Abstract
Previous studies in rodents showed that chronic stress induces structural and functional alterations in several brain regions, including shrinkage of the hippocampus and the prefrontal cortex, which are accompanied by cognitive and emotional disturbances. Reduced expression of the neural cell adhesion molecule (NCAM) following chronic stress has been proposed to be crucially involved in neuronal retraction and behavioral alterations. Since NCAM gene polymorphisms and altered expression of alternatively spliced NCAM isoforms have been associated with bipolar depression and schizophrenia in humans, we hypothesized that reduced expression of NCAM renders individuals more vulnerable to the deleterious effects of stress on behavior. Here, we specifically questioned whether mice in which the NCAM gene is inactivated in the forebrain by cre-recombinase under the control of the calcium–calmodulin-dependent kinase II promoter (conditional NCAM-deficient mice), display increased vulnerability to stress. We assessed the evolving of depressive-like behaviors and spatial learning and memory impairments following a subchronic stress protocol (2 weeks) that does not result in behavioral dysfunction, nor in altered NCAM expression, in wild-type mice. Indeed, while no behavioral alterations were detected in wild-type littermates after subchronic stress, conditional NCAM-deficient mice showed increased immobility in the tail suspension test and deficits in reversal spatial learning in the water maze. These findings indicate that diminished NCAM expression might be a critical vulnerability factor for the development of behavioral alterations by stress and further support a functional involvement of NCAM in stress-induced cognitive and emotional disturbances.
Introduction
In rodents, 3 to 5 weeks of chronic stress have been shown to induce cognitive deficits in a variety of learning and memory task (Luine et al. Citation1994; Conrad et al. Citation1996; Park et al. Citation2001; Venero et al. Citation2002; Sandi et al. Citation2003; Li et al. Citation2006; Conrad Citation2010). These defects were mostly accompanied by morphological alterations in the hippocampus, such as shortening and debranching of apical dendrites as well as alterations of presynaptic mossy fiber terminals in CA3 pyramidal neurons (Woolley et al. Citation1990; Watanabe et al. Citation1992; Magarinos et al. Citation1997; Conrad et al. Citation1999; McEwen Citation1999, Citation2005; Sousa et al. Citation2000; McEwen and Lasley Citation2003). In addition, chronic stress was also found to induce atrophy of distal dendritic branches and a loss of dendritic spines in layers II and III pyramidal neurons of the prefrontal cortex (Wellman Citation2001; Brown et al. Citation2005; Radley et al. Citation2005; Cerqueira et al. Citation2007; Holmes and Wellman Citation2009). Similar chronic stress protocols, that induced these cognitive and structural alterations, were also shown to reduce the expression of the neural cell adhesion molecule (NCAM), particularly in the hippocampus but also in other forebrain areas (Sandi et al. Citation2001; Touyarot and Sandi Citation2002; Venero et al. Citation2002; Alfonso et al. Citation2006; Sandi and Touyarot Citation2006; Shin et al. Citation2009; Bisaz et al. Citation2011). Given the key role of NCAM in the formation and stabilization of neuronal networks during development and activity-dependent synaptic rearrangements in adulthood (Kiss and Muller Citation2001; Rougon and Hobert Citation2003; Kleene and Schachner Citation2004; Walmod et al. Citation2004; Maness and Schachner Citation2007; Conboy et al. Citation2010; Muller et al. Citation2010), stress-induced NCAM reduction has been hypothesized to critically contribute to chronic stress-induced cognitive and neuronal disturbances (Sandi Citation2004; Sandi and Bisaz Citation2007). It is important to note, however, that opposite changes have been found in the hippocampus and the amygdala for both dendritic remodeling (Vyas et al. Citation2002) and NCAM expression (Bisaz and Sandi Citation2010) following exposure to chronic stress.
The human chromosome 11q, which contains the NCAM1 gene (Nguyen et al. Citation1986), was reported to be a susceptibility locus for various neuropsychiatric disorders, including bipolar disorders and schizophrenia (Lewis et al. Citation2003; Segurado et al. Citation2003; Farmer et al. Citation2007; Sullivan et al. Citation2007). More specifically, altered expression of differently spliced NCAM1 transcripts, including the secreted NCAM isoform and the variable alternative spliced exon (VASE)containing NCAM isoforms, has been associated with both schizophrenia and bipolar disorders (Barbeau et al. Citation1995; Poltorak et al. Citation1995; Vawter et al. Citation1998; Vawter et al. Citation1999; Vawter et al. Citation2001). More recently, several single nucleotide polymorphisms (SNPs) in the NCAM1 gene have been identified as potential risk factors for the development of bipolar disorders and schizophrenia in Japanese (Arai et al. Citation2004) and North American (Atz et al. Citation2007) subpopulations.
Altogether these studies led us to hypothesize that reduced expression of NCAM might enhance vulnerability to develop stress-induced behavioral alterations. To test this hypothesis, we performed a ‘gene × environment interaction’ study to evaluate whether mice with a conditional ablation of the NCAM gene in the forebrain (Bukalo et al. Citation2004) would display cognitive and emotional alterations after a subchronic stress procedure of a duration (i.e. 2 weeks) that has been shown not to induce substantial cognitive impairments and depressive-like behaviors in adult male wild-type mice (Ducottet and Belzung Citation2005; Strekalova et al. Citation2005; Advani et al. Citation2009; Kazlauckas et al. Citation2011). To this end, we exposed conditional NCAM-deficient mice and their wild-type littermates to 14 days of subchronic unpredictable stress and evaluated the evolving of depressive-like behaviors in a tail suspension test (TST), as well as spatial and reversal learning in the Morris water maze. We found that following subchronic stress, in contrast to wild-type littermates that do not display cognitive or emotional alterations, conditional NCAM-deficient mice display increased immobility in the TST, as well as impaired reversal spatial learning in the water maze. Our results support the view that reduced NCAM expression in the forebrain might be a critical vulnerability factor for the development of stress-induced cognitive and emotional disturbances.
Materials and methods
Animals
All experiments involving conditional NCAM-deficient mice were conducted on in-house bred age-matched (5–9 months old) male conditional NCAM-deficient mice and their wild-type male littermates (NCAMff). The generation of the conditional NCAM-deficient mice has been described previously (Bukalo et al. Citation2004). Briefly, homozygous NCAM-floxed female mice were bred with homozygous NCAM-floxed male mice that express cre-recombinase under the control of the promoter of the α-subunit of the calcium–calmodulin-dependent protein kinase II (αCaMKII). The progeny were homozygous for the NCAM-floxed alleles, half of them carried the αCaMKII-cre transgene (NCAMffcre) and the rest were wild-type littermates (NCAMff). All mice were backcrossed for more than 10 generations into the C57BL/6J background and were housed in groups of two to four in standard plastic cages on a 12 h light/dark cycle (lights on at 07:30 h). Temperature in the animal housing room was maintained at 21 ± 1°C.
Experiments on C57BL/6J wild-type mice were conducted on 4-month-old C57BL/6J wild-type male mice obtained from Charles River Laboratory (L'Arbresle Cedex, France). Mice were habituated to our animal facility for 18 days before beginning the experiments. Throughout the experimental period (besides the daily behavioral manipulations) mice had ad libitum access to water and food. All procedures were performed in accordance with the Swiss National Institutional Guidelines on Animal Experimentation, and they were approved by the Swiss Cantonal Committee for Animal Experimentation. The number of mice used in the present study was kept to a minimum, as was animal suffering in all procedures.
Subchronic unpredictable stress
NCAMffcre mice and NCAMff littermates were either left undisturbed (N = 5–6/group) or exposed to 14 days of subchronic unpredictable stress (N = 6/group). The subchronic stress protocol consisted of exposing mice daily at an unpredictable time (between 08:00 and 16:00 h) to one of the following stress stimuli: 6 min tail suspension (only on days 1 and 14); 3 × 0.4 mA inescapable footshocks; 4 h exposure to soiled, damp sawdust; 2 h exposure on an elevated platform; 1 h immobilization in a plastic tube, 6.5 cm (L) × 4 cm (ID); 30 min exposure to 16°C; 2 days reversed light/dark cycle; 10 min exposure to an older, aggressive conspecific; 1 h exposure to trimethylthiazoline; and 8 h with a 40°cage inclination. All mice were weighed and the state of their coat was evaluated every 3–5 days.
Tail suspension test
The emergence of depressive-like behaviors of subchronically stressed NCAMffcre and NCAMff mice was assessed in two TSTs, applied on the first (day 1) and on the last day (day 14) of the subchronic stress protocol. Additionally, we also tested 10 C57BL/6J adult wild-type male mice in two TSTs, which were performed with a testing interval of 2 weeks. The TST is an analog of the forced swim test and is based on the finding that mice suspended by the tail alternately struggle and hang immobile. Briefly, mice were individually suspended by their tail (attached 2 cm from the tail tip) from a horizontal metal bar fixed approximately 40 cm above the floor. The duration of immobility (defined as the lack of movements beside respiration for periods lasting longer than 1 s) was measured during a 6 min testing period. Two mice (one from each stress group) managed to climb up their trail during the testing period and were excluded from the analysis.
Spatial and reversal learning in the Morris water maze
One day after the last stressors had been applied, we examined the impact of 14 days of subchronic unpredictable stress in NCAMffcre and NCAMff mice on spatial and reversal learning, as well as on long-term spatial memory in the Morris water maze. The water maze experiments were conducted in a white circular pool (140 cm diameter) filled with opaque colored water (26 ± 1°C) and a hidden platform (10 × 10 cm) submerged approximately 1.3 cm under the water surface, as previously described (Bisaz et al. Citation2011). Briefly, one day before training, all mice were habituated to the room, apparatus, and water by giving them a 2 min free swim trial with no platform present, for later assessment of average swim speed. Spatial learning of stressed and control mice of both genotypes was assessed over 12 trials on the 3 following days (four trials/day), during which the escape platform remained in the same quadrant. In this spatial learning task (days 1–3), trials were collapsed into blocks of two consecutive trials for the data analyses. On day 4, a 60 s probe test was carried out, during which the platform was removed from the pool. The percentage of time mice swam in the target, opposite, and adjacent quadrants was measured and served as an indication of a long-term spatial memory. Cognitive flexibility was further assessed during 3 days of reversal learning [i.e. days 4 (after probe test), 5 and 6], in which the platform position was kept constant during the four trials of each day but varied on each successive day, such that the platform locations in each of the three remaining quadrants were used (Kesner Citation2000; Cerqueira et al. Citation2007). For the analysis, individual trials from each training day (i.e. days 4–6) were collapsed into first, second, third, and fourth trials, as previously described (Cerqueira et al. Citation2005, Citation2007). In order to minimize possible confounds caused by motivational, motor, and sensory effects, the spatial and reversal learning performance of the mice in the water maze was analyzed in terms of the distance swum to find the submerged platform.
Quantitative immunoblotting of NCAM
Expression levels of three main NCAM isoforms (NCAM-180, NCAM-140, and NCAM-120) were measured in immunoblots of crude synaptosomal preparations of hippocampal and medial prefrontal cortex (mPFC) fractions of subchronically stressed and control adult male C57BL/6J wild-type mice, as previously described (Bisaz and Sandi Citation2010; Bisaz et al. Citation2011). Briefly, synaptosomes from each mouse were incubated overnight at room temperature with endoneuraminidase-N (AbCys, Paris, France; final dilution 1:120) to selectively cleave the polysialic acid moiety of NCAM. The reaction was stopped by boiling samples at 100°C for 5 min in 70 mM Tris–HCl (pH 6.8), 33 mM NaCl, 1 mM ethylenediaminetetraacetic acid, 2% sodium dodecyl sulfate (SDS), 0.01% bromophenol blue, 10% glycerol, and 3% dithiothreitol. About 5 μg of total protein from each sample was separated on 7.5% SDS-polyacrylamide gel electrophoresis and transferred to a nitrocellulose membrane (Protran, Whatman GmbH, Dassel, Germany). After saturation of nonspecific sites with 5% nonfat dry milk in 10 mM Tris–HCl (pH 7.4), containing 150 mM NaCl, 0.05% Tween-20 (TBST), the membranes were probed overnight at 4°C with primary antibodies against NCAM (1:5000; Millipore, Temecula, CA, USA) or actin (1:20,000; Sigma, St. Louis, MO, USA), washed with TBST, incubated for 2 h with the appropriate secondary horseradish peroxidase-linked antibodies (NCAM, 1:1000 goat anti-rabbit IgG, Molecular Probes, Basel, Switzerland; Actin, 1:10,000 goat anti-mouse IgG, Calbiochem, Basel, Switzerland), and finally developed using the SuperSignal West Dura Substrate (Pierce, Rockford, IL, USA). Bands were detected using the ChemiDoc XRS system (Bio-Rad, Hercules, CA, USA) and densitometry analysis on bands was calculated using Bio-Rad Quantity One 4.2.3 software (Bio-Rad Laboratories AG, Switzerland). Following normalization to within-lane actin (not shown), the expression of all three main NCAM isoforms was expressed as the percentage of the unstressed, control mice. The linear range of specific antibody signal detection was determined at the outset of these experiments, and all experimental samples were loaded at a concentration within the linear range of the antibody signal detection.
Data analysis
All the results are expressed as the mean ± standard error of the mean (SEM) and were analyzed with the StatView version 5.0 software package (SAS Institute, Cray, NC, USA). The data were analyzed with paired and unpaired Student's t-tests or by analysis of variance (ANOVA) with or without repeated measures, as appropriate. Post hoc tests [Fisher's Protected least significant difference (PLSD)] were applied whenever ANOVA yielded significant interactions. In the water maze experiments, in addition to analyzing global effects of stress and genotype over the whole learning and reversal learning procedure (with repeated measures ANOVA), we also analyzed stress effects for each genotype, as well as genotype effects in control and stressed groups separately. Moreover, since considerable data in the literature led us to set the a priori hypothesis that stress and genotype effects might manifest as sporadic impairments on individual trial blocks (Venero et al. Citation2002; Sandi et al. Citation2003; Bukalo et al. Citation2004; Cambon et al. Citation2004; Touyarot et al. Citation2004; Wright and Conrad Citation2008; Bisaz et al. Citation2011), unpaired Student's t-tests were also applied to individual trial blocks in the water maze experiments. The significance of the results was accepted at p ≤ 0.05.
Results
Stress-induced body weight change
Body weight changes were assessed in stressed and control mice of both genotypes by measuring individual body weights before (NCAMff control: 35.2 ± 1.1 g, stressed: 36.6 ± 1.5 g; NCAMffcre control: 32.5 ± 1.2 g, stressed: 38.3 ± 2.7 g) and 1 day after subchronic stress exposure (NCAMff control: 35.3 ± 1.2 g, stressed: 34.0 ± 1.1 g; NCAMffcre control: 32.4 ± 1.2 g, stressed: 35.8 ± 2.4 g). One-way ANOVAs on percentage body weight change (from pre-stress values) revealed a significant effect for the “stress” factor ; F(1,19) = 69.778, p < 0.0001]. Fisher PLSD post hoc tests were significant between control and stressed mice of both genotypes (NCAMff: p < 0.0001; NCAMffcre: p < 0.0001), indicating a significant body weight reduction of both NCAMff and NCAMffcre mice following 2 weeks of subchronic stress. Additionally, ANOVAs also revealed a lack of significance for the “genotype” factor [F(1,19) = 0.001, n.s.] and in the “stress × genotype” interaction [F(1,19) = 0.359, n.s.], indicating the control and stressed mice of both genotypes showed comparable body weight change following 2 weeks of stress exposure.
Figure 1. Percentage body weight change of control and subchronically stressed conditional NCAM-deficient mice (NCAMffcre) and their NCAMff littermates. Both stressed NCAMffcre and NCAMff mice displayed a significant reduction in percentage body weight when compared with control, non-stressed mice of the same genotype. Results are mean ± SEM (N = 5–6/group; **p < 0.01, unpaired Student's t-test versus controls of the same genotype).



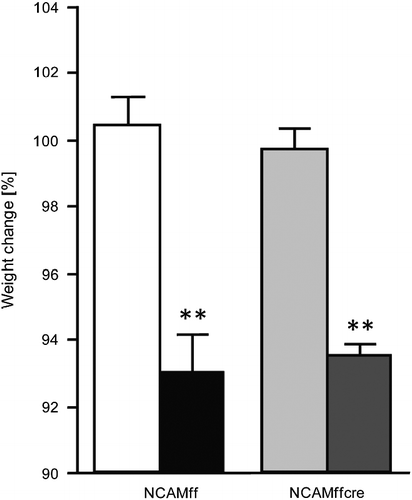
Effect of subchronic stress on depressive-like behaviors
The evolving of depressive-like behaviors was assessed in two TSTs, applied on the first (day 1) and on the last day (day 14) of the subchronic stress protocol (). Paired Student's t-test for time spent immobile revealed no significant “day” effect for NCAMff mice (t4 = 0.719, n.s.), but a significant change for NCAMffcre mice (t4 = − 2.614, p = 0.05), denoting their longer immobility time on day 14 than on day 1 (). Comparing immobility time between mice of both genotypes on the individual testing days, unpaired Student's t-test indicated no significant “genotype” effect on day 1 (t8 = 0.013, n.s.) but a significant longer immobility time of NCAMffcre mice on day 14, when compared to NCAMff mice (; t8 = 3.887, p = 0.005). For comparison, we also exposed undisturbed C57BL/6J wild-type mice to two TSTs with a testing interval of 14 days. As shown in , paired Student's t-test for time spent immobile revealed no significant “day” effect for these mice (t9 = − 0.086, n.s.).
Figure 2. Immobility time of subchronically stressed conditional NCAM-deficient mice (NCAMffcre) and their NCAMff littermates in two TSTs performed on the first (day 1) and last day (day 14) of the subchronic stress procedure. No difference in the time mice spent immobile was found between NCAMffcre and NCAMff mice on the TST performed on day 1 of the stress procedure. However, stressed NCAMffcre mice displayed a significantly longer immobility time during the TST on day 14 of the stress procedure when compared with NCAMff littermates. Additionally, although NCAMff littermates showed similar immobility times in both TST tests, subchronically stressed NCAMffcre mice displayed a significant increase in their immobility time on the TST on day 14, when compared with the TST on day 1. Non-stressed wild-type mice showed similar immobility times when tested in two TSTs occurring with a testing interval of 2 weeks. Results are mean ± SEM (N = 5–10/group; **p < 0.01, unpaired Student's t-test versus NCAMff of the same day; #p < 0.05, paired Student's t-test versus day 1 of the same genotype).


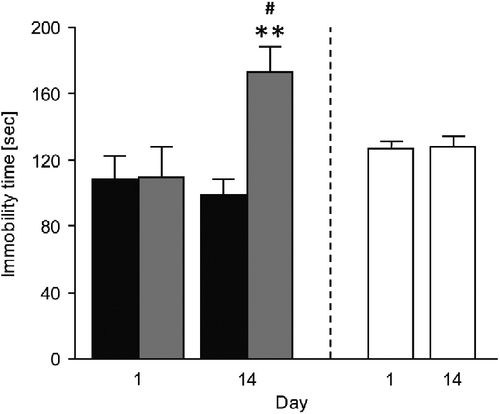
These results indicate that only stressed NCAMffcre mice showed signs of emergence of depressive-like behaviors, as indicated by their increased immobility time in the TST from the first to the second exposure occurring 2 weeks afterwards, whereas stressed NCAMff and unstressed wild-type mice did not show these behavioral alterations.
Effect of subchronic stress on spatial and reversal learning
One day prior to the water maze experiments (i.e. 1 day after the cessation of the subchronic stress procedure), mice were given a 2 min free swim trial to habituate them to the water and testing procedures as well as to measure average swim speeds (all measures were n.s. difference between the groups; data not shown). Since in most cases repeated measures ANOVAs yielded significance for the distance swum across blocks of trials, indicating that overall the mice progressively improved their performance to find the platform, the specific statistical details are not included for the sake of clarity, except when a lack of significance was found.
We first analyzed global effects of stress and genotype on spatial learning (day 1–3) in the water maze. Repeated measures ANOVAs on the distance swum to find the platform revealed no significant effect neither for the “stress” [F(1,19) = 1.751, n.s.] and “genotype” [F(1,19) = 0.139, n.s.] factors nor for their interaction [F(1,19) = 0.027, n.s.]. ANOVAs further indicated no significant effects for the paired interactions between these factors (all n.s.). However, they revealed significant effects for the “trial block × stress × genotype” interaction [F(5,95) = 2.647, p = 0.028].
We then addressed the question of whether conditional NCAM-deficient (NCAMffcre) mice have higher vulnerability to subchronic stress than their NCAMff littermates, and therefore stress effects on spatial learning were first analyzed separately for each genotype (). The respective repeated measures ANOVAs for the average distance mice had to swim to find the hidden platform indicated a lack of significance for the “stress” factor in both genotypes [NCAMff: F(1,9) = 0.897, n.s.; NCAMffcre: F(1,10) = 0.841, n.s.]. However, since substantial data in the literature indicate that chronic stress leads to mild learning impairments in the water maze that only manifest in certain training trials (Venero et al. Citation2002; Sandi et al. Citation2003; Touyarot et al. Citation2004; Stewart et al. Citation2005; Wright and Conrad Citation2008; Bisaz et al. Citation2011), we also performed unpaired Student's t-tests for each block of trials to explore this possible effect. Although no significant stress effect was found for any of the trial blocks in NCAMff mice (; for all p ≥ 0.19), stressed NCAMffcre mice displayed significantly longer swim distance to find the platform on trial block 3 (t10 = − 2.436, p = 0.035) and on trial block 5 (t10 = − 3.033, p = 0.013) than their unstressed controls ().
Figure 3. Spatial and reversal learning and long-term spatial memory of control and subchronically stressed conditional NCAM-deficient mice (NCAMffcre) and NCAMff littermates in the Morris water maze. (A) No significant difference was found between stressed and control NCAMff mice during the 3 days of spatial learning. (B) During the probe test on day 4, both, stressed and control, NCAMff mice spent significantly more time in the target/trained quadrant than in the opposite and adjacent quadrants. (C) No significant differences were found in reversal learning between stressed and control NCAMff mice. (D) Stressed NCAMffcre mice swam a significantly longer distance to find the hidden platform on trial blocks 3 (B3) and 5 (B5) when compared to control NCAMffcre mice. (E) Although control NCAMffcre mice displayed a significant quadrant preference and spent significantly more time in the target than in the opposite and adjacent quadrants, stressed NCAMffcre mice failed to show significant quadrant preference. (F) During the 3 days of reversal learning, stressed NCAMffcre mice displayed overall a significantly longer distance swum when compared to control NCAMffcre mice. Additionally, stressed NCAMffcre mice swam a significantly longer distance to find the hidden platform on the second and fourth reversal learning trials when compared to control mice of the same genotype. Results are mean ± SEM (N = 5–6/group; *p ≤ 0.05, **p ≤ 0.01, unpaired Student's t-test versus control mice of the same genotype; +p ≤ 0.05, the Fisher PLSD post hoc analysis versus all other quadrants of the same condition and genotype; #p < 0.05, repeated measures ANOVA versus control mice of the same genotype). ○ NCAMff control, • NCAMff stressed,

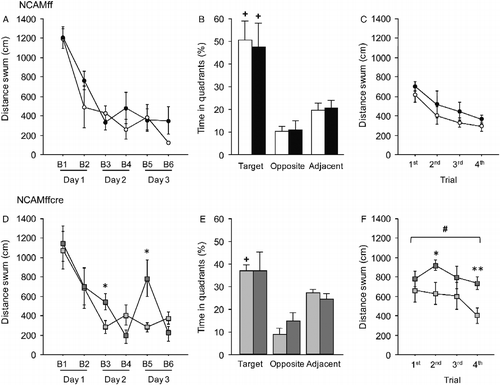
In addition to the analysis of stress effects within each genotype, we also analyzed the genotype effects on spatial learning separately for control and stressed mice. For control mice, repeated measures ANOVAs revealed a lack of significance for the “genotype” factor [F(1,10) = 0.189, n.s.] and the “genotype × trial block” interactions [F(5,50) = 0.947, n.s.]. However, control NCAMffcre mice swam significantly longer distances to find the submerged platform on trial block 6 (t10 = 3.234, p = 0.005) than control NCAMff mice. For stressed mice, repeated measures ANOVAs indicated significance for the “genotype × trial block” interaction [F(5,45) = 2.468, p = 0.046], but not for the “genotype” factor [F(1,9) = 0.017, n.s.]. Additionally, stressed NCAMffcre mice swam significantly longer distances on trial blocks 3 (t9 = 1.849, p = 0.049) and 5 (t9 = 1.997, p = 0.039) than stressed NCAMff mice.
Long-term spatial memory was tested on day 4, by submitting all mice to a 60 s probe test in which the platform had been removed from the pool. ANOVAs for each experimental group revealed a significant higher percentage of time spent in target than in the opposite and adjacent quadrants [NCAMff control: F(2,12) = 15.167, p = 0.0005; NCAMff stressed: F(2,15) = 7.993, p = 0.004; NCAMffcre control: F(2,15) = 33.395, p < 0.0001; NCAMffcre stressed: F(2,15) = 3.916, p = 0.05]. Interestingly, Fisher PLSD post hoc analyses for percentage of time spent swimming in the target versus opposite and adjacent quadrants were significant (p ≤ 0.01) for control and stressed NCAMff mice as well as for control NCAMffcre mice (), they revealed a lack of significant quadrant preference for stressed NCAMffcre mice (; p = 0.14).
Unpaired Student's t-tests revealed no significance in the percentage of time mice spent in swimming in the target quadrant between control and stressed mice of both genotypes (; NCAMff: t9 = 0.216, n.s.; NCAMffcre: t10 = 0.006, n.s.). Control NCAMffcre mice spent a significantly lower percentage of time in the target quadrant than control NCAMff mice (t10 = − 1.759, p = 0.05).
Cognitive flexibility was assessed during 3 days of reversal learning (days 4–6), in which the platform position was kept constant during the four trials of each day but varied on each successive day. Repeated measures ANOVAs revealed a significant effect for the “stress” [F(1,19) = 10.337, p = 0.005] and “genotype” [F(1,19) = 20.442, p = 0.0003] factors. Subsequent ANOVAs indicated a lack of significance for paired interactions between each of these factors (all n.s.).
Analysis of stress effects in NCAMff mice with repeated measures ANOVAs revealed indicated a lack of effect for either the “stress” factor [F(1,9) = 2.205, n.s.] or the “trial × stress” interaction [F(3,27) = 0.034, n.s.], indicating, as expected, no significant difference between control and stressed NCAMff mice in the swim distance to find the hidden platform during the reversal learning sessions (). Unpaired Student's t-tests performed on data from individual trials revealed no significant effect of stress for NCAMff mice (for all p>0.21). Importantly, repeated measures ANOVAs for data from NCAMffcre mice revealed a lack of significance for the “trial” factor [F(3,30) = 1.776, n.s.] and for the “trial × stress” interaction [F(3,30) = 0.566, n.s.], indicating that control and stressed NCAMffcre mice failed to decrease the distance needed to swim to find the hidden platform over the four consecutive trials during the reversal learning sessions (). Overall stressed NCAMffcre mice had to swim a significantly longer distance to find the hidden platform during the reversal learning sessions than control NCAMffcre mice ; F(1,10) = 8.643, p = 0.016]. Moreover, when individual trials were analyzed separately, stressed NCAMffcre mice displayed significant longer swim distance on the second (t10 = − 2.434, p = 0.038) and fourth (t10 = − 3.325, p = 0.009) trials of the reversal learning sessions.
When data were analyzed separately for control and stress conditions, repeated measures ANOVAs on data from control mice revealed a significant effect for the “genotype” factor [F(1,9) = 6.918, p = 0.03] but not for the “trial × genotype” interaction [F(3,27) = 0.57, n.s.]. Unpaired Student's t-tests for individual trials further revealed significant differences between genotypes in the second (t9 = 1.934, p = 0.043) and third trials (t9 = 2.241, p = 0.026), with control NCAMffcre mice showing a significant longer swim distance in these two trials than control NCAMff mice. Moreover, repeated measures ANOVAs on data from stressed mice revealed a significant effect for the “genotype” factor [F(1,10) = 14.944, p = 0.003], but a lack of significance for the “genotype × trial” interaction [F(3,30) = 2.019, n.s.]. Stressed NCAMffcre mice swam a significantly longer distance to find the hidden platform on the second (t10 = 2.724, p = 0.021), third (t10 = 2.371, p = 0.04), and fourth (t10 = 4.845, p = 0.0007) trials than stressed NCAMff mice. Thus, the reduced cognitive flexibility of control NCAMffcre mice became even more pronounced when these mice were exposed to 2 weeks of subchronic stress.
Taken together, these data indicate that NCAMffcre mice exposed to subchronic stress show mild impairments in spatial learning and long-term spatial memory, as well as a marked impairment in reversal learning abilities. In contrast, NCAMff littermates remained largely unaffected in these cognitive abilities by the same stress procedure.
Effect of subchronic stress on NCAM expression in the hippocampus and mPFC
We have previously shown that C57BL/6J wild-type mice chronically stressed over 4 weeks present a decrease of NCAM-180 and NCAM-140 expression in the hippocampus, while NCAM expression levels in the mPFC were not significantly altered. These changes were accompanied by stress-induced mild impairment in spatial learning and marked reversal learning deficits (Bisaz et al. Citation2011). The question arises here as to what extent the shorter stress protocol performed in the current study, that does not affect wild-type mice on these cognitive capabilities, would affect NCAM expression levels in these brain regions. Thus, we examined NCAM expression levels in the hippocampus and mPFC in adult male C57BL/6J wild-type mice after exposure to the 14-day subchronic stress protocol. As shown in , repeated measures ANOVAs for the three major NCAM isoforms in hippocampal fractions revealed no significant effect for either the “stress” factor [F(1,8) = 2.707, n.s.], the “isoform” factor [F(2,16) = 0.366, n.s.], or in the “stress × isoform” interaction [F(2,16) = 0.366, n.s.]. Additionally, unpaired Student's t-tests for each of the NCAM isoforms individually revealed no significant effects of stress (p ≥ 0.2). Similarly, in mPFC fractions (), repeated measures ANOVAs revealed no significant effect of the “stress” factor [F(1,8) = 0.101, n.s.], the “isoform” factor [F(1,8) = 0.292, n.s.], and the “stress × isoform” interaction [F(2,16) = 0.292, n.s.], and unpaired Student's t-tests were p ≥ 0.3 for each of the NCAM isoforms. Altogether, these results indicate that NCAM expression levels in the hippocampus and the mPFC remained unaltered in C57BL/6J wild-type mice exposed to 2 weeks of subchronic stress.
Figure 4. NCAM expression in the hippocampus and the mPFC of control and subchronically stressed C57BL6/J wild-type mice. (A) No significant differences in NCAM expression levels were found in the hippocampus between stressed and control C57BL6/J wild-type mice. (B) Both control and stressed C57BL6/J wild-type mice displayed similar NCAM expression levels in the mPFC. Results are mean ± SEM (N = 5/group). ▪ Wild-type control and ▪ wild-type stressed.
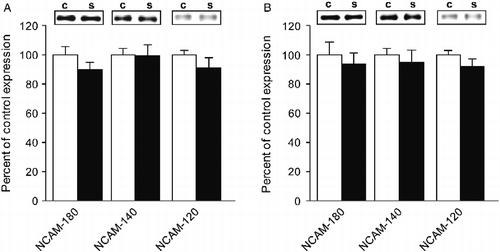
Discussion
Previous studies have shown that 3–4 weeks of chronic stress exposure leads to reduced expression levels of NCAM in the hippocampus and other forebrain areas (Sandi et al. Citation2001; Touyarot and Sandi Citation2002; Venero et al. Citation2002; Touyarot et al. Citation2004; Alfonso et al. Citation2006; Shin et al. Citation2009; Bisaz et al. Citation2011), which were proposed to be involved in the associated structural shrinkage, reduced synaptic features, and behavioral alterations (Sandi Citation2004; Sandi and Bisaz Citation2007; Bisaz et al. Citation2011). Using conditional NCAM-deficient (NCAMffcre) mice in which the NCAM gene is ablated in glutamatergic neurons of the forebrain, we addressed here whether NCAM deficiency alters vulnerability for stress-induced behavioral alterations. Our results showed that NCAMffcre mice displayed signs of depressive-like behaviors, mild impairments in spatial learning and memory, as well as profound impairments in reversal learning following 2 weeks of subchronic stress exposure, while their wild-type littermates (NCAMff) remained largely unaffected by the same stress procedure. We also showed that 2 weeks of subchronic stress exposure did not induce changes in NCAM expression in the hippocampus and the mPFC of wild-type mice, indicating that NCAM alterations within these brain regions may need to occur to effectuate stress-induced cognitive and emotional disturbances.
To our knowledge, this is the first study showing that reduced NCAM expression levels in the forebrain are associated with a higher vulnerability to show behavioral alterations in response to stress in tests widely used in the rodent to assess depressive-like symptoms. These results are in line with evidence from both clinical and experimental research showing that altered NCAM expression might be associated with the occurrence of depressive disorders (Jorgensen Citation1988; Poltorak et al. Citation1996; Vawter et al. Citation1998, Citation1999; Vawter Citation2000; Sandi and Bisaz Citation2007). For instance, the human NCAM1 gene has been postulated as a candidate susceptibility gene for bipolar and major depression disorders (Sandi and Bisaz Citation2007; Brennaman and Maness Citation2010) and SNPs from different NCAM1 regions were associated with a risk for bipolar disorders in Japanese and North American subpopulations (Arai et al. Citation2004; Atz et al. Citation2007). Additional circumstantial evidence for the involvement of altered NCAM expression in depressive-like symptoms has been provided by animal studies. Chronic stress protocols that induce depressive-like behaviors (typically 3–5 weeks; Pothion et al. Citation2004; Dalla et al. Citation2005; Rygula et al. Citation2005; Banasr and Duman Citation2008) were also found to induce considerable reductions of NCAM expression in the hippocampus and other forebrain areas (Sandi et al. Citation2001; Touyarot and Sandi Citation2002; Venero et al. Citation2002; Touyarot et al. Citation2004; Alfonso et al. Citation2006; Bisaz et al. Citation2011). Strikingly, constitutional NCAM knockout (NCAM KO) mice were reported to show depressive-like phenotypes in different behavioral tests indicative of depressive-like behaviors, such as the forced swim test (Stork et al. Citation2000), the sucrose preference test, and the TST (Aonurm-Helm et al. Citation2008). Interestingly, systemic injection of the NCAM mimetic peptide FG loop (a synthetic peptide that mimics the NCAM binding site for the fibroblast growth factor receptors 1 and 2) was able to reverse depressive-like behaviors of NCAM KO mice in both the tail suspension and the sucrose preference tests (Aonurm-Helm et al. Citation2008). Altogether these data indicate that NCAM alterations might be critically involved in the development and exacerbation of depressive-like behaviors.
It is important to note that, contrary to constitutional NCAM KO mice that show depressive-like symptoms under basal conditions (Aonurm-Helm et al. Citation2008), NCAMffcre mice used in the present study show no signs of depressive-like behaviors in a TST under basal, non-stressed conditions, but clear indications for depressive-like behaviors following a 2-week subchronic stress exposure. There are considerable differences between both types of transgenic mice that need to be considered. First, contrary to NCAM KO mice, NCAMffcre mice have normal NCAM expression levels in the forebrain during the early postnatal period, but gradually decrease from the second postnatal week, resulting in approximately 10–20% of normal NCAM expression levels in the hippocampus, amygdala, and mPFC during adulthood (Bukalo et al. Citation2004; Bisaz and Sandi Citation2010; Bisaz et al. Citation2011). Second, unlike NCAM KO mice, which show reduced brain weight and size of the olfactory bulb (Cremer et al. Citation1994), reduced mossy fiber density, and reduced occurrence of mossy fiber terminals on CA3 pyramidal neurons (Tomasiewicz et al. Citation1993; Cremer et al. Citation1997), no morphological abnormalities were found at the light microscopic level in the NCAMffcre mice (Bukalo et al. Citation2004). Third, although NCAM KO mice show increased stimulated corticosterone levels (Stork et al. Citation1997), conditional NCAM-deficient mice have normal basal and stimulated corticosterone levels (Bisaz et al. Citation2011). Finally, although NCAM KO mice have been reported to show increased anxiety-related behaviors (Cremer et al. Citation1994; Stork et al. Citation1997; Stork et al. Citation1999), which are increasingly recognized as an important risk factor for the development of depressive disorders (Sandi and Richter-Levin Citation2009), we have previously shown that NCAMffcre mice display reduced basal anxiety levels in the elevated plus maze and the dark/light avoidance test compared with their NCAMff littermates (Bisaz and Sandi Citation2010; Bisaz et al. Citation2011). Therefore, developmental alterations, differences in stress responsiveness, or abnormal anxiety traits appear not to account for the increased vulnerability to stress-induced depressive-like symptoms reported in the present study.
We also report, here, that exposure to 2-week subchronic stress in NCAMff mice did not affect their performance in spatial and reversal learning and long-term spatial memory. These results are in accordance with the literature, showing that 3–5 weeks of chronic stress exposure is required for cognitive deficits in spatial and reversal learning to occur (Conrad et al. Citation1996; Park et al. Citation2001; Venero et al. Citation2002; Sandi et al. Citation2003; Bisaz et al. Citation2011), while shorter stress periods (i.e. 1–2 weeks) are found to induce either facilitating (Luine et al. Citation1996; Gouirand and Matuszewich Citation2005), impairing (Radecki et al. Citation2005), or no effects on spatial learning and memory (Craig et al. Citation2008; Mello et al. Citation2008). Interestingly, stress-induced cognitive impairments in spatial learning and memory tasks coincided with the structural alterations occurring in different brain areas, including the hippocampus, amygdala, and prefrontal cortex. While chronic stress procedures (typically 3–5 weeks) were shown to negatively alter the structure and function of neurons in the hippocampus (Woolley et al. Citation1990; Watanabe et al. Citation1992; McEwen Citation2000, Citation2003; Fuchs and Flugge Citation2001) and prefrontal cortex (Wellman Citation2001; Cook and Wellman Citation2004; Radley et al. Citation2006; Cerqueira et al. Citation2007; Dias-Ferreira et al. Citation2009), 10 days of subchronic stress was shown to induce none or only slight changes in the dendritic morphology of CA3 neurons (Luine et al. Citation1996; Vyas et al. Citation2002).
NCAMffcre mice responded to subchronic stress with mild spatial learning impairments, which was substantiated only by statistical tests performed on individual blocks of learning trials, but with marked reversal learning impairment in the Morris water maze. The reported reversal learning deficits of subchronically stressed NCAMffcre mice were found in a behavioral task that has been shown to be critically dependent on the integration of both hippocampal and prefrontal cortex functions (Kesner Citation2000). A recent study has shown that similar chronic unpredictable stress procedures over 4 weeks induced structural alterations in the hippocampus and the prefrontal cortex (PFC) as well as reduced LTP induction in hippocampal-to-PFC connections (Cerqueira et al. Citation2007). Given the critical role of NCAM in the formation, stabilization, and plasticity of synaptic contacts (Hartz and Ronn Citation2010; Muller et al. Citation2010), reduced forebrain NCAM expression in NCAMffcre mice (Bukalo et al. Citation2004; Bisaz et al. Citation2011) may result in faster stress-induced destabilization of relevant neuronal circuits in the hippocampus and the hippocampal–prefrontal cortex pathways, rendering mice more vulnerable to develop cognitive impairments when exposed to brief stress periods. Notably, we previously found that 4 weeks of exposure to unpredictable stress does not affect NCAM expression levels in the hippocampus of these mice (Bisaz and Sandi Citation2010).
In conclusion, using a “gene × environment” interaction experimental design, our results indicate that decreased NCAM expression in the forebrain enhances vulnerability to develop stress-induced cognitive and emotional disturbances. NCAM deficits might result in an inability of relevant neuronal networks to respond and adapt appropriately to novel, stressful environments. Although further work is needed to elucidate the role of NCAM in molecular and cellular mechanisms leading to stress-induced behavioral alterations, our findings further support NCAM as being critically involved in the negative effects stress exerts on brain function and cognition.
Acknowledgments
We thank Prof. Melitta Schachner for the initial donation of conditional NCAM-deficient mice breeding pairs, and Coralie Siegmund for help with NCAM immunoblots. This work has been supported by grants from the EU (FP6-2003-LIFESCIHEALTH-II-512012, PROMEMORIA; and FP7-HEALTH-F2M-2008-201600, MemStick), the Swiss National Science Foundation (3100A0-108102 and 310000-120791; Sinergia CRSIK0-122697 and CRSIK0-122691), and intramural funding from the EPFL.
Declaration of interest: The authors report no conflicts of interest. The authors alone are responsible for the content and writing of the paper.
References
- Advani T, Koek W, Hensler JG. 2009. Gender differences in the enhanced vulnerability of BDNF+/ − mice to mild stress. Int J Neuropsychopharmacol. 12:583–588.
- Alfonso J, Frick LR, Silberman DM, Palumbo ML, Genaro AM, Frasch AC. 2006. Regulation of hippocampal gene expression is conserved in two species subjected to different stressors and antidepressant treatments. Biol Psychiatry. 59:244–251.
- Aonurm-Helm A, Jurgenson M, Zharkovsky T, Sonn K, Berezin V, Bock E, Zharkovsky A. 2008. Depression-like behaviour in neural cell adhesion molecule (NCAM)-deficient mice and its reversal by an NCAM-derived peptide, FGL. Eur J Neurosci. 28:1618–1628.
- Arai M, Itokawa M, Yamada K, Toyota T, Haga S, Ujike H, Sora I, Ikeda K, Yoshikawa T. 2004. Association of neural cell adhesion molecule 1 gene polymorphisms with bipolar affective disorder in Japanese individuals. Biol Psychiatry. 55:804–810.
- Atz ME, Rollins B, Vawter MP. 2007. NCAM1 association study of bipolar disorder and schizophrenia: Polymorphisms and alternatively spliced isoforms lead to similarities and differences. Psychiatr Genet. 17:55–67.
- Banasr M, Duman RS. 2008. Glial loss in the prefrontal cortex is sufficient to induce depressive-like behaviors. Biol Psychiatry. 64:863–870.
- Barbeau D, Liang JJ, Robitalille Y, Quirion R, Srivastava LK. 1995. Decreased expression of the embryonic form of the neural cell adhesion molecule in schizophrenic brains. Proc Natl Acad Sci USA. 92:2785–2789.
- Bisaz R, Sandi C. 2010. The role of NCAM in auditory fear conditioning and its modulation by stress: A focus on the amygdala. Genes Brain Behav. 9:353–364.
- Bisaz R, Schachner M, Sandi C. 2011. Causal evidence for the involvement of the neural cell adhesion molecule, NCAM, in chronic stress-induced cognitive impairments. Hippocampus. 21:56–71.
- Brennaman LH, Maness PF. NCAM in neuropsychiatric and neurodegenerative disorders. Neurochem Res. 2010.
- Brown SM, Henning S, Wellman CL. 2005. Mild, short-term stress alters dendritic morphology in rat medial prefrontal cortex. Cereb Cortex. 15:1714–1722.
- Bukalo O, Fentrop N, Lee AY, Salmen B, Law JW, Wotjak CT, Schweizer M, Dityatev A, Schachner M. 2004. Conditional ablation of the neural cell adhesion molecule reduces precision of spatial learning, long-term potentiation, and depression in the CA1 subfield of mouse hippocampus. J Neurosci. 24:1565–1577.
- Cambon K, Hansen SM, Venero C, Herrero AI, Skibo G, Berezin V, Bock E, Sandi C. 2004. A synthetic neural cell adhesion molecule mimetic peptide promotes synaptogenesis, enhances presynaptic function, and facilitates memory consolidation. J Neurosci. 24:4197–4204.
- Cerqueira JJ, Mailliet F, Almeida OF, Jay TM, Sousa N. 2007. The prefrontal cortex as a key target of the maladaptive response to stress. J Neurosci. 27:2781–2787.
- Cerqueira JJ, Pego JM, Taipa R, Bessa JM, Almeida OF, Sousa N. 2005. Morphological correlates of corticosteroid-induced changes in prefrontal cortex-dependent behaviors. J Neurosci. 25:7792–7800.
- Conboy L, Bisaz R, Markram K, Sandi C. Role of NCAM in Emotion and Learning. Neurochem Res. 2010.
- Conrad CD. 2010. A critical review of chronic stress effects on spatial learning and memory. Prog Neuropsychopharmacol Biol Psychiatry. 34:742–755.
- Conrad CD, Galea LA, Kuroda Y, McEwen BS. 1996. Chronic stress impairs rat spatial memory on the Y maze, and this effect is blocked by tianeptine pretreatment. Behav Neurosci. 110:1321–1334.
- Conrad CD, LeDoux JE, Magarinos AM, McEwen BS. 1999. Repeated restraint stress facilitates fear conditioning independently of causing hippocampal CA3 dendritic atrophy. Behav Neurosci. 113:902–913.
- Cook SC, Wellman CL. 2004. Chronic stress alters dendritic morphology in rat medial prefrontal cortex. J Neurobiol. 60:236–248.
- Craig LA, Hong NS, Kopp J, McDonald RJ. 2008. Emergence of spatial impairment in rats following specific cholinergic depletion of the medial septum combined with chronic stress. Eur J Neurosci. 27:2262–2271.
- Cremer H, Chazal G, Goridis C, Represa A. 1997. NCAM is essential for axonal growth and fasciculation in the hippocampus. Mol Cell Neurosci. 8:323–335.
- Cremer H, Lange R, Christoph A, Plomann M, Vopper G, Roes J, Brown R, Baldwin S, Kraemer P, Scheff S, . 1994. Inactivation of the N-CAM gene in mice results in size reduction of the olfactory bulb and deficits in spatial learning. Nature. 367:455–459.
- Dalla C, Antoniou K, Papadopoulou-Daifoti Z, Balthazart J, Bakker J. 2005. Male aromatase-knockout mice exhibit normal levels of activity, anxiety and “depressive-like” symptomatology. Behav Brain Res. 163:186–193.
- Dias-Ferreira E, Sousa JC, Melo I, Morgado P, Mesquita AR, Cerqueira JJ, Costa RM, Sousa N. 2009. Chronic stress causes frontostriatal reorganization and affects decision-making. Science. 325:621–625.
- Ducottet C, Belzung C. 2005. Correlations between behaviours in the elevated plus-maze and sensitivity to unpredictable subchronic mild stress: Evidence from inbred strains of mice. Behav Brain Res. 156:153–162.
- Farmer A, Elkin A, McGuffin P. 2007. The genetics of bipolar affective disorder. Curr Opin Psychiatry. 20:8–12.
- Fuchs E, Flugge G. 2001. Psychosocial stress induces molecular and structural alterations in the brain—How animal experiments help to understand pathomechanisms of depressive illnesses. Z Psychosom Med Psychother. 47:80–97.
- Gouirand AM, Matuszewich L. 2005. The effects of chronic unpredictable stress on male rats in the water maze. Physiol Behav. 86:21–31.
- Hartz BP, Ronn LC. NCAM in long-term potentiation and learning. Neurochem Res. 2010.
- Holmes A, Wellman CL. 2009. Stress-induced prefrontal reorganization and executive dysfunction in rodents. Neurosci Biobehav Rev. 33:773–783.
- Jorgensen OS. 1988. Neural cell adhesion molecule (NCAM) and prealbumin in cerebrospinal fluid from depressed patients. Acta Psychiatr Scand Suppl. 345:29–37.
- Kazlauckas V, Kalinine E, Leke R, Oses JP, Nunes F, Espinosa J, Mioranzza S, Lulhier F, Portela LV, Porciuncula LO, Lara DR. Distinctive effects of unpredictable subchronic stress on memory, serum corticosterone and hippocampal BDNF levels in high and low exploratory mice. Behav Brain Res. 2011.
- Kesner RP. 2000. Behavioral analysis of the contribution of the hippocampus and parietal cortex to the processing of information: Interactions and dissociations. Hippocampus. 10:483–490.
- Kiss JZ, Muller D. 2001. Contribution of the neural cell adhesion molecule to neuronal and synaptic plasticity. Rev Neurosci. 12:297–310.
- Kleene R, Schachner M. 2004. Glycans and neural cell interactions. Nat Rev Neurosci. 5:195–208.
- Lewis CM, Levinson DF, Wise LH, DeLisi LE, Straub RE, Hovatta I, Williams NM, Schwab SG, Pulver AE, Faraone SV, Brzustowicz LM, Kaufmann CA, Garver DL, Gurling HM, Lindholm E, Coon H, Moises HW, Byerley W, Shaw SH, Mesen A, Sherrington R, O'Neill FA, Walsh D, Kendler KS, Ekelund J, Paunio T, Lonnqvist J, Peltonen L, O'Donovan MC, Owen MJ, Wildenauer DB, Maier W, Nestadt G, Blouin JL, Antonarakis SE, Mowry BJ, Silverman JM, Crowe RR, Cloninger CR, Tsuang MT, Malaspina D, Harkavy-Friedman JM, Svrakic DM, Bassett AS, Holcomb J, Kalsi G, McQuillin A, Brynjolfson J, Sigmundsson T, Petursson H, Jazin E, Zoega T, Helgason T. 2003. Genome scan meta-analysis of schizophrenia and bipolar disorder, part II: Schizophrenia. Am J Hum Genet. 73:34–48.
- Li S, Wang C, Wang MW, Murakami Y, Matsumoto K. 2006. Impairment of the spatial learning and memory induced by learned helplessness and chronic mild stress. Pharmacol Biochem Behav. 83:186–193.
- Luine V, Martinez C, Villegas M, Magarinos AM, McEwen BS. 1996. Restraint stress reversibly enhances spatial memory performance. Physiol Behav. 59:27–32.
- Luine V, Villegas M, Martinez C, McEwen BS. 1994. Repeated stress causes reversible impairments of spatial memory performance. Brain Res. 639:167–170.
- Magarinos AM, Verdugo JM, McEwen BS. 1997. Chronic stress alters synaptic terminal structure in hippocampus. Proc Natl Acad Sci USA. 94:14002–14008.
- Maness PF, Schachner M. 2007. Neural recognition molecules of the immunoglobulin superfamily: Signaling transducers of axon guidance and neuronal migration. Nat Neurosci. 10:19–26.
- McEwen B, Lasley EN. 2003. Allostatic load: When protection gives way to damage. Adv Mind Body Med. 19:28–33.
- McEwen BS. 1999. Stress and hippocampal plasticity. Annu Rev Neurosci. 22:105–122.
- McEwen BS. 2000. Effects of adverse experiences for brain structure and function. Biol Psychiatry. 48:721–731.
- McEwen BS. 2003. Interacting mediators of allostasis and allostatic load: Towards an understanding of resilience in aging. Metabolism. 52:10–16.
- McEwen BS. 2005. Glucocorticoids, depression, and mood disorders: Structural remodeling in the brain. Metabolism. 54:20–23.
- Mello PB, Benetti F, Cammarota M, Izquierdo I. 2008. Effects of acute and chronic physical exercise and stress on different types of memory in rats. An Acad Bras Cienc. 80:301–309.
- Muller D, Mendez P, De Roo M, Klauser P, Steen S, Poglia L. Role of NCAM in spine dynamics and synaptogenesis. Neurochem Res. 2010.
- Nguyen C, Mattei MG, Mattei JF, Santoni MJ, Goridis C, Jordan BR. 1986. Localization of the human NCAM gene to band q23 of chromosome 11: The third gene coding for a cell interaction molecule mapped to the distal portion of the long arm of chromosome 11. J Cell Biol. 102:711–715.
- Park CR, Campbell AM, Diamond DM. 2001. Chronic psychosocial stress impairs learning and memory and increases sensitivity to yohimbine in adult rats. Biol Psychiatry. 50:994–1004.
- Poltorak M, Frye MA, Wright R, Hemperly JJ, George MS, Pazzaglia PJ, Jerrels SA, Post RM, Freed WJ. 1996. Increased neural cell adhesion molecule in the CSF of patients with mood disorder. J Neurochem. 66:1532–1538.
- Poltorak M, Khoja I, Hemperly JJ, Williams JR, el-Mallakh R, Freed WJ. 1995. Disturbances in cell recognition molecules (N-CAM and L1 antigen) in the CSF of patients with schizophrenia. Exp Neurol. 131:266–272.
- Pothion S, Bizot JC, Trovero F, Belzung C. 2004. Strain differences in sucrose preference and in the consequences of unpredictable chronic mild stress. Behav Brain Res. 155:135–146.
- Radecki DT, Brown LM, Martinez J, Teyler TJ. 2005. BDNF protects against stress-induced impairments in spatial learning and memory and LTP. Hippocampus. 15:246–253.
- Radley JJ, Rocher AB, Janssen WG, Hof PR, McEwen BS, Morrison JH. 2005. Reversibility of apical dendritic retraction in the rat medial prefrontal cortex following repeated stress. Exp Neurol. 196:199–203.
- Radley JJ, Rocher AB, Miller M, Janssen WG, Liston C, Hof PR, McEwen BS, Morrison JH. 2006. Repeated stress induces dendritic spine loss in the rat medial prefrontal cortex. Cereb Cortex. 16:313–320.
- Rougon G, Hobert O. 2003. New insights into the diversity and function of neuronal immunoglobulin superfamily molecules. Annu Rev Neurosci. 26:207–238.
- Rygula R, Abumaria N, Flugge G, Fuchs E, Ruther E, Havemann-Reinecke U. 2005. Anhedonia and motivational deficits in rats: Impact of chronic social stress. Behav Brain Res. 162:127–134.
- Sandi C. 2004. Stress, cognitive impairment and cell adhesion molecules. Nat Rev Neurosci. 5:917–930.
- Sandi C, Bisaz R. 2007. A model for the involvement of neural cell adhesion molecules in stress-related mood disorders. Neuroendocrinology. 85:158–176.
- Sandi C, Davies HA, Cordero MI, Rodriguez JJ, Popov VI, Stewart MG. 2003. Rapid reversal of stress induced loss of synapses in CA3 of rat hippocampus following water maze training. Eur J Neurosci. 17:2447–2456.
- Sandi C, Merino JJ, Cordero MI, Touyarot K, Venero C. 2001. Effects of chronic stress on contextual fear conditioning and the hippocampal expression of the neural cell adhesion molecule, its polysialylation, and L1. Neuroscience. 102:329–339.
- Sandi C, Richter-Levin G. 2009. From high anxiety trait to depression: A neurocognitive hypothesis. Trends Neurosci. 32:312–320.
- Sandi C, Touyarot K. 2006. Mid-life stress and cognitive deficits during early aging in rats: Individual differences and hippocampal correlates. Neurobiol Aging. 27:128–140.
- Segurado R, Detera-Wadleigh SD, Levinson DF, Lewis CM, Gill M, Nurnberger JIJr, Craddock N, DePaulo JR, Baron M, Gershon ES, Ekholm J, Cichon S, Turecki G, Claes S, Kelsoe JR, Schofield PR, Badenhop RF, Morissette J, Coon H, Blackwood D, McInnes LA, Foroud T, Edenberg HJ, Reich T, Rice JP, Goate A, McInnis MG, McMahon FJ, Badner JA, Goldin LR, Bennett P, Willour VL, Zandi PP, Liu J, Gilliam C, Juo SH, Berrettini WH, Yoshikawa T, Peltonen L, Lonnqvist J, Nothen MM, Schumacher J, Windemuth C, Rietschel M, Propping P, Maier W, Alda M, Grof P, Rouleau GA, Del-Favero J, Van Broeckhoven C, Mendlewicz J, Adolfsson R, Spence MA, Luebbert H, Adams LJ, Donald JA, Mitchell PB, Barden N, Shink E, Byerley W, Muir W, Visscher PM, Macgregor S, Gurling H, Kalsi G, McQuillin A, Escamilla MA, Reus VI, Leon P, Freimer NB, Ewald H, Kruse TA, Mors O, Radhakrishna U, Blouin JL, Antonarakis SE, Akarsu N. 2003. Genome scan meta-analysis of schizophrenia and bipolar disorder, part III: Bipolar disorder. Am J Hum Genet. 73:49–62.
- Shin KY, Won BY, Heo C, Kim HJ, Jang DP, Park CH, Kim S, Kim HS, Kim YB, Lee HG, Lee SH, Cho ZH, Suh YH. 2009. BT-11 improves stress-induced memory impairments through increment of glucose utilization and total neural cell adhesion molecule levels in rat brains. J Neurosci Res. 87:260–268.
- Sousa N, Lukoyanov NV, Madeira MD, Almeida OF, Paula-Barbosa MM. 2000. Reorganization of the morphology of hippocampal neurites and synapses after stress-induced damage correlates with behavioral improvement. Neuroscience. 97:253–266.
- Stewart MG, Davies HA, Sandi C, Kraev IV, Rogachevsky VV, Peddie CJ, Rodriguez JJ, Cordero MI, Donohue HS, Gabbott PL, Popov VI. 2005. Stress suppresses and learning induces plasticity in CA3 of rat hippocampus: A three-dimensional ultrastructural study of thorny excrescences and their postsynaptic densities. Neuroscience. 131:43–54.
- Stork O, Welzl H, Cremer H, Schachner M. 1997. Increased intermale aggression and neuroendocrine response in mice deficient for the neural cell adhesion molecule (NCAM). Eur J Neurosci. 9:1117–1125.
- Stork O, Welzl H, Wolfer D, Schuster T, Mantei N, Stork S, Hoyer D, Lipp H, Obata K, Schachner M. 2000. Recovery of emotional behaviour in neural cell adhesion molecule (NCAM) null mutant mice through transgenic expression of NCAM180. Eur J Neurosci. 12:3291–3306.
- Stork O, Welzl H, Wotjak CT, Hoyer D, Delling M, Cremer H, Schachner M. 1999. Anxiety and increased 5-HT1A receptor response in NCAM null mutant mice. J Neurobiol. 40:343–355.
- Strekalova T, Spanagel R, Dolgov O, Bartsch D. 2005. Stress-induced hyperlocomotion as a confounding factor in anxiety and depression models in mice. Behav Pharmacol. 16:171–180.
- Sullivan PF, Keefe RS, Lange LA, Lange EM, Stroup TS, Lieberman J, Maness PF. 2007. NCAM1 and neurocognition in schizophrenia. Biol Psychiatry. 61:902–910.
- Tomasiewicz H, Ono K, Yee D, Thompson C, Goridis C, Rutishauser U, Magnuson T. 1993. Genetic deletion of a neural cell adhesion molecule variant (N-CAM-180) produces distinct defects in the central nervous system. Neuron. 11:1163–1174.
- Touyarot K, Sandi C. 2002. Chronic restraint stress induces an isoform-specific regulation on the neural cell adhesion molecule in the hippocampus. Neural Plast. 9:147–159.
- Touyarot K, Venero C, Sandi C. 2004. Spatial learning impairment induced by chronic stress is related to individual differences in novelty reactivity: Search for neurobiological correlates. Psychoneuroendocrinology. 29:290–305.
- Vawter MP. 2000. Dysregulation of the neural cell adhesion molecule and neuropsychiatric disorders. Eur J Pharmacol. 405:385–395.
- Vawter MP, Hemperly JJ, Hyde TM, Bachus SE, VanderPutten DM, Howard AL, Cannon-Spoor HE, McCoy MT, Webster MJ, Kleinman JE, Freed WJ. 1998. VASE-containing N-CAM isoforms are increased in the hippocampus in bipolar disorder but not schizophrenia. Exp Neurol. 154:1–11.
- Vawter MP, Howard AL, Hyde TM, Kleinman JE, Freed WJ. 1999. Alterations of hippocampal secreted N-CAM in bipolar disorder and synaptophysin in schizophrenia. Mol Psychiatry. 4:467–475.
- Vawter MP, Usen N, Thatcher L, Ladenheim B, Zhang P, VanderPutten DM, Conant K, Herman MM, van Kammen DP, Sedvall G, Garver DL, Freed WJ. 2001. Characterization of human cleaved N-CAM and association with schizophrenia. Exp Neurol. 172:29–46.
- Venero C, Tilling T, Hermans-Borgmeyer I, Schmidt R, Schachner M, Sandi C. 2002. Chronic stress induces opposite changes in the mRNA expression of the cell adhesion molecules NCAM and L1. Neuroscience. 115:1211–1219.
- Vyas A, Mitra R, Shankaranarayana Rao BS, Chattarji S. 2002. Chronic stress induces contrasting patterns of dendritic remodeling in hippocampal and amygdaloid neurons. J Neurosci. 22:6810–6818.
- Walmod PS, Kolkova K, Berezin V, Bock E. 2004. Zippers make signals: NCAM-mediated molecular interactions and signal transduction. Neurochem Res. 29:2015–2035.
- Watanabe Y, Gould E, Cameron HA, Daniels DC, McEwen BS. 1992. Phenytoin prevents stress- and corticosterone-induced atrophy of CA3 pyramidal neurons. Hippocampus. 2:431–435.
- Wellman CL. 2001. Dendritic reorganization in pyramidal neurons in medial prefrontal cortex after chronic corticosterone administration. J Neurobiol. 49:245–253.
- Woolley CS, Gould E, McEwen BS. 1990. Exposure to excess glucocorticoids alters dendritic morphology of adult hippocampal pyramidal neurons. Brain Res. 531:225–231.
- Wright RL, Conrad CD. 2008. Enriched environment prevents chronic stress-induced spatial learning and memory deficits. Behav Brain Res. 187:41–47.