Abstract
Experiencing stress can be physically and psychologically debilitating to an organism. Women have a higher prevalence of some stress-related mental illnesses, the reasons for which are unknown. These experiments explore differential HPA axis hormone release in male and female rats following acute stress. Female rats had a similar threshold of HPA axis hormone release following low intensity noise stress as male rats. Sex did not affect the acute release, or the return of HPA axis hormones to baseline following moderate intensity noise stress. Sensitive indices of auditory functioning obtained by modulation of the acoustic startle reflex by weak pre-pulses did not reveal any sexual dimorphism. Furthermore, male and female rats exhibited similar c-fos mRNA expression in the brain following noise stress, including several sex-influenced stress-related regions. The HPA axis response to noise stress was not affected by stage of estrous cycle, and ovariectomy significantly increased hormone release. Direct comparison of HPA axis hormone release to two different stressors in the same animals revealed that although female rats exhibit robustly higher HPA axis hormone release after restraint stress, the same effect was not observed following moderate and high intensity loud noise stress. Finally, the differential effect of sex on HPA axis responses to noise and restraint stress cannot readily be explained by differential social cues or general pain processing. These studies suggest the effect of sex on acute stress-induced HPA axis hormone activity is highly dependent on the type of stressor.
Introduction
For many people, the experience of stress can be debilitating, and can have severe negative consequences, including increased susceptibility various psychiatric illnesses. Some of these stress-influenced psychopathologies, such as major depression, generalized anxiety disorder and PTSD, affect approximately twice as many women than men (Bekker & van Mens-Verhulst, Citation2007; Kessler et al., Citation2005; Linzer et al., Citation1996; Olff et al., Citation2007; Stein et al., Citation2000; Tolin & Foa, Citation2006; Vesga-Lopez et al., Citation2008). The hypothalamic-pituitary-adrenal (HPA) axis is one of many systems activated by the experience of physical and psychological threats. Both hypo- and hyper-activity of the HPA axis have been linked to stress-related psychopathologies in humans (Ehlert et al., Citation2001), so understanding how one’s biological sex can affect stress-induced HPA axis activation may inform female-specific vulnerability to certain illnesses. Since rodents and humans share the majority of both central and peripheral components of the HPA axis, the present studies use male and female rats to investigate the effect of sex on the two main hormone regulators of the HPA axis (adrenocorticotropic releasing hormone (ACTH) and corticosterone (CORT)) as well as activation of stress-related brain regions.
Female rats reportedly have markedly different HPA axis activity than male rats. Specifically, female rats exhibit higher basal resting levels of both ACTH and CORT (Critchlow et al., Citation1963; Kitay, Citation1961, Citation1963), as well as significantly higher stress-induced release of HPA axis hormones following a variety of stressors, including foot- or tail-shock (Bland et al., 2005; Heinsbroek et al., Citation1991; Rivier, Citation1991), neonatal handling (Panagiotaropoulous et al., Citation2004), restraint (Aloisi et al., Citation1994; Citation1998; Babb et al., Citation2013; Le Mevel et al., Citation1979; Viau et al., Citation2005), and immune challenge (Seale et al., Citation2004a, Citationb). The present studies use loud noise as a psychological stressor, defined as a stimulus that does not cause physical harm to the organism but which does activate the HPA axis. This stressor has several advantages over the aforementioned stimuli, specifically the commonly used psychological stressor, restraint. First, the neurocircuitry involved in the sensory processing of audiogenic stimuli is well characterized, and has been used previously by our lab to separate neurocircuitry responding to the “stressfulness” of the situation as opposed to the sensory perception of the stimulus (Campeau & Watson Citation1997, 2000; Campeau et al., Citation1997). In addition, the intensity of the noise stimulus (or the loudness, measured in decibels) can be manipulated. Furthermore, we have previously characterized the effect of a single 30-minute audiogenic stressor in male rats demonstrating a graded release of HPA axis hormones in response to increasing intensities of loud noise, and a complementary increase in activation of stress-related neurocircuitry (Burow et al., Citation2005).
The first experiment presented here tested whether female rats exhibit augmented release of HPA axis hormones to very low intensity noise exposure compared to male rats, indicating a lower threshold of stress responsiveness in female compared to male rats. Experiment 2 explored whether females display more prolonged HPA axis hormone release to a higher, but still moderate, intensity noise exposure compared to males, and whether this leads to sex differences in stress-related neurocircuit activation after this stressor (as previously reported following restraint stress (Babb et al., Citation2013)). Because of the unexpected lack of sex differences on HPA axis responses to noise stress in the first 2 experiments, the next experiment tested whether equivalent HPA axis responses after noise stress were due to sex differences in auditory system functioning, assessed by modulation of acoustic startle reflex (Experiment 3). Next the effect of circulating sex steroids on HPA axis hormone release in female rats was tested by comparing HPA axis hormone release to a high intensity loud noise stressor in intact male and female rats, and ovariectomized female rats with or without estrogen replacement (Experiment 4). Experiment 5 directly compared HPA axis hormone release to both restraint and moderate and high intensity noise stress in male and female rats to investigate whether the effect of sex on these measures is dependent on the type of stressor. Restraint and noise exposure differ in two ways that could potentially be the cause of the observed differential effect of sex. Namely, restraint is presented to rats in groups (of both sexes) simultaneously, allowing for the sight, sound, and smell of other rats being stressed, whereas noise is presented in isolated sound-attenuating chambers. Also, restraint typically is preceded by a basal blood sample, whereas noise is not. Therefore it is possible that female rats could be responding more to either of these two factors, leading to the robust effect of sex that is observed after restraint but not noise. Thus, the last experiment (Experiment 6) tested whether the social context of the stressor, or the effect of pain due to blood sampling could influence the effect of sex on HPA axis hormone release to restraint stress. Together, these data suggest that sex differences in stress-induced HPA axis hormone release and brain activation of stress-related neurocircuitry are highly dependent on stressor modality.
Materials and methods
Subjects
Young adult (2-5 m.o.) female and male Sprague-Dawley rats, pair- or group-housed and weighing 200–225 g at the time of arrival were used for all experiments, and were obtained from either Harlan Laboratories (Indianapolis, IN) or Charles River (Portage, MI). All animals were allowed to acclimate to the animal facility for one week prior to any experimental manipulation. To be consistent throughout all experimental manipulations, and because noise exposure requires each animal to be housed separately, all animals were singly housed in clear polycarbonate cages with standard wood shavings bedding and ad libitum access to food (standard rat chow) and water at least 5 days prior to stressor exposure (for both restraint and noise experiments). All colony rooms were maintained on a controlled 12:12 light:dark cycle (lights on at 7am) under constant temperature and humidity. To coincide with the nadir of the diurnal rhythms of both ACTH and CORT, all experimental procedures were performed between 9:00 am and 12:00 pm. Because of the large number of animals used in each study and in order to perform experiments on females in a particular stage of estrous cycle, all studies were performed across several days. Two to eight animals were run on any given day for each experiment, and groups were equally represented across days. Care was taken to always run at least one male each day of an experiment for all experiments. All procedures were reviewed and approved by the Institutional Animal Care and Use Committee of the University of Colorado and conformed to the National Research Council’s (1986) Guide for the Care and Use of Laboratory Animals.
Estrous cycle verification
Prior to any stressor presentation, all female rats were monitored daily for estrous cyclicity via vaginal lavage samples examined with light microscopy. Only females displaying at least two full normal 4–5 days estrous cycles were used in each study. Males in all experiments were handled daily to control for this procedure. On each day of stress presentation, the predicted estrous cycle stage was verified immediately following the stressor. In experiments targeting proestrus specifically, any female not found to be in proestrus was eliminated from statistical analysis.
Stress apparatus
The sound-attenuating acoustic chambers consisted of ventilated double wooden (plywood board) chambers, with the outer chamber lined internally with 2.54 cm insulation (Celotex, Suffolk, England). The internal dimensions of the inner box were 60 cm (w) × 38 cm (d) × 38 cm (h), within which a rat home cage could be placed. The middle of the ceiling of each inner chamber was fitted with a single 6 × 9 inch Optimus speaker (Radio Shack, #12-1769-120 W RMS). Light within the inner chamber was provided by a florescent bulb (15 W) covered with red tissue paper in the upper left corner. Two fans (upper left and lower right corners) allowed fresh air to flow into the inner chamber while also removing stale air. Noise was produced by a General Radio (#1381, Concord, MA) solid-state random noise generator with the bandwidth set at 2 Hz–50 kHz. The output of the noise generator was amplified (Pyramid Studio Pro #PA-600x, Brooklyn, NY) and delivered to each speaker. The speaker characteristics allowed sound delivery between 20 and 27,000 Hz, rolling off quickly (20 dB/octave) at both ends. Noise intensity was measured directly under the speaker on the bottom of the inner chamber with a Sound Level Meter (A scale, #33-2050, Radio Shack). The ambient noise level inside the chamber was approximately 60 dB (background noise level referred to as no noise control level), which was approximately 5 dB above the ambient noise level in the colony rooms due to the noise from the air circulating fans within each chamber. Unless otherwise stated, for each experiment the entire home cage of each animal (singly housed with food and water) was placed into the noise stress apparatus the afternoon prior to noise exposure. Measurement of HPA axis hormones in male rats housed overnight in the apparatus (without any exposure to noise) is similar to basal levels observed in male rats measured in the colony (unpublished observations).
Restraint was conducted in clear ventilated, cylindrical Plexiglas tubes of adjustable length. The size of the tube (23.5 cm in length and 7 cm in diameter for the males; 15.5 cm long and 6.3 cm diameter for the females) restricted movement in all directions with the rat’s tail protruding from the cylinder, but did not interfere with breathing. For experiments using this stressor, animals were transported in their home cages to a room adjacent to where restraint would take place on the afternoon before stressor exposure in order to mimic noise stress procedures.
Acoustic startle testing
Startle testing was performed as the authors have previously described (Masini et al., Citation2008, Citation2012). Briefly, startle testing occurred in eight identical ventilated isolation chambers (Hamilton-Kinder LLC, San Diego, CA), and was illuminated by 8 W bulbs located in the ceiling of the chambers. Each startle chamber contained a rat holder (19.05 × 9.14 × 10.67 cm acrylic cage) held in place on a platform load cell that detects rats’ movements. Startle amplitude was defined as the maximum load cell output (amplified and digitized) during the first 200 ms after the startle stimulus onset. The baseline startle stimuli (pulses) were 50 ms (rise-decay: 1 ms) bursts of white noise at intensities of 105 dBA (sound pressure level, SPL; A scale). Background noise (white noise) of 60 dBA was delivered throughout the startle test. The auditory gap stimuli consisted of a 4, 8, or 16 ms gap in the background white noise (reduction of noise from 60 to 52 dBA, which was the ambient noise intensity in the acoustic chamber) presented 75 ms before the pulse stimuli (GAP trials). Facilitation of the acoustic startle response (pre-pulse facilitation; PPF), was induced with noise increment stimuli consisting of bursts of white noise (rise–decay: 1 ms) at intensities of 62, 64, or 68 dBA, presented 10 ms before the pulse and ending with the presentation of the startle pulses (PPF trials). Pre-pulse inhibition (PPI) of the acoustic startle response was induced with auditory prepulse stimuli consisting of 20 ms bursts of white noise (rise-decay: 1 ms) at intensities of 63, 66, or 70 dB, presented 50 ms before the pulse stimuli (auditory PPI trials). A 400-lux, 20-ms flash of light, provided by arrays of 6 × 20 diodes on each side of the cage (rise–decay: 100 μs), located 3 cm from the cage and extending the entire height of the cage, served as a visual PPI stimulus presented 50 ms before the pulse (Light PPI trials). For the startle test, the rats were placed in the holders, and after a 5 min acclimation period, the first of 20, 105 dBA pulses were presented at a fixed 20 s interstimulus interval (ISI). These initial startle pulses produce some startle habituation, which reduce variability and stabilize baseline startle amplitudes, but they were not used in the estimation of startle modification. Twenty seconds later, the first of 100 additional test trials were presented, with a fixed ISI of 20 s, consisting of pulse alone stimuli (20 trials), intermixed with GAP (10 trials at each gap duration), PPF (10 trials at each prepulse increment intensity level), or PPI trials (10 trials at each prepulse intensity and visual stimulus level). There were 10 occurrences of each trial type in a quasirandom sequence.
Blood collection
For tail blood collection, animals were gently held immobile using a clean towel, and a small incision was made in a lateral tail vein with the corner of a razor blade. Blood was collected using heparinized hematocrit capillaries deposited into ice-chilled 0.5 mL microcentrifuge tubes (for CORT assays), and using uncoated hematocrit capillaries deposited into similar tubes containing EDTA (20 mg/ml) for ACTH assays. This procedure lasted less than 4 min to prevent detection of elevated hormone levels due to the blood collection procedure itself. These blood samples were centrifuged at 14,000 rpm at room temperature for 1 min. After the final stressor exposure, rats were decapitated and trunk blood was collected into large EDTA-coated tubes (Vacutainers). These tubes were then centrifuged at 2000 rpm for 10 min at 4 °C. For all samples, plasma was then pipetted into 0.5 ml Eppendorf microcentrifuge tubes and stored at −80 °C until assayed for hormone concentrations.
Experiment 1: Effect of sex on HPA axis responses to low intensity noise
To test whether sex affects the threshold at which noise stress can activate the HPA axis, male and female (in either diestrus, proestrus, or estrus) rats were exposed to 30 minutes of either 60 (no noise control; male: n = 5; female: n = 26), 75 (male: n = 5; female: n = 19), or 80 (male: n = 5; female: n = 30) dB white noise. These noise intensity levels were chosen based on previous studies using noise in male rats (Burow et al., Citation2005). Immediately following the 30 min noise exposure, animals were sacrificed, trunk blood was collected and plasma concentrations of ACTH and CORT were determined.
Experiment 2: Effect of sex on HPA axis hormone responses to moderate intensity noise and activation of sensory and stress-related brain regions
To determine whether females exhibit a higher acute, and/or more prolonged HPA axis response following the termination of a moderate intensity of noise stress, male and proestrus female rats (n = 7–9/group) were exposed to 90 dB noise (or no noise as controls) for 30 minutes and were sacrificed either immediately following noise exposure (30 min), or 15, 30, or 60 minutes after cessation of the noise (45, 60, and 90 min groups, respectively). This noise intensity consistently produces a significant increase in HPA axis hormones in male rats (Burow et al., Citation2005). In this experiment, only females predicted to be in proestrus were given stressor exposure due to the prior finding that this stage of estrus induces significantly higher HPA axis responses to stress than any other stage (Viau & Meaney, Citation1991). Additional male and proestrus female rats were sacrificed immediately the morning of the experiment to serve as no stress controls. Plasma from all animals was analyzed for concentrations of ACTH and CORT, and brains from no stress controls (0 min time point) and animals sacrificed immediately upon termination of the stressor (30 min time point) were analyzed for c-fos mRNA expression using in situ hybridization. Brain regions analyzed were chosen either due to their involvement in the sensory perception of noise (Burow et al., Citation2005; Campeau & Watson, Citation1997), or because they have previously been reported to be sensitive to a variety of stress situations.
Experiment 3: Effect of sex on auditory system functioning via acoustic startle responses
This experiment tested the failure of female rats to display the expected higher magnitude HPA axis responses is due to sex differences in hearing thresholds. Male (n = 8) and female (n = 13) rats were given acoustic startle testing daily for 5 days in order to have each female tested in each day of the estrous cycle, since both menstrual cycle (Jovaovic et al., Citation2004) and ovarian steroid manipulation in rodents (Charitidi et al., Citation2012) have been documented to affect acoustic startle reflexes. Rats were run in same-sex groups, and the order of testing of these groups was alternated each day. On each day of startle testing, each rat was removed from its home cage and placed into the acoustic startle chamber, and upon cessation of this testing (approximately 120 min daily) were returned to their home cages. The acoustic startle testing included trials for gap detection (which normally inhibits startle response), pre pulse facilitation (PPF) of the acoustic startle response by increment pulses (relatively low intensity pulses immediately preceding the startle pulse), and pre-pulse inhibition (PPI) of the startle response by an auditory or visual pre-pulse. These three different trial types were included on the basis that disruptions of these different prepulse manipulations are linked to different brain regions involved in auditory perception (Bowen et al., Citation2003; Ison et al., Citation1991; Kelly et al., Citation1996), and are routinely used to test auditory threshold shifts (Parham & Willott, Citation1988; Willott et al., Citation1994). The visual PPI trial type was included to serve as a non-auditory cue control. One to two cycles later, the same animals (male and females in proestrus) were singly housed and left in their home cages in noise chambers overnight. The following morning animals were exposed to 30 min of 80 dB noise, immediately following which animals were sacrificed, trunk blood was obtained, and plasma CORT concentrations were determined. Again, proestrus was targeted as the stage of estrous cycle due to this stage likely having the largest magnitude HPA axis responses to stress (Viau & Meaney, Citation1991).
Experiment 4: Effect of sex and ovariectomy with or without estrogen replacement on HPA axis responses to high intensity noise
For this experiment only, male and female rats (Sprague-Dawley strain originally obtained from Harlan Laboratories) were raised in the animal colony at the University of Colorado at Boulder. When they were approximately 3 months of age, females were ovariectomized via bilateral incisions and implanted with silastic capsules as described previously (Strom et al., Citation2008). Briefly, 30 mm long silastic tubing segments (Inner/Outer Diameter: 1.575/3.173 mm, Dow Corning, Fisher Scientific Inc, Waltham, MA) were filled with 70 µl of either sesame oil vehicle (OVX; n = 10) or 17β-estradiol (E2; 180 µg/ml; Sigma) in sesame oil (OVX + E2; n = 10), and were sealed with 5 mm long segments of 2 mm diameter wooden applicator sticks. Other female (intact females; n = 12) and male (intact males; n = 10) rats received sham surgery on the same days: identical bilateral incisions were made into the muscle walls and immediately sutured without removal of any tissues. Animals were singly housed following surgery, and were given one week to recover from surgery prior to further experimental manipulation. All rats were then habituated to being transported down the hallway from the colony room to a behavioral testing suite for 5 consecutive days to reduce HPA axis hormone increases due to this manipulation. Basal blood samples were taken outside the colony room on the second day of this habituation (prior to being transported to the testing suite). The morning after the 5th day of habituation, all rats were brought to the testing suite and remained untouched for 15 min, and were then exposed to 30 min of 105 dB noise stress, immediately after which a blood sample was taken for plasma determination of ACTH and CORT. Intact females were monitored for stage of estrous cycle, but no specific stage was targeted. Forty-eight hours later, all animals were again transported from the colony room to the behavioral testing suite and left untouched for at least 15 min, but were then exposed to 30 min of restraint (these data have been previously published, as well as estradiol concentrations of all animals at baseline and after restraint; see Babb et al., Citation2013).
Experiment 5: Effect of sex on HPA axis responses to restraint and then noise stress in the same male and proestrus female rats
In the previous experiment, one group of sham-operated intact females was compared directly to male rats also receiving sham surgery. However, the estrous cycle of these female sham-operated intact females was not controlled. It is possible that had the proestrus cycle stage been targeted in the intact females in this study, higher HPA axis hormone concentrations to high intensity noise in proestrus female compared to male rats may have been observed. Therefore, this experiment tested whether male (n = 27) and female rats in proestrus (n = 27) would still exhibit similar HPA axis responses to moderate or high intensity noise stress, even after demonstration of a robust sex difference following an initial restraint exposure. On the afternoon before the first stressor (restraint), male and diestrus female rats (singly housed) were transported to a room adjacent to where stress occurred. This room was empty except for these animals, and was identical to the main colony room in terms of light cycle, temperature, and humidity conditions. The following morning, all animals were exposed to 30 minutes of restraint, male and female rats alternated, and all rats staggered by 5 min such that several animals were being restrained on the same bench at the same time. Blood was collected in the same room via tail nicks from all animals just prior to, and immediately after, restraint, as well as 30 minutes after being returned to their home cage after the 30 min blood sampling (0, 30, and 60 min time points, respectively). Following the final tail nick at 60 min, stage of estrous cycle (proestrus) was verified for all the females, and all animals were returned to their home cages and were returned to the animal colony. One estrous cycle later for the females, the same animals (male and female) were exposed to 30 minutes of 80 dB noise, and blood was again collected from a tail vein. Two to three weeks later the same males and females (again in proestrus) were then exposed to 30 minutes of 105 dB noise, and were sacrificed immediately for collection of trunk blood and plasma determination of ACTH and CORT concentrations.
Experiment 6: Influence of social context and blood sampling on the effect of sex on HPA axis responses
To test the hypothesis that female rats display higher HPA axis responses to restraint but not noise due to the social context of the stressor (noise was always presented to rats in isolated sound-attenuating chambers; restraint was always presented in groups where all animals can potentially hear, see, and smell other rats also being stressed), male and female rats on each test day were exposed to either restraint in a group setting or in isolation (n = 7–9/group). Our hypothesis was that female rats may have exhibited higher HPA axis responses to restraint stress than male rats because female rats were responding more to the sight, smell, and/or sound of other rats being stressed around them more than male rats, rather than just to the experience of the restraint itself. This hypothesis would then explain why no effect of sex on HPA axis hormone responses to noise is observed, since this stressor is presented to isolated rats. Treatment conditions were alternated each day. Animals in both treatment conditions were staggered by 5 minutes to allow time for baseline blood collection prior to the start of stress, and 4–6 animals were stressed on each day. On the morning of restraint, a baseline blood sample was taken via tail nick from males and females in proestrus. Animals were then placed in a restraint tube, and then were placed either in one open sound-attenuating chamber normally used for noise stress exposure next to other animals also being restrained, or one animal was placed in one of these chambers, and the doors were closed to isolate each animal inside for the duration of the restraint stress. In this manner the social context of the restraint stressor itself was manipulated; restraint either occurred to one rat in isolation, or to several rats all being restrained in a small space. All animals were restrained for 30 min each, and blood was collected immediately following the end of the restraint via tail nick, and the animal was returned to its home cage. The home cages of the group stressed animals were left in the same room as restraint occurred, and all blood collections were also performed in this room, so animals being stressed in groups were exposed not only to the sounds, smells, etc from other rats also being stressed, but also of other rats having blood samples collected. The home cages of the animals stressed in isolation were in a separate adjacent room, and all blood collections were done in a third room, so none of these animals were exposed to the cues of other animals being stressed. A final blood sample was taken again via tail nick 30 minutes after returning the animals to their home cages. After the final blood sampling, stage of estrous cycle was verified in the females and males were handled. Then all animals were returned to the main colony.
The animals remained in the main colony experimentally untouched for ∼2 weeks. Then to test whether female rats display higher magnitude HPA axis responses to restraint stress due to the initial baseline blood sample that is typically taken prior to presentation of this stress, the same males and females (again in proestrus) were placed in restraint tubes for 30 minutes (without basal blood sampling) and a single blood collection via tail nick was taken either immediately after the cessation of the stressor, or 30 minutes after returning to their home cage (n = 7–9/group). All animals were restrained in a group setting, and all animals remained in the restraint room until all the tail nicks were done for each day. The animals were then returned to the main animal colony. Blood samples from both experiments were then analyzed for plasma ACTH and CORT concentrations.
ACTH IRMA
Plasma ACTH concentrations were assayed using a commercially available immunoradiometric assay kit (Cat # 27130; DiaSorin, Stillwater, MN); which has a sensitivity of 1.5 pg/ml, and inter- and intra-assay coefficients of ∼4.6% and ∼4.18%, respectively. This IRMA kit recognizes 100% of whole molecule ACTH (detects all 39 amino acids), and as such is very specific, with undetectable crossreactivity for α- and β-MSH, as well as β-Endorphin. The assay was performed according to the kit directions. Briefly, 200 µl of plasma or plasma diluted with zero standard (provided with the kit) was incubated overnight with a 125I-labelled monoclonal antibody specific for ACTH amino acids 1–17, a goat polyclonal antibody specific for ACTH amino acids 26–39, and a polystyrene bead coated with a mouse anti-goat IgG. Only ACTH in the sample with all 39 amino acids bound both antibodies to form an antibody complex on the polystyrene bead. The following day, tubes containing the radioactive beads were washed to remove any unbound reagents and the amount of radioactivity still left on each bead was determined with a gamma counter. The concentration of ACTH for each sample was calculated by fitting values for each sample into a standard curve of known ACTH dilutions processed concurrently.
CORT ELISA
Plasma CORT concentrations were analyzed using a commercially available kit (Cat. # 901-097; Assay Designs, Ann Arbor, MI). This is a highly specific assay (100% cross-reactivity with CORT, 28.6% with deoxycorticosterone, and 1.7% with progesterone), with a sensitivity of 26.99 pg/ml, and inter-/intra-assay variability of ∼ 7.5% and ∼ 9.7%, respectively. The kit directions were followed with the exception of a modification to use a smaller volume of plasma as follows. The steroid displacement reagent (0.5 µl/ml; provided with the kit) was added to the assay buffer. Ten milliliters of plasma was then diluted 1:50 with the amended assay buffer. The diluted plasma samples were then processed according to the kit directions. This method was found to result in assayed CORT levels equivalent to the method in the kit directions (data not shown). Briefly, plasma samples and diluted CORT standards were incubated in a 96-well plate coated with anti-sheep antibody raised in donkey, together with CORT with a covalently attached alkaline phosphatase molecule, and a sheep polyclonal antibody for CORT. After a 2 h incubation period the plate was washed to remove all unbound reagents and a substrate was added. After 1 hr the color reaction was stopped and the intensity of color in each well was analyzed at 405 nm using a microplate reader (Biotek EL808; Winooski, VT). The concentration of CORT for each sample was calculated by fitting unknown values into a standard curve of known CORT dilutions processed concurrently.
In situ hybridization
The method for in situ hybridization has been described previously (Day et al., Citation2005). Briefly, whole brains stored at −80 °C were mounted on chucks at −20 °C. Ten-micrometer thick sections were cut on a cryostat (Model 1850; Leica Microsystems, Buffalo Grove, IL), thaw-mounted onto polylysine-coated slides and stored at −80 °C. Slides were taken from storage and immediately fixed in cold 4% paraformaldehyde for 1 hr, acetylated in 0.1 M triethanolamine (pH 8.0) with 0.25% acetic anhydride for 10 min, dehydrated through graded ethanols to 100% and air dried. A riboprobe against c-fos mRNA (680 bp; courtesy of Dr. T. Curran, St. Jude Children’s Hospital, Memphis, TN) was generated using standard transcriptional methods and labeled with 35S-UTP (Perkin Elmer, Waltham, MA). Brain sections were hybridized overnight at 55 °C with the riboprobe diluted to 1–3 × 106 cpm per 70 µl in hybridization buffer containing 50% formamide, 10% dextran sulfate, 3× saline sodium citrate, 50 mM sodium phosphate (pH 7.4), 1× Denhardt’s solution, 0.1 mg/ml yeast tRNA, and 10 mM dithiothreitol. The following day, the probe was washed from the slides, and they were then treated with RNase A (200 µg/ml; pH 8.0) at 37 °C for 1 h, washed to a final stringency of 0.1× saline sodium citrate for 1 h at 65 °C, dehydrated again in graded ethanols to 100%, and air dried. Slides were then exposed to X-ray film (BioMax-MR; Eastman Kodak, Rochester, NY) for 7–12 days. Test films were included with slides run concurrently and were used to optimize the exposure time of the films containing experimental slides. Films were then analyzed as described below.
Levels of c-fos mRNA were analyzed by computer-assisted optical densitometry by an experimenter blind to the treatment conditions. Images of each individual brain section were captured digitally (CCD camera, model XC-77; Sony, Toyko, Japan), and digital images were then analyzed using Scion Image (Version 4.03 for Windows; Scion Corp., Frederick, MD). Prior to analysis, signal for all groups was verified to be within the linear range, with all pixels being contained within 30–200 (of a 1–250 max range) in order to insure that hybridization was not at saturating levels. First, the relative optical density of the x-ray film was determined using a macro within Scion Image (written by Dr S. Campeau) which allowed the automatic determination of a signal above background. Specifically, for each section, a background sample was taken over an area of white matter, and the signal threshold was set as 3.5 standard deviations above the mean gray value of the background. The remaining pixels above this threshold were then analyzed within the brain region of interest. It should be noted that although background criteria were relatively stringent, this method still results in a few pixels above background in areas on the x-ray film not on the brain section, indicating that even weak intensity mRNA signal on the tissue is detected. For consistency, a different template was created for each brain region, and was placed using anatomical landmarks based on the white matter distribution of the unstained tissue, according to a standard rat brain atlas (Paxinos & Watson, Citation2005). The number of pixels above background was multiplied by the signal above background to give an integrated density value for both hemispheres throughout the rostral-caudal extent of each brain region of interest. The mean integrated density for each animal was then calculated by averaging the highest 2 or 3 values for each hemisphere depending on the brain region resulting in a single value for each animal representing the peak of c-fos mRNA expression for each brain region of interest. These raw values were used for statistical analysis. Standardized scores (z scores) were then calculated for each brain region to allow for the display of many brain regions with very different patterns of mRNA expression on the same graph. As we have done previously (Campeau et al., Citation2010; Campeau & Watson, Citation1997), and for presentation purposes only, the mean standard (z) score was calculated as: (group mean – “population” mean) / “population” SD, with the “population” referring to all animals in the experiment. By definition then, all standard deviations for mean standard scores = 1 (and are not shown).
Statistical analysis
All hormone data were analyzed with appropriate one-, two-, or three-way ANOVAs (and with repeated measures when appropriate), with significance set at p = 0.05. Significant main effects in repeated measures ANOVAs were explored further with one-way ANOVAs. Further post-hoc analyses, when necessary, were performed using independent samples t-tests. Raw values of c-fos mRNA expression data were analyzed using a two-way ANOVA with stress and sex as the independent variables (Experiment 2), and statistical significance for these tests was set at p < 0.01 to reduce the possibility of Type I error. For presentation purposes only, these raw data were transformed into standardized (z) scores and a constant (1.0) was added to make all values positive (). SPSS Statistics (version 18 for Windows, SPSS Inc., Chicago, IL) was used for all statistical analyses.
Results
Experiment 1: Effect of sex on HPA axis responses to low intensity noise
demonstrates the effect of noise at low intensities and sex on HPA axis hormone secretion. There was no effect of stage of estrous cycle on either ACTH or CORT secretion at any of the intensities analyzed (p’s > 0.05), so the female data were pooled for further analysis. Noise induced a significant activation of the HPA axis in all animals overall, as seen in a two-way ANOVA by a main effect of noise intensity on both ACTH (F2,89 = 6.57, p < 0.01; ) and CORT (F2,89 = 7.80, p < 0.01; ) concentrations. Post hoc analyses revealed that ACTH concentrations after 80 dB noise were significantly higher than at 60 dB (controls) (p’s < 0.05), and that CORT concentrations after 80 dB noise were significantly higher than at 60 and 75 dB (p’s < 0.05). Overall, males and females did not show different thresholds of noise intensity to activate the HPA axis. Although there was no main effect of sex on either ACTH (F1,89 = 3.39, p = 0.069) or CORT (F1,89 = 0.85, p = 0.36), specific analysis of controls demonstrated that female rats had higher basal levels of ACTH (t22 = 2.07, p < 0.05), and a trend towards significantly higher CORT levels (t22 = 1.71, p = 0.06) at 60 dB (no noise controls) compared to male rats. There was not a significant interaction between sex and noise intensity (p > 0.05).
Figure 1. The effect of sex on ACTH (A) and CORT (B) concentrations at baseline (60 dB) and after 30 min of low-intensity noise stress (75 and 80 dB). All values are displayed as means ± 1 SEM. *: p < 0.05 compared to male rats at the same intensity. #: p < 0.05 compared to baseline (60 dB), regardless of sex. ^: p < 0.05 compared to 75 dB noise, regardless of sex.
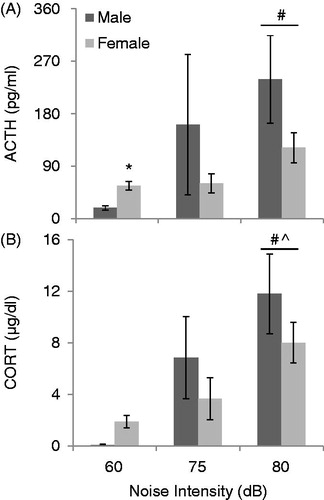
Experiment 2: Effect of sex on HPA axis hormone responses to moderate intensity noise and activation of sensory and stress-related brain regions
shows the effect of sex on ACTH responses to noise across time. Thirty minutes of 90 dB noise resulted in a significant increase in ACTH concentration overall in both males and proestrus females, as seen by a significant main effect of time on ACTH concentration (F4,71 = 17.119, p < 0.001). Post-hoc tests revealed that ACTH levels were significantly higher at 30 min than at all other time points (p < 0.001), and that by 45 min ACTH levels were back down near baseline levels for both sexes (p’s > 0.05). No other time points for ACTH concentrations differed significantly from each other. Overall, there was no main effect of sex on ACTH secretion. However, there was a sex by time interaction (F4,71 = 2.594, p = 0.044), and post hoc tests revealed that this was due to male rats having a higher concentration of ACTH at 30 minutes as compared to female rats (p < 0.05).
Figure 2. The effect of sex on the recovery from HPA activation in response to 30 min of 90 dB noise (black bar). All values are displayed as means ± 1 SEM. *: p < 0.05 compared to males at that time point. #: p < 0.05 compared to baseline (0 min) regardless of sex. (A) ACTH levels increased significantly in all animals after 30 min, and were back down to baseline levels by 45 minutes for both sexes. Post-hoc tests for a significant sex by time interaction revealed that males had significantly higher levels of ACTH than females at 30 min (p < 0.05). (B) CORT levels increased significantly for all animals by 30 minutes, and remained elevated until 60 minutes for both sexes. In addition, females had significantly higher baseline levels of CORT than males (p < 0.05).
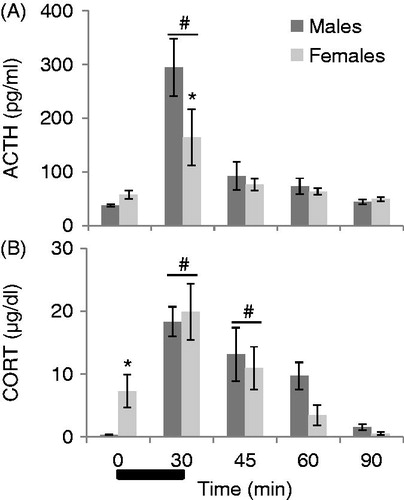
shows the effect of sex on CORT responses to 30 minutes of 90 dB white noise. Overall, noise also significantly increased CORT concentrations regardless of sex (F1, 71 = 14.433, p < 0.001). Post hoc tests revealed that CORT concentrations at 30 minutes were significantly higher than all other time points except for 45 minutes (p’s < 0.05). At 45 minutes, CORT levels were still significantly higher than at 90 minutes and baseline (p’s < 0.05). By 60 minutes, CORT concentrations were not significantly different from baseline for both sexes (p’s > 0.05). There was no main effect of sex, however females had significantly higher CORT levels at baseline as compared to males (t13 = 2.627, p < 0.05). There was no sex by time interaction on CORT secretion. The effect of sex on basal (from 0 min time point) and noise stress-induced (from 30 min time point) c-fos mRNA expression is displayed in (raw values) and (z scores + 1.0). Noise significantly increased c-fos mRNA expression in the brains of all animals (p’s < 0.01). However, there was no main effect of sex in any brain region analyzed at either 0 or 30 min of noise stress, as well as no significant sex by stress interactions (p’s > 0.01; see and ).
Table 1. Mean integrated densities/100 (1 SEM/100) of c-fos mRNA expression following 30 min of 90 dB noise stress.
Experiment 3: Effect of sex on auditory system functioning via acoustic startle responses
The effect of sex on various types of pre-pulse manipulations of the startle response (GAP detection, PPF, or PPI), baseline startle amplitude, and CORT response to 30 min of 80 dB noise is displayed in . Each female was in each of the 4 stages of the estrous cycle at least once throughout the 5 day testing period. Analyzing data from each day of the estrous cycle once from each female revealed no main effect of the estrous cycle on baseline startle amplitude, or any of the pre-pulse manipulations measured (p’s > 0.05; data not shown), so further data analysis compared male to female data regardless of the stage of the estrous cycle. Data from male and female rats were then collapsed across days of testing, so that each data point represents the average response for that stimulus type across the 5 days. Although there was no significant effect of sex on any of the pre-pulse manipulations examined (p’s > 0.05; ), female rats exhibited a significantly higher baseline startle amplitude compared to male rats (t19 = 2.09, p = 0.03; ). However, the same male and female rats (in their next proestrus stage) did not differ in their CORT response to 30 min of 80 dB noise (t18 = 2.1, p > 0.05; ).
Figure 3. The effect of sex on relative c-fos mRNA expression at baseline and following 30 min of 90 dB noise in stress-related (PL, IL, LS, BSTav, MPOA, DMH, PVN, MeA, BLA) and auditory processing (Aud Ctx, MGN, IC, VCA) brain regions. Data are expressed as mean standard (z) scores +1.0 (see “In situ hybridization” section for more information). Raw values for these data are displayed in . There was no effect of sex on either basal or stress-induced c-fos expression in any brain region examined. Abbreviations: PL: prelimbic cortex; IL: infralimbic cortex; LS: lateral septum; BSTav: bed nucleus of the stria terminalis, anteroventral subdivision; MPOA: medial preoptic area; DMH: dorsomedial nucleus of the hypothalamus; PVN: paraventricular nucleus of the hypothalamus; MeA: medial nucleus of the amygdala; BLA: basolateral nucleus of the amygdala; Aud Ctx: auditory cortex; MGN: medial geniculate nucleus of the thalamus; IC: inferior colliculus; VCA: ventral cochlear nucleus.
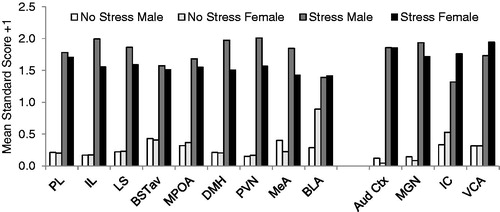
Figure 4. The effect of sex on pre-pulse suppression (GAP and PPI) and facilitation (PPF) of the acoustic startle response. All values are displayed as means ± 1 SEM. (A) No effect of sex was observed on any manipulation of the acoustic startle response (p’s > 0.05). Values represent means of the average for each animal across 5 days of testing. (B) Baseline acoustic startle amplitude (corrected for body weight (BW)) is significantly higher in female rats. Values also represent means of each animals’ average across the 5 test days. *: p < 0.05 compared to male rats. (C) No effect of sex on CORT concentration in response to 30 min of 80 dB noise was observed in the same animals.
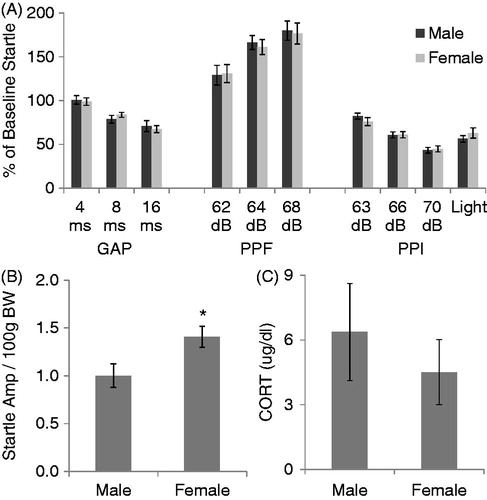
Experiment 4: Effect of sex and ovariectomy with or without estrogen replacement on HPA axis responses to high intensity noise
The effect of sex and ovariectomy with or without E2 replacement on HPA axis responses to noise stress are displayed in . Estradiol levels at both basal and stress timepoints in all animals is published elsewhere (Babb et al., Citation2013). At baseline, there was no main effect of treatment group on ACTH concentrations (F3,38 = 2.40, p = 0.08; ). However, following 30 min of noise stress, there was a significant effect of treatment group (F3,38 = 5.39, p = 0.003). Specifically, males and OVX females had significantly higher ACTH concentrations than intact females (Sham) and OVX + E2 females (p’s < 0.05). Male and OVX female ACTH concentrations did not differ significantly, nor did intact female and OVX + E2 ACTH concentrations. There was a significant main effect of treatment group on basal (F3,38 = 3.34, p = 0.03) and stress-induced (F3,38 = 4.18, p = 0.01) CORT concentrations (). Specifically, intact females and OVX + E2 females had significantly higher basal CORT concentrations than males, and OVX + E2 females were also significantly higher than OVX females (p’s < 0.05). Male and OVX females, as well as intact and OVX females CORT concentrations did not differ significantly (p’s > 0.05) before stress. After noise stress, males had significantly higher CORT concentrations than intact and OVX + E2 females, and OVX females had significantly higher CORT concentrations than OVX + E2 females (p’s < 0.05). No other groups’ CORT concentrations differed significantly following stress (p’s > 0.05).
Figure 5. The effects of sex (Intact male and intact female) and ovariectomy with (OVX + E2) or without (OVX) estradiol replacement on HPA axis responses to 30 min of 105 dB noise are displayed in . Intact females were monitored for estrous cycle, but were not given stress while in a particular stage. All values are displayed as means ± 1 SEM. *: p < 0.05 compared to both intact male and OVX female rats. #: p < 0.05 compared to intact male rats. (A) ACTH concentrations before (Basal) and after noise stress. (B) CORT concentrations before (Basal) and after noise stress.
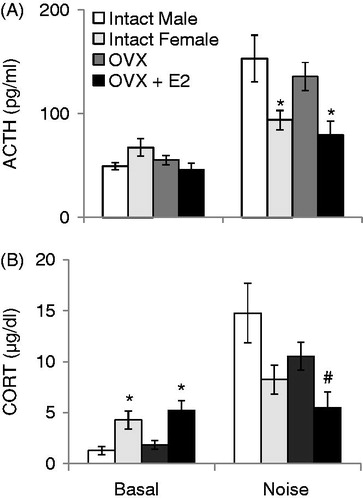
Experiment 5: Effect of sex on HPA axis responses to restraint and noise stress in the same male and proestrus female rats
The effect of sex on plasma ACTH and CORT concentrations in response to two different acute stressors in the same animals are displayed in . Restraint stress significantly increased ACTH concentrations in all animals, as demonstrated by a main effect of time (F2,104 = 147.89, p < 0.001; ). In addition, females had significantly higher ACTH compared to males overall (F1,52 = 35.68, p < 0.001). There was also a significant interaction between time and sex (F2,104 = 14.35, p < 0.001); females had a significantly greater increase in ACTH following restraint despite having significantly higher basal ACTH levels. Area under the curve analysis for ACTH concentrations overall confirmed that female ACTH concentrations were significantly higher than in males (t52 = 5.88, p < 0.001). Basal and restraint-stress-induced plasma CORT concentrations are displayed in . Restraint significantly increased CORT concentrations in all animals (F2,104 = 155.63, p < 0.001). Overall, females also had significantly higher CORT concentrations than males (F1,52 = 40.43, p < 0.001). In addition, there was a statistically significant interaction between sex and time (F2,104 = 15.37, p < 0.001). Restraint led to a significantly greater increase of CORT concentrations at 30 and 60 minutes in female rats despite having already elevated baseline concentrations compared to male rats (p’s < 0.001). Area under the curve analysis for CORT concentrations overall confirmed that female CORT concentrations were significantly higher than in males (t52 = 6.29, p < 0.001).
Figure 6. The effect of sex and type of stress on HPA axis responses in the same male and proestrus female rats (given restraint, then 80 dB noise, then 105 dB noise) is displayed in . Black bar represents 30 min of restraint stress. *: p < 0.05 compared to male rats at the same time point. (A) Restraint significantly increased ACTH concentrations in all animals, and female rats had significantly higher basal and stress-induced ACTH levels compared to males. (B) Restraint also increased CORT concentrations significantly. Females had significantly higher CORT levels at every time point compared to males. (C) There were no significant differences in ACTH levels due to either sex or noise intensity. (D) No effect of sex or noise intensity on CORT concentrations was observed.
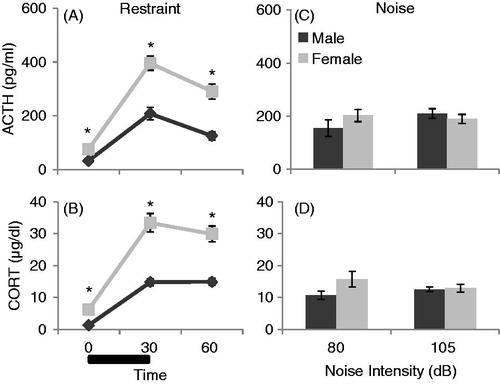
The effect of sex on HPA axis responses to moderate (80 dB) and high (105 dB) intensity noise stress in the same animals is displayed in . There was no significant effect of sex on ACTH concentration following either 80 dB (t50 = 1.30, p = 0.20) or 105 dB (t50 = 0.69, p = 0.49) noise, as demonstrated in . In addition, there was no significant effect of sex on CORT concentration after either 80 dB (t50 = 1.83, p = 0.07) or 105 dB (t50 = 0.26, p = 0.80) noise, as displayed in .
Experiment 6: Influence of social context and prior blood sampling on the effect of sex on HPA axis responses
displays HPA axis responses to 30 min of restraint given in close proximity with other rats being restrained simultaneously (Group Stressed) or given in isolation (Isolation Stressed) in male and female rats. Plasma ACTH and CORT concentrations were analyzed initially with a 3-way repeated measures design with time (0, 30, and 60 min), sex (Male and Female), and social context (Group or Isolation) as the independent variables. Restraint significantly increased ACTH concentrations in all animals, demonstrated by a main effect of time (F2,56 = 85.73, p < 0.001; ). Importantly, exposing females to restraint stress in isolation did not eliminate the sex difference seen in HPA axis responses. Specifically, females had significantly higher levels of ACTH overall, as demonstrated by a main effect of sex (F1,28 = 23.90, p < 0.001). There was also a trend for a time by sex interaction (F2,56 = 3.00, p = 0.058), and tests of within-subjects contrasts showed a significant linear fit for this interaction (F1,28 = 4.44, p = 0.044), meaning that females had a trend for a larger increase in ACTH concentrations after stress (30 and 60 min; see ). A further analysis of the change in ACTH concentrations from 0 to 30 min revealed a significant main effect of sex (F1,31 = 5.12, p = 0.03), demonstrating that females had a significantly sharper rise in ACTH concentrations between these time points compared to males, regardless of the social context of the stressor (Group versus Isolated). Although there was no main effect of social context (F1,28 = 2.53, p = 0.14), there was a trend for a time by social context interaction (F2,56 = 3.06, p = 0.055), where animals stressed in isolation, regardless of sex, tended to have higher, rather than lower, ACTH concentrations at 30 min. There was no significant two-way interaction between sex and social context and no significant three-way interaction between time, sex and social context of stress on ACTH concentrations ().
Figure 7. The effects of sex (male and females in proestrus) and social context of stress (restraint given in Groups versus in Isolation) on CORT concentrations over time (0, 30, and 60 min) are displayed in . Restraint stress for 30 min (black bar) was administered immediately after basal blood sampling performed at 0 min. (A) ACTH concentrations overall were significantly affected by stress exposure (p < 0.001) and sex (p < 0.001), but not social context (p = 0.14). Restraint stress significantly increased ACTH concentrations overall, although to a greater extent in females compared to males. (B) CORT concentrations overall were also significantly affected by stress exposure (p < 0.001) and by sex (p = 0.001), but not social context (p = 0.82). Restraint stress significantly increased CORT concentrations, and female rats had significantly higher CORT concentrations compared to males. There was also a significant time by social context interaction, where animals stressed in isolation had a significantly more prolonged response to restraint stress than animals stressed in groups, regardless of sex (p = 0.002).
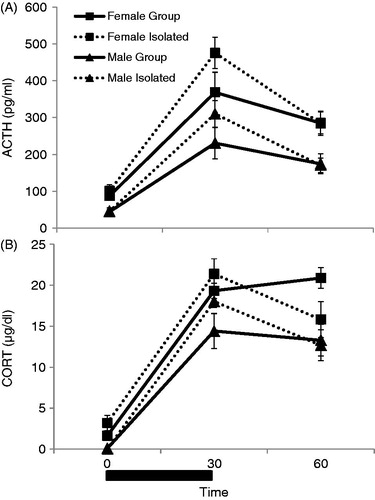
A three-way repeated measures ANOVA also revealed a main effect of time (F2,27 = 239.88, p < 0.001) and of sex (F1,28 = 13.93, p = 0.001) on plasma CORT concentrations (). Restraint significantly increased CORT concentration in all animals, and overall female rats had significantly higher CORT concentrations than male rats. Although there was no main effect of social context of the stressor on CORT concentrations (F1,28 = 0.06, p = 0.82), there was a significant time by social context interaction on CORT concentration (F2,56 = 5.86, p = 0.005). Post-hoc tests revealed that this interaction was due to animals restrained in isolation having a more prolonged CORT response to stress (F1,31 = 11.75, p = 0.002). There was not a significant time by sex interaction (p = 0.20) or time by sex by social context interaction (p = 0.20) on CORT concentrations, meaning that the overall effect of sex did not differ depending on either the time point, or the social context of the stressor (restrained in close proximity or in isolation).
shows HPA axis responses to restraint with a single blood sample taken at either 30 minutes (immediately after the stressor) or at 60 minutes (30 minutes after animals were returned to their home cages). Overall, eliminating a baseline blood sample prior to restraint did not eliminate the sex difference in HPA axis activation previously seen after the experience of this stressor. Thirty minutes of restraint followed by a single tail nick resulted in females having larger HPA axis responses as compared to males at both time points. Overall, females had statistically higher ACTH (F1,25 = 6.221, p < 0.05) and CORT (F1,25 = 11.339, p < 0.01) concentrations regardless of the time point. Furthermore, overall all animals had significantly lower ACTH (F1,25 = 10.128, p < 0.01) and CORT (F1,25 = 10.189, p < 0.01) concentrations at 60 minutes as compared to 30 minutes regardless of sex. There was no significant sex by time interaction for either hormone.
Figure 8. The effect of sex (males compared to females in proestrus) on HPA Axis responses to 30 minutes of restraint without experience of a basal blood sample is displayed in . *: p < 0.05 compared to female rats at the same time. #: p < 0.05 compared to 30 min time point. (A) Females had significantly higher ACTH concentrations than males in response to 30 minutes of restraint when one blood sample was taken either immediately after (30 min), or 30 min after being returned to the home cage (60 min). All animals overall had significantly lower ACTH levels when blood samples were taken at 60 min compared to animals that had a blood sample taken immediately following restraint (30 min). (B) Females also had significantly higher CORT concentrations than males at both time points, and all animals had significantly lower CORT levels when tested at 60 minutes than animals sampled at 30 min.
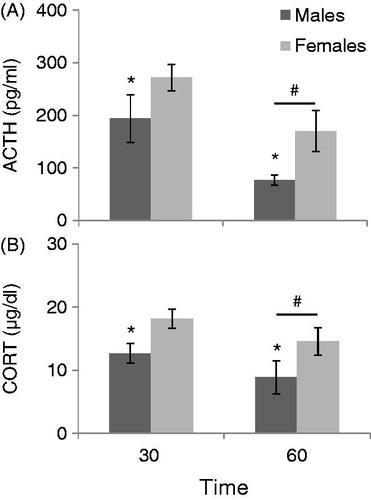
Discussion
The experiments presented here investigated HPA axis hormone profiles before and after two different acute stressors in male and female Sprague-Dawley rats. Taken together, it appears that the effect of sex on HPA axis hormone activation is stressor-specific. The data presented here using loud noise as a stressor not only conflicted with our predictions, but also conflict with previous literature demonstrating that females had significantly higher concentration of CORT following just 10 minutes of loud noise (Seale et al., Citation2004a). It is possible that a sex difference in HPA axis activation after noise stress exists at a time point earlier than 30 min, since this is the earliest time point after initiation of a stressor observed in these studies. However, for both stressors, we consistently observed that females have higher baseline concentrations of the stress hormones ACTH and CORT, which is an effect that has been noted for approximately 50 years (Kitay, Citation1961). Therefore, it is possible that the previously reported sex difference following such a short duration of noise stress (Seale et al., Citation2004a) simply reflects initial basal sex differences in HPA axis hormones, and not stress-induced sex differences in HPA axis hormone increases, per se. Indeed, even when very low intensity noise stimuli are used, female rats do not appear to display higher HPA axis hormone release than male rats, and if anything male rats may have a higher fold increase in response to these low intensity stressors than female rats. Although the males in this experiment appear to have drastically more variability in HPA axis responses to these low intensity noise stressors, the small sample size of the males compared to the females (three groups of females in three stages of estrous cycle collapsed into one group for analysis) likely caused the small variation in the female group. Regardless, what is clear is that compared to the animals receiving no noise (60 dB), females do not appear to be more sensitive to low intensity noise stress than males. Furthermore, this pattern appears to be supported when rats are given a more moderate intensity noise stressor. Importantly, comparison of both acute restraint and noise stress in the same male and female rats revealed a robust effect of sex on HPA axis hormone concentrations following restraint stress, but no such effect following noise stress. It is important to point out that in this experiment larger magnitude HPA axis hormone concentrations were expected to be observed following 105 dB compared to 80 dB noise. The lack of increase in these hormones to 105 dB noise compared to 80 dB noise likely reflects some adaptation, or habituation of the stress response, since 105 dB was the second noise stressor exposure (and third stressor exposure overall) in these same animals over the course of several weeks. However, when intact male and female rats (following sham surgery) were exposed to an initial 105 dB noise exposure without prior restraint exposure, the opposite effect of sex was observed than was expected, namely that male rats displayed higher HPA axis hormone release than female rats following noise exposure.
In these experiments, careful verification of estrous cycle was performed after each stressor, and in experiments specifically targeting the stage of proestrus, animals were eliminated from analyses if they did not fit this stage. This stage was expected to illuminate the largest effect of sex following any stressor, since females in proestrus have been shown to have larger HPA axis responses to stress than other stages of the estrous cycle (Viau & Meaney, Citation1991). However, consistent with our previous observation that a females’ stage of estrous cycle does not affect hormone release or central activation of stress-related brain regions to acute restraint stress (Babb et al., Citation2013), no effect of the estrous cycle was observed in any experiment presented here. Female rats, even when in proestrus, do not appear to have higher HPA axis hormone responses to noise stress than males, and in some cases appear to have significantly smaller magnitude responses than males. Furthermore, removal of circulating sex steroids by ovariectomy actually increased HPA axis hormone responses to loud noise (mimicking intact male rats), and replacement with 17β-estradiol after this procedure did not augment, but instead reduced HPA axis hormone responses to loud noise (mimicking intact female rats). Although the regimen of chronic estradiol removal/replacement utilized in this study may not reflect exactly how estradiol acts in the normally dynamic system of the intact female, these data do provide evidence that estradiol may actually be inhibiting HPA axis hormone release to noise stress, unlike previous reports of the augmenting effect of this hormone on stress-induced HPA axis activity (Viau & Meaney, Citation1991; Viau et al., Citation2005). However, under basal conditions it does appear that estradiol (both in intact and ovariectomized females replaced with this hormone) has an activational effect on resting glucocorticoid hormone concentrations. That is, our data is consistent with the idea that estradiol increases adrenal sensitivity basally, producing augmented glucocorticoid levels when estradiol is present, as demonstrated by others (Figueiredo et al., Citation2007). Our observation that replacement with estradiol in ovariectomized rats appears to decrease stress-induced ACTH release following both restraint (Babb et al., Citation2013) and loud noise is also consistent with previous reports of the effects of sex steroid replacement on stress-induced ACTH release, although estradiol appears to play a smaller role, at least following loud noise, in stress-induced glucocorticoid release. Because we also observed that removing circulating sex steroids in the female actually increased stress-induced HPA axis hormone release to levels similar to intact males, these data also challenge the hypothesis that sex differences in stress-induced HPA axis hormone release are caused by estrogens augmenting, and androgens inhibiting, stress-induced HPA axis hormone release, as suggested by others (Viau et al., Citation2005). More research is needed to understand the mechanism through which circulating sex steroids affect HPA axis function.
These studies explored several possibilities of a stressor-specific effect of sex. First, the possibility that female rats have a less sensitive auditory system than male rats was tested with pre-pulse manipulation of the acoustic startle response. If this had been the case, it is conceivable that the lack of sex differences observed following noise stress could be attributed to female rats having higher auditory thresholds, leading to blunted HPA axis responses in this sex compared to male rats. However, not only did both sexes have equal suppression (GAP and PPI trials) and facilitation (PPF trials) of the acoustic startle response, but female rats actually had significantly higher baseline responses to the startle stimulus itself, suggesting that if anything, female rats may be behaviorally more sensitive to auditory stimulation compared to male rats, and not vice versa. Second, we tested the intriguing possibility that females could be having a larger HPA axis hormone response to restraint stress (typically given simultaneously in groups of animals in close proximity to each other) compared to noise stress (given in isolation in sound-attenuating chambers) due to the social cues of the stressor that are present or not. The hypothesis tested in this experiment was that the social context during restraint stress (sight, smell, and sound of other rats being stressed simultaneously) was in effect an additional stressor to the rats, and that these cues might have a greater impact in female compared to male rats. However, in contradiction to this hypothesis, the effect of sex did not disappear when male and female rats were given restraint in isolation. Female rats still displayed higher HPA axis hormones to restraint compared to male rats, regardless of whether the stress occurred in isolation or in close proximity to other rats. Furthermore, if anything, restraint given in isolation tended to increase HPA axis responses rather than decrease the activation of these hormones. Female rats reportedly have different pain sensitivity than male rats (Aloisi et al., Citation1994; Gamaro et al., Citation1998; Hurley et al., Citation2008), therefore we also tested the idea that female rats were displaying higher HPA axis responses to restraint stress due to the pain of blood sampling that typically occurred just prior to the presentation of this stressor. Female rats still displayed higher HPA axis responses to restraint without blood sampling prior to this stressor, suggesting that sex differences in response to restraint are not caused by differential pain processing in male and female rats. It is possible that these effects are not generalizable to all strains of rats, since this variable can affect stress responses (Bielajew et al., Citation2003; Dhabhar et al., Citation1993). It is also possible that single housing of animals in these experiments could have differentially affected females, leading to chronically elevated glucocorticoid levels prior to stressor exposure, although females still exhibited higher HPA axis hormone release following restraint stress and were housed in a similar manner.
It is possible that restraint is perceived by the animal as more stressful than noise stress, and thus brings out sex differences in HPA axis activation that noise stress does not. Experiencing restraint involves multiple sensory systems (e.g. auditory, somatosensory, visual, and olfactory systems,as compared to noise stress, which is assumed to be almost exclusively auditory. Restraint can involve not only the auditory system, but also activates many forebrain regions responsive to somatosensory input, including cortical regions, hippocampus, and prefrontal cortex (Chowdhury et al., Citation2000). We have demonstrated previously that female rats exhibit higher restraint stress-induced activation of the medial preoptic area (MPOA), bed nucleus of the stria terminalis (BST), and the PVN, despite similar activation of brain regions associated with somatosensory processing (Babb et al., Citation2013). In the present studies we demonstrate that noise can also significantly activate regions involved in stress responses, including the PVN, MPOA, and BSTav of the female brain, as well as others that we have previously reported to be activated by noise stress in male rats (Burow et al., Citation2005). However, despite significant activation of these stress-responsive brain regions, no significant effect of sex was observed in any of these regions (namely the MPOA and BSTav, regions found previously to accompany the higher stress-induced c-fos expression observed in the female PVN; Babb et al., Citation2013), or in regions involved in auditory processing ( and ). Therefore it is unlikely that noise stress does not induce sex differences in HPA axis responses simply because it is less stressful, since it clearly produces a stress response in both sexes, both peripherally in HPA axis hormone concentrations, and centrally in relevant brain regions. Our best hypothesis is that, for yet to be determined reasons, restraint stress is somehow inherently more stressful for female compared to male rats, relatively; noise stress alternatively is somehow inherently more stressful to male compared to female rats. The specific attributes relating to each type of stressor responsible for the differential effect of sex on HPA axis hormones following exposure to them, remain elusive.
Conclusions
The experiments presented here suggest that for some stressors, such as restraint, sex differences are robust and large in magnitude, where female rats demonstrate consistently higher magnitude of HPA axis responses to acute presentations of this stressor than male rats. However, results obtained herein using loud noise stress illustrate that sex differences are not always present, or can even exist in the opposite direction, where male rats exhibit significantly higher HPA axis responses to acute stress exposures compared to female rats. Whereas the factors responsible for differential effects of stressor modality have not been ascertained, it appears that auditory threshold, social cue, and nociceptive factors are not the primary stimulus dimensions responsible for stressor-specific differences in stress-induced HPA axis activation. More research is warranted to determine why stressors of different modalities can illicit differential effects of sex on stress-induced HPA axis activity.
Declaration of interest
The authors report no conflicts of interest. The authors alone are responsible for the content and writing of the paper.
This work was supported by NIH R01 MH077152 awarded to S. Campeau.
Acknowledgements
The authors would like to thank Dr. Robert Spencer (University of Colorado at Boulder, Department of Psychology and Neuroscience) for generously providing the authors with the restraint tubes used in this experiment and for his invaluable support of this work.
References
- Aloisi AM, Ceccarelli I, Lupo C. (1998). Behavioral and hormonal effects of restraint stress and formalin test in male and female rats. Brain Res Bull 47:57–62
- Aloisi AM, Steenbergen HL, van de Poll NE, Farabollini F. (1994). Sex-dependent effects of restraint on nociception and pituitary-adrenal hormones in the rat. Physiol Behav 55:1–5
- Babb JA, Masini CV, Day HEW, Campeau S. (2013). Sex differences in activated corticotropin-releasing factor neurons within stress-related neurocircuitry and hypothalamic-pituitary-adrenocortical axis hormones following restraint in rats. Neuroscience 234:40–52
- Bekker MH, van Mens-Verhulst J. (2007). Anxiety disorders: sex differences in prevalence, degree, and background, but gender-neutral treatment. Gend Med 4:S178–93
- Bielajew C, Konkle ATM, Kentner AC, Baker SL, Stewart A, Hutchins AA, Santa-Maria Barbagallo L, Fourirzos G. (2003). Strain and gender specific effects in the forced swim test: effects of previous stress exposure. Stress 6:269–80
- Bowen GP, Lin D, Taylor MK, Ison JR. (2003). Auditory cortex lesions in the rat impair both temporal acuity and noise increment thresholds, revealing a common neural substrate. Cerebral Cortex 13:815–22
- Burow A, Day HE, Campeau S. (2005). A detailed characterization of loud noise stress: Intensity analysis of hypothalamo-pituitary-adrenocortical axis and brain activation. Brain Res 1062:63–73
- Campeau S, Akil H, Watson SJ. (1997). Lesions of the medial geniculate nuclei specifically block corticosterone release and induction of c-fos mRNA in the forebrain associated with audiogenic stress in rats. J Neurosci 17:5979–92
- Campeau S, Nyhuis TJ, Sasse SK, Kryskow EM, Herlihy L, Masini CV, Babb JA, et al. (2010). Hypothalamic pituitary adrenal axis responses to low-intensity stressors are reduced after voluntary wheel running in rats. J Neuroendocrinol 22:872–88
- Campeau S, Watson SJ. (1997). Neuroendocrine and behavioral responses and brain pattern of c-fos induction associated with audiogenic stress. J Neuroendocrinol 9:577–88
- Campeau S, Watson SJ. (2000). Connections of some auditory-responsive posterior thalamic nuclei putatively involved in activation of the hypothalamo-pituitary-adrenocortical axis in response to audiogenic stress in rats: an anterograde and retrograde tract tracing study combined with Fos expression. J Comp Neurol 423:474–91
- Charitidi K, Meltser I, Canlon B. (2012). Estradiol treatment and hormonal fluctuations during the estrous cycle modulate the expression of estrogen receptors in the auditory system and the prepulse inhibition of acoustic startle response. Endocrinol 153:4412–21
- Chowdhury GM, Fujioka T, Nakamura S. (2000). Induction and adaptation of Fos expression in the rat brain by two types of acute restraint stress. Brain Res Bull 52:171–82
- Critchlow V, Liebelt RA, Bar-Sela M, Mountcastle W, Lipscomb HS. (1963). Sex difference in resting pituitary-adrenal function in the rat. Am J Physiol 205:807–15
- Day HE, Nebel S, Sasse S, Campeau S. (2005). Inhibition of the central extended amygdala by loud noise and restraint stress. Eur J Neurosci 21:441–54
- Dhabhar FS, McEwen BS, Spencer RL. (1993). Stress response, adrenal steroid receptor levels and corticosteroid-binding globulin levels – a comparison between Sprague-Dawley, Fischer 344 and Lewis rats. Brain Res 616:89–98
- Ehlert U, Gaab J, Heinrichs M. (2001). Psychoneuroendocrinological contributions to the etiology of depression, posttraumatic stress disorder, and stress-related bodily disorders: the role of the hypothalamus-pituitary-adrenal axis. Biol Psych 57:141–52
- Figueiredo HF, Ulrich-Lai YM, Choi DC, Herman JP. (2007). Estrogen potentiates adrenocortical responses to stress in female rats. Am J Physiol Endocrinol Metab 292:E1173–82
- Gamaro GD, Xavier MH, Denardin JD, Pilger JA, Ely DR, Ferreira MB, Dalmaz C. (1998). The effects of acute and repeated restraint stress on the nociceptive response in rats. Physiol Behav 63:693–7
- Heinsbroek RP, Van Haaren F, Feenstra MG, Endert E, Van De Poll, N. (1991). Sex- and time-dependent changes in neurochemical and hormonal variables induced by predictable and unpredictable footshock. Physiol Behav 49:1251–6
- Hurley RW, Adams MCB. (2008). Sex, Gender, and Pain: An overview of a complex field. Anesthes Analges 107:309–17
- Ison JR, O’Connor K, Bowen GP, Bocirnea A. (1991). Temporal resolution of gaps in noise by the rat is lost with functional decortication. Behav Neurosci 105:33–40
- Jovanovic T, Szilagyi S, Chakravorty S, Fiallos AM, Lewison BJ, Parwani A, Schwartz MP, et al. (2004). Menstrual cycle phase effects on prepulse inhibition of acoustic startle. Psychophysiol 41:401–6
- Kelly JB, Rooney BJ, Phillips DP. (1996). Effects of bilateral auditory cortical lesions on gap-detection thresholds in the ferret (Mustela putorius). Behav Neurosci 110:542–50
- Kessler RC, Berglund P, Demler O, Jin R, Merikangas KR, Walters EE. (2005). Lifetime prevalence and age-of-onset distributions of DSM-IV disorders in the National Comorbidity Survey Replication. Arch Gen Psychiatry 62:593–602
- Kitay JI. (1961). Sex differences in adrenal cortical secretion in the rat. Endocrinol 68:818–24
- Kitay JI. (1963). Pituitary-adrenal function in the rat after gonadectomy and gonadal hormone replacement. Endocrinol 73:253–60
- Le Mevel JC, Abitbol S, Beraud G, Maniey J. (1979). Temporal changes in plasma adrenocorticotropin concentration after repeated neurotropic stress in male and female rats. Endocrinol 105:812–17
- Linzer M, Spitzer R, Kroenke K, Williams JB, Hahn S, Brody D, deGruy F. (1996). Gender, quality of life, and mental disorders in primary care: results from the PRIME-MD 1000 study. Am J Med 101:526–33
- Masini CV, Babb JA, Nyhuis TJ, Day HEW, Campeau S. (2012). Auditory cortex lesions do not disrupt habituation of HPA axis responses to repeated noise stress. Brain Res 1443:1–9
- Masini CV, Day HEW, Campeau S. (2008). Long-term habituation to repeated loud noise is impaired by relatively short interstressor intervals in rats. Behav Neurosci 122:210–23
- Olff M, Langeland W, Draijer N, Gersons BP. (2007). Gender differences in posttraumatic stress disorder. Psychol Bull 133:183–204
- Panagiotaropoulous T, Papaioannou A, Pondiki S, Prokopiou A, Stylianopoulou F, Gerozissis K. (2004). Effect of neonatal handling and sex on basal and chronic stress-induced corticosterone and leptin secretion. Neuroendocrinology 79:109–18
- Parham K, Willott JF. (1988). Acoustic startle response in young and aging C57BL/6J and CBA/J mice. Behav Neurosci 102:881–6
- Paxinos G, Watson C. (2005). The rat brain in stereotaxic coordinates, 5th edition. Burlington, MA: Elsevier Academic Press
- Rivier C. (1991). Gender, sex steroids, corticotrophin-releasing factor, nitric oxide, and HPA response to stress. Pharmacol Biochem Behav 64:739–51
- Seale JV, Wood SA, Atkinson HC, Bate E, Lightman SL, Ingram CD, Jessop DS, Harbuz MS. (2004a). Gonadectomy reverses the sexually diergic patterns of circadian and stress-induced hypothalamic-pituitary-adrenal axis activity in male and female rats. J Neuroendocrinol 16:516–24
- Seale JV, Wood SA, Atkinson HC, Harbuz MS, Lightman SL. (2004b). Gonadal steroid replacement reverses gonadectomy-induced changes in the corticosterone pulse profile and stress-induced hypothalamic-pituitary-adrenal axis activity of male and female rats. J Neuroendocrinol 16:989–98
- Stein MB, Walker JR, Forde DR. (2000). Gender differences in susceptibility to posttraumatic stress disorder. Behav Res Ther 38:619–28
- Ström JO, Theodorsson E, Theodorsson A. (2008). Order of magnitude differences between methods for maintaining physiological 17beta-oestradiol concentrations in ovariectomized rats. Scan J Clin Lab Investig 68:814–22
- Tolin DF, Foa EB. (2006). Sex differences in trauma and posttraumatic stress disorder: a quantitative review of 25 years of research. Psychol Bull 132:959–92
- Vesga-Lopez O, Schneier FR, Wang S, Heimberg RG, Liu S-M, Hasin DS, Blanco C. (2008). Gender differences in generalized anxiety disorder: results from the National Epidemiologic Survey on Alcohol and Related Conditions (NESARC). J Clin Psychiatry 69:1606–16
- Viau V, Bingham B, Davis J, Lee P, Wong M. (2005). Gender and puberty interact on the stress-induced activation of parvocellular neurosecretory neurons and CRH mRNA expression in the rat. Endocrinol 146:137–46
- Viau V, Meaney MJ. (1991). Variations in the hypothalamic-pituitary-adrenal response to stress during the estrous cycle in the rat. Endocrinology 129:2503–11
- Willott JF, Carlson S, Chen H. (1994). Prepulse inhibition of the startle response in mice: relationship to hearing loss and auditory system plasticity. Behav Neurosci 108:703–13