male and female squirrels with or without LPS showed significant increase in spleen as well as thymus weight under SD (10L:14D), whereas significant decrease was observed under LD exposure (16L:8D) when compared with control (NDL) male and female squirrels with or without LPS injection, respectively (data not shown). Hence, these measures showed clear sex and photoperiod effects.
Total leukocyte and percent lymphocyte counts
Females showed significantly increased percent lymphocyte (% LC) and total leukocyte (TLC) when compared with males of all the photoperiodic exposed groups in both categories, i.e. with or without LPS treatment (significant t-values; data not shown). Both male and female squirrels with or without LPS injection showed significantly increased % LC and TLC under SD (10L:14D), whereas significant decrease was observed under LD exposure (16L:8D) when compared with control (NDL) male and female squirrels with or without LPS injection, respectively (data not shown). Hence, these measures also showed clear sex and photoperiod effects.
Delayed-type hypersensitivity (%)
Female squirrels with or without LPS on all photoperiod regimes showed significant (p < 0.01) increase in the % DTH response when compared with male squirrels as shown by t-values (data not shown). Further, males (F3,24 = 92.07; MSe = 19.6; p < 0.01; α = 0.05) and females (F3,24 = 133.4; MSe = 24.5; p < 0.01; α = 0.05) without LPS treatment presented an increased DTH response under the SD condition, and a decreased response under the LD condition when compared with control NDL squirrels (). Similarly, following LPS treatment, the males (F3,24 = 144.6; MSe = 24.8; p < 0.01; α = 0.05) and females (F3,24 = 229.2; MSe = 25.5; p < 0.01; α = 0.05) showed a significantly increased DTH response under SD condition, but a decreased response under the LD condition when compared with NDL squirrels ().
Figure 2. LPS treatment and DTH (pinna swelling) under different photoperiods. Squirrels were sensitized and tested by cutaneous oxazolone application; NDL, 12L:12D, 12 h light 12 h dark; SD, 10L:14D; LD, 16L:8D, N = 7 per group. Data are Mean +/ − SEM. **p < 0.01 vs. respective female treatment in the same photoperiodic group; paired t-test; a: p < 0.01 vs. control males in NDL group; b: p < 0.01 control females in NDL group; c: p < 0.01 vs. LPS-treated males in NDL group; d: p < 0.01 vs. LPS-treated females in NDL group; one-way ANOVA followed by post-hoc test Tukey's HSD. M − : untreated, control, male; F − : untreated, control, female; M+: LPS-treated male; F+: LPS-treated female.
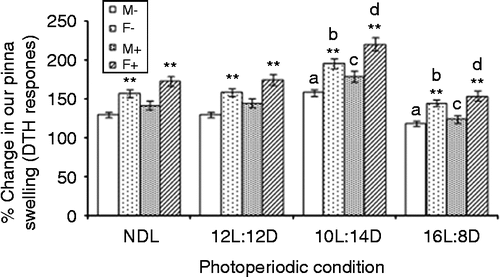
F-value for the sex factor (F1,96 = 1207; MSe = 23.64; p < 0.01; α = 0.05) showed significant difference in the mean values of the % DTH response. Further, LPS treatment (F1,96 = 230.9; MSe = 23.64; p < 0.01; α = 0.05) and photoperiod (F3,96 = 588.8; MSe = 23.64; p < 0.01; α = 0.05) significantly affected the % DTH response. Following factorial ANOVA (2 × 2 × 4) at α = 0.05, only the sex × photoperiod interaction (F3,96 = 10.57; MSe = 23.64; p < 0.01) significantly affected the DTH response whereas sex × LPS and sex × LPS × photoperiod showed no significant effect.
Percent SR splenocytes and thymocytes
Females on all the photoperiod regimes showed significantly increased % SR for both splenocytes and thymocytes when compared with male squirrels (data not shown).
Males (F3,24 = 285.1; MSe = 22.2; p < 0.01; α = 0.05) and females (F3,24 = 346.1; MSe = 29.7; p < 0.01; α = 0.05) without LPS treatment showed increased % SR of splenocytes under the SD condition, but decreased % SR under the LD condition when compared with control NDL squirrels (). LPS-treated males (F3,24 = 396.6; MSe = 19.7; p < 0.01; α = 0.05) and females (F3,24 = 302.2; MSe = 34.9; p < 0.01; α = 0.05) showed significantly increased % SR of splenocytes under the SD condition, but decreased % SR under the LD condition when compared with NDL squirrels ().
Figure 3. LPS treatment and % SR of splenocytes (a) and thymocytes (b) under different photoperiods. NDL, 12L:12D, 12 h light 12 h dark; SD, 10L:14D; LD, 16L:8D, N = 7 per group. Data are Mean +/ − SEM. Measurements were by thymidine [3H] incorporation in vitro. **p < 0.01 vs. respective female treatment in the same photoperiodic group; paired t-test; a: p < 0.01 vs. control males in NDL group; b: p < 0.01 control females in NDL group; c: p < 0.01 vs. LPS-treated males in NDL group; d: p < 0.01 vs. LPS-treated females in NDL group; one-way ANOVA followed by post-hoc test Tukey's HSD. M − : untreated, control, male; F − : untreated, control, female; M+: LPS-treated male; F+: LPS-treated female.
![Figure 3. LPS treatment and % SR of splenocytes (a) and thymocytes (b) under different photoperiods. NDL, 12L:12D, 12 h light 12 h dark; SD, 10L:14D; LD, 16L:8D, N = 7 per group. Data are Mean +/ − SEM. Measurements were by thymidine [3H] incorporation in vitro. **p < 0.01 vs. respective female treatment in the same photoperiodic group; paired t-test; a: p < 0.01 vs. control males in NDL group; b: p < 0.01 control females in NDL group; c: p < 0.01 vs. LPS-treated males in NDL group; d: p < 0.01 vs. LPS-treated females in NDL group; one-way ANOVA followed by post-hoc test Tukey's HSD. M − : untreated, control, male; F − : untreated, control, female; M+: LPS-treated male; F+: LPS-treated female.](/cms/asset/e0096e5d-b2ab-4e06-9ca8-b10a756e9daa/ists_a_606515_f0003_b.gif)
Similarly, % SR of thymocytes increased under the SD condition whereas it decreased under the LD condition in the males with LPS (F3,24 = 194.9; MSe = 35.6; p < 0.01; α = 0.05) or without LPS (F3,24 = 127; MSe = 31.6; p < 0.01; α = 0.05) treatment when compared with their counterparts under NDL condition. Percent SR increased under SD condition significantly whereas it decreased significantly under the LD condition in females with LPS (F3,24 = 429.8; MSe = 33.4; p < 0.01; α = 0.05) or without LPS (F3,24 = 190.5; MSe = 36.2; p < 0.01; α = 0.05) treatment when compared with the females under the NDL condition ().
A significant difference was observed between the mean values for % SR of splenocytes of the male and female squirrels (F1,96 = 1207; MSe = 26.69; p < 0.01; α = 0.05). Further, LPS treatment (F1,96 = 205.6; MSe = 26.69; p < 0.01; α = 0.05) and photoperiod (F3,96 = 1292; MSe = 26.69; p < 0.01; α = 0.05) significantly affected % SR of splenocytes. Following factorial ANOVA (2 × 2 × 4) at α = 0.05, only the sex × photoperiod interaction (F3,96 = 18.38; MSe = 26.69; p < 0.01) significantly affected splenocyte proliferation whereas sex × LPS and sex × LPS × photoperiod showed no significant effect on % SR of splenocytes. Similarly % SR of thymocytes also showed significant difference for the mean values of the male and female squirrels (F1,96 = 1211; MSe = 33.6; p < 0.01; α = 0.05). Further, LPS treatment (F1,96 = 328; MSe = 33.6; p < 0.01; α = 0.05) and photoperiod (F3,96 = 922; MSe = 33.6; p < 0.01; α = 0.05) significantly affected % SR of thymocytes. At α = 0.05, the interaction between sex × photoperiod (F3,96 = 49.7; MSe = 33.6; p < 0.01) and sex × LPS (F1,96 = 10.3; MSe = 33.6; p < 0.01) significantly affected thymocyte proliferation whereas the sex × LPS × photoperiod interaction showed no significant effect.
Serum anti-KLH-IgG assay
Serum anti-KLH-IgG titer was measured at two different times (5 and 10 days). Both male and female squirrels presented significantly increased anti-KLH-IgG production at 10 days post-immunization when compared with squirrels at 5 days under NDL as well as the other three photoperiodic groups. Hence, the level of anti-KLH-IgG at 10 days is presented. At 5 days as well as 10 days after treatment, a significantly increased anti-KLH-IgG titer was observed in females experiencing all types of photoperiodic conditions, including NDL when compared with that in the males of all the respective groups (data not shown).
At 10 days post-treatment, anti-KLH-IgG level was significantly increased under SD whereas it decreased under LD in males with LPS (F3,24 = 143.5; MSe = 12.7; p < 0.01; α = 0.05) or without LPS (F3,24 = 81.8; MSe = 13.46; p < 0.01; α = 0.05) treatment when compared with their counterparts under control NDL. Similarly, increased anti-KLH-IgG level was observed under SD whereas a significant decrease was noted in females under LD with LPS (F3,24 = 150.5; MSe = 16.16; p < 0.01; α = 0.05) or without LPS (F3,24 = 75.38; MSe = 15.57; p < 0.01; α = 0.05) treatment when compared with females under NDL ().
Figure 4. LPS treatment and KLH-IgG titer (as % plate positive) under different photoperiods. NDL, 12L:12D, 12 h light 12 h dark; SD, 10L:14D; LD, 16L:8D, N = 7 per group. Data are Mean +/ − SEM. **p < 0.01 vs. respective female treatment in the same photoperiodic group; paired t-test; a: p < 0.01 vs. control males in NDL group; b: p < 0.01 control females in NDL group; c: p < 0.01 vs. LPS-treated males in NDL group; d: p < 0.01 vs. LPS-treated females in NDL group; one-way ANOVA followed by post-hoc test Tukey's HSD. M − : untreated, control, male; F − : untreated, control, female; M+: LPS-treated male; F+: LPS-treated female.
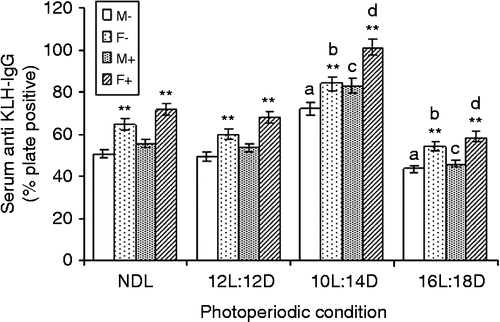
Following factorial ANOVA (2 × 2 × 4), F-value of the sex factor (F1,208 = 22.12; MSe = 86.17; p < 0.01; α = 0.05) showed a significant difference in the mean anti-KLH-IgG levels of males and females. Significant difference in the mean values at 5 and 10 days for anti-KLH-IgG was also observed (days: F1,208 = 81.31; MSe = 86.17; P < 0.01; α = 0.05). Photoperiod also showed a significant effect on anti-KLH-IgG level. A significant difference in mean values of the anti-KLH-IgG response was observed among the different photoperiodic groups (photoperiod: F3,208 = 64.66; MSe = 86.17; P < 0.01; α = 0.05). Further, at α = 0.05 an interaction between sex × days (F3,208 = 13.9; MSe = 86.17; p < 0.01) and sex × photoperiod (F3,208 = 2.91; MSe = 86.17; p < 0.05) interaction significantly affected anti-KLH-IgG level, whereas sex × days × photoperiod interaction showed no significant effect.
Circulating concentrations of melatonin and corticosterone
Significantly greater serum melatonin concentration was recorded in females under all four photoperiodic regimens than in males under the respective photoperiodic conditions with or without LPS treatment (significant t-statistic values; data not shown). Serum melatonin concentration increased under the SD regimen whereas it decreased under the LD condition in males with LPS (F3,24 = 592.4; MSe = 21.43; p < 0.01; α = 0.05) or without LPS (F3,24 = 501.2; MSe = 24.17; p < 0.01; α = 0.05) treatment compared with that in their counterparts under control NDL. Similarly, the SD condition increased serum melatonin concentration and decreased it significantly under the LD condition in females with LPS (F3,24 = 874.3; MSe = 18.04; p < 0.01; α = 0.05) or without LPS (F3,24 = 719.7; MSe = 21.86; p < 0.01; α = 0.05) treatment compared with females under NDL ().
Figure 5. LPS treatment and serum melatonin (a) and corticosterone (b) concentrations under different photoperiods. NDL, 12L:12D, 12 h light 12 h dark; SD, 10L:14D; LD, 16L:8D, N = 7 per group. Data are Mean +/ − SEM. **p < 0.01 vs. respective female treatment in the same photoperiodic group; paired t-test; a: p < 0.01 vs. control males in NDL group; b: p < 0.01 control females in NDL group; c: p < 0.01 vs. LPS-treated males in NDL group; d: p < 0.01 vs. LPS-treated females in NDL group; one-way ANOVA followed by post-hoc test Tukey's HSD. M − : untreated, control, male; F − : untreated, control, female; M+: LPS-treated male; F+: LPS-treated female.
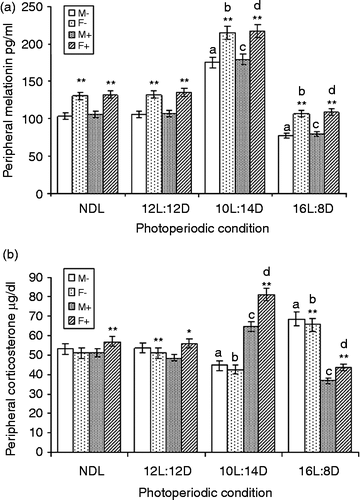
Following factorial ANOVA (2 × 2 × 4), significant difference was recorded in the mean values of the serum melatonin concentration of males and females (F1,96 = 1125.5; MSe = 21.38; p < 0.01; α = 0.05). Further, LPS treatment (F1,96 = 6.31; MSe = 21.38; p = 0.01; α = 0.05) and photoperiod (F3,96 = 2624; MSe = 21.38; p < 0.01; α = 0.05) significantly affected melatonin concentration. At α = 0.05, only the sex × photoperiod interaction (F3,96 = 10.37; MSe = 21.38; p < 0.01) significantly affected melatonin concentration, whereas sex × LPS and sex × LPS × photoperiod showed no significant effect.
In non-LPS-treated squirrels, there was no sex difference in serum corticosterone under NDL and SD. There was a significant decrease in the females kept under 12L:12D and LD condition when compared with the respective non-LPS-treated males (data not shown). LPS treatment significantly increased the serum corticosterone concentration of the females under all four photoperiods when compared with the respective males (paired t-test; data not shown). Serum corticosterone concentration decreased under SD and increased under LD in males without LPS treatment (F3,24 = 28.8; MSe = 24.69; p < 0.01; α = 0.05) when compared with NDL males. LPS treatment increased the corticosterone concentration in males under SD but decreased it under LD condition (F3,24 = 63.3; MSe = 14.55; p < 0.01; α = 0.05) when compared with their counterparts under the control NDL condition ().
Similarly, non-LPS-treated females showed decreased serum corticosterone concentration under SD, which increased on LD (F3,24 = 20.73; MSe = 30.54; p < 0.01; α = 0.05). LPS treatment increased the corticosterone concentration under SD which decreased under LD (F3,24 = 102.7; MSe = 16.53; p < 0.01; α = 0.05) when compared with NDL females (). Factorial ANOVA (2 × 2 × 4) showed a significant difference in serum corticosterone concentration of males and females (F1,96 = 13.44; MSe = 21.58; p < 0.01; α = 0.05). Further, LPS treatment (F1,96 = 4.31; MSe = 21.58; p < 0.05; α = 0.05) and photoperiod (F3,96 = 9.65; MSe = 21.58; p < 0.01; α = 0.05) significantly altered corticosterone concentration. At α = 0.05, only the sex × photoperiod (F3,96 = 2.77; MSe = 21.58; p < 0.05) and the sex × LPS (F1,96 = 41.7; MSe = 21.58; p < 0.01) interaction significantly affected corticosterone concentration; sex × LPS × photoperiod showed no significant effect.
Cytokine Elisa (Il-1β, Il-6, And Tnf-α)
Serum concentrations of the pro-inflammatory cytokines, i.e. IL-1β, IL-6, and TNF-α, were significantly less in females in all the photoperiod conditions when compared with those of the respective males (paired t-test; data not shown). IL-1β concentration was significantly decreased in males under SD but increased under LD with LPS (F3,24 = 354.4; MSe = 19.02; p < 0.01; α = 0.05) or without LPS (F3,24 = 210.9; MSe = 33.67; p < 0.01; α = 0.05) treatment compared with their counterparts under NDL. Similarly, females showed decreased IL-1β concentration under SD, which increased under LD with LPS (F3,24 = 463.06; MSe = 14.2; p < 0.01; α = 0.05) or without LPS (F3,24 = 192.18; MSe = 30.7; p < 0.01; α = 0.05) treatment compared with NDL females ().
Figure 6. LPS treatment and serum IL-1β (a), IL-6 (b), and TNF-α (c) concentrations under different photoperiods. NDL, 12L:12D, 12 h light 12 h dark; SD, 10L:14D; LD, 16L:8D, N = 7 per group. Data are Mean +/ − SEM. **p < 0.01 vs. respective female treatment in the same photoperiodic group; paired t-test; a: p < 0.01 vs. control males in NDL group; b: p < 0.01 control females in NDL group; c: p < 0.01 vs. LPS-treated males in NDL group; d: p < 0.01 vs. LPS-treated females in NDL group; one-way ANOVA followed by post-hoc test Tukey's HSD. M − : untreated, control, male; F − : untreated, control, female; M+: LPS-treated male; F+: LPS-treated female.
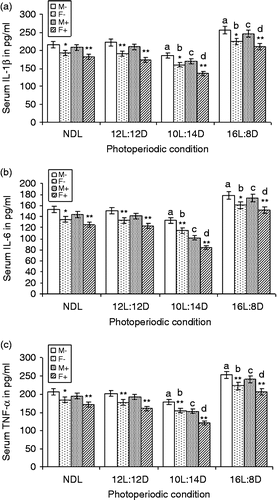
Serum IL-6 concentration was also decreased under SD and increased under LD males with LPS (F3,24 = 441.62; MSe = 13.82; p < 0.01; α = 0.05) or without LPS (F3,24 = 135.7; MSe = 24.8; p < 0.01; α = 0.05) treatment compared with that of their NDL counterparts. Similarly, females had decreased serum IL-6 concentration under SD and increased concentration under LD with LPS (F3,24 = 358.5; MSe = 15.2; p < 0.01; α = 0.05) or without LPS (F3,24 = 130.4; MSe = 22.4; p < 0.01; α = 0.05) treatment compared with NDL females (). Serum TNF-α concentration also decreased under SD and increased under LD in males with (F3,24 = 475.5; MSe = 18.4; p < 0.01; α = 0.05) or without LPS (F3,24 = 349.2; MSe = 20.09; p < 0.01; α = 0.05) treatment compared that with respective males under NDL. Females with LPS (F3,24 = 556.9; MSe = 15.57; p < 0.01; α = 0.05) or without LPS (F3,24 = 267.6; MSe = 23.3; p < 0.01; α = 0.05) treatment showed decreased serum TNF-α concentration under SD and increased concentration under LD compared with the LDS females ().
Factorial ANOVA (2 × 2 × 4) showed significant difference in the serum IL-1β concentration in male and female squirrels (F1,96 = 1090; MSe = 24.42; p < 0.01; α = 0.05). LPS treatment (F1,96 = 308.5; MSe = 24.42; p < 0.01; α = 0.05) and photoperiod (F3,96 = 1075; MSe = 24.42; p < 0.01; α = 0.05) significantly affected IL-1β level. Sex × LPS (F1,96 = 18.83; MSe = 24.42; p < 0.01; α = 0.05) and sex × photoperiod (F3,96 = 2.98; MSe = 24.42; p < 0.05; α = 0.05) interaction significantly affected IL-1β level, but the sex × LPS × photoperiod showed no significant effect.
Significant differences were observed for serum concentrations of IL-6 in male and female squirrels (F1,96 = 538.2; MSe = 19.09; p < 0.01; α = 0.05). LPS treatment (F1,96 = 308.5; MSe = 19.09; p < 0.01; α = 0.05) and photoperiod (F3,96 = 896.6; MSe = 19.09; p < 0.01; α = 0.05) significantly affected serum IL-6 concentration. Only the sex × photoperiod (F3,96 = 2.86; MSe = 19.09; p < 0.05; α = 0.05) interaction significantly affected IL-6 level, whereas sex × LPS and sex × LPS × photoperiod showed no significant effect. Significant differences were found for serum TNF-α concentration in male and female squirrels (F1,96 = 1178; MSe = 19.37; p < 0.01; α = 0.05). LPS treatment (F1,96 = 581; MSe = 19.37; p < 0.01; α = 0.05) and photoperiod (F3,96 = 1568; MSe = 19.37; p < 0.01; α = 0.05) significantly affected serum TNF-α concentration. Sex × LPS (F1,96 = 17.16; MSe = 19.37; p < 0.01; α = 0.05) and sex × photoperiod (F3,96 = 2.79; MSe = 19.37; p < 0.05; α = 0.05) interactions significantly affected TNF-α level, whereas sex × LPS × photoperiod showed no significant effect.
Discussion
In general, exposure to LPS results in an acute inflammatory response characterized by the activation of immune cells and the release of cytokines into the circulation (Wen and Prendergast Citation2007). In this study, there were sexually dimorphic responses to the endotoxin LPS in immune responses of photoperiodically entrained squirrels. Following LPS treatment, humoral and cellular immunity increased in squirrels entrained to SD, whereas it decreased in squirrels of both sexes entrained to LD when compared with control NDL squirrels. High-serum corticosterone, melatonin, and low pro-inflammatory cytokine (IL-1β, IL-6, and TNF-α) levels in SD-entrained squirrels indicate an adaptive organization against LPS-induced stress compared with LD and 12L:12D entrained squirrels of both sexes. However, female squirrels with lower immune parameters, corticosterone, melatonin, and cytokine serum levels than the males under all photoperiodic conditions, indicates significant adaptability to counter inflammatory stress induced by LPS. Enhanced release of pro-inflammatory cytokines (IL-1β, IL-6, and TNF-α) after LPS results in a series of immune and endocrine responses that mimic bacterial infection (Klein and Nelson Citation1999; Prendergast et al. Citation2007).
Endogenous glucocorticoids have potent inhibitory effects on sickness behaviors (Goujon et al. Citation1997) and attenuate pro-inflammatory cytokine production in the periphery (Pezeshki et al. Citation1996). Therefore, in SD-exposed squirrels, increased corticosterone level might have inhibited the behavioral and thermoregulatory responses to LPS on one hand and decreased the levels of IL-1β, IL-6, and TNF-α in the circulation on the other. In addition to inhibiting cytokine production, SD might have suppressed the expression of sickness responses via a parallel effect on the HPA axis. We found that female squirrels were evidently more responsive to presumed corticosterone suppression of pro-inflammatory cytokines than male squirrels.
In this study, increased immune cell numbers (TLC, % LC, DTH) in SD squirrels were consistent with SD upregulation of HPA axis activity. Female squirrels under SD condition showed higher level of corticosterone than males (under SD condition) following LPS challenge which might have contributed to decreasing pro-inflammatory cytokines level (IL-1β, IL-6, and TNF-α) more effectively. After LPS treatment, IL-1β, IL-6, and TNF-α levels were significantly higher in LD squirrels than in SD, 12L:12D and NDL squirrels. Longer duration of melatonin secretion under SD condition could also be one of the reasons for recovery of squirrels as compared to LD (shorter duration of melatonin secretion) and control squirrels (NDL and 12L:12D) under LPS-induced stress, as melatonin is known to have an anti-inflammatory effect (Cuzzocrea and Reiter Citation2002).
Further, we also suggest that the decreased production of cytokines in SD squirrels may reflect an adaptive reorganization (i.e. attenuation) to prepare them for the adverse conditions of winter. Thus, Indian palm squirrels utilize photoperiodic information to orchestrate their responses before the onset of challenging environmental conditions (winter). Interestingly, immune reactions in SD-entrained female squirrels were more efficient than in SD-entrained males, and than in LD-entrained squirrels of both the sexes. Decrease in pro-inflammatory cytokine production and increase in corticosterone concentration under SD could be a result of altered day length, which enhanced the immune responses.
Males and females often differ in the amount and type of stress they experience and encounter, especially during the breeding season. Thus, differential exposure to stressors and subsequent changes in glucocorticoid secretion influence the allocation of resources to immune function (Zuk and McKean Citation1996). Laboratory studies in rodents have demonstrated that basal corticosterone concentrations are higher in females and increase more rapidly in females than in males after exposure to stressors (Critchlow et al. Citation1963). Importantly, stress responses and the production of glucocorticoids should be considered as a part of the mechanisms underlying seasonal changes in immune function. High serum concentration of corticosterone was observed in female squirrels experiencing all the three photoperiodic groups as compared with that the male squirrels. Contrary to the idea that glucocorticoids suppress immune function, the observed increase in corticosterone concentration under SD evidently as a stimulator of immune function and was associated with a significant increase in cellular as well as humoral immune responses in the squirrels.
Glucocorticoids act on multiple targets to enhance or inhibit various cellular activities, an action adapted to provide the altered metabolic, endocrine, nervous, and immunological needs necessary for survival (Turnbull and Rivier Citation1999). The present findings indicate that interactions between reproductive condition and stress responses together may moderate immune responses in female squirrels. Females are reported to exhibit higher adrenocorticotrophic hormone responses to stress, and increased baseline and stress-induced glucocorticoid release than males (Shanks et al. Citation1994). Along with corticosterone, melatonin is also reported to attenuate the LPS-induced production of cytokines and prostaglandins (Nava et al. Citation1997) as well as fever (Wen and Prendergast Citation2007). Thus, melatonin along with corticosterone may have enabled SD-exposed squirrels, especially females, to cope against stress and pro-inflammatory cytokines.
Sexual dimorphism in humoral immune function could provide an adaptive advantage for seasonal breeders, at least during winter, using SD length as a signal to “switch on” the enhanced immune function associated with winter to give greater protection from environmental stressors (Ahmad and Haldar Citation2009). Female squirrels on SD showed an enhanced skin DTH response compared with SD-exposed males and LD-exposed females. DTH reactions are antigen-specific, cell-mediated immune responses and have generally been considered as a measure of effector T-cell function, but also involve immunological memory for a specific antigen. Here, the present findings in SD squirrels indicate enhanced inflammatory responses dependent on memory T cells, as reported for Siberian hamsters by Bilbo et al. (Citation2002a).
KLH, which is a potent antigen (Dixon et al. Citation1966), sensitized the squirrels and provoked anti-KLH-IgG production. The squirrels showed sustained effect of photoperiod on T-cell-dependent humoral immunity. SD females showed the highest anti-KLH-IgG titer as compared with SD males, and with 12L:12D, control NDL and LD squirrels of either sex. Photoperiod significantly affected IgG production following immunization with LPS in both the male and female squirrels. KLH-induced Ig production was greater in females than in the males under SD. This finding is consistent with the prevailing hypothesis of enhanced antibody production in response to infection in females compared with males among a variety of species, including the Syrian hamster (Schuurs and Verheul Citation1990).
In summary, photoperiod regulated immune responses in the tropical rodent F. pennanti and provided differential ability (depending upon day length) to adjust against stress induced by LPS. Decreases in pro-inflammatory cytokine production and increases in corticosterone production under the SD condition might be the mechanism by which day length alters the immune response to LPS, an infection mimic stressor. Future research is needed to test causal relationships, indicated in this study, between changes in melatonin and corticosterone production with photoperiod and immune responses to infection, and how these are influenced by sex (i.e. roles of gonadal hormones). This study found that environmental and sex influences on immune surveillance and function are profound.
Acknowledgments
The authors thank the Indian Council Medical Research, New Delhi, for financial support as Junior Research Fellow to Dr Raise Ahmad. Department of Science and Technology, New Delhi, supported the work, and instrument provided by Alexander von Humboldt Foundation, Bonn, Germany, is gratefully acknowledged.
Declaration of interest: The authors report no conflicts of interest. The authors alone are responsible for the content and writing of the paper.
References
- Ahmad R, Haldar C. 2009. Photoperiod-testicular-immune interaction in a seasonal breeder Indian palm squirrel Funambulus pennanti during the reproductively inactive and active phases. J Neuroendocrinol. 21:2–9.
- Bilbo SD, Dhabhar FS, Viswanathan K, Saul A, Yellon SM, Nelson RJ. Short day lengths augment stress-induced leukocyte trafficking and stress-induced enhancement of skin immune function. Proc Natl Acad Sci USA. 2002a; 99:4067–4072.
- Bruning JL, Knitz BL. 1977. Computational handbook of statistics. Palo Alto, CA: Scott, Foresman and Company.
- Critchlow V, Liebelt RA, Bar-Sela M, Mountcastle W, Lipscomb HS. 1963. Sex difference in resting pituitary adrenal function in the rat. Am J Physiol. 205:807–815.
- Cuzzocrea S, Reiter RJ. 2002. Pharmacological actions of melatonin in acute and chronic inflammation. Curr Top Med Chem. 2:153–165.
- Dantzer R. 2001. Cytokine-induced sickness behavior: Where do we stand?. Brain Behav Immun. 15:7–24.
- Demas GE, Chefer V, Talan MI, Nelson RJ. 1997. Metabolic costs of mounting an antigen-stimulated immune response in adult and aged C57BL/6J mice. Am J Physiol. 273:R1631–R1637.
- Dhabhar FS. 2002. A hassle a day may keep the doctor away: Stress and the augmentation of immune function. Integr Comp Biol. 42:556–564.
- Dhabhar FS, McEwen BS. 1999. Enhancing versus suppressive effects of stress hormones on skin immune function. Proc Natl Acad Sci USA. 96:1059–1064.
- Dixon F, Jacot-Guillarmod H, McConahey PJ. 1966. The antibody responses of rabbits and rats to hemocyanin. J Immunol. 97:350–355.
- Dunn AJ. 2007. The HPA axis and the immune system: A perspective. NeuroImmun Biol. 7:3–15.
- Eidinger D, Garrett TJ. 1972. Studies of the regulatory effects of the sex hormones on antibody formation and stem cell differentiation. J Exp Med. 136:1098–1116.
- Exton MS. 1997. Infection-induced anorexia: Active host defence strategy. Appetite. 29:369–383.
- Goujon E, Laye S, Parnet P, Dantzer R. 1997. Regulation of cytokine gene expression in the central nervous system by glucocorticoids: Mechanisms and functional consequences. Psychoneuroendocrinology. 22:S75–S80.
- Haldar C, Dubey S. 1996. Diurnal variations in circulating estradiol, testosterone, melatonin and harderian gland porphyrin concentration in Indian palm squirrel, Funambulus pennanti. Indian J Exp Biol. 34:695–697.
- Haldar C, Saxena N. 1988. Pineal gland and humidity effects on testicular function of the Indian palm squirrel, Funambulus pennanti. J Pineal Res. 5:411–418.
- Haldar C, Singh R. 2001. Pineal modulation of thymus and immune function in a seasonally breeding tropical rodent, Funambulus pennanti. J Exp Zool. 289:90–98.
- Haldar C, Sharma S, Singh SS. 2006. Reproductive phase dependent circadian variations of plasma melatonin, testosterone, thyroxine and corticosterone in Indian palm squirrel, Funambulus pennanti. Biol Rhythm Res. 37:1–10.
- Klein SL, Nelson RJ. 1999. Activation of the immune-endocrine system with lipopolysaccharide reduces affiliative behaviors in voles. Behav Neurosci. 113:1042–1048.
- Krzych U, Strausser HR, Bressler JP, Goldstein AL. 1981. Effects of sex hormones on some T and B cell functions, evidenced by differential immune expression between male and female mice and cyclic pattern of immune responsiveness during the estrous cycle in female mice. Am J Reprod Immunol. 1:73–77.
- Nava F, Calapai G, Facciola G, Cuzzocrea S, Giuliani G, De Sarro A, Caputi AP. 1997. Melatonin effects on inhibition of thirst and fever induced by lipopolysaccharide in rats. Eur J Pharmacol. 331:267–274.
- Nelson RJ, Demas GE. 1996. Seasonal changes in immune function. Q Rev Biol. 71:511–548.
- Nelson RJ, Drazen DL. 1999. Melatonin mediates seasonal adjustments in immune function. Reprod Nutr Dev. 39:383–398.
- Nelson RJ, Demas GE, Klein SL. 1998. Photoperiodic mediation of seasonal breeding and immune function in rodents: A multifactorial approach. Integr Comp Biol. 38:226–237.
- Pauly JL, Soakal JE. 1972. A simplified technique for in vitro studies of lymphocytes reactivity. Proc Soc Exp Biol Med. 140:40–44.
- Pezeshki G, Pohl T, Schobitz B. 1996. Corticosterone controls interleukin-1 beta expression and sickness behavior in the rat. J Neuroendocrinol. 8:129–135.
- Prendergast BJ, Kampf-Lassin A, Yee JR, Galang J, McMaster N, Kay LM. 2007. Winter day lengths enhance T lymphocyte phenotypes, inhibit cytokine responses, and attenuate behavioral symptoms of infection in laboratory rats. Brain Behav Immun. 21:1096–1108.
- Rollag MD, Niswender GD. 1976. Radioimmunoassay of melatonin in sheep exposed to different light regimes. Endocrinology. 98:482–488.
- Schuurs AHWM, Verheul HAM. 1990. Effects of gender and sex steroids on the immune response. J Steroid Biochem. 35:157–172.
- Shanks N, McCormick CM, Meaney MJ. 1994. Sex differences in hypothalamic–pituitary–adrenal responding to endotoxin challenge in the neonate: Reversal by gonadectomy. Brain Res Dev Brain Res. 79:260–266.
- Turnbull AV, Rivier CL. 1999. Regulation of the hypothalamic–pituitary–adrenal axis by cytokines: Actions and mechanisms of action. Physiol Rev. 79:1–71.
- Wen JC, Prendergast BJ. 2007. Photoperiodic regulation of behavioral responsiveness to pro-inflammatory cytokines. Physiol Behav. 90:717–725.
- Zuk M, McKean KA. 1996. Sex differences in parasite infections: Patterns and processes. Int J Parasitol. 26:1009–1024.