Abstract
The long-term effects of comfort food in an anxiogenic model of stress have yet to be analyzed. Here, we evaluated behavioral, endocrine and metabolic parameters in rats submitted or not to chronic unpredictable mild stress (CUMS), with access to commercial chow alone or to commercial chow and comfort food. Stress did not alter the preference for comfort food but decreased food intake. In the elevated plus-maze (EPM) test, stressed rats were less likely to enter/remain in the open arms, as well as being more likely to enter/remain in the closed arms, than were control rats, both conditions being more pronounced in the rats given access to comfort food. In the open field test, stress decreased the time spent in the centre, independent of diet; neither stress nor diet affected the number of crossing, rearing or grooming episodes. The stress-induced increase in serum corticosterone was attenuated in rats given access to comfort food. Serum concentration of triglycerides were unaffected by stress or diet, although access to comfort food increased total cholesterol and glucose. It is concluded that CUMS has an anorexigenic effect. Chronic stress and comfort food ingestion induced an anxiogenic profile although comfort food attenuated the endocrine stress response. The present data indicate that the combination of stress and access to comfort food, common aspects of modern life, may constitute a link among stress, feeding behavior and anxiety.
Introduction
Stress is considered a major risk factor for the development of various chronic diseases, such as metabolic syndrome, anxiety and other affective disorders (Cohen et al., Citation2012; Pacák & Palkovits, Citation2001; van Praag, Citation2004). Although stress has traditionally been defined as a non-specific response of the organism to environmental demands, the so-called “stressors” (Selye, Citation1974), it is now known that various stressors can trigger specific physiological and behavioral responses (Pacák & Palkovits, Citation2001; Zafar et al., Citation1997). Acute stress rapidly activates the sympathetic nervous system (SNS), thereafter activating the Hypothalamic-Pituitary-Adrenal (HPA) axis. Although activation of those two axis constitutes a hallmark of the stress response, the level of activation is stressor-specific.
In healthy individuals, the stress response is short-lived because activation of the SNS is rapidly counter balanced by the parasympathetic nervous system and the HPA axis response is terminated via negative feedback loops. Glucocorticoids act on receptors at the level of the paraventricular nucleus and the pituitary to inhibit the production and release of corticotropin-releasing hormone and adrenocorticotropic hormone. Indirect negative feedback from limbic structures such as the medial prefrontal cortex and hippocampus also contributes to termination of the HPA axis response (McEwen & Gianaros, Citation2010). Although the stress response is necessary for survival and adaptation, disorders can arise when it is prolonged and, in vulnerable individuals, exposure to chronic stress can adversely affect various aspects of health (McEwen & Gianaros, Citation2010).
Stress has long been associated with changes in dietary preference and food intake, as well as with weight gain and fat accrual, although the specific mechanisms are poorly understood. Psychological stress is linked to visceral deposition of fat, which is in turn associated with increased health risks (Chrousos, Citation2000). Accordingly, in animal models stress is known to suppress appetite (Bazhan et al., Citation2007; De Souza et al., Citation2000; Harris et al. Citation2001) and in human studies, it became clear that stress can decrease or increase food intake (Adam & Epel Citation2007; Epel et al., Citation2004) with stress-induced obesity being more prevalent (Anderson et al., Citation2006; Brunner et al., Citation2007). Indeed, repeated exposure of rats to chronic restraint stress modifies not only the amount of food intake but also eating patterns, particularly concerning the ingestion of sweet food (Ely et al., Citation1997). It has been demonstrated that humans under stress also show an increased preference for foods rich in sugar and fat (Tomiyama et al., Citation2011). Furthermore, eating foods rich in fat and carbohydrates during stress reduces the endocrine stress response (Dallman et al., Citation2003, Citation2005; Ortolani et al., Citation2011). It has been proposed that the desire to eat foods that are more satisfying is attributable to the effect of glucocorticoids that are released during stress (Pecoraro et al., Citation2005), as well as activation of the neural systems involved in the cognitive and reward aspects of ingestive behavior (Torres & Nowson, Citation2007).
We recently reported that the anorexigenic effect of foot-shock stress in rats is independent of leptin and is restricted to commercial chow intake, since no changes in the intake of comfort food was observed (Ortolani et al., Citation2011). We found that, in the Elevated Plus-Maze (EPM) and in the open field tests, rats submitted to foot-shock stress (one session per day for three consecutive days; i.e. short-term stress) exhibited less anxiety than did non-stressed rats, and that access to comfort food potentiated that effect. However, there have been no studies of the long-term effects of comfort food intake in a model of stress that is recognized as being anxiogenic.
In the present study, we evaluated behavioral, endocrine and metabolic parameters, including feeding behavior, exhibited by rats submitted to chronic unpredictable mild stress (CUMS) and given access to comfort food. We hypothesized that this chronic stress model, which is recognized as producing anxiety, would alter feeding patterns and increase the serum corticosterone concentration and that the intake of comfort food would attenuate these effects promoted by stress in rats.
Materials and methods
Animals
Forty-nine adult male Wistar rats, weighing 200 to 250 g at the beginning of the experiments, were used. The rats were housed in a temperature-controlled room (22 ± 2 °C), on a 12 h/12 h light/dark cycle (lights on at 07:00 h). The rats were housed in groups of five or four during the acclimation phase. After the first day of CUMS, control and stressed rats were housed individually in Plexiglas cages with sawdust covering the cage floor.
During the experiments, the rats were cared for in accordance with the Federation of American Societies for Experimental Biology Statement of Principles for the Use of Animals in Research & Education. The experimental protocols were approved by the Institutional Committee for Ethics in Animal Experimentation of the Federal University of São Paulo (certificate 1400/11).
The rats were distributed into four groups, according to diet and experimental protocol: those given access to commercial chow (CC) only, submitted to CUMS or not (CC-CUMS group and CC-control group, respectively); and those given access to commercial chow and to comfort food (CF), submitted to CUMS or not (CC/CF-CUMS group and CC/CF-control group, respectively). The diets were started three days before the first stress session and were maintained throughout the experimental period.
Diet composition
All rats were given ad libitum access to commercial chow for rodents (Nuvilab; Nuvital, Curitiba, Brazil) and tap water. The CC/CF-CUMS and CC/CF-control groups were also given ad libitum access to comfort food, which was composed of powdered commercial rodent chow (Nuvilab; Nuvital), peanuts (Hikari, São Paulo, Brazil), milk chocolate (Garoto, Vila Velha, Brazil) and sweet (cornstarch) cookies (Tostines; Nestlé, São Paulo, Brazil) in a proportion of 3:2:2:1. The comfort food diet, which was pelletised, provided the following (Ortolani et al., Citation2011): 20% protein, 20% fat, 48% carbohydrate and 4% fibre. Caloric densities, determined with an adiabatic calorimeter (C400; IKA Works, São Paulo, Brazil), were found to be 17.03 kJ/g for the commercial chow diet and 21.40 kJ/g (35% of calories as fat) for the comfort food diet (Estadella et al., Citation2004). To determine the amount of food consumed by each rat, we weighed the food remaining in the feeders at the end of each 24-hour period, using a digital scale.
CUMS protocol
The CUMS protocol was performed as previously described (Moreau et al., Citation1994a,Citationb), and shown in . Rats were subjected to six different stressors, which varied from day to day, for 14 consecutive days, as follows: two 30-min periods of restraint, achieved through confinement in a small acrylic cylinder (16 × 7.5 × 7.0 cm); lights on overnight; water and food deprivation overnight (no access to water and food), followed by 2 h of restricted access to food (45 mg of food pellets were placed into a Petri dish which was positioned on the floor of the cage); water deprivation overnight (no access to water), followed by 1 h of contact with an empty bottle; damp sawdust overnight; and inversion of the light/dark cycle from Friday night to Monday morning.
Table 1. Chronic unpredictable mild stress protocol (Moreau et al., 1994).
EPM test
After the last day of the CUMS protocol, the rats were evaluated in the EPM, under a 60 lux light, as previously described (Melo et al., Citation1995; Ortolani et al., Citation2011; Pellow et al., Citation1985). The rats were placed individually in the centre of the maze, facing one of the closed arms at the junction between open and closed arms. For a period of 5 min, the rat’s behavior was registered on video. A rat was considered to have entered an arm of the maze if all four paws were within that arm. Conventional parameters of anxiety-like behavior were monitored, including the number of entries into closed arms, the number of entries into open arms and the total time spent in each arm. The ratio between time spent in open or closed arms and time spent in the open plus the closed arms was calculated and multiplied by 100 to obtain the percentage of time spent in all arms. These categories were defined as in previous studies (Anseloni & Brandão, Citation1997; Cruz et al., Citation1994; Ortolani et al., Citation2011). After the removal of each rat, the maze was cleaned with a 10% ethanol solution.
Open field test
Immediately after the EPM test, each rat was evaluated in the open field, under a 60 lux light. The apparatus consists of an acrylic cylinder, 72 cm in diameter, and 60 cm high; the base is divided into 12 equal areas (rectangles measuring 13.3 by 15.0 cm). As in the EPM test, the rat’s behavior was registered on video for a period of 5 min. Rats were placed individually in the center of the open field and left for 5 min, and the following parameters were analyzed: latency to the first crossing (a crossing defined as the rat entering another square with all four paws); time spent in the periphery and in the centre of the apparatus; number of crossings; and number of rearing and grooming episodes. After each trial, the apparatus was cleaned with a 10% ethanol solution.
Biochemical analyses
After the last day of chronic stress, one group of rats that were not evaluated in the behavioral tests were euthanized by conscious decapitation between 08:00 h and 11:00 h. Blood was collected in plastic vials and centrifuged for 20 min at 3363 g after which aliquots of serum were removed and stored at −80 °C until assayed for metabolic markers.
Serum corticosterone concentrations were determined by enzyme-linked immunoassay using a commercial kit (Enzo Life Science Inc., Ann Arbor, MI, USA). Samples were run in duplicate, and results are reported as ng/mL. Concentration was determined as percentage bound by using a standard curve ranging from 32 to 20,000 pg/mL. Kit sensitivity was 27 pg/mL and the intrassay coefficient of variation was 8%. For serum glucose, we used Accu-Chek (Roche Diagnostics, São Paulo, Brazil) for glucose test. For serum total cholesterol and triglycerides levels, a standard enzymatic colorimetric kit was used (Labtest Diagnostic S.A., Lagoa Santa, Brazil). Samples were run in duplicate, and the results were reported as mg/dl.
Statistical analysis
Results are expressed as mean ± standard error of mean. Unpaired Student’s t test was used to evaluate significant differences between unstressed and stressed rats. To analyze the effect of stress and the type of diet two-way analysis of variance followed by the Bonferroni test was used. Values of p < 0.05 were considered statistically significant.
Results
Food intake
Food intake was influenced by stress (F[1,39] = 23.59; p < 0.0001) and diet (F[1,39] = 2549; p < 0.0001). Rats in the CC-control group ingested less amount of commercial chow during the observation period compared with CC-CUMS group (p = 0.013, ). shows that these groups ate similar amounts in the day before the start of the CUMS protocol (Day 0), and that the difference between groups first became significant after day 10 of the CUMS protocol (F[1,19] = 241.8; p < 0.05). An apparent difference between CC-control and CC-CUMS on day 1 was not confirmed by statistical analysis. The rats that had access to both diets (CC/CF-CUMS and CC/CF-control groups) showed a significant preference for comfort food (F[1,39] = 2427; p < 0.0001; ). The rats in the CC/CF-CUMS group ate similar amounts of food compared with the other groups in the day before the CUMS protocol started (Day 0), but this group reduced their intake of commercial chow, as compared to control rats, after day 5 (F[1,39] = 15.19; p < 0.0001; ) and reduced their comfort food intake on day 3, after the food restriction period of the CUMS protocol (F[1,39] = 24.58; p < 0.0001; ). Therefore, the rats submitted to CUMS decreased their total caloric intake (F[1,49] = 83330; p < 0.0001) as compared to control rats. Also, an interaction between stress and comfort food was observed (F[1,49] = 30540; p < 0.0001).
Figure 1. Daily food intake (mean ± sem; n = 10 rats/group) by rats submitted (CUMS) or not (control) to chronic unpredictable mild stress and given access to commercial chow only (CC). Note: On day 0, rats were not submitted to CUMS (the day before the stress protocol), on days 2 and 9, rats submitted to CUMS had access to food and water for only 12 h and restricted access for an additional 2 hours. On days 3 and 10, rats submitted to CUMS had access to water for only 12 h. *p < 0.05 versus controls (two-way ANOVA with Bonferroni test).
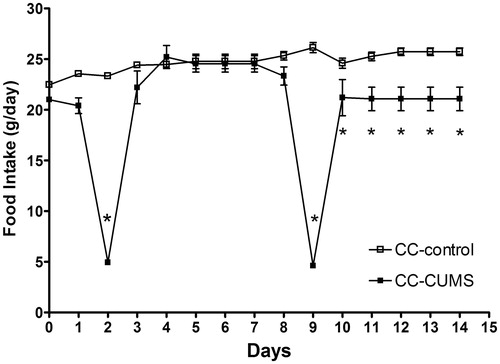
Figure 2. Daily intake of commercial chow (CC) and comfort food (CF) shown separately for the control group and for the chronic unpredictable mild stress group (CUMS) given the combined food choice. Hence, total daily food intake (not shown) for the control and CUMS groups can be interpolated by summing daily CC and CF intakes for each group. Data are mean ± sem, n = 10 rats/group. On day 0, rats were not submitted to CUMS (the day before the stress protocol); on days 2 and 9, rats submitted to CUMS had access to food and water for only 12 h and restrict access for an additional 2 hours. On days 3 and 10, rats submitted to CUMS had access to water for only 12 h. *p < 0.05 versus controls (two-way ANOVA with Bonferroni test).’.
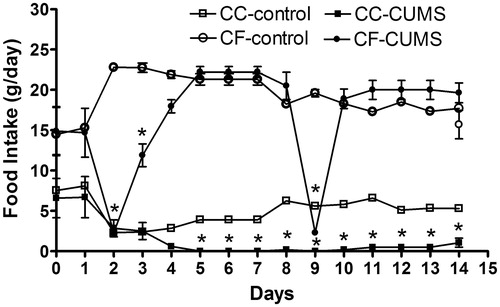
Table 2. Daily food and caloric intake, initial body weight and body weight gain by rats submitted (CUMS) or not (control) to chronic unpredictable mild stress and given access either to commercial chow (CC) only or to commercial chow and comfort food (CC/CF).
Although all of the rats gained weight during the experimental protocol, the stress and the diet both affected the body weight. The weight gain was less pronounced in the CC-CUMS and CC/CF-CUMS group rats than in the CC-control and CC/CF-control group rats (F[1,48] = 42.02; p < 0.0001). However, the weight gain was greater in the CC/CF-CUMS group rats than in the CC-CUMS group rats (F[1,48] = 26.41; p < 0.0001; ).
Behavioral analysis
The stress protocol affected behavior in the EPM. In comparison with their respective control groups, the CC-CUMS and CC/CF-CUMS groups showed a lower percentage of entries into and less time spent in the open arms of the EPM (F[1,37] = 19.80; p < 0.0001 and F[1,37] = 15.07; p < 0.0005, respectively), whereas the percentage of entries into and the time spent in the closed arms were both higher (F[1,37] = 19.80; p < 0.0001 and F[1,37] = 8.74; p = 0.005, respectively), as shown in . Comfort food intake also affected the time spent in the arms of the EPM. In comparison with the CC-control rats, the CC/CF-control rats spent less time in the open arms (F[1,37] = 12.76; p = 0.001) and more time in the closed arms (F[1,37] = 8.89; p = 0.005). There was no interaction between stress and diet in the entries and the time spent in the arms of the EPM.
Figure 3. Percentage of entries into (A) and time spent in (B) the open and closed arms of the elevated plus-maze by rats submitted (CUMS) or not (control) to chronic unpredictable mild stress and given access either to commercial chow (CC) only or to commercial chow (CC) and comfort food (CF). (n = 10 rats/group). *p < 0.05, stress effect; **p < 0.05, diet effect (two-way ANOVA followed by Bonferroni test).
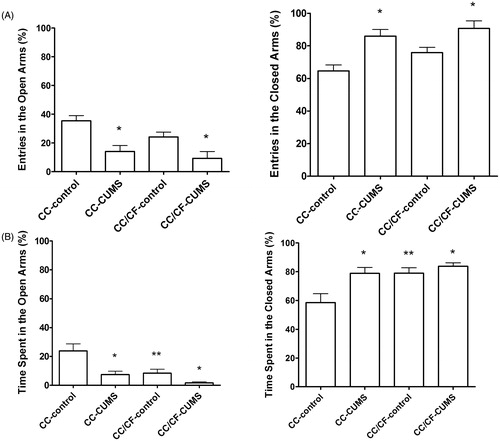
In the open field tests (), the stress protocol affected the time spent in the centre and the periphery. The CC-CUMS and CC/CF-CUMS group rats spent less time in the centre of the open field than did the corresponding control rats (F[1,37] = 10.88; p = 0.002), consequently spending more time in the periphery (F[1,37] = 10.19; p = 0.003). Access to comfort food did not alter those parameters. Neither stress nor diet affected the latency to the first crossing, the number of crossings, or the number of rearing and grooming episodes.
Table 3. Behavior in the open field test of rats submitted (CUMS) or not (control) to chronic unpredictable mild stress and given access either to commercial chow only (CC) or to commercial chow and comfort food (CC/CF).
Hormone and metabolic parameters
Serum corticosterone concentration was higher in the CC-CUMS group rats than in the CC-control group rats (F[1,24] = 7.94; p = 0.0005). Serum corticosterone concentration did not differ between the CC-control and CC/CF-control groups. However, the stress-induced increase in serum corticosterone concentration was attenuated in the CC/CF-CUMS rats ().
Figure 4. Serum corticosterone concentration (mean ± sem) by rats submitted (CUMS) or not (control) to chronic unpredictable mild stress and given access either to commercial chow (CC) only or to commercial chow (CC) and comfort food (CF). CC-control group: n = 8 rats/group; CC-CUMS group: n = 5 rats/group; CC/CF-control group: n = 8 rats/group; CC/CF-CUMS group: n = 4 rats/group. *p < 0.05, stress effect; **p < 0.05, interaction between stress and diet effects (two-way ANOVA with Bonferroni test).
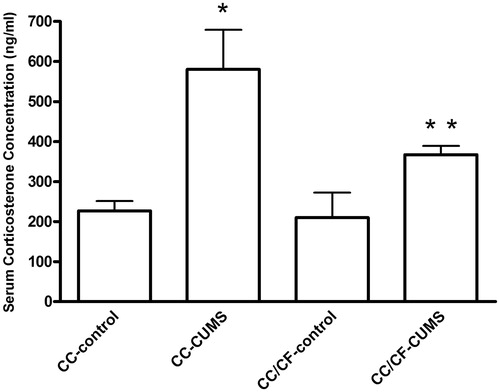
shows that the serum total cholesterol and glucose concentrations were not affected by stress. However, these concentrations were significantly higher in the CC/CF-control rats than in the CC-control rats (F[1,18] = 5.58; p = 0.032; F[1,17] = 4.675; p = 0.048, respectively). There was no interaction between stress and diet. Serum triglyceride concentration was unaffected by the CUMS protocol or diet composition.
Table 4. Serum concentrations of glucose, total cholesterol and triglycerides of rats submitted (CUMS) or not (control) to chronic unpredictable mild stress and given access either to commercial chow (CC) only or to commercial chow and comfort food (CC/CF).
Discussion
Stress is known to suppress appetite in laboratory rodents (Bazhan et al., Citation2007; De Souza et al., Citation2000; Harris et al., Citation2001), whereas in humans stress-induced obesity is more prevalent (Anderson et al., Citation2006; Brunner et al., Citation2007). Indeed, data presented here show that rats submitted to CUMS decreased their intake of commercial chow and comfort food, a different behavior compared with foot-shock stressed rats that reduced their intake of commercial chow but did not alter the intake of comfort food (Ortolani et al., Citation2011). This difference might be attributable to the type, duration, or severity of the stressor (Pecoraro et al., Citation2004). A stress-induced reduction in food intake has been demonstrated both as an acute response after a single stress and as a maintained decrease in 24-h food intake during and after repeated daily restraint stress (Krahn et al., Citation1990; Shimizu et al., Citation1989). Rats submitted to 3 h/day of restraint stress for 5 days increased their intake of fat and sucrose (Dallman et al., Citation2003; La Fleur et al., Citation2004; Liang et al., Citation2008; Pecoraro et al., Citation2004). However, in rats exposed to the same stressor for only 1 h/day, 5 days/week for 40 days, no such change was observed (Krolow et al., Citation2010). Glucocorticoids released during the stress response are considered to be orexigenic. Nevertheless, there are other influences in the regulation of food intake (Bazhan & Zelena, Citation2013) that should be taken into consideration, such as the catecholamines released by the sympathetic nervous system and adrenal medulla (Berthoud & Morrison, Citation2008); the other HPA axis hormones, including corticotropin-releasing hormone and adrenocorticotropic hormone; leptin and insulin (Adam & Epel, Citation2007); and the activation of the neural system involved with the cognitive, affective (reward), emotional and behavioral aspects of ingestive behavior (Torres & Nowson, Citation2007).
In the present study, all of the rats showed a clear preference for comfort food, and that preference was not altered by stress. From data in , it can be seen that in the normal chow-fed (CC) groups the stress-evoked reduction in caloric intake was more pronounced (about 92%) than it was in the chow-fed groups given comfort food (CF) (∼76%). Considering that the comfort food is more palatable than the commercial chow, it can be suggested that the stressed (CUMS) rats probably did not develop anhedonia. However, Moreau et al., (Citation1994) found that rats submitted to the CUMS protocol decreased responsiveness to a rewarding stimulus, which was a bottle of drinking water with sucrose, and this decrease is considered an indication of anhedonia. However, we did not test the rat preference for sucrose, the gold standard method to test anhedonia.
It has been previously reported that the intake of comfort food reduces the HPA stress response (Dallman et al., Citation2003; Pecoraro et al., Citation2004; Ortolani et al., Citation2011), and this was evidenced by our finding since the stress-induced increase in the serum corticosterone concentration was attenuated in the rats with access to comfort food. It has been proposed that the intake of comfort food activates the reward system in the brain and dampens the stress responses (Dallman et al., Citation2003). In obese patients, the overconsumption of comfort foods is thought of as a kind of addiction, which is supported by imaging studies that have revealed dysregulation of the dopaminergic reward circuitry, similar to that observed in drug addiction (Volkow et al., Citation2011). Indeed, the ingestion of high-calorie foods is known to counteract some effects of stress, probably via the same mechanism reported for addiction disorders (Kiefer & Grosshans, Citation2009). The rewarding system was not addressed in the present work. However, we found that the rats submitted to CUMS showed an increased level of anxiety, evidenced by fewer entries into and less time spent in the open arms of the EPM, as well as less time spent in the centre of the open field. Such an anxiogenic effect of stress has previously been reported by others (Carobrez & Bertoglio, Citation2005; Krolow et al., Citation2010; Prut & Belzung, Citation2003; Teegarden & Bale, Citation2007). In contrast, in a previous study (Ortolani et al., Citation2011), we showed that foot-shock stress has an opposite, anxiolytic effect on rats submitted to the EPM test. These conflicting results may be due to the biphasic dose-dependent effects of glucocorticoids on anxiety-related behaviors: anxiolytic effects at low doses and anxiogenic effects at high doses (Vafaei et al., 2008). In addition, it has been hypothesized that the brain categorizes stressors and utilizes neural response pathways that vary in accordance with the assigned stress category (Herman et al., Citation2003). Categorization proponents (Sawchenko et al., Citation1996; Herman & Cullinan, Citation1997; Sawchenko et al., Citation2000; Herman et al., Citation2003) generally suggest that the brain discriminates between two major types of stressor: (1) stimuli which produce actual disturbances of physiological status that overwhelm specific homeostatic mechanisms, e.g. haemorrhage or infection; (2) stimuli which threaten the individual’s current or anticipated state, e.g. social conflict, aversive environmental stimuli, predator-related cues, failure to satisfy internal drives. Stressors in the first category have been labeled with terms such as physical or systemic; those of the second category have commonly been labeled as psychological or emotional. If the brain does deal with stressors categorically, one might predict that stressors should elicit category-specific patterns of neuronal activity in the brain. Cespedes et al., (Citation2010) have reported that different brain areas are activated by foot-shock and restraint stress. In this case, the different nature of the stimuli might explain the different behavioral response to foot-shock stress (Ortolani et al., Citation2011) and to CUMS, as found in the present study.
It is of note that, in the present study, the control rats with access to comfort food for 17 days also spent less time in the open arms of the EPM. Fulton & Sharma, (Citation2013) also reported that rats fed a high-fat diet for 12 weeks had higher levels of anxiety and depression, increased HPA axis responses to stress and biochemical changes in the reward system. These data indicate that, despite reducing the corticosterone response to stress, access to comfort food can affect other systems related or not to anxiety, such as the animal’s metabolic profile.
It is known that there is a relationship between stress, high intake of fat and metabolic disorders (Froy, Citation2007; Koolhaas et al., Citation2011). In humans, stress has been known to increase serum triglyceride concentrations (Rosmond et al., Citation1998). However, in animals, this response is variable, and the main effects are found in chronic stress, with a reduction or increase, or even no significant changes (Chuang et al., Citation2010; Ricart-Jané et al., Citation2002). CUMS and access to comfort food did not alter the serum triglyceride concentrations in the present study. However, the comfort food (high-fat diet) intake increased serum concentrations of cholesterol and glucose. There is an extensive literature characterizing responses to high-fat feeding in rodents, and the findings of the present study are in line with previous reports (Buettner et al., Citation2007; Yang et al., Citation2007). It is known that high fat diets produce a consistent and significant increase in body weight, hypertrophy and hyperplasia of adipocytes, dyslipidemia, and insulin resistance, depending on the amount of fat in the diet, duration of feeding, and strain of the experimental animal (Buettner et al., Citation2006; Woods et al., Citation2003). Therefore, consumption of energy rich high-fat diet may alleviate stress-induced anxiety, reduce the endocrine stress response, but probably will lead to obesity and metabolic diseases.
Conclusions
The present data indicate that CUMS has an anorexigenic effect on the intake of commercial chow and comfort food, in contrast with our previous finding that foot-shock stress altered only the intake of commercial chow (Ortolani et al., Citation2011). In the present study, the intake of comfort food attenuated the endocrine component of the stress response, as represented by the serum corticosterone concentration. Nevertheless, chronic emotional stress and the intake of comfort food induced an anxiogenic profile. Taken together, these data indicate that the combination of stress and access to comfort food, both of which are elements of modern life, may represent a link between stress and feeding behavior, as well as between stress and anxiety in people.
Declaration of interest
The authors report no conflicts of interest. The authors alone are responsible for the content and writing of the paper. This study received financial support from the Brazilian Coordenação de Aperfeiçoamento de Pessoal de Nível Superior (CAPES, Office for the Advancement of Higher Education Personnel, Federal Government, Brasil) and from the Fundação de Amparo à Pesquisa do Estado de São Paulo (FAPESP, Foundation for Research Support of the State of São Paulo, SP, Brasil).
References
- Adam TC, Epel SE. (2007). Stress, eating and the reward system. Physiol Behav 91:449–58
- Anderson SE, Cohen P, Naumova EN, Must A. (2006). Association of depression and anxiety disorders with weight change in a prospective community-based study of children followed up into adulthood. Arch Pediatr Adolesc Med 160(3):285–91
- Anseloni VCZ, Brandão ML. (1997). Ethopharmacological analysis of behaviour of rats using variations of the elevated plus-maze. Behav Pharmacol 8:533–40
- Bazhan NV, Makarova EN, Shevchenko A, Iakovleva TV. (2007). Repeating of emotional stress prevents development of melanocortin obesity and type 2 diabetes in the mice with the Agouti yellow mutation. Ross Fiziol Zh Im IM Sechenova 93:1237–44
- Bazhan N, Zelena D. (2013). Food-intake regulation during stress by the hypothalamo-pituitary-adrenal axis. Brain Res Bull 95:46–53
- Berthoud HR, Morrison C. (2008). The brain, appetite, and obesity. Ann Rev Psychol 59:55–92
- Brunner EJ, Chandola T, Marmot MG. (2007). Prospective effect of job strain on general and central obesity in the Whitehall II Study. Am J Epidemiol 165(7):828–37
- Buettner R, Parhofer KG, Woenckhaus M, Wrede CE, Kunz-Schughart LA, Schölmerich J, Bollheimer LC. (2006). Defining high-fat-diet rat models: metabolic and molecular effects of different fat types. J Mol Endocrinol 36(3):485–501
- Buettner R, Scholmerich J, Bollheimer LC. (2007). High-fat diets: modeling the metabolic disorders of human obesity in rodents. Obesity 15(4):798–808
- Carobrez AP, Bertoglio LJ. (2005). Ethological and temporal analyses of anxiety like behavior: the elevated plus-maze model 20 years on. Neurosci Biobehav Rev 29:1193–205
- Cespedes IC, de Oliveira AR, da Silva JM, da Silva AV, Sita LV, Bittencourt JC. (2010). mRNA expression of corticotropin-releasing factor and urocortin 1 after restraint and foot shock together with alprazolam administration. Peptides 31(12):2200–8
- Chrousos GP. (2000). The role of stress and the hypothalamic-pituitary-adrenal axis in the pathogenesis of the metabolic syndrome: neuro-endocrine and target tissue related causes. Int J Obes Relat Metab Disord 24:S50–5
- Chuang JC, Cui H, Mason BL, Mahgoub M, Bookout AL, Yu HG, Perello M, Elmquist JK, et al. (2010). Chronic social defeat stress disrupts regulation of lipid synthesis. J Lipid Res 51(6):1344–53
- Cohen S, Janicki-Deverts D, Doyle WJ, Miller GE, Frank E, Rabin BS, Turner RB. (2012). Chronic stress, glucocorticoid receptor resistance, inflammation and disease risk. Proc Natl Acad Sci USA 109(16):5995–9
- Cruz AP, Frei F, Graeff FG. (1994). Ethopharmacological analysis of rat behavior on the elevated plus-maze. Pharmacol Biochem Behav 49(1):171–6
- Dallman MF, Pecoraro N, Akana SF, La Fleur SE, Gomez F, Houshyar H. (2003). Chronic stress and obesity: a new view of ‘‘comfort food’’. Proc Natl Acad Sci USA 100:11696–701
- Dallman MF, Pecoraro N, La Fleur SE. (2005). Chronic stress and comfort foods: Self-medication and abdominal obesity. Brain Behav Immun 19:275–80
- De Souza J, Butler AA, Cone RD. (2000). Disproportionate inhibition of feeding in A(y) mice by certain stressors: a cautionary note. Neuroendocriology 72:126–32
- Ely DR, Dapper V, Marasca J, Corrêa JB, Gamaro GD, Xavier MH, Michalowski MB, et al. (1997). Effect of restraint stress on feeding behavior of rats. Physiol Behav 61(3):395–8
- Epel E, Jimenez S, Brownell K, Stroud L, Stoney C, Niaura R. (2004). Are stress eaters at risk for the metabolic syndrome? Ann N Y Acad Sci 1032:208–10
- Estadella D, Oyama LM, Dâmaso AR, Ribeiro EB, Do Nascimento CO. (2004). Effect of palatable hyperlipidic diet on lipid metabolism of sedentary and exercised rats. Nutrition 20:218–24
- Froy O. (2007). The relationship between nutrition and circadan rhythms in mammals. Front Neuroendocrinol 28(2–3):61–71
- Fulton S, Sharma S. (2013). Diet-induced obesity promotes depressive-like behavior that is associated with neural adaptations in brain reward circuitry. Int J Obes 37(3):382–9
- Harris RB, Zhou J, Shi M, Redmann S, Mynatt RL, Ryan DH. (2001). Overexpression of agouti protein and stress responsiveness in mice. Physiol Behav 73:599–608
- Herman J, Cullinan W. (1997). Neurocircuitry of stress. Trends Neurosci 20:78–84
- Herman JP, Figueiredo H, Mueller NK, Ulrich-Lai Y, Ostrander MM, Choi DC, Cullinan WE. (2003). Central mechanisms of stress integration: hierarchical circuitary controlling hypothalamo-pituitary-adrenocortical responsiveness. Front Neuroendocrinol 24:151–80
- Kiefer F, Grosshans M. (2009). What can addiction research contribute towards the understanding of obesity? Nervenarzt 80:1040–9
- Koolhaas JM, Bartolomucci A, Buwalda B, de Boer SF, Flügge G, Korte SM, Meerlo P, et al. (2011). Stress revisited: a critical evaluation of the stress concept. Neurosci Biobehav Rev 35(5):1291–301
- Krahn DD, Gosnell BA, Majchrzad MJ. (1990). The anorectic effects of CRH and restraint stress decrease with repeated exposures. Biol Psychiatr 27:1094–102
- Krolow RCG, Noschang D, Arcego AC, Andreazza W, Peres CA, Gonçalves C, Dalmaz MF. (2010). Consumption of a palatable diet by chronically stressed rats prevents effects on anxiety-like behavior but increases oxidative stress in a sex-specific manner. Appetite 55:108–16
- La Fleur SE, Akana SF, Manalo SL, Dallman MF. (2004). Interaction between corticosterone and insulin in obesity: regulation of lard intake and fat stores. Endocrinology 145:2174–85
- Liang S, Byers DM, Irwin LN. (2008). Sex and diet affect the behavioral response of rats to chronic mild stressors. Physiol Behav 93:27–36
- McEwen BS, Gianaros PJ. (2010). Central role of the brain in stress and adaptation: links to socioeconomic status, health, and disease. Ann N Y Acad Sci 1186:190–222
- Melo, LL, Brandão, ML. (1995). Involvement of 5-HT1A and 5-HT2 receptors of the inferior colliculus in aversive states induced by exposure of rats to the elevated plus-maze test. Behav Pharmacol 6(4):413–17
- Moreau JL, Borgulya J, Jenck F, Martin JR. (1994). Tolcapone: a potential new antidepressant detected in a novel animal model of depression. Behav Pharmacol 5(3):344–50
- Moreau JL, Bourson A, Jenck F, Martin JR, Mortas P. (1994). Curative effects of the atypical antidepressant mianserin in the chronic mild stress-induced anhedonia model of depression. J Psychiatry Neurosci 19(1):51–6
- Ortolani D, Oyama LM, Ferrari EM, Melo LL, Spadari-Bratfisch RC. (2011). Effects of comfort on food intake, anxiety-like behavior and the stress response in rats. Physiol Behav 103(5):487–92
- Pacák K, Palkovits M. (2001). Stressor specificity of central neuroendocrine responses: implications for stress-related disorders. Endocr Rev 22(4):502–48
- Pecoraro N, Reyes F, Gomez F, Bhargava A, Dallman MF. (2004). Chronic stress promotes palatable feeding, which reduces signs of stress: feedforward and feedback effects of chronic stress. Endocrinology 145(8):3754–62
- Pecoraro N, Gomez F, Dallman MF. (2005). Glucocorticoids dose-dependently remodel energy stores and amplify incentive relativity effects. Psychoneuroendocrinology 30(9):815–25
- Pellow S, Chopin P, File SE, Briley M. (1985). Validation of open: closed arm entries in an elevated plus-maze as a measure of anxiety in the rat. J Neurosci Methods 14(3):149–67
- Prut L, Belzung C. (2003). The open field as a paradigm to measure the effects of drugs on anxiety-like behaviors: a review. Eur J Pharmacol 463:3–33
- Ricart-Jané D, Rodríguez-Sureda V, Benavides A, Peinado-Onsurbe J, López-Tejero MD, Llobera M. (2002). Immobilization stress alters intermediate metabolism and circulating lipoproteins in the rat. Metabolism 51(7):925–31
- Rosmond R, Dallman MF, Björntorp P. (1998). Stress-related cortisol secretion in men: relationships with abdominal obesity and endocrine, metabolic and hemodynamic abnormalities. J Clin Endocrinol Metab 83(6):1853–9
- Sawchenko PE, Brown ER, Chan RK, Ericsson A, Li HY, Roland BL, Kovacs KJ. (1996). The paraventricular nucleus of the hypothalamus and the functional neuroanatomy of visceromotor responses to stress. Prog Brain Res 107:201–22
- Sawchenko P, Li H, Ericcson A. (2000). Circuits and mechanisms governing hypothalamic responses to stress: a tale of two paradigms. Prog Brain Res 122:61–80
- Selye H. (1974). Hormones and nonspecific resistance. Proc Rudolf Virchow Med Soc City NY 28:79–89
- Shimizu N, Oomura, Y, Kai Y. (1989). Stress-induced anorexia in rats mediated by serotonergic mechanisms in the hypothalamus. Physiol Behav 46:835–41
- Teegarden SL, Bale TL. (2007). Decreases in dietary preference produce increased emotionality and risk for dietary relapse. Biol Psychiatry 61(9):1021–9
- Tomiyama AJ, Dallman MF, Epel ES. (2011). Comfort food is comforting to those most stressed: evidence of the chronic stress response network in high stress women. Psychoneuroendocrinology 36(10):1513–19
- Torres SJ, Nowson CA. (2007). Relationship between stress, eating behavior, and obesity. Nutrition 23:887–94
- Vafaei AA, Rashidy-Pour A, Taherian AA. (2008). Peripheral injection of dexamethasone modulates anxiety related behaviors in mice: an interaction with opioidergic neurons. Pak J Pharm Sci 21:285–9
- van Praag HM. (2004). Can stress cause depression? Prog Neuropsychopharmacol Biol Psychiatr 28(5):891–907
- Volkow ND, Wang GJ, Baler RD. (2011). Reward, dopamine and the control of food intake: implications for obesity. Trends Cogn Sci 15(1):37–46
- Woods SC, Seeley RJ, Rushing, PA, D’Alessio D, Tso P. (2003). A controlled high-fat diet induces an obese syndrome in rats. J Nutr 133(4):1081–7
- Yang Y, Zhou L, Gu Y, Zhang Y, Tang J, Li F, Shang W, et al. (2007). Dietary chickpeas reverse visceral adiposity, dyslipidemia and insulin resistance in rats induced by a chronic high-fat diet. Brit J Nutr 98(4):720–6
- Zafar HM, Paré WP, Tejani-Butt SM. (1997). Effect of acute or repeated stress on behavior and brain norepinephrine system in Wistar-Kyoto (WKY) rats. Brain Res Bull 44(3):289–95