Abstract
Comparison of exposure to certain predominantly emotional stressors reveals a qualitatively similar neuroendocrine response profile as well as a reduction of physiological responses after daily repeated exposure (adaptation). However, particular physical components of the stressor may interfere with adaptation. As defective adaptation to stress can enhance the probability to develop pathologies, we studied in adult male rats (n = 10/group) swimming behavior (struggling, immobility and mild swim) and physiological responses (ACTH, corticosterone and rectal temperature) to daily repeated exposure to forced swim (20 min, 13 d) at 25 or 36 °C (swim25 or swim36). Rats were repeatedly blood-sampled by tail-nick and hormones measured by radioimmunoassay. Some differences were observed between the two swim temperature groups after the first exposure to forced swim: (a) active behaviors were greater in swim25 than swim36 groups; (b) swim25 but not swim36 caused hypothermia; and (c) swim36 elicited the same ACTH response as swim25, but plasma corticosterone concentration was lower for swim36 at 30 min post-swim. After daily repeated exposure, adaptation in ACTH secretion was observed with swim36 already on day 4, whereas with swim25 adaptation was not observed until day 13 and was of lower magnitude. Nevertheless, after repeated exposure to swim25 a partial protection from hypothermia was observed and the two swim conditions resulted in progressive reduction of active behaviors. Thus, daily repeated swim at 25 °C impairs adaptation of the hypothalamic-pituitary-adrenal axis as compared to swim at 36 °C, supporting the hypothesis that certain physical components of predominantly emotional stressors can interfere with the process of adaptation.
Introduction
Daily repeated exposure to a wide range of stressors has been found to reduce certain physiological responses to an acute challenge with the same (homotypic) stressor while maintaining or increasing the response to novel (heterotypic) stressors. This reduced impact of repeated exposure to the homotypic stressor has been termed adaptation or habituation. The latter term assumes that adaptation to repeated stress follows the rules of habituation (Groves & Thompson, Citation1970), although there is some evidence that the process is likely to be more complex than a simple habituation phenomenon observed in responses such as the orienting reflex (Grissom & Bhatnagar, Citation2009; Martí & Armario, Citation1998).
Regardless of theoretical consideration about the processes involved in adaptation to daily repeated stressors, it is important to identify physiological or biological markers of adaptation. In this regard, the most extensively used marker has been the activation of peripheral variables of the hypothalamic-pituitary-adrenal (HPA) axis, namely plasma concentration of adrenocorticotropic hormone (ACTH) and corticosterone, although there are also seminal studies with plasma concentrations of catecholamines (Kvetnansky et al., Citation1984, Citation2009; Martí & Armario, Citation1998). Stress-induced hyperglycaemia, which basically reflects adrenaline release, is also a good marker of adaptation (Armario et al., Citation1988b, Citation1990). In contrast to ACTH, corticosterone secretion could not be always appropriate as a marker of adaptation to severe stressors when measured only immediately after the stressor, for two main reasons: the prompt saturation of the adrenocortical secretory response with an intermediate concentration of ACTH (Keller-Wood & Dallman, Citation1984), and the increase in maximum adrenocortical responsiveness to ACTH after exposure to chronic severe stressors (Armario et al., Citation1985; Ulrich-Lai et al., Citation2006).
Repeated exposure to stress is also reflected in an overall reduction of brain activation as measured by expression of immediate early genes, particularly c-fos (i.e. Chen & Herbert, Citation1995; Girotti et al., Citation2006; Melia et al., Citation1994; Ons et al., Citation2010). Although these data indicate that adaptation to repeated stress is a phenomenon likely affecting a wide range of responses, there is evidence that different physiological variables show a different pattern of adaptation to daily repeated stress. More particularly, certain variables related to the activation of the sympathetic nervous system (e.g. heart rate) are more resistant to adaptation than responses of the HPA axis and adrenaline secretion (Stamp & Herbert, Citation2001). Moreover, increasing the unpredictability of exposure to the same stressor by daily changing duration and time of day impairs the adaptation of the HPA axis but not of increased glucose mobilization (Martí & Armario, Citation1997).
In general, adaptation of peripheral HPA hormone responses is quite consistently observed after repeated exposure to predominantly emotional stressors (i.e. restraint, immobilization), whereas HPA adaptation failed in response to certain (not all) systemic stressors (Armario, Citation2006). Forced swim (FS) is a stressor of lower intensity than immobilization on wooden boards, when directly compared in terms of HPA activation and changes in food intake and body weight gain (unpublished). However, we previously reported that daily repeated exposure to forced swim in water at 25 °C does not result in a reduction of the ACTH response, despite clear evidence for adaptation in terms of behavior as well as in terms of hypothermia and plasma glucose concentration (Dal-Zotto et al., Citation2000). The stress-induced HPA response has a high metabolic cost and a negative impact on brain homeostasis. Therefore, when the confronted stressor is not actually harmful, as is usually the case with predominantly emotional stressors, it is adaptive for the organism to reduce the HPA response in order to conserve resources and prevent behavioral disorders (Campeau et al., Citation2011; Nesse et al., Citation2007). Hence it is important to characterize factors impairing adaptation of the HPA axis.
We hypothesized that some specific systemic components of certain stressors may interfere with adaptation of the HPA axis. More specifically, we postulated that lack of adaptation of the ACTH response to daily forced swim exposure at 25 °C would be related to the temperature of the water, which results in stress-induced hypothermia. To test this hypothesis, in the present experiment we compared the behavioral and physiological responses to daily repeated forced swim exposure (20 min) in water at 25 °C and 36 °C. Both stressful situations reduced body weight gain to the same extent as compared to controls, indicating no marked differences in intensity between them. Food intake was not controlled in the present experiment because we previously observed no changes in food intake after chronic forced swim at 25 °C (Dal-Zotto et al., Citation2000).
Methods
Animals and general procedure
Male Sprague–Dawley rats obtained from the breeding centre of the Universitat Autònoma de Barcelona were used. They were aged 2–3 months at the beginning of the experiments. The rats were housed in pairs in polypropylene opaque wire-topped cages with a solid bottom (21.5 × 46.5 × 14.5 cm; Type “1000 cm2”, Panlab S.L.U., Barcelona, Spain) containing wood shavings as bedding (Lignocel 3/4, Harlan InterfaunaIbérica, Barcelona, Spain) under standard conditions of temperature (21 ± 1 °C) and in a 12 h:12 h light/dark schedule (lights on at 08:00 h). Food (SAFE-diet A04, Panlab S.L.U., Barcelona, Spain) and water were available ad libitum. The experimental protocol was approved by the Committee of Ethics of the Universitat Autònoma de Barcelona, following the “Principles of laboratory animal care” and was carried out in accordance with the European Communities Council Directive (86/609/EEC).
The experimental treatments were always carried out in the morning, between 08:00 h and 13:00 h. Starting at least two days after their arrival, all rats were handled at least three times on different days for approximately 2 min a day. Immediately after handling, rectal temperature (RT) was quickly measured using a thermistor probe (Cibertec, Madrid, Spain), and blood samples were taken by the tail nick procedure under resting conditions to habituate rats to the procedures. The tail-nick procedure consisted of gently wrapping the rats with a cloth, making a 2 mm incision at the end of one of the tail veins and then massaging the tail while collecting (within 2 min) 300 µl of blood into ice-cold EDTA capillary tubes (Sarsted, Granollers, Spain). This procedure is extensively used in our laboratory and by others because low resting concentration of hormones are obtained under appropriate conditions (Belda et al., Citation2004; Vahl et al., Citation2005). Cage-mates were sampled simultaneously (two experimenters sampled at the same time while a third gently held the two rats). Blood was centrifuged at 4930 × g (15 min, 4 °C), and plasma was frozen (−20 °C) until assay. Rats were assigned at random to the different experimental groups according to their date of birth and body weight. The blood sampling room was different from the vivarium and the stress rooms.
Experimental design
Thirty rats were randomly assigned to three groups (n = 10 per group): Control: never exposed to stress, but handled; chronic forced swim in water at 25 °C (swim25) or at 36 °C (swim36). Rats in the swim groups were exposed daily to 20 min of forced swim stress from day 1 to 13. Immediately after the stress, RT was quickly measured. On days 1, 2, 4 and 13 all rats were blood-sampled after stress (swim) or handling, and 30 min after the end of stress (R30). RT was measured just before blood sampling because of the minor contribution of RT measurements to the already high concentration of hormones after swimming and to precisely obtain swim-induced hypothermia. The rats were weighed 24 h before the first swim exposure and 24 h after the last one.
Forced swim procedure
Rats were placed in transparent Plexiglas cylindrical tanks (height: 40 cm, internal diameter: 19 cm), containing water (25 °C or 36 °C) to a depth of 24 cm. Four identical tanks were used and four rats, separated by opaque screens, were exposed simultaneously. The behavior of the rats, viewed from the front of the tank was recorded on videotape and further measured in the first 5-min period with a stopwatch by one of the team members (C.R), who was unaware of the previous treatment of the rats. Behaviors measured were as follows (Armario et al., Citation1988a): (i) struggling, which was when the rats were diving, climbing or strongly moving all four limbs, or the front limbs were breaking the surface of the water or scratching the walls, and (ii) immobility, which was when the rats remained motionless, keeping their heads out of the water. The remainder of activity was categorized as mild swim, and essentially consisted of swimming around the cylinder while moving all four limbs.
Biochemical analysis
Plasma ACTH and corticosterone concentrations were determined by double-antibody radioimmunoassay (RIA). In brief, the ACTH RIA used 125I-ACTH (PerkinElmer Life Science, Boston, MA) as the tracer, rat synthetic ACTH 1–39 (Sigma, Barcelona, Spain) as the standard and an antibody raised against rat ACTH (rb7) kindly provided by Dr W.C. Engeland (Department of Surgery, University of Minnesota, Minneapolis, MN). The characteristics of the antibody have been described previously (Engeland et al., Citation1989) and we followed a non-equilibrium procedure. The corticosterone RIA used 125I-corticosterone-carboximethyloxime-tyrosine-methylester (ICN-Biolink 2000, Barcelona, Spain), synthetic corticosterone (Sigma, Barcelona, Spain) as the standard and an antibody raised in rabbits against corticosterone–carboximethyloxime-BSA kindly provided by Dr G. Makara (Inst. Exp. Med., Budapest, Hungary). The characteristics of the antibody and the basic RIA procedure have been described previously (Zelena et al., Citation2003). All samples to be statistically compared were run in the same assay to avoid inter-assay variability. The intra-assay coefficient of variation was 3.8% for ACTH and 7.8% for corticosterone. The sensitivity of the assays was 12.5 pg/ml for ACTH and 0.1 μg/dl for corticosterone.
Statistical analysis
The “Statistical Package for Social Science” (SPSS) (SPSS Inc., Chicago, IL) program was used (version 17 for Windows). We used the generalized linear model (GzLM) (McCulloch & Shayle, Citation2001) or generalized linear models with repeated measures (generalized estimating equations, GEE) (Hardin & Hilbe, Citation2003). The generalized linear model is a more flexible statistical tool than the standard general lineal model because several types of distribution of the data could be chosen. Normality distribution and identity as a link function was chosen to be the one that better fitted the data. As a method of estimation, the maximum likelihood (ML) was used in all cases. The significance of the effects was determined by the Wald chi-square statistic.
To study the changes in body weight, a GzLM was used with group as the between-subjects factor (three levels: control, swim25 and swim36), followed by post-hoc sequential Bonferroni comparisons (Holm, Citation1979). To analyze the influence of previous experience with each stressor on the dynamics of the response of systemic HPA hormones (ACTH and corticosterone), a GEE analysis was performed that included one between-subjects factor (group, two levels: swim25 and swim36) and two within-subjects factors: day (four levels: days 1, 2, 4 and 13) and sampling time (two levels: swim, and R30).
The GEE was also performed for swimming behavior (struggling, immobility and mild swim), with one between subjects factor (group, two levels: swim25 and swim36) and one within-subjects factor: day (four levels: days 1, 2, 4 and 13). Finally, because only rats exposed to swim at 25 °C experienced hypothermia, the adaptation of RT was analyzed only for this group, with one within-subjects factor: day (13 levels: 1 to 13).
Results
Body weight gain
As shows, rats repeatedly exposed to swim (n = 10 each group) showed lower body weight gain over the chronic stress period than stress-naive (control rats) [Group: Wald χ2(2) = 39.33, p < 0.001]. Further comparisons showed a similar reduction of body weight gain in the two swim groups as compared to controls (p < 0.05 versus control).
Figure 1. Body weight gain in stress-naive (control) rats and rats chronically exposed to forced swim at 25 °C (swim25) or 36 °C (swim36). A GzLM was used. Group mean +/− s.e.m. (n = 10 for each group) body weight gain over the 13 days of treatment are represented. Groups labelled with different symbols are statistically different (at least p < 0.05 after sequential Bonferroni post-hoc).
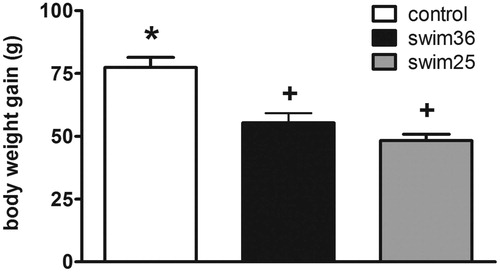
ACTH responses to swimming
Resting ACTH concentrations in control rats were around 50 pg/ml (not shown). When the ACTH responses to swim were analysed (), no effect of group, but significant effects of day, sampling time and the interaction day × group were observed [Wald χ2(3) = 47.7, p < 0.001, Wald χ2(1) =171.6, p < 0.001 and Wald χ2(3) = 13.5, p < 0.01, respectively]. Further comparisons revealed that ACTH concentration in group swim36 was already decreased on day 4 as compared with day 1 (p < 0.01), with a further decline between days 4 and 13 (p < 0.01). In contrast, rats exposed to swim25 did not show reduced ACTH response to FS until day 13 (p < 0.01 compared with day 1), indicating that ACTH adaptation develops later in the rats swimming at 25 °C.
Figure 2. Plasma concentrations of ACTH (A) and corticosterone (B) for rats exposed to forced swim at 36 °C (swim36, black bars) or 25 °C (swim25, grey bars). A GEE model was used. Group mean +/− s.e.m. (n = 10 for each group) are represented. Samples were taken immediately after the stressor (S) and at 30 min post-stress (R30) on days 1, 2, 4 and 13. Horizontal lines indicate average resting concentration of ACTH and corticosterone measured in an additional control group. In all cases, one symbol indicates p < 0.05, two symbols p < 0.01, three symbols p < 0.001; * denotes significant difference with respect to day 1 (ACTH) and to the corresponding sampling time on day 1 (corticosterone); Δ denotes significant difference with respect to the preceding day (ACTH) and to the corresponding sampling time on the preceding day (corticosterone); and # denotes significant differences between the two swim groups (swim 25 and swim36) on the same day and at the same sampling time.
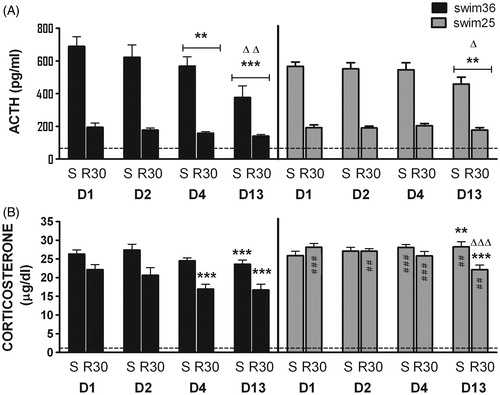
Corticosterone responses to swimming
Resting corticosterone concentrations in control rats were always around 1 µg/dl (not shown). Regarding the corticosterone response to stress (), significant effects of group [Wald χ2(1) = 12.8, p < 0.001], day [Wald χ2(3) = 69.2, p < 0.001], sampling time [Wald χ2(1) = 46.5, p < 0.001] and the interactions day × sampling time [Wald χ2(3) = 28.3, all p < 0.001], day × group [Wald χ2(3) = 10.2, p < 0.05] and sampling time × group [Wald χ2(1) = 17.3, p < 0.001] were observed. Finally, only a trend toward significance was observed for the interaction day × sampling time × group [Wald χ2(3) = 7.09, p = 0.069]. Due to the complexity of the interactions we made only those post-hoc comparisons considered as most relevant to the hypothesis.
When the two groups were compared, higher corticosterone concentration in the post-swim period (R30) was found in rats exposed to swim at 25 °C as compared with 36 °C (p < 0.001), indicating that the lower water temperature impairs post-stress recovery of corticosterone secretion by a mechanism evidently independent of ACTH. Similarly, the corticosterone concentration at R30 was always lower in swim36 than swim25 rats (at least p < 0.01), while between group differences immediately after stress were restricted to days 4 and 13, with higher levels in swim25 than swim36 rats (p < 0.001 and p < 0.01, respectively).
Study of the corticosterone response along the days revealed reduced corticosterone concentrations in swim36 rats at R30 on days 4 and 13 as compared to day 1 (p < 0.001 in the two cases), with no differences between days 4 and 13. Thus, as with ACTH, adaptation of the corticosterone response was already evident on day 4 in swim36 rats. In swim25 rats, corticosterone concentration was higher on day 13 than on day 1 immediately after stress (p < 0.01), whereas it was lower at R30 (p < 0.001).
Behaviors during forced swimming
As shows, struggling behavior was affected by day [Wald χ2(3) = 190.1, p < 0.001], group [Wald χ2(1) = 21.7, p < 0.001] and the interaction day × group [Wald χ2(3) = 20.5, p < 0.001]. Although all rats reduced their struggling behavior after repeated exposure, those exposed to swim at 25 °C struggled more than those swimming at 36 °C on days 1, 2 (both p < 0.001) and 4 (p < 0.01). Time spent immobile in the tank () was affected by day [Wald χ2 (3) = 184.0, p < 0.001], group [Wald χ2(1) = 75.4, p < 0.001] and the interaction group × day [Wald χ2(3) = 9.3, p < 0.05 respectively]. Rats swimming at 36 °C spent more time immobile than those swimming at 25 °C on all days analysed (always p < 0.001) and both groups showed a progressive increase in immobility over the days. Regarding mild swim behavior (), significant effects of day, group and day × group interaction were observed [Wald χ2 (3) = 33.2, p < 0.001, Wald χ2(1) = 62.1, p < 0.001 and Wald χ2(3) = 17.5, p < 0.001 respectively]. Time spent swimming decreased over the days only in the swim36 group and rats swimming at 25 °C showed significantly higher levels of mild swim than the swim36 group on all days.
Figure 3. Effects of chronic swim exposure at 25 °C (solid line) or 36 °C (dotted line) on struggling (A), immobility (B) and mild swim (C) on days 1, 2, 4 and 13. Behavior was only analyzed during the first 5 min of daily exposure, using a GEE model. Data are expressed as group mean +/− s.e.m. (n = 10 each group). Two symbols indicate p < 0.01 and three symbols p < 0.001. * denotes significant difference with respect to day 1 within the same group. Differences between groups are all significant with exception of struggling on days 4 and 13.
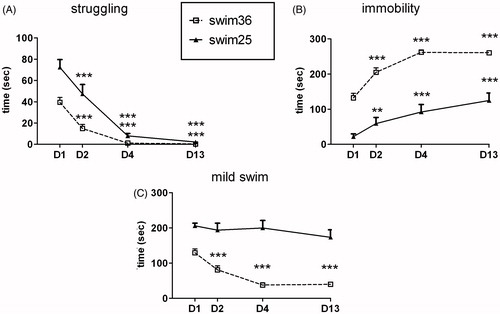
Rectal temperature
The marked reduction in rectal temperature observed in rats exposed to swim at 25 °C showed a partial recovery over the days (day effect: Wald χ2 (11) = 864.8, p < 0.001, ), indicating that rats partially adapted to stress-induced hypothermia after repeated daily exposure to the situation.
Figure 4. Effects of chronic exposure to swim at 25 °C (solid line) on rectal temperature (RT), changes with respect to pre-swim values from day 1 to 13. A GEE was used. Data are expressed as group mean +/− s.e.m. (n = 10 each group). Two symbols indicate p < 0.01, three symbols p < 0.001. * denotes differences in RT versus day 1 (rats swimming at 36 °C are not included in the statistical analysis). The statistical analysis revealed an effect of days, indicating a progressive reduction of hypothermia over the days.
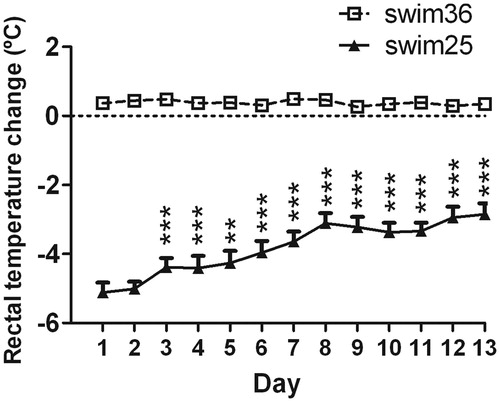
Discussion
We previously reported that daily exposure to forced swim (25 °C) resulted in clear adaptation in terms of behavior, swim-induced hypothermia and plasma glucose concentration (Dal-Zotto et al., Citation2000), but no adaptation at all was observed regarding ACTH secretion. The present study tested the hypothesis that swim-induced hypothermia may interfere with adaptation of the HPA axis. The study showed only small differences in the ACTH and corticosterone secretory responses during the first exposure to forced swim as a function of the temperature of water (25 °C versus 36 °C), but adaptation to daily repeated swim developed faster in rats exposed to 36 °C. Hence, the present results support the hypothesis that certain physical components of a predominantly emotional stressor can modify the HPA response and interfere with the process of adaptation to repeated exposure.
Daily repeated exposure to a wide range of stressors has been repeatedly found to reduce the ACTH and/or corticosterone responses to an acute challenge with the same (homotypic) stressor while maintaining or increasing the response to novel (heterotypic) stressors (Martí & Armario, Citation1998). In general, whereas adaptation of peripheral HPA hormones is quite consistently observed after repeated exposure to predominantly emotional stressors (restraint, immobilization), HPA adaptation failed in response to certain (not all) systemic stressors (Martí & Armario, Citation1998; Rabasa et al., Citation2011a).
Some extensively used laboratory stressors (foot-shock, restraint, immobilization, forced swim), while considered as predominantly emotional on the basis of the pattern of brain activation that they induce (Cullinan et al., Citation1995; Li & Sawchenko, Citation1998; Ons et al., Citation2004; Vallès et al., Citation2006), have also certain physical components (pain, changes in body temperature, muscular activity) that add particular characteristics to each stressor. The extent to which these characteristics are important regarding adaptation to daily repeated exposure is not well-known. We have recently reported a consistent adaptation of the HPA axis and the plasma glucose response after repeated exposure to immobilization on boards but not after repeated sessions of foot-shock, despite the former stressor being stronger than foot-shock on the basis of several physiological changes that consistently reflect the intensity of stressors, such as plasma concentration of ACTH and corticosterone and reduction of food intake and body weight gain (Rabasa et al., Citation2011b). Instead, a slight sensitization of the response was observed, which might be due to the pain from foot-shocks, or perhaps to induction of low-grade inflammatory processes.
Although the study of the influence of water temperature on forced swim behavior was not the main objective of the present work, during the first exposure to forced swim, some differences were observed between the two groups. During the first 5 min of exposure to forced swim, higher levels of struggling and mild swim (and lower levels of immobility) were observed at 25 °C. These data reveal greater overall levels of activity at the lower temperature, in accordance with some previous reports in rats (Abel & Hannigan, Citation1992; Abel, Citation1993; Linhorst et al., Citation2008; Pinter et al., Citation2011). However, there is also evidence in rats (Kelly et al., Citation2011) and particularly in mice (Arai et al., Citation2000; Peeters et al., Citation1992; Taltavull et al., Citation2003) that immobility increases during exposure to low water temperature. The exact reasons for the discrepancies are unclear. Inducing hypothermia prior to exposure of rats to forced swim enhances immobility in the test (Rauch & Lieberman, Citation1990) and hypothermia can depress brain glucose consumption (Taltavull et al., Citation2003) and therefore brain activity, including control of motor function. Discrepancies may arise from the precise temperature of water (affecting the degree of hypothermia) as well as from particular postures of floating that differ between rats and mice and may also depend on the size of the tank (Kelly et al., Citation2011). Interestingly, an opposite relationship between immobility and hypothermia has been reported in two different mouse strains, which has been related to different coping strategies facing aversive situations (Bachli et al., Citation2008). Moreover, water temperature can also influence the response to antidepressants (Bachli et al., Citation2008). Water temperature is a poorly characterized factor that merits further study regarding the use of forced swim to characterize novel putative antidepressant drugs.
Despite differences in activity, the plasma ACTH and corticosterone concentrations immediately after the swim did not differ between the two water temperatures, in accordance with a previous report (Pinter et al., Citation2011). However, at 30 min post-swim differences in ACTH were not noted, although plasma corticosterone concentration was higher in those rats swimming at 25 °C. Thus, temperature of the water affected the post-stress dynamics of plasma corticosterone concentrations, with a delayed post-swim decline at 25 °C as compared to 36 °C. It could be argued that the lack of differences in ACTH concentrations could be due to a ceiling effect of swim on ACTH release. However, this is unlikely as ACTH release observed after forced swim is lower than the maximum observed with other stressors in our conditions (around 1000 pg/ml; Rabasa et al., Citation2011a), and no group differences in ACTH level were observed during the post-swim period. As no differences were observed for ACTH, it appears that temperature of the water may affect corticosterone secretion independently of circulating ACTH. There is the possibility that physiological changes associated with hypothermia may indirectly affect adrenal responsiveness to ACTH, for instance potentiating adrenal responsiveness to ACTH by a direct action on the adrenal or by altering direct sympathetic signals to the gland (Bornstein et al., Citation2008). Alternatively, swim at 25 °C (hypothermia) may reduce corticosterone metabolism. To our knowledge, there is no experimental evidence for either possibility.
As struggling strongly decreased and immobility strongly increased throughout the 20 min daily swim session already on day 1 (not shown), we focused on behavior during the first 5 min of the daily exposure to evaluate adaptation to repeated exposure. A progressive decrease was observed over the days in struggling, and the opposite in immobility, during the first 5 min at both temperatures. However, some differences emerged in mild swim as it was progressively reduced over the days in the swim36 but not the swim25 group. Overall, higher levels of active behavior during swim at 25 °C may reflect the greater aversiveness of water at 25 °C and/or be a way to reduce hypothermia under these conditions. No hypothermia developed in rats swimming at 36 °C, whereas, as expected (Arai et al., Citation2000; Drugan et al., Citation2005; Linhorst et al., Citation2008; Porsolt et al., Citation1979), a marked hypothermia was found at 25 °C. Partial protection from hypothermia was observed after swim exposure for several days, despite some reduction of active behavior, indicating the emergence of physiological protective mechanisms. Both progressive reduction of active behaviors and protection from hypothermia has been previously reported after daily repeated exposure to forced swim at 2 °C (Girardot & Holloway, Citation1985) and 25 °C (Abel & Hannigan, Citation1992; Dal-Zotto et al., Citation2000).
The most interesting results regarding our initial hypothesis was that despite adaptation of the HPA axis after daily exposure to swim at both water temperatures, the rats exposed to forced swim at 25 °C took more days of exposure to reach significant HPA adaptation than those swimming at 36 °C. Thus, reduction of the ACTH response was observed on day 4 in the swim36 group, with an additional reduction from day 4 to 13, while it was only observed on day 13 in the swim25 group. Indeed in the latter rats adaptation of corticosterone secretion was only significant on day 13 and restricted to the post-swim period. These data are not entirely compatible with our previous failure to find adaptation of the ACTH response to repeated forced swim exposure at 25 °C, although a modest adaptation of the corticosterone response was observed (Dal-Zotto et al., Citation2000). Since the magnitude of HPA adaptation may change across experiments even within the same laboratory, it is unclear whether the discrepancies between the present and previous experiment are due to this factor or to minor changes in the procedure. For instance, in the present experiment the rats were only blood-sampled after the swim, whereas in the previous experiment rats were sampled before and after exposure to swim and this may have compromised adaptation. Nevertheless, overall the present and previous results support the hypothesis that certain physical components of stressors can interfere with the process of adaptation that is consistently observed after repeated exposure to emotional or predominantly emotional stressors.
The reason why lower water temperature interferes with adaptation of the HPA axis is evidently not due to difference in intensity of the swim stress at the two temperatures. First, adaptation of the HPA axis after repeated exposure to immobilization, a stressor much more severe than forced swim, is consistently observed in our laboratory (Armario et al., Citation1988b; Márquez et al., Citation2004; Rabasa et al., Citation2011a,Citationb). Second, ACTH concentrations were similar with both swim situations, both immediately after swim and at 30 min post-swim, indicating that they did not markedly differ in intensity. The most plausible explanation for the present results is that the hypothermia induced by forced swimming at 25 °C may interfere with the process of adaptation of the HPA axis. It is noteworthy that in our previous report with forced swim at 25 °C, adaptation of plasma glucose level was observed despite no adaptation of ACTH secretion (Dal-Zotto et al., Citation2000). It thus appears that the hypothermia may interfere specifically with the adaptation of the HPA axis. One possibility is that maintenance of high concentration of glucocorticoids is important to restrain hypothermia. Experiments dealing with a putative role of glucocorticoids in drug or stress-induced hypothermia generally support this possibility (Del Río-García et al., Citation1985; Janocko & Mycek, Citation1986; Vidal et al., Citation1984). Alternatively, glucocorticoids may play no direct role in the control of body temperature, but participate in the development of adaptive changes, including the progressive reduction of hypothermia, triggered by daily exposure to the situation. Finally, hypothermia-induced changes in other hormones may favour the activation of the HPA axis, thus opposing adaptation.
In conclusion, the present results indicate that behavioral and ACTH and corticosterone responses to forced swim are affected by temperature of the water. Hence, adaptation of the HPA axis after repeated exposure was impaired by swim at 25 °C, supporting the hypothesis that specific systemic, physical, components of some laboratory emotional stressors may interfere with HPA adaptation.
Declaration of interest
The authors report no conflicts of interest. This work was supported by grants from the Spanish Ministry of Science and Innovation (SAF2008-01175 and SAF2011-28313), Instituto de Salud Carlos III (RD06/0001/0015, RedesTemáticas de InvestigaciónCooperativa en Salud, Ministerio de Sanidad y Consumo), Plan NacionalsobreDrogas and Generalitat de Catalunya (SGR2009-16). Cristina Rabasa and Raúl Delgado were recipients of a MEC Fellowship.
References
- Abel EL, Hannigan JH. (1992). Effects of chronic forced swimming and exposure to alarm substance: physiological and behavioral consequences. Physiol Behav 52:781–5
- Abel EL. (1993). Physiological correlates of the forced swim test in rats. Physiol Behav 54:309–17
- Arai I, Tsuyuki Y, Shiomoto H, Satoh M, Otomo S. (2000). Decreased body temperature dependent appearance of behavioral despair in the forced swimming test in mice. Pharmacol Res 42:171–6
- Armario A. (2006). The hypothalamic-pituitary-adrenal axis: what can it tell us about stressors? CNS Neurol Disord Drug Targets 5:485–501
- Armario A, Gavaldà A, Martí O. (1988a). Forced swimming test in rats: effect of desipramine administration and the period of exposure to the test on struggling behavior, swimming, immobility and defecation rate. Eur J Phamacol 158:207–12
- Armario A, Hidalgo J, Giralt M. (1988b). Evidence that the pituitary-adrenal axis does not cross-adapt to stressors: comparison to other physiological variables. Neuroendocrinology 47:263–7
- Armario A, Martí J, Gil M. (1990). The serum glucose response to acute stress is sensitive to the intensity of the stressor and to habituation. Psychoneuroendocrinology 15:341–7
- Armario A, Restrepo C, Castellanos JM, Balasch J. (1985). Dissociation between adrenocorticotropin and corticosterone responses to restraint after previous chronic exposure to stress. Life Sci 36:2085–92
- Bachli H, Steiner MA, Habersetzer U, Wotjak CT. (2008). Increased water temperature renders single-housed C57BL/6J mice susceptible to antidepressant treatment in the forced swim test. Behav Brain Res 187:67–71
- Belda X, Márquez C, Armario A. (2004). Long-term effects of a single exposure to stress in adult rats on behavior and hypothalamic–pituitary–adrenal responsiveness: comparison of two outbred rat strains. Behav. Brain Res 154:399–408
- Bornstein SR, Engeland WC, Ehrhart-Bornstein M, Herman JP. (2008). Dissociation of ACTH and glucocorticoids. Trends Endocrinol Metab 19:175–80
- Campeau S, Liberzon I, Morilak D, Ressler K. (2011). Stress modulation of cognitive and affective processes. Stress 14:503–19
- Chen X, Herbert J. (1995). Regional changes in c-fos expression in the basal forebrain and brainstem during adaptation to repeated stress: correlations with cardiovascular, hypothermic and endocrine responses. Neuroscience 64:675–85
- Cullinan WE, Herman JP, Battaglia DF, Akil H, Watson SJ. (1995). Pattern and time course of immediate early gene expression in rat brain following acute stress. Neuroscience 64:477–505
- Dal-Zotto S, Martí O, Armario A. (2000). Influence of single or repeated experience of rats with forced swimming on behavioural and physiological responses to the stressor. Behav Brain Res 114:175–81
- Del Río-García J, Cremades A, Milanes MV, Perez D. (1985). Effects of morphine and their antagonism by dexamethasone on body temperature in restrained and unrestrained guinea-pigs. Pharmacol Res Commun 17:385–94
- Drugan RC, Eren S, Hazi A, Silva J, Christianson JP, Kent S. (2005). Impact of water temperature and stressor controllability on swim stress-induced changes in body temperature, serum corticosterone, and immobility in rats. Pharmacol Biochem Behav 82:397–403
- Engeland WC, Miller P, Gann DS. (1989). Dissociation between changes in plasma bioactive and immunoreactiveadrenocorticotropin after hemorrhage in awake dogs. Endocrinology 124:2978–85
- Girardot MN, Holloway FA. (1985). Naltrexone antagonizes the biobehavioral adaptation to cold water stress in rats. Pharmacol Biochem Behav 22:769–79
- Girotti M, Pace TW, Gaylord RI, Rubin BA, Herman JP, Spencer RL. (2006). Habituation to repeated restraint stress is associated with lack of stress-induced c-fos expression in primary sensory processing areas of the rat brain. Neuroscience 138:1067–81
- Grissom N, Bhatnagar S. (2009). Habituation to repeated stress: get used to it. Neurobiol Learn Mem 92:215–24
- Groves PM, Thompson RF. (1970). Habituation: a dual-process theory. Psychol Rev 77:419–50
- Hardin JW, Hilbe JM. (2003). Generalized estimating equations. Boca Raton: Chapman & Hall/CRC
- Holm S. (1979). A simple sequentially rejective multiple test procedure. Scand Stat Theory Appl 6:65–70
- Janocko L, Mycek MJ. (1986). Prolonged hypothermia after phenobarbital-induced anesthesia in adrenalectomized rats. Pharmacol Biochem Behav 24:1651–8
- Keller-Wood ME, Dallman MF. (1984). Corticosteroid inhibition of ACTH secretion. Endocr Rev 5:1–24
- Kelly KJ, Donner NC, Hale MW, Lowry CA. (2011). Swim stress activates serotonergic and nonserotonergic neurons in specific subdivisions of the rat dorsal raphe nucleus in a temperature-dependent manner. Neuroscience 197:251–68
- Kvetnansky R, Németh S, Vigas M, Oprsalová Z, Jurcovicova J. (1984). Plasma catecholamines in rats during adaptation to intermittent exposure to different stressors. In: Usdin E, Kvetnansky R, Axelrod J, editors. Stress, The role of catecholamines and other neurotransmitters. New York: Gordon and Breach Science Publishers. p 537–62
- Kvetnansky R, Sabban EL, Palkovits M. (2009). Catecholaminergic systems in stress: structural and molecular genetic approaches. Physiol Rev 89:535–606
- Li HY, Sawchenko PE. (1998). Hypothalamic effector neurons and extended circuitries activated in “neurogenic” stress: a comparison of footshock effects exerted acutely, chronically, and in animals with controlled glucocorticoid levels. J Comp Neurol 393:244–66
- Linthorst AC, Flachskamm C, Reul JM. (2008). Water temperature determines neurochemical and behavioural responses to forced swim stress: an in vivo microdialysis and biotelemetry study in rats. Stress 11:88–100
- Márquez C, Nadal R, Armario A. (2004). The hypothalamic-pituitary-adrenal and glucose responses to daily repeated immobilisation stress in rats: individual differences. Neuroscience 123:601–12
- Martí O, Armario A. (1998). Anterior pituitary response to stress: time-related changes and adaptation. Int J Dev Neurosci 16:241–60
- Martí O, Armario A. (1997). Influence of regularity of exposure to chronic stress on the pattern of habituation of pituitary-adrenal hormones, prolactin and glucose. Stress 1:179–89
- McCulloch CE, Shaile RS. (2001). Generalized, linear and mixed models. New York: John Wiley & Sons, INC
- Melia KR, Ryabinin AE, Schroeder R, Bloom FE, Wilson MC. (1994). Induction and habituation of immediate early gene expression in rat brain by acute and repeated restraint stress. J Neurosci 14:5929–38
- Nesse RM, Bhatnagar S, Young EA. (2007). The evolutionary origins and functions of the stress response. In: Fink G, editor. The encyclopedia of stress (2nd ed.). New York: Academic Press. Vol 1, p 965–70
- Ons S, Martí O, Armario A. (2004). Stress-induced activation of the immediate early gene Arc (activity-regulated cytoskeleton-associated protein) is restricted to telencephalic areas in the rat brain: relationship to c-fos mRNA. J Neurochem 89:1111–18
- Ons S, Rotllant D, Marín-Blasco IJ, Armario A. (2010). Immediate early gene response to repeated immobilization: Fos protein and arc mRNA levels appears to be less sensitive than c-fos mRNA to adaptation. Eur J Neurosci 31:2043–52
- Peeters BW, Smets RJ, Broekkamp CL. (1992). The involvement of glucocorticoids in the acquired immobility response is dependent on the water temperature. Physiol Behav 51:127–9
- Pintér O, Domokos A, Mergl Z, Mikics E, Zelena D. (2011). Do stress hormones connect environmental effects with behavior in the torcedswim test? Endocr J 58:395–407
- Porsolt RD, Deniel M, Jalfre M. (1979). Forced swimming in rats: hypothermia, immobility and the effects of imipramine. Eur J Pharmacol 57:431–6
- Rabasa C, Delgado-Morales R, Muñoz-Abellán C, Nadal R, Armario A. (2011a). Adaptation of the hypothalamic-pituitary-adrenal axis and glucose to repeated immobilization or restraint stress is not influenced by associative signals. Behav Brain Res 217:232–9
- Rabasa C, Muñoz-Abellán C, Daviu N, Nadal R, Armario A. (2011b). Repeated exposure to immobilization or two different footshock intensities reveals differential adaptation of the hypothalamic-pituitary-adrenal axis. Physiol Behav 103:125–33
- Rauch TM, Lieberman HR. (1990). Tyrosine pretreatment reverses hypothermia-induced behavioral depression. Brain Res Bull 24:147–50
- Stamp J, Herbert J. (2001). Corticosterone modulates autonomic responses and adaptation of central immediate-early gene expression to repeated restraint stress. Neuroscience 107:465–79
- Taltavull JF, Chefer VI, Shippenberg TS, Kiyatkin EA. (2003). Severe brain hypothermia as a factor underlying behavioral immobility during cold-water forced swim. Brain Res 97:244–7
- Ulrich-Lai YM, Figueiredo HF, Ostrander MM, Choi DC, Engeland WC, Herman JP. (2006). Chronic stress induces adrenal hyperplasia and hypertrophy in a subregion-specific manner. Am J Physiol Endocrinol Metab 291:965–73
- Vahl TP, Ulrich-Lai YM, Ostrander MM, Dolgas CM, Elfers EE, Seeley RJ, D'Alessio DA, Herman JP. (2005). Comparative analysis of ACTH and corticosterone sampling methods in rats. Am J Physiol Endocrinol Metab 289:823–8
- Vallès A, Martí O, Armario A. (2006). Long-term effects of a single exposure to immobilization: a c-fos mRNA study of the response to the homotypic stressor in the rat brain. J Neurobiol 66:591–602
- Vidal C, Suaudeau C, Jacob J. (1984). Regulation of body temperature and nociception induced by non-noxious stress in rat. Brain Res 297:1–10
- Zelena D, Mergl Z, Foldes A, Kovacs KJ, Toth Z, Makara GB. (2003). Role of hypothalamic inputs in maintaining pituitary-adrenal responsiveness in repeated restraint. Am J Physiol Endocrinol Metab 285:1110–17