Abstract
It is established that stress impairs spatial learning and memory via the hypothalamus–pituitary–adrenal axis response. Dopamine D1 receptors were also shown to be responsible for a stress-induced deficit of working memory. However, whether stress affects the subsequent emotional learning and memory is not elucidated yet. Here, we employed the well-established one-trial step-through task to study the effect of an acute psychological stress (induced by tail hanging for 5, 10, or 20 min) on emotional learning and memory, and the possible mechanisms as well. We demonstrated that tail hanging induced an obvious stress response. Either an acute tail-hanging stress or a single dose of intraperitoneally injected dopamine D1 receptor antagonist (SCH23390) significantly decreased the step-through latency in the one-trial step-through task. However, SCH23390 prevented the acute tail-hanging stress-induced decrease in the step-through latency. In addition, the effects of tail-hanging stress and/or SCH23390 on the changes in step-through latency were not through non-memory factors such as nociceptive perception and motor function. Our data indicate that the hyperactivation of dopamine D1 receptors mediated the stress-induced deficit of emotional learning and memory. This study may have clinical significance given that psychological stress is considered to play a role in susceptibility to some mental diseases such as depression and post-traumatic stress disorder.
Introduction
Extensive studies have focused on the effects of acute stress in regulating hippocampal-dependent spatial memory and synaptic plasticity (Kim and Yoon Citation1998; Garcia Citation2001). Mechanistic studies have demonstrated that the hypothalamus–pituitary–adrenal (HPA) axis response to stress plays a critical role. The corticosterone and glucocorticoid receptor-mediated signaling pathway in the hippocampus is the leading step to induced cognitive and plasticity deficits. Recently, the role of adrenergic activity in the basolateral amygdala was also shown to mediate hippocampal-dependent spatial memory deficits and synaptic plasticity impairments after stress (Roozendaal et al. Citation2004). In addition, it has been shown that prefrontal cortex-dependent working memory is impaired after acute stress (Arnsten Citation2007; Srikumar et al. Citation2007), and evidence demonstrates that the stress-induced working memory deficit is via excessive release of dopamine in prefrontal cortex (Arnsten and Goldman-Rakic Citation1998; Arnsten et al. Citation2000; Morrow et al. Citation2000) because the impairment is prevented by a selective dopamine D1 receptor antagonist, SCH23390 (Arnsten and Goldman-Rakic Citation1998). However, whether acute stress affects the subsequent emotional learning and memory is not elucidated yet.
The one-trial step-through task, a passive avoidance paradigm, which depends on hippocampus and amygdala functions, is considered to be a model of emotional learning and memory. In addition, an HPA axis response and increased level of dopamine in the hippocampus and amygdala after acute stress have been reported (Inglis and Moghaddam Citation1999; Miura et al. Citation2005). This leads to the hypothesis that acute stress affects learning and memory through hyperactivation of dopamine mechanisms in the hippocampus and amygdala. Therefore, we explored this by employing the passive avoidance paradigm and determined the effect of an acute tail-hanging stress on emotional learning and memory in mice, and investigated the underlying mechanisms associated with corticosterone or dopamine.
Materials and methods
Animals
This study was approved by the Ethics and Experimental Animal Committee of Kunming Institute of Zoology, Chinese Academy of Sciences, and by the National Institutes of Health Guidelines for the Care and Use of Laboratory Animals (NIH Guidelines). All experiments were conducted during the light phase. Collection of blood samples was performed under acute anesthesia, and all efforts were made to minimize the suffering. The mice used were 4–6 weeks old, body weight 20 ± 2 g (mean ± SEM) males and females of the Kunming strain (Animal House Center, Kunming Medical College, Kunming). Mice were cage housed (no more than five mice in one cage, usually three mice per cage) and given free access to food and water in an established facility with a 12:12 h light/dark cycle (with lights on at 08:00 am and lights off at 20:00 pm), 50 ± 10% humidity and thermoregulated environment (22.0 ± 1.0°C). All subjects were allowed to acclimate to handling by the experimenter and the laboratory environment for one week before the beginning of the experiments. The mice were randomly allocated to different groups: (1) three stressed groups—mice were stressed for 5, 10, and 20 min, and mice were subjected to behavior test or blood collection immediately after stress; (2) stressed with 20 min recovery groups—subjects were stressed for 20 min, and the behavior test or blood collection was then carried out 20 min after stress; (3) SCH23390-treated groups—SCH23390 at doses of 0.0125, 0.025, 0.05 mg/kg was injected (intraperitoneally, i.p), to individual mice of each group. Behavior tests were performed 20 min after injection; (4) SCH23390 plus stress group—immediately after injection of SCH23390 (0.05 mg/kg), mice were stressed for 20 min, and then the behavior test was performed immediately after that stress; (5) time-matched controls and/or 0.9% saline (vehicle)-injected control groups.
Drug treatment
SCH23390 (Sigma, St. Louis, MO) was dissolved in 0.9% saline immediately before injection. Drug solution (0.0125, 0.025, 0.05 mg/kg SCH23390, respectively) or saline (0.2 ml per subject) was injected (i.p) to mice individually.
Stress protocol
Rodents are naturally afraid of height, but mice are much more active than rats and difficult to keep on an elevated platform. Here, psychological stress was evoked by hanging the tail for 5, 10, or 20 min in each group, respectively. Mice were suspended by using sticky tape to fix the tail (2 cm to tip) to a horizontal shaft that was 1.5 m high above the ground in the center of the experimental room. The stress exposure was carried out in the light phase between 09:00 am and 10:30 am.
Serum preparation and corticosterone concentration measurement
Blood was collected by cardiac puncture under ether anesthesia, and this process was completed in less than 3 min for each mouse. The blood samples were placed in tubes at 4°C and centrifuged after clotting to obtain serum samples. Serum corticosterone concentration (ng/ml) was determined as described before (Gao et al. Citation2008) by radioimmunoassay, using a commercial kit with intra-assay variability 2.3–3.9% coefficient of variation, high sensitivity (1.6 ng/ml) and wide assay range (6.20–2000 ng/ml) (Diagnostic Systems Laboratories, Webster, TX).
One-trial step-through task
The one-trial step-through task was conducted using a GEMINI passive avoidance system (San Diego Instruments, San Diego, CA) as previously described (Wang and Cai Citation2008). Briefly, the mice were exposed to three experimental sessions, including an adaptation session (AS), training session (TS), and retention session (RS) with 24 h intervals. Each mouse was allowed to become familiar with the avoidance system for 5 min during the AS, was trained by delivering a foot shock (0.33 mA, 3 s) during TS, and tested for memory performance during RS (a cut-off time of 300 s was set). In this study, mice were treated with stress and/or drug before TS (not AS and RS).
Nociception test
The hot-plate test was conducted to evaluate the effects of the psychological stress and/or SCH23390 on nociception of mouse. This test was performed with an aluminum slab bonded to thin high-surface heating elements providing even temperature to the entire test area (Yinhe CS-501, Chongqing, China). The heating plate was kept at a constant temperature of 55.0 ± 0.5°C. Each mouse was individually placed on the plate to test the latency to licking its paw or jumping from the plate. A 40 s cut-off time was used to prevent injury.
Data analysis
Data are expressed as mean ± SEM. Data were evaluated by ANOVA with least significance difference (LSD) post-hoc tests (using SPSS13.0 program), and the significance level was set at p < 0.05.
Results
Acute tail hanging is an effective psychological stressor for mice
We found that there is a stress duration-dependent increase in serum corticosterone concentration, which gradually increased from 5 to 10 and 20 min exposure, and reached statistical significance in the last two groups compared to control mice (F(4,33) = 12.417, p < 0.001; ). This stress-induced increase in serum corticosterone concentration did not decrease significantly in 20 min following tail-hanging stress (F(4,33) = 12.417, p>0.05; ). Meanwhile, we found that the mice showed behavioral signs of stress during the tail-hanging treatment such as “freezing” immobility, piloerection, urination, and defecation. Taken together, we demonstrated here that acute tail hanging is an effective psychological stressor for mice.
Figure 1. The effect of the acute tail-hanging stress on serum corticosterone concentration. Blood samples were collected immediately after tail-hanging stress for 5, 10, or 20 min (A), or 20 min after the stress for 20 min (B). The data are mean ± SEM. **p < 0.01 vs. control group, ANOVA and LSD post-hoc tests, n = 6–8 per group.
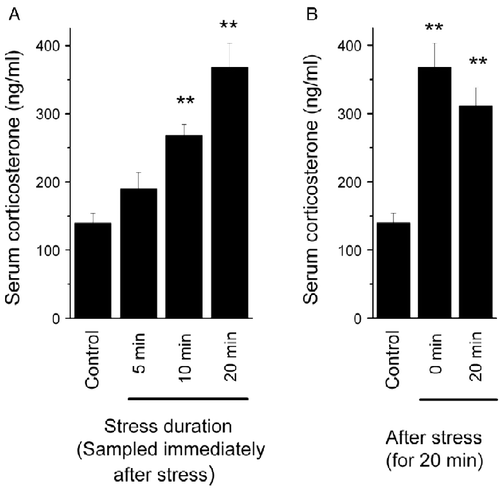
Acute tail-hanging stress and/or SCH23390 have no significant effects on nociception
Using the hot-plate test, we tested the effects of the acute tail-hanging stress and/or SCH23390 on nociception. It was found that immediately after acute tail-hanging stress for 10 or 20 min, the latency in the hot-plate test tended to increase, by about 25% when compared to control mice, although this was not statistically significant (F(6,61) = 0.212, p>0.05; ). Giving 20 min rest time following stress or administration of SCH23390 (0.025, 0.05 mg/kg) did not significantly alter the latency in the hot-plate test (F(6,61) = 0.212, p>0.05; ). This suggested that nociception was not significantly altered by acute tail-hanging stress or SCH23390.
Figure 2. Effects of acute tail-hanging stress on pain perception in the hot-plate test, and of the dopamine D1 receptor antagonist (SCH23390). The latency to licking paw or jumping from the plate was not significantly different among the experimental groups (p>0.05). The data are mean ± SEM and were evaluated by ANOVA, n = 8–10 per group.
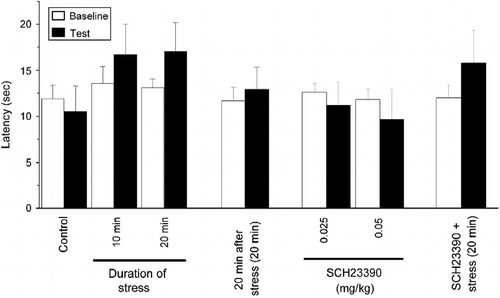
Emotional learning and memory were impaired by an acute tail-hanging stress via hyperactivation of dopamine D1 receptors
We found that the step-through latency in the RS was significantly decreased in mice trained immediately after a tail-hanging stress for 10 or 20 min, when compared to control mice (F(3,39) = 9.501, p < 0.05 and p < 0.001, respectively; ). However, the step-through latency in the RS was not significantly decreased in stressed mice if they were trained 20 min after tail-hanging stress for 20 min (F(2,28) = 6.265, p>0.05 compared to control; ), although the corticosterone concentration was still high when they were trained (see for detail). In addition, we found that SCH23390 dose-dependently shortened the step-through latency in the RS of the one-trial step-through behavior test (F(3,64) = 6.731; p < 0.05 for control vs. 0.025 mg/kg SCH23390 group, and p < 0.001 for control vs. 0.05 mg/kg SCH23390 group; ). This is consistent with previous results showing that the dopamine D1 receptor antagonist SCH23390 impairs amygdala-dependent memory formation and retrieval in a fear-conditioning task (Pezze and Feldon Citation2004). However, we found that the step-through latency shortened by stress was rescued by pharmacological blockade of dopamine D1 receptors with SCH23390 (0.05 mg/kg) (F(3,38) = 7.602, p < 0.01; ). The change in step-through latency in the one-trial step-through task in the RS is possibly due to the alteration in motor function or pain perception rather than a memory deficit. However, we did not find a significant change in nociception by stress or SCH23390 (see above and ). Additionally, in the one-trial step-through paradigm, the latency in the TS represents motivation, excitatory/inhibitory state of the nervous system, and motor function, which have been seen as non-memory factors. The data were analyzed using a two-way ANOVA, and it revealed that, among mice stressed by tail hanging, there were no significant effect on sex (F(1,35) = 1.052, p = 0.312), group (F(3,35) = 1.000, p = 0.404), or sex × group interaction (F(3,35) = 1.606, p = 0.206). The data for SCH23390-treated mice were analyzed as well, and similarly, no significant effects on sex (F(1,60) = 1.322, p = 0.391), group (F(3,60) = 0.863, p = 0.465), or sex × group interaction (F(3,60) = 1.204, p = 0.225) were observed. Therefore, we concluded that those non-memory factors were not significantly changed by stress or SCH23390.
Figure 3. Emotional memory was impaired by an acute tail-hanging stress via hyperactivation of dopamine D1 receptors. The step-through latency in the RS was gradually decreased by stress depending on the duration of prior tail hanging, when they were trained immediately after stress (A) (*p < 0.05, **p < 0.01 vs. control). However, the stress-induced decrease of step-through latency was prevented when they were trained 20 min after stress (B) [**p < 0.01 vs. control; ##p < 0.01 vs. stressed without 20 min recovery (0 min) mice]. SCH23390 dose-dependently decreased step-through latency in the RS of the one-trial step-through task (C) (*p < 0.05, **p < 0.01 vs. saline-injected control). However, administration of 0.05 mg/kg SCH23390 effectively prevented the stress-induced shortening of step-through latency (D) (**p < 0.01 vs. saline control; #p < 0.05; ##p < 0.01 vs. SCH23390+stress group). The data are mean ± SEM and were evaluated by ANOVA and LSD tests, n = 9–18 per group.
![Figure 3. Emotional memory was impaired by an acute tail-hanging stress via hyperactivation of dopamine D1 receptors. The step-through latency in the RS was gradually decreased by stress depending on the duration of prior tail hanging, when they were trained immediately after stress (A) (*p < 0.05, **p < 0.01 vs. control). However, the stress-induced decrease of step-through latency was prevented when they were trained 20 min after stress (B) [**p < 0.01 vs. control; ##p < 0.01 vs. stressed without 20 min recovery (0 min) mice]. SCH23390 dose-dependently decreased step-through latency in the RS of the one-trial step-through task (C) (*p < 0.05, **p < 0.01 vs. saline-injected control). However, administration of 0.05 mg/kg SCH23390 effectively prevented the stress-induced shortening of step-through latency (D) (**p < 0.01 vs. saline control; #p < 0.05; ##p < 0.01 vs. SCH23390+stress group). The data are mean ± SEM and were evaluated by ANOVA and LSD tests, n = 9–18 per group.](/cms/asset/d457692b-e03e-4df4-b89b-068046e041bb/ists_a_607525_f0003_b.gif)
Discussion
In this study, we took the advantage of the fact that rodents are naturally afraid of height, and being at a high elevation induces psychological stress. However, mice are more active than rats and difficult to keep on an elevated platform. Therefore, the established elevated platform stress protocol for rats is not suitable for mice. Here, according to behavioral responses, psychological stress was evoked by hanging the tail for 5, 10, and 20 min. In this study, the mice showed behavioral signs of stress during the tail-hanging treatment such as “freezing” immobility, piloerection, urination, and defecation. Immunoassay results also revealed that the serum corticosterone concentration was significantly increased by tail hanging. Therefore, it is inferred that tail hanging was an effective psychological stressor for mice. We consider this a useful finding, given the large number of studies requiring mice to explore molecular mechanisms of stress.
The passive avoidance test is based on the brief pain caused by foot shocks and its associated emotional response, so changes in pain perception affect the performance in the one-trial step-through paradigm. For over 30 years, studies have established that exposure to acute or chronic stressors causes pain suppression, commonly known as stress-induced analgesia (Butler and Finn Citation2009). A large number of neurotransmitters and neuropeptides are reportedly involved in stress-induced analgesia (Butler and Finn Citation2009). Furthermore, a growing literature documents the important influence of duration of stress (Parikh et al. Citation2011) and organismic factors such as age and genotype on pain sensitivity (Mogil and Belknap Citation1997; Stohr et al. Citation1998; Vendruscolo et al. Citation2004). In this study, although a significant increase in latency on the hot-plate test was not observed after tail-hanging stress, we did find that this psychological stress tended to increase the latency by around 25% in the hot-plate model when compared with control mice. The reason why stress-induced analgesia was not evident after tail-hanging stress as that of some previous reports may depend on the type of stress, its duration, and strain of animal. Any tendency for tail-hanging stress to reduce pain perception might have an effect on step-through latency, which represents learning and memory. However, we found that SCH23390 (0.05 mg/kg) or recovery for 20 min after the psychological stress did not alter pain perception in stressed mice. Moreover, the memory deficit induced by stress was rescued. Therefore, the effect of stress on the shortening of step-through latency in the RS of the one-trial step-through task is predominantly associated with learning and memory. Our results indicate that exposure to a brief stress impaired consolidation of an emotional memory, and that a brief psychological stress may disrupt the subsequent stress-related learning and memory when experienced in different contexts.
It is well known that too little or too much activation of dopamine D1 receptors is harmful for prefrontal cortex-dependent working memory (Cai and Arnsten Citation1997; Goldman-Rakic et al. Citation2004). In addition, it has been shown that stress-induced hyperactivation of dopamine D1 receptors is responsible for a working memory deficit after stress (Arnsten and Goldman-Rakic Citation1998; Arnsten et al. Citation2000; Morrow et al. Citation2000). In this study, our results indicated an inverted U shape for effects of dopamine D1 receptors on emotional learning and memory similar to that seen in prefrontal cortex. We found that SCH23390 injection-induced hypoactivation of dopamine D1 receptor impaired emotional learning and memory because the step-through latency was shortened. This effect was obviously not through alteration in pain perception since SCH23390 did not significantly alter the latencies in the hot-plate model compared to saline-treated mice. Furthermore, we demonstrated that SCH23390 did not alter the stress response of mice because stress induced a tendency to increased latency on the hot plate with or without SCH23390 treatment. Also, the mice showed similar behavioral signs of stress during the tail-hanging treatment such as “freezing” immobility, piloerection, urination, and defecation. However, the shortening of step-through latency indicated by tail-hanging stress was significantly prevented by SCH23390 administration. This indicates that hyperactivation of dopamine D1 receptors is responsible for the stress-induced deficit in emotional learning and memory, even though the stress-impaired emotional memory was not completely reversed by SCH23390. It is possible that the dose of SCH23390 was not high enough to completely block the stress-induced memory impairment. Alternatively, corticosterone might be involved because a stress-increased corticosterone level may cause spatial learning and memory impairments (de Kloet Citation2000, Citation2004). However, in this study, we found that the mice given 20 min recovery after stress still maintain a high level of serum corticosterone, but the learning and memory performance was not significantly altered compared with control mice. Hence, the results downplay the role of corticosterone in mediating emotional memory deficits after tail-hanging stress in our model. It is not known in which brain structure hyperactivation of dopamine D1 receptors is critical for the stress-induced deficit of emotional learning and memory. Future experiments using behavioral tests and local intracerebral drug administration are necessary to address these questions.
Taken together, our data indicate that hyperactivation of dopamine D1 receptors mediates an acute stress-induced deficit in emotional learning and memory. This study may have clinical significance, given that psychological stress is considered to play a role in susceptibility to some mental diseases such as depression, anxiety, and post-traumatic stress disorder.
Acknowledgments
We are grateful to Dr Richard Flannery for providing us with critical suggestions and English editorial service. We also would like to thank the reviewers and editors for giving us great comments. This work was supported by the National Basic Research Program (G1999054000) and the National Natural Sciences Foundation of China (30070251) to Dr Jingxia Cai.
Declaration of Interests: The author reports no conflicts of interest. The authors alone are responsible for the content and writing of the paper.
References
- Arnsten AF. 2007. Catecholamine and second messenger influences on prefrontal cortical networks of “representational knowledge”: A rational bridge between genetics and the symptoms of mental illness. Cereb Cortex. 17 Suppl. 1: i6–i15.
- Arnsten AF, Goldman-Rakic PS. 1998. Noise stress impairs prefrontal cortical cognitive function in monkeys: Evidence for a hyperdopaminergic mechanism. Arch Gen Psychiatry. 55:362–368.
- Arnsten AF, Murphy B, Merchant K. 2000. The selective dopamine D4 receptor antagonist, PNU-101387G, prevents stress-induced cognitive deficits in monkeys. Neuropsychopharmacology. 23:405–410.
- Butler RK, Finn DP. 2009. Stress-induced analgesia. Prog Neurobiol. 88:184–202.
- Cai JX, Arnsten AF.. 1997. Dose-dependent effects of the dopamine D1 receptor agonists A77636 or SKF81297 on spatial working memory in aged monkeys. J Pharmacol Exp Ther. 283:183–189.
- de Kloet ER. 2000. Stress in the brain. Eur J Pharmacol. 405:187–198.
- de Kloet ER. 2004. Hormones and the stressed brain. Ann N Y Acad Sci. 1018:1–15.
- Gao Y, Han H, Xu R, Cao J, Luo J, Xu L. 2008. Effects of prolonged exposure to context following contextual fear conditioning on synaptic properties in rat hippocampal slices. Neurosci Res. 61:385–389.
- Garcia R. 2001. Stress, hippocampal plasticity, and spatial learning. Synapse. 40:180–183.
- Goldman-Rakic PS, Castner SA, Svensson TH, Siever LJ, Williams GV. 2004. Targeting the dopamine D1 receptor in schizophrenia: Insights for cognitive dysfunction. Psychopharmacology (Berl). 174:3–16.
- Inglis FM, Moghaddam B. 1999. Dopaminergic innervation of the amygdala is highly responsive to stress. J Neurochem. 72:1088–1094.
- Kim JJ, Yoon KS. 1998. Stress: Metaplastic effects in the hippocampus. Trends Neurosci. 21:505–509.
- Miura H, Qiao H, Kitagami T, Ohta T, Ozaki N. 2005. Effects of fluvoxamine on levels of dopamine, serotonin, and their metabolites in the hippocampus elicited by isolation housing and novelty stress in adult rats. Int J Neurosci. 115:367–378.
- Mogil JS, Belknap JK. 1997. Sex and genotype determine the selective activation of neurochemically-distinct mechanisms of swim stress-induced analgesia. Pharmacol Biochem Behav. 56:61–66.
- Morrow BA, Roth RH, Elsworth JD. 2000. TMT, a predator odor, elevates mesoprefrontal dopamine metabolic activity and disrupts short-term working memory in the rat. Brain Res Bull. 52:519–523.
- Parikh D, Hamid A, Friedman TC, Nguyen K, Tseng A, Marquez P, Lutfy K. 2011. Stress-induced analgesia and endogenous opioid peptides: The importance of stress duration. Eur J Pharmacol. 650:563–567.
- Pezze MA, Feldon J. 2004. Mesolimbic dopaminergic pathways in fear conditioning. Prog Neurobiol. 74:301–320.
- Roozendaal B, Hahn EL, Nathan SV, de Quervain DJ, McGaugh JL. 2004. Glucocorticoid effects on memory retrieval require concurrent noradrenergic activity in the hippocampus and basolateral amygdala. J Neurosci. 24:8161–8169.
- Srikumar BN, Raju TR, Shankaranarayana Rao BS. 2007. Contrasting effects of bromocriptine on learning of a partially baited radial arm maze task in the presence and absence of restraint stress. Psychopharmacology (Berl). 193:363–374.
- Stohr T, Schulte Wermeling D, Szuran T, Pliska V, Domeney A, Welzl H, Weiner I, Feldon J. 1998. Differential effects of prenatal stress in two inbred strains of rats. Pharmacol Biochem Behav. 59:799–805.
- Vendruscolo LF, Pamplona FA, Takahashi RN. 2004. Strain and sex differences in the expression of nociceptive behavior and stress-induced analgesia in rats. Brain Res. 1030:277–283.
- Wang GW, Cai JX. 2008. Reversible disconnection of the hippocampal-prelimbic cortical circuit impairs spatial learning but not passive avoidance learning in rats. Neurobiol Learn Mem. 90:365–373.