Abstract
The neural cell adhesion molecule (NCAM) plays a crucial role in stress-related brain function, emotional behavior and memory formation. In this study, we investigated the functions of the glucocorticoid and serotonergic systems in mice constitutively deficient for NCAM (NCAM−/− mice). Our data provide evidence for a hyperfunction of the hypothalamic–pituitary–adrenal axis, with enlarged adrenal glands and increased stress-induced corticosterone release, but reduced hippocampal glucocorticoid receptor expression in NCAM−/− mice when compared to NCAM+/+ mice. We also obtained evidence for a hypofunction of 5-HT1A autoreceptors as indicated by increased 8-0H-DPAT-induced hypothermia. These findings suggest a disturbance of both humoral and neural stress systems in NCAM−/− mice. Accordingly, we not only confirmed previously observed hyperarousal of NCAM−/− mice in various anxiety tests, but also observed an increased response to novelty exposure in these animals. Spatial learning deficits of the NCAM−/− mice in a Morris Water maze persisted, even when mice were pretrained to prevent effects of novelty or stress. We suggest that NCAM-mediated processes are involved in both novelty/stress-related emotional behavior and in cognitive function during spatial learning.
Introduction
Ample evidence suggests that the neural cell adhesion molecule (NCAM) is involved in innate and acquired responses to novelty and stress. For instance, changes in the expression of NCAM observed after stressful experiences have been implicated in stress-dependent modulation of memory formation and altered affective behavior following stress exposure (Bisaz & Sandi, Citation2012; Bisaz et al., Citation2009, Citation2011; Sandi et al., Citation2005; Venero et al., Citation2002). Indeed, association of polymorphisms in the NCAM gene with stress-sensitive mental disturbances, such as bipolar disorder and schizophrenia, have been reported (Arai et al., Citation2004; Atz et al., Citation2007).
Studies on NCAM-deficient (NCAM−/−) mice are crucial to understand the neural processes underlying these phenomena. These animals are viable and show only mild deficits in postnatal central nervous system development (Cremer et al., Citation1994). However, they display profound disturbance of emotional behaviors including aggression, anxiety, and depression-like behaviors in association with a sensitization of the serotonergic system (Stork et al., Citation1997, Citation1999, Citation2000). Moreover, NCAM−/− mice show striking abnormalities that pertain to malfunctions of synaptic plasticity in the adult mouse and deficits in learning and memory (for reviews, see Berezin & Bock, Citation2004; Irintchev & Schachner, Citation2012; Maness & Schachner, Citation2007; Rønn et al., Citation2000; Schachner, Citation1997). Specifically, memory deficits in a contextual and auditory fear conditioning paradigm are linked to disturbances in stimulus salience coding and changes in amygdalo-hippocampal network patterns (Albrecht et al., Citation2009; Stork et al., Citation2000). Moreover, impairments in acquisition and retention in the hippocampus-dependent Morris water maze task for spatial learning were found that could at least in part be accounted for by increased stress-related floating behavior (Cremer et al., Citation1994; Stork et al., Citation2000).
Considering the importance of stress for the development of emotional disturbances and the influence of stress susceptibility on distinct processes of learning and memory (Bogdan et al., Citation2011; Salehi et al., Citation2010), in the current study we investigated the function of the humoral and neural stress systems in NCAM−/− mice and its implication for the previously observed changes in cognitive and emotional behavior. Our data demonstrate changes in plasma corticosterone levels and hippocampal glucocorticoid receptor (GR) expression indicative of a chronic stress state in these animals. The consequences of these changes for serotonin system function, novelty response and spatial memory were investigated. The combined findings indicate that NCAM-mediated cell interactions are critical for the normal functioning of the humoral and neural stress systems controlling emotional behavior and memory formation.
Material and methods
Animals and husbandry
NCAM−/− and NCAM+/+ mice were obtained from heterozygous (NCAM+/− x NCAM+/−) breeding pairs, which had been inbred for at least 8 generations on the C57BL/6 background. Mice were held under specific pathogen-free conditions with food and water being supplied ad libitum. At the age of 3 months, male mice were taken into the experimental animal facility and maintained in a reversed 12 hr light/dark cycle (light off at 7:00 am) under constant conditions (21 ± 1 °C; 60% humidity) for 2 weeks before the experiments started. The experimental room was adjacent to the animal facility and illuminated with red light. The equipment for experiments was cleaned after each mouse/test by wiping with water and 70% ethanol. All experiments were performed during the dark phase of the mice between 10:00 and 18:00 h, with at least one day between different tests. All tests were recorded by a video camera and evaluated by a trained experimenter blind to the genotype of the mice.
Determination of basal and stress-induced plasma corticosterone levels
Plasma corticosterone concentrations were determined in experimentally naïve NCAM+/+ mice and NCAM−/− littermates at baseline conditions and after exposure to a stressor (measurement of body temperature or tail suspension). All blood samples were collected between 13:00 and 16:00 h to minimize diurnal variations of plasma corticosterone levels. For baseline measurements, mice were taken in their home cages from the facility to an adjacent room where they were immediately decapitated. The transport time between housing facility and test room was less than 15 s. Blood was collected in heparinized capillaries within 3 min after being carried from the facility. For measurements after stress exposure, mice were taken from the housing facility (adjacent to the procedure room) and body temperature was determined immediately afterwards (see section “Hypothermia”). After 15 min, the body temperature was measured again. Blood samples were taken within 3 min after the last measurement of body temperature. Another batch of NCAM−/− and NCAM+/+ mice underwent the tail suspension test for 6 min and mice were placed back into their home cage and transported back to the animal facility. Blood samples from these mice were collected exactly 30 min after onset of the tail suspension test. All blood samples were centrifuged at 1700 g and 4 °C for 20 min. Plasma was stored at −20 °C. Plasma corticosterone concentrations were analyzed in duplicate by radioimmunoassay, using a specific antibody against corticosterone (MP Biomedicals, Illkirch, France). Adrenal glands were collected from twelve young adult NCAM−/− mice and thirteen age-matched NCAM+/+ mice, and weighed.
Measurement of GR mRNA expression
Total RNA from hippocampi of NCAM−/− and NCAM+/+ mice was isolated using the RNeasy Lipid Tissue kit (Qiagen, Hilden, Germany) in conjunction with on column DNAse I treatment to deplete contaminating genomic DNA. Equal amounts of RNA were then reverse transcribed using Supercript II (Invitrogen, Frankfurt, Germany) and a random hexamer primer (Metabion, Martinsried, Germany). Real-time PCR reaction was carried out in a volume of 20 µl on an ABI 7900 HT sequencer using a real-time PCR core kit (Eurogentec, Seraing, Belgium). Sequences of primers (Metabion) were: glucocorticoid receptor (GR, Nr3c1) (forward 5′-CAG CAT GCC GCT ATC GAA A-3′; reverse 5′-CGC GGC AGG AAC TAT TGT TTT-3′; Genebank accession number: NM_008173.2), and, for reference, hypoxanthine guanine phosphoribosyl transferase (Hprt; Jakovcevski et al., Citation2011; Maurer-Morelli et al., Citation2012) (forward 5′-GTT CTT TGC TGA CCT GCT GGA-3′; reverse 5′-TCC CCC GTT GAC TGA TCA TT-3′; Genebank accession number: NM_013556.1).
Determination of 8-hydroxy-2-(dipropylamino)-tetralin-induced hypothermia
Body temperature was measured by inserting a thermocouple probe (1.5 mm in diameter; CyberSense, Nicholasville, KY) 2 cm deep into the rectum. The mouse was held by the tail head-down and gently pressed to the cage wall. The temperature was stable within approximately 5s, and the handling lasted approximately 10 s. Immediately after the first measurement of body temperature (baseline), mice were injected (i.p.) with 8-hydroxy-2-(dipropylamino)-tetralin (8-OH-DPAT) (Sigma-Aldrich, St. Louis, MO) in saline and saline only as control. The injection volume for all solutions was the same (4 µl/g body weight). Different batches of mice were used for each dosage: saline; 0.01 mg/kg; 0.5 mg/kg; 1.0 mg/kg and 2.0 mg/kg. Body temperature was measured every 15 min for 2 hrs and then every 30 min until 3 h after injection. 8-OH-DPAT-induced hypothermia was calculated by the maximal decrease in body temperature from baseline for each mouse during the 3 hrs post-injection.
Anxiety and novelty response
Each eighteen NCAM+/+ mice and NCAM−/− mice housed in pairs with one male NCAM+/+ and one male NCAM−/− littermate mouse were used. Animals underwent a series of previously investigated exploration and anxiety tests (open field, elevated plus maze, light/dark avoidance) and were then finally studied for their novelty response in a new cage/new object task.
Open-field test
The open field test was performed in an enclosed arena, consisting of wood laminated with light-gray resin (50 cm × 50 cm and 40 cm high) and illuminated with white light (50 lux). Mice were gently placed into a transparent plastic cylinder (11 cm in diameter and 23 cm high) in one corner of the arena. The cylinder was removed 30 s after placing the mice, which were then allowed to freely move in the arena for 15 min. Exploratory activity was analyzed with EthoVision and Observer software (Noldus, Wageningen, The Netherlands).
Elevated plus maze
The elevated plus maze consisted of two opposite open arms and two opposite closed arms (each 30 cm × 5 cm) extending from a central platform (5 cm × 5 cm), elevated 75 cm above the ground. The closed arms were surrounded by 15 cm high walls. The maze consisted of white plastic. The experiment was performed under dim red light conditions (maximally 2 lux). Mice were started from the center facing an open arm, and exploration of the maze was video recorded for 5 min using an infrared camera. Exploration of closed and open arms was determined with The Observer.
Light/dark preference test
For this test, the arena was the same as used for the open-field test (Morellini & Schachner, Citation2006). A vertical projector differentially illuminated the floor of the open field: one quadrant was illuminated with dim red light (25 lux) while the rest of the arena was brightly lit with white light (200 lux). Mice were started from one of the three corners illuminated with white light and allowed to freely move for 10 min. Exploration of the different zones was analyzed with EthoVision.
New cage/new object tests
The new cage and new object tests were performed on two consecutive days. On day 1, mice were video recorded for 5 min after being placed into a new cage (38 cm × 22 cm and 15 cm high) with fresh bedding. Then, mice were left undisturbed for 24 h. On day 2, mice were video recorded as on day 1. Then, the top of the cage was gently opened and a new object was introduced into the cage. The new object was a plastic water bottle for rodents (7 cm × 7 cm and 10 cm high) with the bottom cut off and with an entrance of 3 × 4 cm2 on one side. The bottle was placed into one side of the cage with the entrance facing the center of the cage. Behavior was video recorded for 5 min after the new object was introduced into the cage. The following behavioral parameters were analyzed: rearing (on wall and off wall as defined in the open-field test), risk assessment (the mouse stretching its body forward and retracting to the original position without forward locomotion), tail-rattling (rapid movement of the tail), sniffing at object (nose was in direct contact with the object), rear at object (vertical exploration of the object by standing on the hind paws with one or two front paws at the object), head in (entering the object with at least the head) and time spent motionless at the side opposite to the object (no locomotion or activity besides movements of the head).
Spatial learning and memory
Mice were tested for spatial learning in the water maze paradigm. To reduce stress effects, one batch NCAM+/+ and NCAM−/− littermate mice underwent a pre-training procedure before starting the learning protocol in the Morris water maze test, while another batch of NCAM+/+ and NCAM−/− littermate mice underwent the Morris water maze test without pre-training.
Pre-training
A cage (38 × 22 cm and 15 cm high) was filled with water (21 ± 1 °C), which was made opaque with non-toxic white paint. Visual cues were occluded by brown curtains surrounding the cage. Mice had to find a platform (14 cm in diameter) that was submerged 1 cm beneath the water. Four trials per day were given with an inter-trial interval of 7–10 min. The trial duration was maximally 60 s. Starting points and platform positions were changed pseudo-randomly between the trials. After 2 days of training in the water filled cage, the mice underwent the visible platform version of the Morris water maze test. Again, the maze was surrounded by brown curtains with no visible landmarks, and the platform was cued with a black and white cylinder (4 cm in diameter and 14 cm high) placed on its center. Mice performed 4 trials with an inter-trial interval of 7–10 min. Mice started from 4 different positions and the platform was moved between trials (four different locations).
Morris water maze test
Mice were trained to find a platform (14 cm in diameter) in a circular pool with a diameter of 155 cm and white walls (10 cm high) above the water level. The pool was placed in the center of the experimental room (3.5 × 3.5 m), which was provided with six 50 × 50 cm landmarks placed on the walls at a height of 150–180 cm (Law et al., Citation2003). The platform was placed at 39 cm from the wall and submerged 1 cm beneath the water (21 ± 1 °C), which was made opaque with non-toxic white paint. During the experiment, mice were kept in an adjacent room illuminated by red dim light. Mice were taken to the pool in a plastic cup handled from a long stick. Six different starting positions were pseudo-randomized between trials. The maximum duration for each trial was 90 s (probe trials 60 s). About 20 s after the animals had climbed onto the platform, they were taken to their home cage where they were allowed to dry and warm under red light. The mice that did not find the platform were guided to the platform. Mice were given 4 trials per day with an inter-trial interval of 7–10 min. The probe trial for short-term memory (10 min after the latest training trial) was performed between trials 2 and 3 on day 8, while long-term memory was tested on day 9 (24 hrs after the last trial). To test visual abilities and motivation to find the platform, mice were trained with a visible platform as described above, starting 1 hr after the probe trial for long-term memory. During acquisition, the latency to find the platform, total distance moved, mean velocity and mean distance to the wall were analyzed using the software EthoVision. For the probe trials, also entries and time spent in defined zones (quadrants and platform positions) were calculated.
Statistical analysis
All data were analyzed with non-parametric statistics. Normal distribution was tested using the Kolmogorov–Smirnov normality test. Differences between groups were tested with the Mann–Whitney U-test. Since there is no non-parametric procedure for multifactorial analysis, a parametric analysis of variance for repeated measures (ANOVA) having the genotype as between factor and time intervals or trials as within factor followed by the Newman–Keuls post-hoc test was used.
Results
Glucocorticoid system
Two-way ANOVA showed a significant effect of treatment (F(1,10) = 24.89, p < 0.001), “cage-control” versus “stressed”, indicating that plasma corticosterone concentrations increased in both NCAM+/+ and NCAM−/− mice after stress exposure as compared to baseline. Moreover, a general effect of genotype (F(1,20) = 12.17, p < 0.001) but no interaction between genotype and treatment was observed, as NCAM−/− mice had higher corticosterone levels than NCAM+/+ mice in all treatment groups (Newman Keuls test, p < 0.05; ). Moreover, adrenal glands were heavier in NCAM−/− mice when compared to NCAM+/+ mice (; p < 0.05, Mann–Whitney U-test).
Figure 1. Abnormal sensitivity of the humoral and neural stress systems in NCAM−/− mice. (A) NCAM−/− mice (white bars; n = 13) tend to have higher plasma corticosterone levels at baseline compared to NCAM+/+ mice (black bars; n = 9). Mice of both genotypes have increased plasma corticosterone levels after both stressor 1 (rectal temperature measurement; n = 12 NCAM−/− and 10 NCAM+/+) and stressor 2 (tail suspension; n = each 6 per genotype). However, NCAM−/− mice present with significantly higher stress-induced corticosterone levels than NCAM+/+ mice. (B) NCAM−/− mice (n = 13) show a hypertrophy and increased weight of adrenal glands, compared to NCAM+/+ mice (n = 12). (C) Glucocorticoid receptor (GR) mRNA expression in the hippocampus of NCAM−/− mice (n = 8) is reduced when compared to NCAM+/+ mice (n = 9). These changes are indicative of a chronic hyperactivity of the HPA axis in NCAM−/− mice. Values indicate group means ± SEM; *, ** p ≤ 0.05, 0.01, respectively, compared to NCAM+/+.
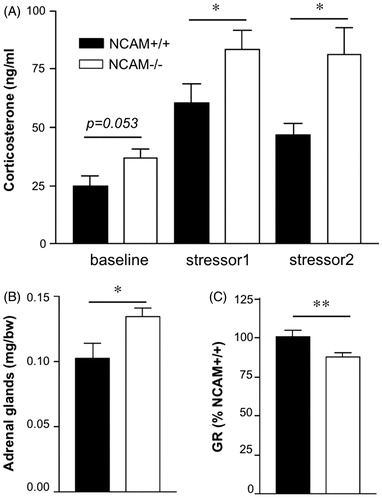
In conjunction with the analysis of HPA axis function, levels of GR mRNA were determined in the hippocampus, being one of the major brain regions involved in the feedback regulation of the HPA axis and playing an important role in the corticosterone-mediated regulation of response to novelty, learning and memory, anxiety and fear (de Kloet et al., Citation1998; Korte, Citation2001). GR mRNA levels were found to be decreased in NCAM−/− as compared to NCAM+/+ mice (; p < 0.01, Mann–Whitney U-test).
Serotonergic system
Systemic application of 8-OH-DPAT led to a dose-dependent decline of the body temperature in both genotypes () as shown by the significant effect of dosage on body temperature. There was an effect of genotype (F(1,15) = 17.21; p < 0.001) and 8-OH-DPAT dosage (F(4,60) = 26.61; p < 0.001), as well as an interaction between genotype and dosage (F(4,60) = 4.53; p < 0.01). The decrease in body temperature was lower in NCAM−/− than NCAM+/+ mice at both 1 mg/kg and 2 mg/kg body weight (p < 0.001 and p < 0.01, respectively; Newman–Keuls post-hoc comparison).
Figure 2. 8-OH-DPAT induced hypothermia. The hypothermic response to doses of 1.0 or 2.0 mg/kg of 8-OH-DPAT is reduced in NCAM−/− mice (n = 10 and 14, respectively), compared to NCAM+/+ mice (n = 8, 11). No significant difference between genotypes is seen at lower doses (n = 12 NCAM−/− versus 11 NCAM+/+ for saline, 9 versus 9 for 0.01 mg/kg and 13 versus 10 for 0.5 mg/kg). These data indicate a desensitization of 5-HT1A autoreceptors in the dorsal Raphe nucleus. Values indicate group means ± SEM; **, *** p ≤ 0.01, 0.001, respectively, compared to NCAM+/+.
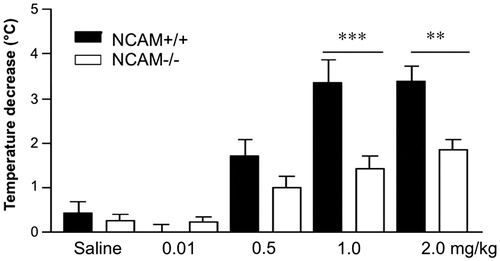
Anxiety and novelty response
In a series of tests we confirmed previously observed increases in arousal and anxiety in NCAM−/− mice: in the open field test they displayed higher locomotion, as indicated by increased distance moved (Mann–Whitney U-test; p < 0.01), but reared less on walls as compared to NCAM+/+ mice (p < 0.05). Likewise, they showed an increased total distance moved in the light/dark test and increased entries into the dark compartment (p < 0.001). In the elevated plus maze, the exploration of open arms (p < 0.001, compared to NCAM+/+), but not that of closed arms was increased in the NCAM−/− mice ().
Figure 3. Increased arousal and novelty response in NCAM−/− mice. (A) NCAM−/− mice (n = 18) show higher locomotor activity and reduced wall-supported rearing in comparison to NCAM+/+ mice (n = 18) in an open field. (B) Increased locomotor activity and increased number of entries to the dark are evident in a light/dark test of anxiety. (C) In the elevated plus maze, NCAM−/− mice show a remarkable increase in open arm exploration, but no change in closed arm exploration. (D) NCAM−/− mice in the new cage/new object test show increased rearing on both test days, indicating an enhanced novelty response. Values indicate group means ± SEM; *, **, *** p ≤ 0.05, 0.01, 0.001, respectively, compared to NCAM+/+. ## p ≤ 0.01 compared to NCAM+/+ mice on day 1.
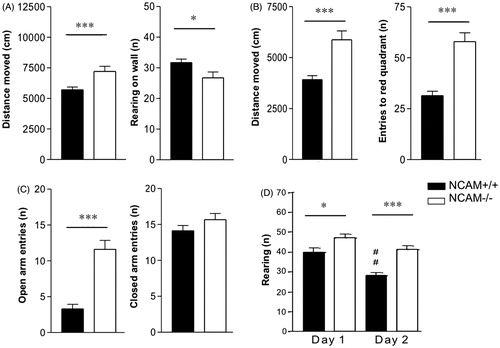
Finally, we tested the novelty response of NCAM−/− and NCAM+/+ mice by placing them into a fresh home cage and observed that NCAM−/− mice reared more often in this situation than their NCAM+/+ littermates (p < 0.05) (). On the following day, we further analyzed the object-directed exploration and trait anxiety by placing a new object into the home cage. NCAM−/− mice explored the novel object with higher frequency than NCAM+/+ mice: sniffing at (31.64 ± 2.8 versus 18.12 ± 2.02 in NCAM+/+; p < 0.001), rearing on (3.36 ± 1.06 versus 1.2 ± 0.7 in NCAM+/+; p < 0.01; ) and entering into the object (1.91 ± 0.41 versus 0.24 ± 0.12 in NCAM+/+; p < 0.001) were increased in the mutant mice. At the same time, risk assessment behavior towards the object was reduced, as seen by the small number of stretched attend postures of NCAM−/− mice (1.23 ± 0.34 versus 2.88 ± 0.55 in NCAM+/+; p < 0.05).
Spatial learning
Two batches of mice were tested. One batch received pre-training (PT): Familiarization to swimming in water and learning to escape onto a platform (first in a water-filled cage and then in a pool with a visible platform). The other batch started immediately with the spatial learning task.
Learning without pre-training
During spatial acquisition, NCAM−/− mice swam longer distances until finding the platform (; p < 0.001; effect of genotype; repeated measures ANOVA), but when the platform was cued at the end of the experiment to test for motivation and visual accuracy, no differences were found between the genotypes in the latency to find the platform (data not shown) and in the total distance moved.
Figure 4. Pre-training in NCAM−/− mice improves emotional, but not cognitive parameters in a Morris water maze task. (A,C,E,G) NCAM−/− mice (white dots/bars, n = 12) without pre-training (VP) show delayed acquisition (p ≤ 0.001) (A) and reduced long-term memory (G) accompanied by increased signs of stress and arousal, for instance floating behavior during the first training day and increased swimming velocity during later stages of the learning process as compared to NCAM+/+ mice (black rhomb/bars; n = 11). (B,D,F,H) Pre-training (PT) reduces the emotional response of floating in both genotypes, but does not ameliorate the deficit in learning (B; p ≤ 0.01) or long-term memory of the spatial task (NCAM−/−, n = 14; NCAM+/+, n = 11). Moreover, swim speed is similarly high in both genotypes. Values indicate group mean values ± SEM; *, **, *** p ≤ 0.05, 0.01, 0.001, compared to NCAM+/+.
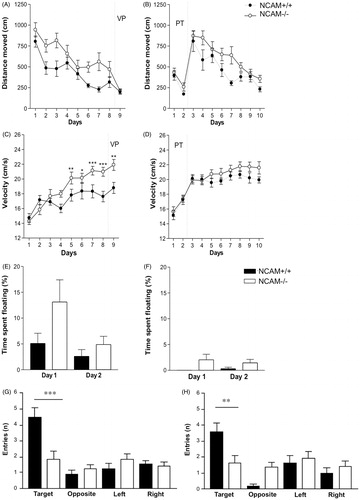
NCAM−/− mice increased their mean velocity more readily than NCAM+/+ mice (): Significant differences in velocity were detectable from day 5 onward (day 5, 9: p < 0.01; day 7, 8: p < 0.001 and day 6: p < 0.05 Newman–Keuls post-hoc comparison after repeated measures ANOVA; ). The mutants also tended to spend more time floating () on the first day of training as compared to NCAM+/+ mice, but this floating behavior declined to the same and lower level as seen in NCAM+/+ mice at the second day of acquisition.
Also, genotype differences in short-term memory were detected. Yet, both genotypes preferred the target quadrant (values are given as mean percentage of time with standard error of the mean; NCAM+/+ mice: 47.15 ± 4.27; NCAM−/− mice: 37.67 ± 2.48). The number of entries into the platform position as an indicator of precision was similar in NCAM−/− and NCAM+/+ mice. However, NCAM−/− mice were impaired in long-term memory as compared to NCAM+/+ mice. Both genotypes preferred the target quadrant (values given as mean percentage of time with standard error of the mean; NCAM+/+ mice: 48.79 ± 4.72; NCAM−/− mice: 36.86 ± 2.72), but NCAM−/−mice spent less time there than NCAM+/+ mice (Newman–Keuls test; p < 0.001). They also showed a less precise memory than NCAM+/+ mice, with search time spent at the exact platform position not above chance level and with a reduced number of entries to this position, when compared to NCAM+/+ mice (p < 0.001; ).
Learning with pre-training
During pre-training, genotypes did not differ in any of the parameters analyzed: Latency to find the platform (data not shown), total distance moved and swimming velocity were similar for NCAM+/+ and NCAM−/− mice.
During spatial memory acquisition, NCAM−/− mice swam longer distances until finding the platform than NCAM+/+ mice (repeated measures ANOVA, effect of genotype: p < 0.01; ). They also needed more time to find the platform than NCAM+/+ mice (p < 0.01).
Yet, NCAM−/− and NCAM+/+ mice did not differ significantly in the mean velocity, in contrast to the increased velocity of NCAM−/− mice without pre-training (). Floating behavior was nearly absent in both genotypes when the spatial acquisition was started ().
Under these conditions, genotype differences in short-term memory were detected. Both genotypes preferred the target quadrant, but NCAM−/− mice stayed less time in the target area (NCAM+/+ mice: 45.67 ± 2.61; NCAM−/− mice: 34.83 ± 4.12). Also, the number of entries into the platform position were lower with NCAM−/− mice than in NCAM+/+ littermates, indicating that NCAM−/− mice were less precise when locating the platform (Newman--Keuls test, p < 0.01; ). In addition, NCAM−/− mice were impaired in long-term memory, as compared to NCAM+/+ littermates. Only NCAM+/+ mice clearly preferred the target quadrant (NCAM+/+ mice: 41.73 ± 2.57; NCAM−/− mice: 33.83 ± 4.76), with the time spent in the target platform position being not above the chance level for NCAM−/− mice ().
Discussion
Evidence indicates that NCAM mediates stress effects on the nervous system, in particular, in the context of memory formation and in the control of affective behaviors. Here we demonstrate (1) that NCAM deficiency in mice disturbs the function of the neural and humoral stress systems in a manner reflecting a chronic stress state and (2) that NCAM−/− mice show an increased responsiveness to novelty. Moreover, (3) spatial learning deficits persist even when learning-associated arousal in NCAM−/− mice is reduced through pre-training.
We observed in NCAM−/− mice an increased adrenal gland weight, a tendency for increased plasma baseline corticosterone levels and significantly increased corticosterone levels after acute stress exposure, as well as reduced hippocampal GR mRNA expression. These combined changes are typical of a chronic hyperactivity of the HPA axis, as observed in chronically stressed rodents (Choi et al., Citation2008; Desarnaud et al., Citation2008; Hügin-Flores et al., Citation2004; Sheppard et al., Citation1990). These changes may indeed be caused by a defect in the feedback regulation of the HPA system, as GR haplodeficient (GR+/−) mice have a normal basal level but increased stress-induced corticosterone levels (Ridder et al., Citation2005). Glucocorticoids show a tight interplay with central serotonergic functions during the response to stress, and 5-HT1A receptors are thought to be the central mediators in the relationship between the serotonergic and HPA systems (Chaouloff, Citation1993; McAllister-Williams et al., Citation2001). For instance, there is evidence that stimulation of post-synaptic 5-HT1A receptors at the level of the hypothalamic paraventricular nucleus increases the secretion of corticotropin and corticosterone (Pan & Gilbert, Citation1992). Moreover, increased stress-induced levels of extracellular 5-HT are observed in the hippocampus of GR+/− mice (Linthorst et al., Citation2000), and the corticosterone-induced increase of 5-HT1A receptor expression was elevated in GR+/− mice (Hensler et al., Citation2010).
Partial and full 5-HT1A receptor agonists are thougth to exert anxiolytic effects, mainly via somatodendritic autoreptors in the Raphe nuclei. A contribution of postsynaptic receptors has, however, also been described (Schreiber & De Vry, Citation1993). We have described an increased responsiveness of NCAM−/− mice to the anxiolytic-like effects of low doses of the 5-HT1A receptor agonists buspirone and 8-OH-DPAT, although no abnormalities were apparent in these animals in 5-HT1A receptor binding/distribution or in the content of 5-HT and its metabolites in limbic brain areas (Stork et al., Citation1999). In the current study, we further investigated the serotonergic system of NCAM−/− mice by analyzing 8-OH-DPAT-induced hypothermia, as an in vivo model of somatodendritic 5-HT1A autoreceptor function (Bill et al., Citation1991; El Yacoubi et al., Citation2003; Man et al., Citation2000). A significantly reduced hypothermic response of NCAM−/− compared to NCAM+/+ mice was observed. In view of these findings, it is likely that the previously observed hypersensitivity to anxiolytic doses of buspirone and 8-OH-DPAT in NCAM−/− mice may have involved changes in pre- and postsynaptic 5-HT1A receptor function. The serotonergic imbalance in these animals may be a consequence of stress and altered HPA axis function, as both repetitive serotonergic stimulation (Martin et al., Citation1992) and intermittant stimulation with corticosterone can produce a comparable desensitization of autoreceptors (Man et al., Citation2000; McAllister-Williams et al., Citation2001). However, it should be considered that NCAM deficiency may also interfere with signalling processes downstream of the 5-HT1A receptor (Delling et al., Citation2002). The combined observations support the view of a generalized functional imbalance of humoral and neural stress systems in NCAM−/− mice, which may explain the previously observed change in anxiety and arousal in these animals. In fact, high activity in the open field and elevated plus maze tests, similar to those in NCAM−/− mice, have also been reported in rats with an enhanced stress response of the HPA axis (Kalinichev et al., Citation2002; Steimer & Driscoll, Citation2003). Moreover, coordinate changes of corticosterone secretion and 5-HT1A autoreceptor function are evident in rats selected for their high vs low response to novelty (Calvo et al., Citation2011). NCAM−/− mice accordingly showed an increased activity and lack of habituation in the new cage/new object task, indicating an increased response to novelty. It may be worth mentioning in this context that 5-HT1A receptor agonists show strong structural and functional similarities to small organic compounds mimicking polysialic acid, a glycan attached to the protein backbone of the three major isoforms of NCAM which are independent in their action of their functions as 5-HT1A receptor agonists (Loers et al., Citation2013) The PSA mimicking functions are abolished in primary cultures of several types of neurons and glial cells of NCAM−/− mice compared to NCAM+/+ mice. It remains to be seen how this relationship would impact on NCAM-dependent behavior and the effects of NCAM deficiency.
Functions of the HPA axis and the serotonin system (Egasihra et al., Citation2006; Elvander-Tottie et al., Citation2009; Wolff et al., Citation2004) are known to be critical for performance in spatial memory tasks. Moreover, NCAM–corticosterone interactions have been suggested to be involved in the effects of psychosocial stress on spatial learning and its association with novelty reactivity (Touyarot et al., Citation2004). We hypothesized that an enhanced response to novelty would be involved in the learning deficits of NCAM−/− mice and tested whether amelioration of training-related stress through pre-training (Beiko et al., Citation2004) would improve their performance in the Morris water maze test. In fact, high initial floating and increased swimming velocity that were evident without pre-training, were not observed in NCAM−/− mice when pre-training was performed. Still, no amelioration of spatial memory deficits was apparent in the probe trial of pre-trained NCAM−/− mice, and short-term memory deficits became evident in these mice in particular under pre-training conditions. Our data confirm previous findings of disturbed spatial memory in NCAM−/− mice (Cremer et al., Citation1994; Stork et al., Citation2000), and argue for an involvement of NCAM-mediated mechanisms of synaptic plasticity (Bukalo et al., Citation2004; Senkov et al., Citation2006; Stoenica et al., Citation2006), independent of training-associated emotional responses. Our findings are also in agreement with observations on the dissociation of spatial memory performance and emotional behavior resulting from transgenic rescue in NCAM−/− mice expressing the NCAM180 isoform in mature neurons (Stork et al., Citation2000). On the other hand, exposure to predator stress interferes with both spatial memory and NCAM180 expression in the hippocampus (Sandi et al., Citation2005). Thus, both stress-related and stress–independent NCAM mechanisms appear to contribute to adaptive behavior and learning in mice. In this context it is interesting to mention that genetic inactivation of GRs through point mutation results in spatial memory deficits without significant effects on anxiety-like behaviors (Oitzl et al., Citation2001). Hence, reduced hippocampal GR function may affect memory formation in NCAM−/− mice even under low stress conditions and with apparently normal emotional responses. It will be important to determine the precise interplay of different NCAM-mediated functions during learning and to analyze, e.g., whether an altered HPA function in NCAM−/− mice differentially affects distinct phases of memory formation.
Declaration of interest
The authors report no conflicts of interest. The authors alone are responsible for the content and writing of this article. This work was supported by the German Research Foundation (Deutsch-Israelische Projektkooperation, HE1128/16 to O.S.) and by the Li Kashing Foundation at Shantou University Medical College.
References
- Albrecht A, Bergado-Acosta JR, Pape H-C, Stork O. (2009). Role of the neural cell adhesion molecule (NCAM) in amygdalo-hippocampal interactions and salience determination of contextual fear memory. Int J Neuropsychopharmacol 13:661–74
- Arai M, Itokawa M, Yamada K, Toyota T, Arai M, Haga S, Ujike H, et al. (2004). Association of neural cell adhesion molecule 1 gene polymorphisms with bipolar affective disorder in Japanese individuals. Biol Psychiat 55:804–10
- Atz ME, Rollins B, Vawter MP. (2007). NCAM1 association study of bipolar disorder and schizophrenia: polymorphisms and alternatively spliced isoforms lead to similarities and differences. Psychiatr Genet 17:55–67
- Beiko J, Lander R, Hampson E, Boon F, Cain DP. (2004). Contribution of sex differences in the acute stress response to sex differences in water maze performance in the rat. Behav Brain Res 151:239–53
- Berezin V, Bock E. (2004). NCAM mimetic peptides: pharmacological and therapeutic potential. J Mol Neurosci 22:33–9
- Bill DJ, Knight M, Forster EA, Fletcher A. (1991). Direct evidence for an important species difference in the mechanism of 8-OH-DPAT-induced hypothermia. Br J Pharmaco 103:1857–64
- Bisaz R, Sandi C. (2012). Vulnerability of conditional NCAM-deficient mice to develop stress-induced behavioral alterations. Stress 15:195–206
- Bisaz R, Conboy L, Sandi C. (2009). Learning under stress: a role for the neural cell adhesion molecule NCAM. Neurobiol Learn Mem 91:333–42
- Bisaz R, Schachner M, Sandi C. (2011). Causal evidence for the involvement of the neural cell adhesion molecule, NCAM, in chronic stress-induced cognitive impairments. Hippocampus 21:56–71
- Bogdan R, Santesso DL, Fagerness J, Perlis RH, Pizzagalli DA. (2011). Corticotropin-releasing hormone receptor type 1 (CRHR1) genetic variation and stress interact to influence reward learning. J Neurosci 31:13246–54
- Bukalo O, Fentrop N, Lee AY, Salmen B, Law JW, Wotjak CT, Schweizer M, et al. (2004). Conditional ablation of the neural cell adhesion molecule reduces precision of spatial learning, long-term potentiation, and depression in the CA1subfield of mouse hippocampus. J Neurosci 24:1565–77
- Calvo N, Cecchi M, Kabbaj M, Watson SJ, Akil H. (2011). Differential effects of social defeat in rats with high and low locomotor response to novelty. Neuroscience 183:81–9
- Chaouloff F. (1993). Physiopharmacological interactions between stress hormones and central serotonergic systems. Brain Res Brain Res Rev 18:1–32
- Choi DC, Furay AR, Evanson NK, Ulrich-Lai YM, Nguyen MM, Ostrander MM, Herman JP. (2008). The role of the posterior medial bed nucleus of the stria terminalis in modulating hypothalamic–pituitary–adrenocortical axis responsiveness to acute and chronic stress. Psychoneuroendocrinology 33:659–69
- Cremer H, Lange R, Christoph A, Plomann M, Vopper G, Roes J, Brown R, et al. (1994). Inactivation of the N-CAM gene in mice results in size reduction of the olfactory bulb and deficits in spatial learning. Nature 367:455–9
- de Kloet ER, Vreugdenhil E, Oitzl M, Joëls M. (1998). Brain corticosteroid receptor balance in health and disease. Endocr Rev 19:269–301
- Delling M, Wischmeyer E, Dityatev A, Sytnyk V, Veh RW, Karschin A, Schachner M. (2002). The neural cell adhesion molecule regulates cell-surface delivery of G-protein-activated inwardly rectifying potassium channels via lipid rafts. J Neurosci 22:7154–64
- Desarnaud F, Jakovcevski M, Morellini F, Schachner M. (2008). Stress downregulates hippocampal expression of the adhesion molecules NCAM and CHL1 in mice by mechanisms independent of DNA methylation of their promoters. Cell Adh Migr 2:38–44
- Egashira N, Yano A, Ishigami N, Mishima K, Iwasaki K, Fujioka M, Matsushita M, et al. (2006). Investigation of mechanisms mediating 8-OH-DPAT-induced impairment of spatial memory: involvement of 5-HT1A receptors in the dorsal hippocampus in rats. Brain Res 1069:54–62
- Elvander-Tottie E, Eriksson TM, Sandin J, Ogren SO. (2009). 5-HT(1A) and NMDA receptors interact in the rat medial septum and modulate hippocampal-dependent spatial learning. Hippocampus 19:1187–98
- El Yacoubi M, Bouali S, Popa D, Naudon L, Leroux-Nicollet I, Hamon M, et al. (2003). Behavioral, neurochemical, and electrophysiological characterization of a genetic mouse model of depression. Proc Natl Acad Sci USA 100:6227–32
- Hensler JG, Vogt MA, Gass P. (2010). Regulation of cortical and hippocampal 5-HT(1A) receptor function by corticosterone in GR+/− mice. Psychoneuroendocrinology 35:469–74
- Hügin-Flores ME, Steimer T, Aubert ML, Schulz P. (2004). Mineralo- and glucocorticoid receptor mrnas are differently regulated by corticosterone in the rat hippocampus and anterior pituitary. Neuroendocrinology 79:174–84
- Irintchev A, Schachner M. (2012). The injured and regenerating nervous system: immunoglobulin superfamily members as key players. Neuroscientist 18:452–66
- Jakovcevski M, Schachner M, Morellini F. (2011). Susceptibility to the long-term anxiogenic effects of an acute stressor is mediated by the activation of the glucocorticoid receptors. Neuropharmacology 61:1297–305
- Kalinichev M, Easterling KW, Plotsky PM, Holtzman SG. (2002). Long-lasting changes in stress-induced corticosterone response and anxiety-like behaviors as a consequence of neonatal maternal separation in Long-Evans rats. Pharmacol Biochem Behav 73:131–40
- Korte SM. (2001). Corticosteroids in relation to fear, anxiety and psychopathology. Neurosci Biobehav Rev 25:117–42
- Law JW, Lee AY, Sun M, Nikonenko AG, Chung SK, Dityatev A, Schachner M, Morellini F. (2003). Decreased anxiety, altered place learning, and increased CA1 basal excitatory synaptic transmission in mice with conditional ablation of the neural cell adhesion molecule L1. J Neurosci 23:10419–32
- Linthorst AC, Flachskamm C, Barden N, Holsboer F, Reul JM. (2000). Glucocorticoid receptor impairment alters CNS responses to a psychological stressor: an in vivo microdialysis study in transgenic mice. Eur J Neurosci 12:283–91
- Loers G, Saini V, Mishra B, Papastefanaki F, Lutz D, Chaudhury S, Ripoll DR, Wallqvist A. (2013). Nonyloxytryptamine mimics polysialic acid and modulates neuronal and glial functions in cell culture. J Neurochem 2013 Aug 20. doi: 10.1111/jnc.12408. [Epub ahead of print]
- Man MS, Young AH, McAllister-Williams RH. (2000). Corticosterone modulation of somatodendritic 5-HT1A receptor function in mice. J Psychopharmacol 16:245–52
- Maness P, Schachner M. (2007). Neural recognition molecules of the immunoglobulin superfamily: signaling transducers of axon guidance and neuronal migration. Nat Neurosci 10:19–26
- Martin KF, Phillips I, Hearson M, Prow MR, Heal DJ. (1992). Characterization of 8-OH-DPAT-induced hypothermia in mice as a 5-HTlA autoreceptor response and its evaluation as a model to selectively identify antidepressants. Br J Pharmacol 107:15–21
- Maurer-Morelli CV, de Vasconcellos JF, Reis-Pinto FC, Rocha Cde S, Domingues RR, Yasuda CL, Tedeschi H, et al. (2012). A comparison between different reference genes for expression studies in human hippocampal tissue. J Neurosci Methods 208:44–7
- Morellini F, Schachner M. (2006). Enhanced novelty-induced activity, reduced anxiety, delayed resynchronization to daylight reversal and weaker muscle strength in tenascin-C-deficient mice. Eur J Neurosci 23:1255–68
- McAllister-Williams RH, Anderson AJ, Young AH. (2001). Corticosterone selectively attenuates 8-OH-DPAT-mediated hypothermia in mice. Int J Neuropsychopharmacol 4:1–8
- Oitzl MS, Reichardt HM, Joëls M, de Kloet ER. (2001). Point mutation in the mouse glucocorticoid receptor preventing DNA binding impairs spatial memory. Proc Natl Acad Sci USA 98:12790–5
- Pan L, Gilbert F. (1992). Activation of 5-HT1A receptor subtype in the paraventricular nuclei of the hypothalamus induces CRH and ACTH release in the rat. Neuroendocrinology 56:797–802
- Ridder S, Chourbaji S, Hellweg R, Urani A, Zacher C, Schmid W, Zink M, et al. (2005). Mice with genetically altered glucocorticoid receptor expression show altered sensitivity for stress-induced depressive reactions. J Neurosci 25:6243–50
- Rønn LC, Berezin V, Bock E. (2000). The neural cell adhesion molecule in synaptic plasticity and ageing. Int J Dev Neurosci 18:193–9
- Salehi B, Cordero MI, Sandi C. (2010). Learning under stress: the inverted-U-shape function revisited. Learn Mem 17:522–30
- Sandi C, Woodson JC, Haynes VF, Park CR, Touyarot K, Lopez-Fernandez MA, Venero C, Diamond DM. (2005). Acute stress-induced impairment of spatial memory is associated with decreased expression of neural cell adhesion molecule in the hippocampus and prefrontal cortex. Biol Psychiatry 57:856–64
- Schachner M. (1997). Neural recognition molecules and synaptic plasticity. Curr Opin Cell Biol 9:627–34
- Schreiber R, De Vry J. (1993). Neuronal circuits involved in the anxiolytic effects of the 5-HT1A receptor agonists 8-OH-DPAT ipsapirone and buspirone in the rat. Eur J Pharmacol 249:341–51
- Senkov O, Sun M, Weinhold B, Gerardy-Schahn R, Schachner M, Dityatev A. (2006). Polysialylated neural cell adhesion molecule is involved in induction of long-term potentiation and memory acquisition and consolidation in a fear-conditioning paradigm. J Neurosci 26:10888–98
- Sheppard KE, Roberts JL, Blum M. (1990). Differential regulation of type II corticosteroid receptor messenger ribonucleic acid expression in the rat anterior pituitary and hippocampus. Endocrinology 127:431–9
- Steimer T, Driscoll P. (2003). Divergent stress responses and coping styles in psychogenetically selected Roman high-(RHA) and low-(RLA) avoidance rats: behavioural, neuroendocrine and developmental aspects. Stress 6:87–100
- Stoenica L, Senkov O, Gerardy-Schahn R, Weinhold B, Schachner M, Dityatev A. (2006). In vivo synaptic plasticity in the dentate gyrus of mice deficient in the neural cell adhesion molecule NCAM or its polysialic acid. Eur J Neurosci 23:2255–64
- Stork O, Welzl H, Cremer H, Schachner M. (1997). Increased intermale aggression and neuroendocrine response in mice deficient for the neural cell adhesion molecule (NCAM). Eur J Neurosci 9:1117–25
- Stork O, Welzl H, Wotjak CT, Hoyer D, Delling M, Cremer H, Schachner M. (1999). Anxiety and increased 5-HT1A receptor response in NCAM null mutant mice. J Neurobiol 40:343–55
- Stork O, Welzl H, Wolfer D, Schuster T, Mantei N, Stork S, Hoyer D, et al. (2000). Recovery of emotional behaviour in neural cell adhesion molecule (NCAM) null mutant mice through transgenic expression of NCAM180. Eur J Neurosci 12:3291–306
- Touyarot K, Venero C, Sandi C. (2004). Spatial learning impairment induced by chronic stress is related to individual differences in novelty reactivity: search for neurobiological correlates. Psychoneuroendocrinology 29:290–305
- Venero C, Tilling T, Hermans-Borgmeyer I, Schmidt R, Schachner M, Sandi C. (2002). Chronic stress induces opposite changes in the mRNA expression of the cell adhesion molecules NCAM and L1. Neuroscience 115:1211–19
- Wolff M, Costet P, Gross C, Hen R, Segu L, Buhot MC. (2004). Age-dependent effects of serotonin-1A receptor gene deletion in spatial learning abilities in mice. Brain Res Mol Brain Res 130:39–48