Abstract
Adrenal progesterone secretion increases along with corticosterone in response to stress in male and female rats to modulate some stress responses. Here we investigated the role of sex steroids in sex differences in the progesterone response to 60 min of restraint stress in adult male and female rats. Comparisons between males and females in the progesterone response were evaluated in parallel with corticosterone responses. From day 5 to 7 after gonadectomy, female and male rats were treated with estradiol or testosterone, respectively (OVX-E and ORCH-T groups), or oil (OVX and ORCH groups). Female rats in proestrus, intact and 7 d adrenalectomized (ADX) male rats were also studied. At 10:00 h, blood samples were withdrawn via an implanted jugular cannula before (−5 min), during (15, 30, 45, 60 min) and after (90 and 120 min) restraint stress to measure plasma progesterone and corticosterone concentrations by radioimmunoassay. Intact male and proestrus female rats exhibited similar progesterone responses to stress. Gonadectomy did not alter the amount of progesterone secreted during stress in female rats but decreased secretion in male rats. Unlike corticosterone, the progesterone response to stress in females was not influenced by estradiol. In males, testosterone replacement attenuated the progesterone and corticosterone responses to stress. Basal secretion of progesterone among intact, ORCH and ADX males was similar, but ADX-stressed rats secreted little progesterone. Hence, the gonads differently modulate adrenal progesterone and corticosterone responses to stress in female and male rats. The ovaries enhance corticosterone but not progesterone secretion, while the testes stimulate progesterone but not corticosterone secretion.
Introduction
For many decades, the stress responses to various harmful stimuli have been evaluated by corticosterone release from the adrenal glands. This hormone is the final product of the activation of the hypothalamus-pituitary-adrenal axis (HPA) and provides an endocrine pathway by which this stress response system interacts with many body functions during a variety of challenges (Chrousos & Gold, Citation1992). The pattern of corticosterone response to many stressors as well as the influence of age, sex and the modulatory role of sex hormones in this response are well documented (Critchlow et al., Citation1963; Goldstein & Kopin, Citation2007; Handa et al., Citation1994; Kitay, Citation1961, Citation1963; Romeo et al, Citation2005; Viau & Meaney, Citation1991). However, a number of other hormones and factors are released by this stress response system to maintain the dynamic equilibrium of the organism (Chrousos & Gold, Citation1992).
Progesterone is one of the primary steroids synthesized from cholesterol and is a precursor of many active steroids hormones in essentially all steroidogenic tissues, including corticosterone from the adrenal cortex. Progesterone secretion increases in response to a variety of stressors (Andersen et al., Citation2004; Budec et al., Citation2002; Deis et al., Citation1989; Fajer et al., Citation1971; Fenske, Citation1986; Jaroenporn et al., Citation2007; Nequin et al., Citation1975; Plas-Roser & Aron, Citation1981; Schaeffer & Aron, Citation1987; Traslavinã & Franci, Citation2011; Valdez et al., Citation2012; Welsh & Jonhson, Citation1981) and evidently modulates many body functions. Centrally, progesterone, through its neuroactive metabolite, 5α-pregnan-3α-ol-20-one, also known as 3α,5α-tetrahydroprogesterone (3α,5α-THP) or allopregnanolone, has been implicated as a regulator of stress responses because progesterone decreases HPA axis function by inhibiting hypothalamic gamma-aminobutyric acid type A receptors (GABA(A) receptor) that regulate corticotropin-releasing hormone (CRH) transcription and peptide levels (Brunton et al., Citation2009; Frye, Citation2007). In addition to their action on the HPA axis, 3α,5α-THP may act on GABA(A), N-Methyl-d-aspartate (NMDA) and dopamine receptors to facilitate sexual receptivity, conditioning and rewarding behaviors and to counteract stress-induced anxiety effects (Frye, Citation2007; Lovick, Citation2012).
Although there have been many reports about progesterone secretion and its action in neuroendocrine and behavioural responses to stress, the great majority of these studies were conducted only in females (Budec et al., Citation2002; Fajer et al., Citation1971; Nequin et al., Citation1975; Plas-Roser & Aron, Citation1981; Traslavinã & Franci Citation2011; Valdez et al., Citation2012) or only in males (Andersen et al., Citation2004; Fenske, Citation1986; Jaroenporn et al., Citation2007; Romeo et al., Citation2005; Schaeffer & Aron, Citation1987; Welsh & Jonhson, Citation1981). In these studies, the progesterone response was evaluated in parallel with corticosterone secretion in the systemic circulation only in five studies (Andersen et al., Citation2004; Fenske, Citation1986; Traslavinã & Franci, Citation2011; Valdez et al., Citation2012; Welsh & Jonhson, Citation1981). Two of these studies did not find an increase in progesterone secretion in response to confinement stress (Fenske, Citation1986) or to prolonged restraint stress (Andersen et al., Citation2004). Deis et al. (Citation1989) performed their study in both sexes; however, only the progesterone response was evaluated. In contrast, two other studies assessed corticosterone and progesterone responses to stress, but sex differences were not considered (Traslavinã & Franci, Citation2011; Welsh & Jonhson, Citation1981). Hence, to our knowledge, there are no studies in the literature describing the time-course of the progesterone response to acute stress that considers differences between sexes and the role of gonadal steroids in this response. In this study, we conducted a detailed investigation of the role of sex steroids in the sex differences in the progesterone response to acute restraint stress in adult male and female rats. We hypothesized that progesterone response to stress, likely corticosterone response, would be higher in proestrus female than in male rats. We also expected that progesterone responses to stress would diverge in a sex-dependent manner according to the sex hormone milieu. The comparisons of progesterone responses in males and females were evaluated in parallel with corticosterone responses to explore the possibility of additional sex-related differences in adrenocortical function.
Materials and methods
Animals
Female and male Wistar rats weighing 280–300 g were obtained from the animal house of the University of São Paulo at Ribeirão Preto and housed individually in plastic cages (30 × 19 × 13 cm) under conditions of controlled lighting (lights on 06:00 h–18:00 h) and temperature (22 ± 0.5 °C). The air exchange rate was 10 room volumes/h. Food and water were provided ad libitum. All experimental protocols were approved by the Ethics Committee on the Use of Experimental Animals of the University of São Paulo, Ribeirão Preto (License number: 09. 1.536.53.9).
Experimental design
Experiment 1: Effect of acute restraint stress on progesterone and corticosterone responses in male and proestrus female rats
We used females in proestrus because basal corticosterone levels in female rats are higher than in males only in this phase of the cycle (Atkinson & Waddell, Citation1997; Critchlow et al., Citation1963; Raps & Barthe, Citation1970). Vaginal smears were taken daily, and only rats showing regular 4 d cycles were used. Jugular vein cannulation for serial blood sampling was performed on males and during diestrus II of cycling female rats at 17:00 h. The next morning, at 09:00 h, vaginal smears were taken to confirm the proestrus phase. At 10:00 h, intact male and proestrus female rats were submitted to 60 min of restraint stress. Blood samples were withdrawn before (−5 min), during (15, 30, 45, 60 min) or after (90 and 120 min) restraint stress to evaluate plasma progesterone and corticosterone concentrations using radioimmunoassay (RIA). The control rats were kept in their cages and were subjected to the same blood sampling protocol. Male rats were euthanized by conscious decapitation at the end of the experiment. Female rats were euthanized the next day (estrus), the ovaries were removed and the oviducts were dissected and squashed between two microscope slides. The number of oocytes from both oviduct ampullae was counted under a microscope (Zeiss, Goettingen, Germany) with a 2.5 × lens to confirm the occurrence of ovulation. Only data from ovulating rats were used in the study.
Experiment 2: Effect of sex steroids on progesterone and corticosterone responses to acute restraint stress in male and female rats
Ten days prior to the experiment, female and male rats were gonadectomized (ovariectomized, OVX, and orchiectomized, ORCH, groups, respectively). During days 7–10 after surgery, the rats were injected subcutaneously with corn oil (OVX and ORCH groups), estradiol (OVX-E group, 10 µg/0.2 mL/rat of estradiol cypionate) or testosterone (ORCH-T group, 0.25 mg/0.2 mL/rat of testosterone propionate; see explanation in “Material and methods” section). On day 10, the jugular vein was cannulated at 17:00 h for serial blood sampling. On day 11, the rats were subjected (restraint groups) or not (control groups) to 60 min of restraint stress at 10:00 h and subjected to the same blood sampling protocol of experiment 1 for progesterone and corticosterone measurement.
Experiment 3: Effect of adrenalectomy on progesterone and corticosterone responses to acute restraint stress
Intact male rats were subjected to bilateral adrenalectomy and received corticosterone replacement (25 mg/L) for 7 consecutive days, starting immediately after the adrenalectomy. On day 6, the jugular vein was cannulated. On day 7, adrenalectomized rats (ADX) were subjected (ADX restraint group) or not (ADX control group) to 60 min of restraint stress and were subsequently subjected to the same blood sampling protocol as in experiments 1 and 2 for progesterone and corticosterone measurement.
Surgery and prophylactic care
Under tribromoethanol anesthesia (250 mg/kg bw, 0.1 ml/100 g, i.p.), a SILASTIC brand cannula (Dow Corning Corp., Midland, MI) was inserted through the external jugular vein into the right atrium as previously described (Harms & Ojeda, Citation1974). Under a mixture of ketamine and xylazine (0.1 mL/100 g bw, i.p. ketamine chlorhydrate; Agner, São Paulo, Brazil; 80 mg/kg bw and xylazine Coopazine coopers, São Paulo, Brazil; 10 mg/kg bw) anesthesia, female rats underwent bilateral ovariectomy in which the ovaries were removed through small lateral incisions in the skin and muscle layer. In male rats, the testes were exposed through a midline incision in the scrotum, the spermatic cords were ligated, and both testes were removed. Bilateral adrenalectomy was performed via a dorsal midline approach with bilateral incisions in the skin and a small cut through the muscle layer at an angle between the last rib and the vertebral column. The muscle incisions were sutured with dissolvable stitches, and the skin incisions apposed with wound-clips. Shortly after all surgical procedures, the rats were treated with analgesic (Banamine, 2.5 mg/kg, 0.1 mL/rat, s.c.) and antibiotic (Pentabiotic Fort Dodge, 0.2 ml/rat, i.m.).
Blood samples
A length of polyethylene tubing (PE-50) filled with heparinized saline (0.9% NaCl, 30 IU heparin/mL) was connected to the jugular catheter. Blood samples of 200 µL were withdrawn into plastic heparinized syringes. After the removal of each blood sample, an equal volume of warmed sterile 0.9% NaCl was injected through the catheter. The plasma was separated by centrifugation at 1200 × g for 20 min at 4 °C and stored at −20 °C until the hormone assay.
Restraint stress paradigm
The restraint stress consisted of placing the rats in a plastic cylinder that was well matched to the size and weight of the rats (5 cm inside diameter and 25.5 cm in length). The proximal and distal ends were sealed by PVC covers, with a central orifice of 3 cm in diameter, that were custom-made to allow protrusion of the snout and tail. This procedure took approximately 1 min, and the duration of the restraint stress period was 60 min from that point.
Hormone replacement
For 3 consecutive days, the ovariectomized rats received subcutaneous injections of 10 µg/0.2 mL/rat of estradiol cypionate (Pfizer, São Paulo, Brazil) or corn oil (OVX-E and OVX groups, respectively), and the ORCH rats were treated with testosterone propionate (0.25 mg/0.2 mL/rat) or corn oil (ORCH-T and ORCH groups, respectively). The doses of steroids were chosen to yield physiological levels of plasma estradiol (Szawka et al., Citation2009) and testosterone. Three doses of testosterone were tested in the ORCH rats. The subcutaneous injections of 0.25, 0.4 and 0.8 mg/0.2 mL/rat that were administered over 3 d resulted in plasma concentrations of 3.43 ± 0.71 ng/mL; 5.3 ± 1.01 ng/mL and 9.32 ± 0.85 ng/mL, respectively. The 0.25 mg/0.2 mL/rat dose was chosen because the resulting plasma concentration was closest to that of the intact rats (1.96 ± 0.19 ng/mL). The ADX rats were given 0.9% saline with corticosterone (25 mg/L; Sigma-Aldrich, St Louis, MO) dissolved in 0.5% ethanol to drink (Uchoa et al., Citation2009).
Hormone assays
Progesterone, testosterone and corticosterone were assayed using double-antibody RIA. The plasma progesterone and testosterone concentrations were determined using specific DSL-Active kits (Diagnostics Systems Laboratories, Webster, TX). Corticosterone concentration was measured after plasma extraction with 100% ethanol. The homogenate was stirred vigorously for 25 s and centrifuged at 1200 × g for 15 min at 4 °C. The supernatant was poured into a new test tube and lyophilized for 3 h. The samples were re-suspended in 200 µL of 0.1% albumin phosphate buffer. A standard curve was generated using successive dilutions (1:2) of the standard solution (20 ng/mL) with albumin phosphate buffer. After the standard (50 µL) or the sample, the primary antibody (100 µL) and the labeled hormone (50 µL/5000 cpm) were added to the test tubes and kept at room temperature for 24 h. The separation of the free hormone was performed using charcoal. The samples were centrifuged for 20 min at 1200 × g, and the supernatant was poured into a capped tube, to which 2.5 mL of scintillation fluid was added for the measurement using a beta radiation counter. The primary antibody and standard were provided by Sigma, and the 3H-labelled hormone was provided by Amersham (San Francisco, CA). The lower limits of detection for progesterone, testosterone and corticosterone were 0.02, 0.01 and 0.05 ng/mL, respectively. The intra-and inter-assay coefficients of variation were 5% and 2.5% for progesterone, 7.5% and 8.1% for testosterone and 6.6% and 9% for corticosterone, respectively.
Statistical analyses
The data are presented as group mean ± SEM. The progesterone and corticosterone plasma concentrations over the experimental period were analyzed using a two-way ANOVA followed by the Bonferroni post hoc test. The integrated progesterone and corticosterone secretory responses were expressed as the area under the curve with respect to increase (AUCinc of the interval 15–60 min (Pruessner et al., Citation2003) and determined using the trapezoid rule. In experiments 1 and 2, the differences in AUCinc were determined using a two-way ANOVA followed by the Bonferroni post hoc test. The differences in experiment 3 were determined using a one-way ANOVA followed by the Newman–Keuls post hoc test. The differences among groups were considered statistically significant when p < 0.05. All statistical analyses were performed with GraphPad Prism (GraphPad Software Inc, San Diego, CA).
Results
Experiment 1: Effect of acute restraint stress on progesterone and corticosterone responses in male and proestrus female rats
Both progesterone and corticosterone concentrations increased significantly during the restraint period regardless of sex ( and ). Two-way ANOVA showed a significant increase in progesterone secretion during stress in both proestrus female (F(6,85) = 4.448; p < 0.001) and male rats (F(6,81) = 6.795; p < 0.0001), starting at 15 min after the beginning of the stress session in both sexes (p < 0.001). Progesterone concentrations returned to levels not significantly different from controls 0 (time point: 60 min) and 30 min (time point: 90 min), after the end of the stress session, in females and males, respectively (). Total progesterone secreted at rest or under stress conditions did not differ among sexes (F(1, 26) = 96; p < 0.0001; ). Corticosterone secretion responded to stress similarly to progesterone. Corticosterone secretion increase significantly during stress in both proestrus female (F(6,90) = 4.266; p < 0.001) and male rats (F(6,94) = 6.976; p < 0.0001). Similar to progesterone, corticosterone concentrations also returned to resting levels 30 min after the end of the restraint session (). There was an increase in the total amount of corticosterone secreted during the stress period in both sexes (F(1,26) = 66.83; p < 0.0001, ). However, female rats displayed higher basal (p < 0.05) and stress-induced corticosterone concentrations (F(1, 26) =28.51; p < 0.0001) compared with male rats.
Figure 1. Effect of restraint stress for 60 min (black line) on plasma progesterone (P) and corticosterone (CORT) concentrations in female rats in proestrus (A and D; Control group: n = 6 and Restraint group: n = 10) and in intact male rats (B and E; Control group: n = 7 and Restraint group: n = 7). Blood samples were withdrawn before (−5 min), during (15, 30, 45 and 60 min) and after (90 and 120 min) the stress session. (C, F) Area under the curve with respect to increase (AUCinc) in the total secretion of P and CORT during the stress response period (15–60 min). The data are shown as the mean ± SEM. A two-way ANOVA followed by a Bonferroni post hoc test was used in all analyses. *p < 0.05 versus control group. Different letters represent significantly different values.
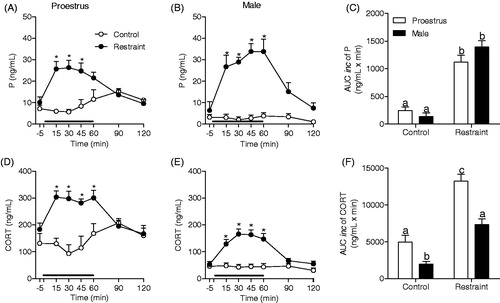
Figure 2. Effect of gonadectomy (GNX) on progesterone (P) and corticosterone (CORT) response to restraint stress for 60 min (black line). Ovariectomized (A and D; OVX control: n = 8 and OVX restraint n = 6) and orchiectomized rats (B and E; ORCH control: n = 5 and ORCH Restraint: n = 5) treated with corn oil. Blood samples were withdrawn before (−5 min), during (15, 30, 45 and 60 min) and after (90 and 120 min) the stress session. (C, F) Area under the curve with respect to increase (AUCinc) in the total secretion of P and CORT during the stress response period (15–60 min) in GNX females (F) and males (M). The data are shown as the mean ± SEM. A two-way ANOVA followed by a Bonferroni post hoc test was used in all analyses. (C and F), the control groups were not compared with the stressed groups. *p < 0.05 versus control group, #p < 0.05 versus OVX control group, ###p < 0.0001 versus GNX restraint group.
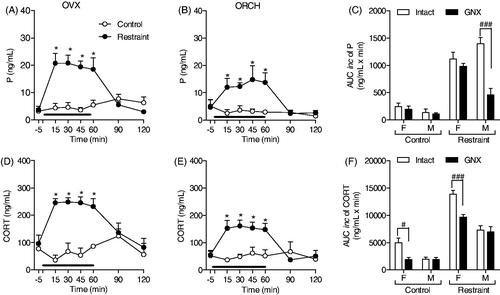
Experiment 2: Effect of sex steroids on progesterone and corticosterone responses to acute restraint stress in male and female rats
Effect of gonadectomy on progesterone and corticosterone responses to stress in male and female rats
shows the effect of 60 min of restraint stress on progesterone and corticosterone secretion in female and male rats. Similar to intact rats, OVX (F(6,77) = 9.115; p < 0.0001) and ORCH groups (F(6,48) = 3.289; p < 0.01) showed significant stress-induced increases in progesterone secretion. In both sexes, progesterone secretion increased only during the stress application (time points: 15–60 min; p < 0.05). shows a sex-related effect of gonadectomy on progesterone secretion (F(1,23) = 9.875; p < 0.01). Ovariectomy did not alter the basal or stress-induced progesterone secretion in females, but orchiectomy decreased the progesterone response (p < 0.05; ). In both sexes, the corticosterone response to stress exhibited the same pattern observed in intact rats (OVX group: F(6,59) = 5.689; p < 0.0001; ORCH group: F(6,46) = 3.370; p < 0.01; ). shows a sex-related effect of gonadectomy on corticosterone secretion both at rest (F(1,19) = 6.899; p < 0.02) and under stress conditions (F(1,23) = 4.77; p < 0.05). Orchiectomy did not alter the basal or stress-induced corticosterone secretion, but ovariectomy decreased corticosterone secretion both at rest and during the stress application.
Effect of estradiol replacement on progesterone and corticosterone responses to stress in female rats
shows the effect of 60 min of restraint stress on progesterone (A) and corticosterone (C) secretion in ovariectomized estradiol-treated female rats. OVX-E rats exhibited the same adrenocortical pattern of response to stress (F(6,77) = 6.226; p < 0.0001 and F(6,59) = 8.315; p < 0.0001, respectively) with significant elevations in progesterone and corticosterone plasma concentrations only during the stress application (time points: 15–60 min; p < 0.05). As shown in , estradiol replacement therapy did not alter the total amount of progesterone at rest and under stress conditions. In contrast, estradiol replacement partially restored the decrease in corticosterone resting concentrations induced by ovariectomy and the stress-induced increase in corticosterone concentrations (F(2,31) = 3.55; p < 0.0001, ).
Figure 3. Effect of estradiol replacement therapy on progesterone (P) and corticosterone (CORT) response to restraint stress for 60 min (black line) in female rats. (A, C) Time course of progesterone and corticosterone responses to stress in ovariectomized rats treated with estradiol (OVX-E control: n = 9 and OVX-E Restraint: n = 5). Blood samples were withdrawn before (−5 min), during (15, 30, 45 and 60 min) and after (90 and 120 min) the stress session. (B, D) Area under the curve with respect to increase (AUCinc) in the total secretion of P and CORT during the stress response period (15–60 min) of proestrus (Control group: n = 6 and Restraint group: n = 10), OVX (OVX control: n = 8 and OVX restraint: n = 6) and OVX-E rats. The hormone data from proestrus and OVX rats are the same previously presented in . The data are shown as the mean ± SEM. A two-way ANOVA followed by a Bonferroni post hoc test was used in all analyses. *p < 0.05 versus control. Different letters represent significantly different values.
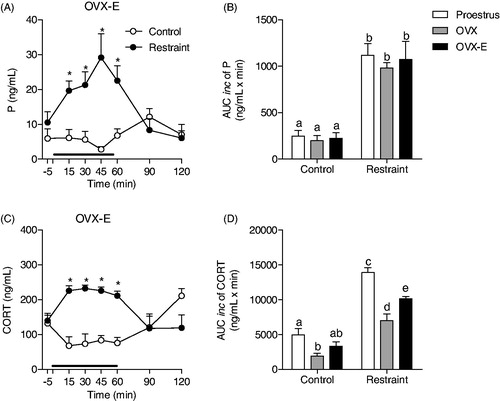
Effect of testosterone replacement on progesterone and corticosterone responses to stress in male rats
shows the effect of 60 min of restraint stress on progesterone (A) and corticosterone (C) secretion in orchiectomized testosterone-treated male rats. Statistical analyses showed no significant stress-induced progesterone or corticosterone secretion in ORCH-T rats (F(6,46) = 0.3607, p = 0.899; and F(6,47) = 0.3743, p = 0.891; ). Testosterone replacement did not restore the decrease in the stress-induced increase in progesterone concentrations induced by orchiectomy (F(2,27) = 17.41; p < 0.0001, ORCH-T versus intact p < 0.0001; ORCH-T versus ORCH p > 0.05; ). shows that testosterone replacement increased corticosterone resting concentrations (F(2,27) = 14.12; p < 0.0001, ORCH-T group versus intact and ORCH groups p < 0.05) and decreased the stress-induced increase in corticosterone concentrations (ORCH-T group versus intact and ORCH groups p < 0.001).
Figure 4. Effect of testosterone replacement therapy on progesterone (P) and corticosterone (CORT) response to restraint stress for 60 min (black line) in male rats. (A, C) Time course of progesterone and corticosterone responses to stress in orchiectomized rats treated with testosterone (ORCH-T Control: n = 4 and ORCH-T Restraint: n = 5). Blood samples were withdrawn before (−5 min), during (15, 30, 45 and 60 min) and after (90 and 120 min) the stress session. (B, D) Area under the curve with respect to increase (AUCinc) in the total secretion of P and CORT during the stress response period (15–60 min) of intact (Control group: n = 7 and restraint group: n = 7), ORCH (ORCH control: n = 5 and ORCH restraint: n = 5) and ORCH-T rats. The hormonal data from intact and ORCH rats are the same previously presented in . The data are shown as the mean ± SEM. A two-way ANOVA followed by a Bonferroni post hoc test was used in all analyses. Different letters represent significantly different values.
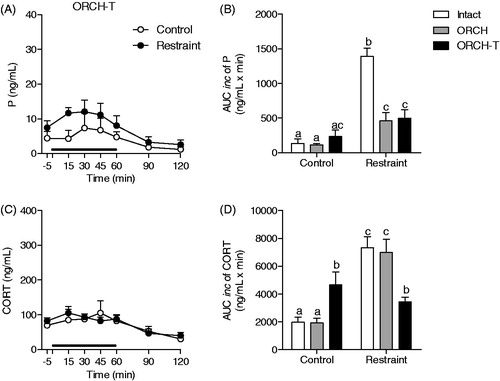
Experiment 3: Effect of adrenalectomy on progesterone and corticosterone responses to acute restraint stress
As shown in , 60 min of restraint stress caused a slight increase in progesterone secretion in the ADX male rats, which was significant only at 30 min after the end of the stress session (F(1,76) = 24.57; p < 0.0001, time point 90 min: p < 0.05). Adrenalectomy per se did not alter the total amount of progesterone secretion in non-stressed rats compared with that in the intact and ORCH rats but blunted the increase induced by restraint stress ()
Figure 5. Effect of adrenalectomy on progesterone (P) response to restraint stress for 60 min (black line) in male rats. The rats were adrenalectomized 7 d prior to experiment (ADX Control: n = 7 and ADX Restraint: n = 6) and were a solution of 0.9% saline plus corticosterone (25 mg/kg) to drink. (A) Blood samples were withdrawn through a jugular catheter before (−5 min), during (15, 30, 45 and 60 min) and after (90 and 120 min) the stress session. (B) Area under the curve with respect to increase (AUCinc) of the total secretion of P during the stress response period (15–60 min) in intact, orchiectomized (ORCH) and adrenalectomized (ADX) male rats. The data from intact and ORCH rats are the same previously presented in . A two-way ANOVA followed by a Bonferroni post hoc test were used in all analyses. The data are shown as the mean ± SEM. *p < 0.05 versus control group. Different letters represent significantly different values.
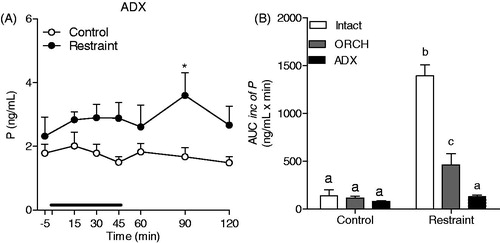
Discussion
The present study has shown that, regardless of sex, the patterns of progesterone and corticosterone secretion induced by acute stress are similar. However, these responses diverge in a sex-dependent manner when sex steroids levels are manipulated. Corticosterone secretion in response to stress is stimulated by ovarian estradiol in females whereas it is inhibited by testosterone in males. However, progesterone secretion induced by stress is not affected by estradiol in females but in males it is stimulated by some testicular factor other than testosterone.
As expected, the plasma corticosterone concentrations of proestrus rats, at rest and under stress conditions, are higher when estradiol plasma levels are high than those in male rats (Kitay, Citation1961; Raps & Barthe, Citation1970). Indeed, the hyperactivity of the HPA axis found during the proestrus phase has been attributed to the high concentrations of estradiol (Kitay, Citation1965; Viau & Meaney, Citation1991). However, progesterone plasma concentrations, basal or stress-induced, were similar in proestrus female and intact male rats, demonstrating that unlike corticosterone, progesterone secretion is not influenced by estradiol.
Ovariectomy did not alter the progesterone response to stress, indicating that the adrenal glands are the only source of progesterone during stress in females, as reported by others (Andersen et al., Citation2004; Budec et al., Citation2002; Romeo et al., Citation2004). Conversely, orchiectomy considerably reduced the progesterone response to stress, indicating that, in addition to the adrenal glands, the testes may also secrete progesterone in stress situations. Thus, we evaluated the contribution of testicular progesterone in the absence of adrenal glands. The finding that adrenalectomy did not affect progesterone secretion makes unclear whether the testes or adrenal glands maintain the basal levels of progesterone in intact rats. Interestingly, the progesterone response to stress was abolished in ADX male rats. Thus, the low progesterone levels, considered to be secreted by the testes, in the ADX rats do not explain the 50% decrease in progesterone response found in the ORCH rats, indicating the existence of a functional and bidirectional crosstalk between the testes and adrenal glands. Indeed, administration of adrenocorticotrophic hormone (ACTH) has been previously shown to stimulate testicular steroidogenesis only in the presence of the adrenal glands, indicating the existence of an adrenal signal to the hypothalamo-pituitary gonadal (HPG) axis in male rats (Feek et al., Citation1989). In turn, orchiectomy alters adrenal weight, morphology and histochemistry, affecting adrenal function (Malendowicz, Citation1979) and adrenal steroidogenesis (Stege et al., Citation1987). Thus, the products of both endocrine glands could be modulating the enzymatic activity of each other to influence their end-product output. For example, orchiectomy increases the adrenocortical expression of the steroid 5α-reductase, which catalyses the conversion of steroids to their 5α-reduced metabolites (Hiromichi et al., Citation1998). Thus, steroid 5α-reductase may be involved in regulating the levels of adrenal progesterone depending on the levels of sex hormones. Moreover, the low progesterone levels in ADX rats may not represent the testicular pattern of stress response in intact rats. Nevertheless, the cellular and molecular mechanisms underlying this bidirectional crosstalk between the testes and adrenal glands are unclear and warrants further investigation.
Curiously, testosterone replacement did not alter the orchiectomy-induced decrease in the progesterone response to stress, indicating that some testicular factor other than testosterone is required to induce the full adrenal progesterone response in males. However, testosterone replacement inhibited the corticosterone response. This unexpected dissociation in the steroidogenesis responses of the adrenal cortex might be due to the complex paracrine interaction between the adrenal cortex and medulla. Additionally, the splanchnic innervation of the adrenal cortex directly affects steroidogenesis, adrenal size and vasculature, which is evidently involved in the fine tuning of adrenocortical function to the needs of the body (Ehrhat-Bornstein et al., Citation1998).
In this study, estradiol replacement only partially prevented the ovariectomy-induced decrease in corticosterone secretion both at rest and during stress conditions. This finding differs from previous studies, in which estradiol replacement in ovariectomized rats re-established the sex differences in corticosterone secretion (Kitay et al., Citation1965). The stimulating effect of estradiol on the HPA axis have been shown to be transient (Viau & Meaney, Citation1991). These authors described a full response to stress only when the stressor is applied up to 24 h after the beginning of the estradiol treatment. In the present study, the restraint stress was applied 72 h after the first injection of estradiol, possibly accounting for the partial re-establishment of the corticosterone response to stress observed in our study.
Moreover, the estradiol replacement did not alter the progesterone response to stress. This finding raises the question of how estradiol increases corticosterone secretion without affecting its precursor. Despite its central actions on the HPA axis, estradiol can directly act on the adrenal glands (Cutler et al., Citation1978), which could positively modulate the enzymatic steps of corticosterone conversion. Moreover, estradiol increases splanchnic nerve activity, which innervates the adrenal cortex and releases several neurotransmitters, which could be producing differential effects on adrenal steroidogenesis by acting on the sympathetic outflow (Charlton, Citation1990; He & Wang, Citation1998; Holgert et al., Citation1998; Tóth, Citation1998).
In addition to its well-known effects on the female reproductive system, progesterone also acts on male reproduction, influencing spermiogenesis, the acrosome reaction and testosterone synthesis in Leydig cells in men (Oettel & Mukhopadhyay, Citation2004). The results shown herein raise an important issue about the role of progesterone during stressful situations. Progesterone also has multiple effects on the central nervous system (CNS), such as neurogenesis, sleep and memory improvement, through its derivative neuroactive steroids (Oettel & Mukhopadhyay, Citation2004). Thus, we might hypothesize that during stress, these effects of progesterone could counteract the deleterious effects induced by the high levels of glucocorticoids. Moreover, recent studies demonstrated that the progesterone metabolite, 3α,5α-THP, has a role in fetal and adolescent neural development, as gestational exposure to stress reduces neural development in juvenile rats of both sexes by altering central progesterone formation (Paris & Frye, Citation2011). Similarly, in late pregnant rats, 3α,5α-THP inhibits oxytocin secretion in response to immune challenges by stimulating endogenous opioids to minimize the risk of preterm labour (Brunton et al., Citation2012).
Additionally, the administration of progesterone and 3α,5α-THP has been shown to attenuate ACTH and corticosterone responses (Patchev et al., Citation1996). Behavioral studies also demonstrate that the increase of 3α,5α-THP levels in the rat hypothalamus after acute swim stress is not prevented by adrenalectomy. These findings indicate the de novo synthesis of this neurosteroid in response to stress (Purdy et al., Citation1991). Hence, the increased secretion of progesterone together with the de novo synthesis within the CNS may play a role, either synergistic or antagonistic to corticosterone, adrenaline and other stress mediators, to cope with neuroendocrine and behavioral adaptation.
This is the first published study to investigate the sex differences in the response to acute stress while comparing the pattern of progesterone and corticosterone response in different hormonal milieu. We have shown that the gonads differently modulate the adrenal progesterone and corticosterone in response to stress in females and males. Although the ovary stimulates corticosterone but not adrenal progesterone secretion, the testis stimulates progesterone but not corticosterone secretion.
Declaration of interest
The authors report no conflicts of interest. This study was financially supported by FAPESP (04/09638-9) and CNPq (130204/2008-0).
Acknowledgements
The authors would like to thank Maicon Renato da Silva, Lívia Maria Pala Anselmo and Ruither de Oliveira Gomes Carolino for technical support.
References
- Andersen ML, Bignotto M, Machado RB, Tufik S. (2004). Different stress modalities result in distinct steroid hormone responses by male rats. Braz J Med Biol Res 37:791–7
- Atkinson HC, Waddell BJ. (1997). Circadian variation in basal plasma corticosterone and adrenocorticotropin in the rat: sexual dimorphism and changes across the estrous cycle. Endocrinology 138:3842–8
- Brunton PJ, Bales J, Russell JA. (2012). Allopregnanolone and induction of endogenous opioid inhibition of oxytocin responses to immune stress in pregnant rats. J Neuroendocrinol 24(4):690–700
- Brunton PJ, McKay AJ, Ochedalski T, Piastowska A, Rebas E, Lachowicz A, Russell JA. (2009). Central opioid inhibition of neuroendocrine stress responses in pregnancy in the rat is induced by the neurosteroid allopregnanolone. J Neurosci 29:6449–60
- Budec M, Koko V, Milovanovic T, Balint-Peric L, Petkovic A. (2002). Acute ethanol treatment increases level of progesterone in ovariectomized rats. Alcohol 26:173–8
- Charlton BG. (1990). Adrenal cortical innervation and glucocorticoid secretion. J Endocrinol 126:5–8
- Chrousos GP, Gold PW. (1992). The concepts of stress and stress system disorders. Overview of physical and behavioral homeostasis. JAMA 267:1244–52
- Critchlow V, Liebelt RA, Bar-Sela M, Mountcastle W, Lipscomb HS. (1963). Sex difference in resting pituitary-adrenal function in the rat. Am J Physiol 205:807–15
- Cutler GB, Barnes KM, Sauer MA, Loriaux DL. (1978). Estrogen Receptor in Rat Adrenal Gland. Endocrinology 102:252–7
- Deis RP, Leguizamon E, Jahn GA. (1989). Feedback regulation by progesterone of stress-induced prolactin release in rats. J Endocrinol 120:37–43
- Ehrhart-Bornstein M, Hinson JP, Bornstein SR, Scherbaum WA, Vinson GP. (1998). Intraadrenal interactions in the regulation of adrenocortical steroidogenesis. Endocr Rev 19(2):101–43
- Fajer AB, Holzbauer M, Newport HM. (1971). The contribution of adrenal gland to the total amount of progesterone produced in the female rat. J Physiol 214(1):115–26
- Feek CM, Tuzi NL, Edwards CR. (1989). The adrenal gland and progesterone stimulates testicular steroidogenesis in the rat in vivo. J Steroid Biochem 32(4):573–9
- Fenske M. (1986). Adrenal function in the Mongolian gerbil (Meriones unguiculatus): influence of confinement stress upon glucocorticoid, progesterone, dehydroepiandrosterone, testosterone and androstenedione plasma levels, adrenal content and in-vitro secretion. Exp Clin Endocrinol 87(1):15–25
- Frye CA. (2007). Progestin influence motivation, reward, conditioning, stress and/or response to drug of abuse. Pharmacol Biochem Behav 86(2):209–19
- Goldstein DS, Kopin IJ. (2007). Evolution of concepts of stress. Stress 10:109–20
- Handa RJ, Burgess LH, Kerr JE, O'Keefe JA. (1994). Gonadal steroid hormone receptors and sex differences in the hypothalamo-pituitary-adrenal axis. Horm Behav 28:464–76
- Harms PG, Ojeda SR. (1974). A rapid and simple procedure for chronic cannulation of the rat jugular vein. J Appl Physiol 36:391–92
- He XR, Wang W, Crofton JT, Share L. (1998). Effects of 17beta-estradiol on sympathetic activity and pressor response to phenylephrine in ovariectomized rats. Am J Physiol 275:R1202–8
- Hiromichi Y, Yoshihiro T, Tadayuki M, Kazunori I. (1998). Steroid 5 α-reductase type 1 immunolocalized in the adrenal gland of normal, gonadectomised, and sex hormone-supplemented rats. Histochem Cell Biol 109:127–34
- Holgert H, Dagerlind A, Hokfelt T. (1998). Immunohistochemical characterization of the peptidergic innervation of the rat adrenal gland. Horm Metab Res 30:315–22
- Jaroenporn S, Nagaoka K,Kasahara C, Ohta R, Watanabe G, Taya K. (2007). Physiological roles of prolactin in the adrenocortical response to acute restraint stress. Endocr J 54(5):703–11
- Kitay JI. (1961). Sex differences in adrenal cortical secretion in the rat. Endocrinology 68:818–24
- Kitay JI. (1963). Effects of testosterone on pituitary corticotrophin and adrenal steroid secretion in male and female rats. Acta Endocrinol (Copenh) 43:601–8
- Kitay JI. (1965). Effects of oophorectomy and various doses of estradiol-17beta on corticosterone production by rat adrenal slices. Proc Soc Exp Biol Med 120:193–6
- Lovick TA. (2012). Estrous cycle and stress: influence of progesterone on the female brain. Braz J Med Biol Res 45(4):314–20
- Malendowicz LK. (1979). Sex differences in adrenocortical structure and function. V. The effects of postpubertal gonadectomy and gonadal hormone replacement on nuclear-cytoplasmatic ratio, morphology and histochemistry of rat adrenal cortex. Folia Histochem Cytochem (Krakow) 17(3):195–214
- Nequin LG, Alvarez JA, Campbell CS. (1975). Alterations in steroids and gonadotropin release resulting from surgical stress during the morning of proestrus in 5-day cyclic rats. Endocrinology 97(3):718–24
- Oettel M, Mukhopadhyay AK. (2004). Progesterone: the forgotten hormone in men? The Aging Male 7:236–57
- Paris JJ, Frye CA. (2011). Gestacional exposure to variable stressors produces decrements in cognitive and neural development of juvenile males and females rats. Curr Top Med Chem 11(13):1706–13
- Patchev VK, Hassan AH, Holsboer DF, Almeida OF. (1996). The neurosteroid tetrahydroprogesterone attenuates the endocrine response to stress and exerts glucocorticoid-like effects on vasopressin gene transcription in the rat hypothalamus. Neuropsychopharmacology 15:533–40
- Plas-Roser S, Aron C. (1981). Stress related effects in the control of sexual receptivity and in the secretion of progesterone by the adrenals in cyclic female rats. Physiol Behav 27(2):261–4
- Pruessner JC, Kirschbaum C, Meinlschmid G, Hellhammer DH. (2003). Two formulas for computation of the area under the curve represent measures of total hormone concentration versus time-dependent change. Psychoneuroendocrinology 28:916–31
- Purdy RH, Morrow AL, Moore Jr PH, Paul SM. (1991). Stress-induced elevation of gamma-aminobutyric acid type A receptor-active steroids in the rat brain. Proc Natl Acad Sci USA 88:4553–7
- Raps D, Barthe PL. (1970). Plasma and adrenal corticosterone levels during the different phases of the sexual cycle in normal female rats. Experientia 27:339–40
- Romeo RD, Bellani R, McEwen BS. (2005). Stress-induced progesterone secretion and progesterone receptor immunoreactivity in the paraventricular nucleus are modulated by pubertal development in male rats. Stress 8(4):265–71
- Romeo RD, Lee SJ, McEwen BS. (2004). Differential stress reactivity in intact and ovariectomized prepubertal and adult female rats. Neuroendocrinology 80:387–93
- Schaeffer C, Aron C. (1987). Stress-related effects on the secretion of progesterone by the adrenals in castrated male rats presented to stimulus males. Involvement of oestrogen. Acta Endocrinol (Copenh) 114(3):440–5
- Stege R, Eriksson A, Henriksson P, Carlstron K. (1987). Orchiectomy or oestrogen treatment in prostatic cancer: effects on serum levels of adrenal androgens and related steroids. Int J Androl 10(4):581–7
- Szawka RE, Rodovalho GV, Monteiro PM, Carrer HF, Anselmo-Franci JA. (2009). Ovarian-steroid modulation of locus coeruleus activity in female rats: involvement in luteinising hormone regulation. J Neuroendocrinol 21(7):629–39
- Toth IE. (1998). Transneuronal labeling of CNS neurons involved in the innervation of the adrenal gland. Horm Metab Res 30:329–33
- Traslaviña GA, Franci CR. (2011). The CRH-R1 receptor mediates luteinizing hormone, prolactin, corticosterone and progesterone secretion induced by restraint stress in estrogen-primed rats. Brain Res 1421:11–19
- Uchoa ET, Sabino HA, Ruginsk SG, Antunes-Rodrigues J, Elias LLK. (2009). Hypophagia induced by glucocorticoid deficiency is associated with an increased activation of satiety-related responses. J Appl Physiol 106(2):596–604
- Valdez SR, Bonafede MM, Carreño NB, Deis RP, Jahn GA. (2012). Lactation defict in OFA hr/hr rats may be caused by differential sensitivity to stress compared with Wistar and Sprague–Dawley rats. Stress 15(4):361–77
- Viau V, Meaney MJ. (1991). Variations in the hypothalamic-pituitary-adrenal response to stress during the estrous cycle in the rat. Endocrinology 129:2503–11
- Welsh TH, Jonhson BH. (1981). Stress-induced alterations in secretion of corticosteroids, progesterone, luteinizing hormone and testosterone in bulls. Endocrinology 109(1):185–90