Abstract
The prevalence of preterm birth (PTB) is high worldwide, especially in developing countries like Brazil. PTB is marked by a stressful environment in intra- as well as extrauterine life, which can affect neurodevelopment and hormonal and physiological systems and lead to long-term negative outcomes. Nevertheless, little is known about PTB and related outcomes later on in childhood. Thus, the goals of the current study were threefold: (1) comparing cortisol and alpha-amylase (sAA) profiles, including cortisol awakening response (CAR), between preterm and full-term children; (2) evaluating whether preterm children are more responsive to acute stress and (3) assessing their memory skills and emotional and behavioral profiles. Basal cortisol and sAA profiles, including CAR of 30 preterm children, aged 6 to 10 years, were evaluated. Further, we assessed memory functions using the Wide Range Assessment of Memory and Learning, and we screened behavior/emotion using the Strengths and Difficulties Questionnaire. The results of preterm children were compared to an age- and sex-matched control group. One week later, participants were exposed to a standardized laboratory stressor [Trier Social Stress Test for Children (TSST-C)], in which cortisol and sAA were measured at baseline, 1, 10 and 25 min after stressor exposure. Preterm children had higher cortisol concentrations at awakening, a flattened CAR and an exaggerated response to TSST-C compared to full-term children. These alterations were more pronounced in girls. In addition, preterm children were characterized by more emotional problems and poorer memory performance. Our findings illustrate the long-lasting and in part sex-dependent effects of PTB on the hypothalamic–pituitary–adrenal (HPA) axis, internalizing behavior and memory. The findings are in line with the idea that early adversity alters the set-point of the HPA axis, thereby creating a more vulnerable phenotype.
Introduction
Every year, around 15 million babies are born prematurely worldwide. With a prevalence rate of 9.2%, Brazil ranks among the top 10 countries in highest numbers of preterm births (PTB) (Blencowe et al., Citation2012). In spite of this, little is known about the outcomes of prematurely born individuals later on in childhood, especially when it comes to stress vulnerability.
With a multifactorial etiology including infection, preeclampsia and prenatal stress (McElrath et al., Citation2008; Wadhwa et al., Citation2011), PTB can be considered as a developmental risk factor. In addition to the intrauterine adversities and the associated perinatal complications, being born early can lead to extrauterine stress exposure. During neonatal care, most preterm newborns are subjected to repeated skin-breaking procedures, mechanical ventilation and limited contact to the parents (Smith et al., Citation2011). Such early-life adversities can shape the developing brain (Davis et al., Citation2011a) and the developing stress system (Glover et al., Citation2010; Lupien et al., Citation2009), and thus constitute risk factors for cognitive and emotional disorders (Wolf, Citation2008).
Studies of hypothalamic–pituitary–adrenal (HPA) axis function are scarce in preterm individuals and have often focused on infants and adults rather than children. Relative adrenal insufficiency has been described in preterm infants, with a likely switch from HPA axis downregulation to an upregulation at 8 months corrected age (Grunau et al., Citation2007). Whether this HPA dysregulation is sustained later in childhood remains unclear (e.g. see Buske-Kirschbaum et al., Citation2007), although there is some evidence for altered cortisol reactivity in preterm adults (Wüst et al., Citation2005). Additionally, the findings of a handful of studies that investigated cortisol awakening response (CAR) in preterm individuals are mixed (Buske-Kirschbaum et al., Citation2007; Gustafsson et al., Citation2010). For the sympathetic nervous system (SNS), such studies are rather scarce as well (Pyhälä et al., Citation2009).
Seeing that PTB involves potential risk factors for cognition and behavior, what are the main domains affected by it? Cognitive deficits in individuals born prematurely have been reported during infancy (Grunau et al., Citation2009), childhood (Cserjesi et al., Citation2012) and adulthood (Strang-Karlsson et al., Citation2010), even in those without evident brain structure abnormalities (Tanskanen et al., Citation2011). However, few studies have analyzed the effects of PTB on different memory domains (Luu et al., Citation2011), although stress effects have been observed most prominently in the hippocampus and prefrontal cortex (Lupien et al., Citation2009; Wolf, Citation2008). Findings regarding working memory are somewhat conflicting (Jongbloed-Pereboom et al., Citation2012). PTB has also been associated with enhanced susceptibility to the development of psychiatric disorders in adulthood (Nosarti et al., Citation2012).
Based on this, our objective was to investigate the long-term consequences of PTB for stress physiology, emotional well-being and memory performance in children. We wanted to assess the two major stress systems during basal conditions as well as in response to an acute stressor. In addition, a broad cognitive test battery was applied which targeted memory functions known to be influenced by stress hormones. To this end, the present study (1) compared cortisol and alpha-amylase (sAA) profiles between preterm and full-term children; (2) evaluated whether preterm children are more responsive to an acute stressor than their full-term peers and (3) assessed their memory, behavior and emotion. We further examined sex differences based on evidence derived from laboratory animals that the female stress system is more heavily influenced by early-life adversities (Glover & Hill, Citation2012). In humans, Sandman et al. (Citation2013) surveyed evidence that there is a sex-dependent viability–vulnerability tradeoff during early development, with the female offspring being more viable, but also more vulnerable. We hypothesized that preterm children would show altered HPA axis functioning and poorer hippocampus-dependent memory than their full-term peers.
Methods and materials
Participants
Thirty Brazilian preterm school-aged children (6–10 years old), who were born between 26 and 36 weeks of gestation with Apgar at 10 min ≥7, participated in this study. The results of preterm participants were compared with an age- and sex-matched control group, which was composed of 31 full-term children. The length of gestation of each participant was confirmed by their medical records. Participants with any acute, chronic medical problems, intake of medication or immunization within 3 months prior to the study were excluded. All children participated voluntarily, and written informed consent was signed by one parent and by the children. Each child received an attendance certificate and a memory performance report. The study protocol was approved by the local ethics committee.
Procedures
The procedures encompassed two different phases. The first phase included: (1) acquisition of demographic and clinical variables (day 1); (2) acquisition of saliva samples on two consecutive days to determine HPA axis and SNS functioning profiles (days 2 and 3); and finally (3) assessment of memory, behavior and emotion of participants (day 4). In the second phase, which was carried out 1 week later, the cortisol and sAA response to a psychosocial stress paradigm was investigated.
Demographic and clinical variables
The demographic variables were obtained with a semi-structured interview with one of the parents. Socioeconomic status was computed as mother’s and father’s education separately (less than middle school graduate, middle school graduate, some high school, high school graduate, some college, college graduate and post-graduation) and type of schools (public versus private). Additionally, each child was weighed and measured to calculate the body mass index (BMI) ().
Table 1. Sociodemographic comparisons between full-term (n = 31) and preterm children (n = 30).
Furthermore, the length of gestation, the causes for PTB as well as prenatal and postnatal variables were taken from participants’ medical records, except for stress during pregnancy ( and ). To screen prenatal stress, the mothers were asked if they had experienced any adverse events during pregnancy and, if so, to report them. The prenatal stress index for each participant was obtained based on the stress scale developed by Holmes & Rahe (Citation1967), which attributes a specific value to each stressor regarding its impact on life. In accordance with this stress scale, death of a close family member, for example, is scored as 63 points, while change in living conditions is scored as 25 points. The use of tobacco, alcohol and glucocorticoids during pregnancy were coded dichotomously as “yes” or “no”. Regarding their birth weight, the participants were classified as small (SGA), adequate (AGA) or large for gestational age (LGA). Hospitalization time during the neonatal period was used as a postnatal stress measure.
Table 2. Total of preterm births (n = 30) split by related risk factors followed by mood of delivery, according to medical records.
Table 3. Pre-natal and post-natal comparisons between full-term (n = 31) and preterm children (n = 30).
Neuroendocrine profile
Cortisol and sAA profiles were evaluated over the course of two consecutive days. On each day, saliva was sampled at the families’ homes using Salivette sampling devices (Sarstedt, Nümbrecht, Germany) at four time points: right after awakening and 30 min post-awakening to determine the CAR (Pruessner et al., Citation1997), in addition at 1600 h and 2100 h in order to characterize the circadian decline. With one of the parents’ presence, the Salivette was placed into the mouth of the children by a trained psychologist or by their parent, who had specific training and received written instructions for it. Participants were instructed to refrain from brushing their teeth, eating and drinking (except water) 90 min before sampling, and to avoid vigorous physical activity during those 2 days. The saliva samples were stored at −80°C until analysis. Free cortisol levels in nmol/l were measured using an immunoassay (IBL, Hamburg, Germany), while a quantitative enzyme kinetic method described elsewhere (Rohleder & Nater, Citation2009) was used to analyze sAA in U/ml. Intra- and inter-assay coefficients of variation were lower than 10% for both assays.
Psychosocial stress response assessment
Neuroendocrine responses (cortisol and sAA) to psychosocial stress were evaluated using the Trier Social Stress Test for Children (TSST-C; Buske-Kirschbaum et al., Citation1997). As described elsewhere (Quesada et al., Citation2012), participants were asked to create (5 min preparation time) and report (5 min free speech) an exciting ending for a story whose beginning had been presented to them. Afterwards they had to perform a mental arithmetic task (5 min). The tasks had to be performed in front of a camera and a committee composed of two medical students who acted in a neutral and rather reserved fashion. In the mental arithmetic task, children from 6 to 7 years old were instructed to serially subtract the number 3 from 758 as fast and as accurately as possible, while the older ones subtracted the number 7 from 758. The observers were blind for the prematurity status of the participants. The TSST-C session was run between 1330 h and 1800 h to control for possible circadian rhythm effects (Tzortzi et al., Citation2009). Saliva was collected before the beginning of the stressor (baseline), 1, 10, and 25 min post-TSST-C test.
Affective changes before and immediately after TSST-C exposure were assessed with a visual rating scale known as the Self-Assessment Manikin (SAM). Participants were asked to choose one manikin out of each domain (happiness, arousal and dominance) which resembled their current mood state. Each domain is represented by five manikins, each one expressing the following affective states: from a broadly smiling (1 point) to a totally frowning manikin (5 points); from a completely relaxed (1 point) to an anxious manikin (5 points); and finally from a controlling (1 point) to a controlled manikin (5 points) (Bradley & Lang, Citation1994).
Behavioral/emotional profile and memory assessment
To screen the behavioral and emotional profiles of participants, at least one of the parents was asked to fill out the Strengths and Difficulties Questionnaire (SDQ; Goodman, Citation1997), which provides five scales: emotional symptoms, conduct problems, hyperactivity, peer problems and prosocial behavior. Each scale is composed of five items, which are rated on a 3-point scale (not true, somewhat true and certainly true). While the sum of the first four scales provides a total difficulties index, the prosocial scale is the only one that evaluates strengths and competencies (Goodman, Citation1997).
Memory and learning skills were assessed by a trained psychologist using all six core and 7 of the 11 optional subtests of the Wide Range Assessment of Memory and Learning, Second Edition (WRAML2) (Sheslow & Adams, Citation2003). The remaining subtests were excluded from the protocol to avoid the test session being too long. The WRAML2 provides three indexes: a Verbal Memory Index (VbM), a Visual Memory Index (ViM) and an Attention/Concentration Index (ACI), which when combined give an overall General Memory Index (GM). Each index is the result of performance in two subtests: Story Memory (SM) and Verbal Learning (VL) for VbM; Design Memory (DM) and Picture Memory (PM) for ViM; Finger Windows (FW) and Number Letter (NL) for ACI. Besides these general indexes, this study also assessed delayed memory recall (VL recall and SM recall), delayed verbal (VbR; SM Recognition, VL Recognition) and visual recognition (ViR; DM recognition and PM recognition) as well as working memory [Symbolic Working Memory (WM)]. Recall and recognition skills were assessed 30 min post-learning.
Participants’ performances were expressed in age-related scaled scores (from 1 to 19) to allow comparisons among the different tasks, except for delayed recall and WM subtests. Raw scores were used for delayed recall performances (0–16 points for each trial in VL recall; 0–64 for the sum of two stories scores in SM recall). This was done because scaled scores are not available for each of the four learning trials separately in the VL recall subtest and because of our interest in the learning curves. The same decision was made for the WM subtest, since scaled scores are available only for individuals age 9 to adults. Since standards are not available for Brazilian children, we used American norms.
Statistical analyses
The statistical analyses were performed using IBM SPSS Statistics 20. The results of the 30 preterm children were compared with those of 31 full-term children (control group). Initially, one more preterm child was enrolled but she decided not to attend the entire study. Nevertheless, we also ran the same statistical analyses with only 30 rather than 31 participants from the control group (excluding the match for the drop-out participant from the preterm group), and the results were very similar (e.g. the reported significant results remained significant). The results reported in this article refer to the analyses regarding the total sample (30 preterm children and 31 full-term children).
Demographic and clinical comparisons between preterm and full-term children were performed using independent sample t-tests for continuous variables and Pearson’s chi-square test for categorical variables. Since mothers’ and fathers’ education was significantly different between the groups, these variables were included as covariates in all statistical analyses. Behavioral and emotional differences were analyzed with the Mann–Whitney U model, since the Shapiro–Wilk test showed the data were skewed.
For cortisol and sAA profile analyses, the mean of the two consecutive days was calculated at each of the four sampling points ((d1 + d2)/2). One full-term child had awakening cortisol (mean) larger than 3SD units above the mean, and this value was corrected to the control group mean plus 2SD. Differences in neuroendocrine profile (cortisol and sAA), including CAR, between preterm and full-term children as well as their physiological (cortisol and sAA) stress reaction were tested by separate analyses of covariance (ANCOVA) with the repeated measurement factor time and the between-subjects factors group and sex. Affective responses to TSST-C were analyzed using one-ANCOVA for repeated measures (before and after TSST-C) with between-subjects group and sex. Additionally, three separate ANOVAs for repeated measures were performed only for the preterm group to investigate the influence of prenatal glucocorticoids treatment on HPA profile and HPA response to TSST-C later in life. Since some cortisol and sAA data were skewed (p < 0.05), these analyses were performed using log-transformed cortisol [(log(cort + 1)] and sAA [(log(sAA + 1)] values. Degrees of freedom were corrected using Greenhouse–Geisser estimates when the sphericity assumption had been violated.
Based on the structure of WRAML2, the performances of preterm and full-term children were compared following three steps. First, differences in overall indexes scores (VbM, ViM, ACI, GM, VbR and ViR) were evaluated using a multivariate analysis of covariance (MANCOVA) followed by Bonferroni’s adjustment (p < 0.008). Second, three separate MANCOVAs with group as independent variable and the two related subtests as dependent variables were conducted for assessing PTB influence on the core subtests performance. Since only a single working memory subtest was applied, its analysis was performed using an ANCOVA. Third, group differences in recall performances were tested by two separate ANCOVAs, one for each subtest (SM and VL), with the repeated measurement factor trials (learning trials versus delayed recall) and the between-subjects factors group and sex. The same statistical analyses were run for verbal (SM recognition and VL recognition) and visual recognition (DM recognition and PM recognition). To protect against an inflated Type I error rate, Bonferroni’s adjustment was also performed for subtest performances (p < 0.004).
The influence of PTB-related factors on memory performance, emotional/behavioral profile and affective/cortisol response to TSST-C was explored using linear regression analyses. PTB diagnosis (0 versus 1) was forced in as the first step, and the selected PTB-associated factors, i.e., birth weight, Holmes–Rahe index (prenatal stress marker) and hospitalization time (early postnatal stress marker) were entered in a stepwise fashion with a probability of F set to 0.05. The mentioned variables were selected because intrauterine growth restriction and early-life stress (ELS) are a potential risk for emotional, cognitive and stress system development.
Results
Sociodemographic and clinical variables
Preterm and full-term children did not differ in any of the demographic variables except their parents’ education (). In clinical variables, most preterm participants were born to mothers with gestational hypertension (). Most of the deliveries were cesarean sections for both groups (preterm and full-term children). As expected, preterm children showed lower birth size and birth weight, besides a longer hospitalization time. Significant differences were also observed in prenatal glucocorticoid treatment for preterm labor, but not in smoking or alcohol use during pregnancy. Additionally, a trend for higher prenatal stress exposure in preterm children compared to their full-term peers was found ().
Neuroendocrine profile
The cortisol concentrations on awakening of two children (one preterm and one full-term) as well as on 30 min after awakening of one child (preterm) could not be assessed because of insufficient amounts of saliva. Thus, these children were excluded from the day cortisol profile analyses.
Cortisol awakening response (CAR)
A 2 group × 2 sex × 2 times (awakening, +30 min) ANCOVA for repeated measures revealed a significant time (F(1,52) = 8.32, p = 0.006) effect and a PTB × time interaction (F(1,52) = 5.29; p = 0.026) (). Trends for a main effect of sex (F(1,52) = 3.77; p = 0.057) and for a PTB × sex interaction (F(1,52) = 3.43; p = 0.070) were observed. Based on the cited trends and previous reports of sex differences (Jones et al., Citation2006), post-hoc analyses were conducted using group and sex as between-subjects factors. A post-hoc Bonferroni-adjusted univariate analysis showed that preterm children had higher cortisol concentrations right at awakening compared to their full-term peers (F(1,52) = 6.66; p = 0.013). Additionally, differences in awakening cortisol concentrations were more pronounced in girls, with preterm girls displaying higher cortisol immediately at awakening than full-term girls (F(1,52) = 4.43; p = 0.040) as depicted in . In contrast, neither a main effect of PTB (p = 0.71) or sex (p = 0.23) nor a PTB × sex interaction (p = 0.22) were observed at 30 min after waking up. Likewise, no main glucocorticoids treatment effect (p > 0.55) or glucocorticoids treatment × time interaction (p > 0.34) were found between preterm treated (GC) and non-treated with glucocorticoids (GC) prenatally.
Figure 1. (A) Salivary cortisol profile of preterm and full-term children. (B) Salivary cortisol profile of preterm and full-term children split by sex. (C) Salivary alpha-amylase (sAA) profile of preterm and full-term children. Statistical analyses were performed using log-transformed data (log +1). The graphs show the estimated marginal means (raw scores adjusted for covariates) of cortisol concentrations as well as sAA concentrations measured on two consecutive days. Error bars represent standard error of mean (SEM). For cortisol, a significant preterm birth by time interaction and a trend of preterm birth by sex interaction were observed in the conducted analyses. Post-hoc analyses (Bonferroni adjusted for multiple comparisons) revealed that preterm children differed from their full-term peers at awakening cortisol concentrations, with preterm girls displaying significant larger cortisol at awakening than full-term girls. Additionally, preterm children showed an attenuated CAR (see “Results” section for additional information). No sex-differences were found in CAR. *p < 0.05 (post-hoc Bonferroni-adjusted univariate tests).
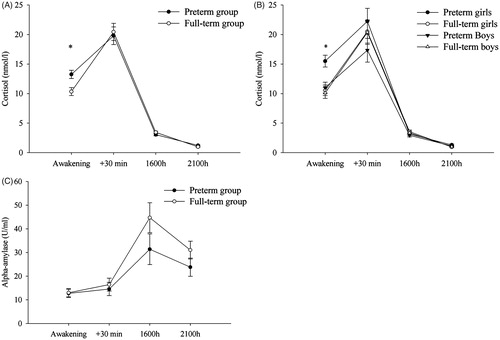
The CAR was computed as the difference between the log of cortisol mean at 30 min after waking up and the log of cortisol mean at awakening (log C+30 mean − log Cawakening mean). ANCOVA with group and sex as between-subjects factors and father’s and mother’s education as covariates revealed a significant preterm effect (F(1,52) = 5.29; p = 0.026). Bonferroni-adjusted pairwise comparison revealed a smaller CAR in preterm children (mean: 6.53 ± 1.39 nmol/l; mean ± SEM) compared to full-term children (SEM; mean: 10.09 ± 1.33 SEM). No differences in CAR (p > 0.34) were observed between the GC and the non-GC preterm groups.
Afternoon/evening cortisol
A 2 group × 2 sex × 2 time (1600 h, 2100 h) ANCOVA revealed a significant time effect (F(1,52) = 40.70, p < 0.001) and a PTB × time (F(1,52) = 4.78; p = 0.033) interaction. However, post-hoc Bonferroni-adjusted univariate analyses failed to show group differences in the afternoon (p = 0.27) or in the evening (p = 0.082) (). Descriptively, preterm children displayed lower cortisol concentrations in the afternoon (mean: 3.05 ± 0.21 SEM) and higher cortisol concentrations in the evening (mean: 1.22 ± 0.09 SEM) than full-term children (mean afternoon: 3.44 ± 0.20 SEM; mean evening: 0.98 ± 0.09 SEM). No main prenatal glucocorticoids treatment effect was found between GC and non-GC preterm groups (p > 0.83).
Alpha-amylase (sAA) profile
Three preterm and three control children were excluded from this analysis due to insufficient saliva. ANCOVA revealed only a non-significant trend of time (p = 0.076) (). Neither a main effect of PTB nor a PTB × time interaction was observed (p > 0.10).
Psychosocial stress test (TSST-C)
Affective response to TSST-C
Here, two full-term children and one preterm were excluded from analyses, because they left the psychosocial stress test in the preparation phase. Another preterm child failed to show up at the TSST-C appointments. Significant stress-induced changes over time in valence (F(1,51) = 4.13, p = 0.047) and arousal (F(1,51) = 5.56, p = 0.022) were found, but not in dominance (p = 0.94). Importantly, preterm and full-term children did not differ in their affective response to the stressor (p > 0.17, data not shown).
Neuroendocrine response to TSST-C
One more participant was excluded from this analysis due to an insufficient amount of saliva at time point C+10. A 2 group × 2 sex × 4 time ANCOVA for repeated measures with mother’s and father’s education as covariates showed significant stress-induced changes over time (F(1.41,70.41) = 3.59, p = 0.048), a main PTB effect (F(1,50) = 8.86, p = 0.004), a PTB × time interaction (F(1.41,70.41) = 3.73, p = 0.043) and a trend of a PTB × sex × time interaction (F(1.41,70.41) = 3.15, p = 0.066). Preterm children had higher cortisol concentrations after TSST-C, i.e. at the +1 (p = 0.008), +10 (p = 0.018) and +25 sampling (p = 0.009), but not at baseline (p = 0.194), as depicted in . Comparing cortisol increase (log cortisol+10 − minus log cortisolbaseline) by using a Bonferroni-adjusted univariate analysis, preterm girls showed a higher increment than preterm boys (p = 0.03) ().
Figure 2. (A) Salivary cortisol in response to the Trier Social Stress Test for Children (TSST-C) for preterm and full-term children. (B) TSST-C results from preterm and full-term children split by sex. (C) sAA response to the TSST-C. Statistical analyses were performed using log-transformed data (log +1). The graphs show the estimated marginal means (adjusted raw data for covariates). Error bars represent standard error of mean (SEM). Stress-induced cortisol changes over time, and a significant main preterm birth effect was found using Bonferroni adjusted analyses. Furthermore, such effect was more pronounced in girls, with preterm girls showing significant larger cortisol response to TSST-C than full-term girls. (A) *p < 0.05, **p < 0.01 between preterm and full-term children and (B) *p < 0.05, **p < 0.01 (post-hoc Bonferroni-adjusted univariate tests) between preterm girls and full-term girls.
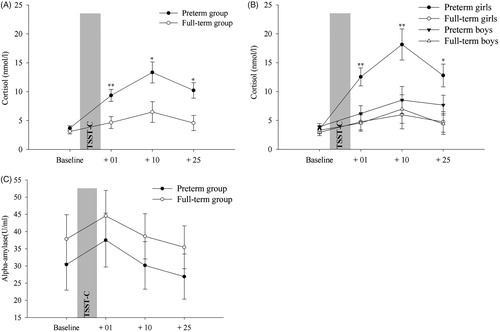
Contrary to the cortisol response, no significant effects or interactions were noted for sAA (p > 0.05) (). Additionally, for preterm children only, neither a main prenatal glucocorticoids treatment effect (p > 0.82) nor a prenatal glucocorticoids treatment × time interaction was found (p > 0.67) in cortisol response to TSST-C.
Behavioral profile (SDQ)
Most questionnaires were filled out by mothers for both groups. For preterm children, 93.3% of questionnaires were filled out only by mothers and 6.7% by fathers (alongside mothers). For full-term children, 83.9% of questionnaires were filled out only by mothers and 16.1% by fathers (alongside mothers or themselves). Chi-square test revealed that the number of questionnaires filled out by mothers and/or fathers did not differ between the two groups (p = 0.25). Furthermore, a t-test with emotional problems as the dependent variable and who filled out the questionnaires as the group variable showed no impact of who filled out the questionnaires on emotional problems (t = 0.48; p = 0.63).
In the SDQ, Mann–Whitney U test revealed that preterm (median = 5.0) and full-term children (median = 4.0) differed in emotional symptoms (z = −2.1; p = 0.037), which were higher in preterm children.
Cognitive profile (WRAML2 performance)
On the Wide Range Assessment of Memory and Learning performance (WRAML2), post-hoc Bonferroni-corrected analyses revealed that the preterm children showed lower general memory (p = 0.006) and visual memory indexes (p = 0.003) than full-term children, but they did not differ in the other two summary indexes, i.e. attention/concentration and delayed verbal and visual recognition (p > 0.21). Nevertheless, note the trend (p = 0.066) in the verbal memory index. For the subtests, significant differences were observed in the Story Memory and Picture Memory subtests and a trend emerged in the Design Memory subtest, with lower performance in preterm children ().
Table 4. WRAML2 performance and group comparisons.
In the delayed retrieval tasks, main PTB effects were found in both recall subtests, i.e. Verbal Learning (F(1,55) = 5.27; p = 0.025) and Story Memory (F(1,55) = 8.98; p = 0.004), but not in recognition (p > 0.15). A post-hoc Bonferroni-corrected univariate test showed that the preterm children recalled fewer words 30 min post-learning (mean: 6.90 ± 0.43 SEM) than control participants (mean: 9.26 ± 0.46 SEM) (p = 0.005), as shown in . They also recalled fewer details of episodes from the two stories in the delayed Story Memory recall subtest (p = 0.043) (). No PTB × sex interaction was found (p > 0.18). Mothers’ education influence was observed only in the Number Letter subtest performance (F(1,57) = 6.90, p = 0.011), with higher scores shown by children whose mothers’ education was higher. WRAML2 performance with Bonferroni-adjusted p values is depicted in .
Influence of PTB-related factors on memory performance, emotional/behavioral profile, affective and cortisol response to TSST-C
The influence of birth weight, prenatal stress (Holmes–Rahe index) and hospitalization time on emotional problems, memory performance (general memory and delayed recall) and affective/cortisol response to TSST-C was explored using regression analyses. In the analysis of general memory as the dependent variable, only birth weight emerged as a predictor, significantly adding to the variance explained by PTB (ΔR2 = 0.07, p = 0.013, β = 0.45). For delayed recall performance, emotional problems and affective/cortisol response to TSST-C as separate dependent variables, the selected factors did not add beyond diagnosis (preterm birth versus birth at term).
Regarding our interest in the association between birth weight as well as length of gestation and the observed emotional, memory and cortisol response to TSST-C (AUCi) outcomes in preterm children, Pearson correlations were performed within the preterm group only (n = 30). Birth weight was associated positively with the general memory index (r = 0.36; p = 0.048), but no significant correlations were observed for length of gestation. In addition, higher cortisol response to TSST-C was shown by preterm children classified as small for gestational age (r = −0.42; p = 0.025). It is also worth noting the positive correlation between the prenatal stress marker (r = 0.47; p = 0.009) and emotional symptoms, and the negative association between length of gestation and hospitalization time (r = −0.68; p < 0.001).
Furthermore, in accordance with exploratory analyses (ANCOVA), no effects of different etiologies leading to premature delivery were observed on the general memory (p = 0.36) index or on emotional problems (p = 0.57).
Discussion
The present study demonstrates HPA alterations, increased emotional problems and poorer memory in preterm individuals tested later on in childhood. There were some sex-specific differences, with preterm girls showing higher cortisol concentrations right at awakening and a higher cortisol response to TSST-C than preterm boys. In addition to poorer general memory, preterm children also showed lower scores in visual memory and delayed verbal recall tasks. No significant performance differences were found in attention, working memory or delayed recognition.
Preterm children had higher cortisol concentrations right at awakening followed by flattened CAR. In line with our findings, higher cortisol concentrations right at awakening were also reported in 8- to 14-year-old preterm children (Buske-Kirschbaum et al., Citation2007) and in 7- to 9-year-old term girls with lower birth weight (Jones et al., Citation2006). Different from our findings and consistent with the scarce studies investigating CAR in preterm or/and low birth weight children (Gustafsson et al., Citation2010; Jones et al., Citation2006), Buske-Kirschbaum et al. (Citation2007) failed to find a significant PTB influence on the CAR.
Nevertheless, attenuated CAR later in life has been observed in individuals exposed to early trauma (Mangold et al., Citation2010) or with an anxious attachment style (Oskis et al., Citation2011), stressors also related to PTB. Finally, similar CAR alterations, i.e. higher cortisol concentrations at awakening and reduced CAR were found recently in pregnant women who delivered an infant prematurely (Entringer et al., Citation2011) and in 9- to 18-year-old girls with an anxious attachment style (Oskis et al., Citation2011). The similarities of CAR profile between our participants and those from the cited studies suggest that at least part of the HPA dysregulation observed in preterm individuals might be a programming effect of adverse events experienced prenatally or during the Neonatal Intensive Care Unit (NICU) stay. Nevertheless, it has to be emphasized that other factors related to PTB might also explain the HPA alterations observed in the current study.
Even though preterm and full-term children did not differ in their affective response to the TSST-C, a stronger cortisol increase was observed in preterm children. Our findings are in line with previous studies, which have observed exaggerated HPA responses to psychosocial stress in low birth weight phenotype in 7- to 9-year-old boys (Jones et al., Citation2006) and young adults (Wüst et al., Citation2005). Additionally, an exaggerated response to TSST-C has been associated with prenatal stress (Entringer et al., Citation2009). In contrast, Buske-Kirschbaum et al. (Citation2007) failed to find differences in stress reactivity in 8- to 14-year-old preterm children.
Although findings suggest that elevated prenatal exposure to synthetic glucocorticoids is associated with a larger cortisol response to a stressor even in full-term infants (Davis et al., Citation2011b), no differences were observed on cortisol measures between preterm children who were exposed prenatally to glucocorticoids and those who were not. However, it is important to emphasize that only eight children in our sample were exposed to glucocorticoids during prenatal time. Our study is therefore underpowered to address this issue.
With respect to SNS, some previous evidence of elevated SNS activity in response to TSST-C has been observed in former preterm adults (Pyhälä et al., Citation2009). However, in the present study, preterm and full-term participants did not differ in sAA response to TSST-C. Taken together, these findings suggest that preterm individuals are more sensitive to acute stressors throughout adulthood. This might put them at an increased risk of developing psychopathologies.
Increased emotional problems in preterm participants were another important finding, corroborating most previous studies (Arpi & Ferrari, Citation2013). It is worth emphasizing that, within preterm group analyses, a higher prenatal stress marker index (Holmes–Rahe) was associated with more pronounced emotional symptoms in childhood. Amygdala and prefrontal cortex dysfunctions due to early-life adversities exposure are potential neural correlates of these emotional problems (Buss et al., Citation2010, Citation2012).
Further, in line with some previous studies, preterm children had deficits in general and visual memory (Baron et al., Citation2012) and in delayed memory recall (Rose et al., Citation2005). Hippocampal atrophy or dysfunctions are possible neural correlates of recall deficits (McClelland et al., Citation2011). No impairments were observed in the recognition domain, which is less reliant on the hippocampus and more perirhinal-cortex dependent (Ford et al., Citation2010). Thus, the impairments observed in recall, but not in recognition, suggest that the hippocampus is more sensitive to prematurity and its associated factors than other medial temporal lobe regions such as the perirhinal cortex. In contrast to some previous studies (Aarnoudse-Moens et al., Citation2012; Luu et al., Citation2011), preterm and full-term children did not differ significantly in verbal working memory performance. However, the findings in this field are somewhat mixed (De Kieviet et al., Citation2012; Sansavini et al., Citation2007). Thus, our data suggest that unlike the hippocampus, the effects of PTB and related factors on the prefrontal cortex are not evident in childhood. It might be that incubated effects emerge once the prefrontal cortex undergoes major development during adolescence (Lupien et al., Citation2009). Interestingly, we found in a previous study (Quesada et al., Citation2012) that acute stress impaired memory retrieval (thought to rely on the hippocampus), but had no effect on working memory (thought to rely on the PFC). Taken together, our results as well as previous work from other labs might suggest that the hippocampus is more sensitive to acute and chronic stress during childhood, relative to the PFC.
In light of the existing literature, the observed negative outcomes in preterm children might be a result of an immature brain being overexposed to intrauterine and extrauterine adversities. Malnutrition, hypertension, prenatal stress, use of synthetic glucocorticoids in pregnancy, perinatal complications, short length of gestation, low birth weight, the exposure to several stressors in NICU and other PTB-related factors can shape the development of the brain (hippocampus, amygdala and prefrontal cortex) and stress system development. Supporting this programming model, fetal exposure to stress has been associated with reduced hippocampal volumes (Sandman et al., Citation2011), and elevated exposure to stressors in the NICU has been linked to structural alterations and dysfunctions of the temporal lobes (Smith et al., Citation2011). Moreover, a compelling body of research has shown associations between chronically altered cortisol and memory impairments (Wolf, Citation2009). The influence of early adversities exposure on the methylation of the glucocorticoids receptor gene in the hippocampus (Meaney, Citation2010) is one plausible mechanism to explain these programming effects.
In line with this and with the findings of Raz et al. (Citation2012), in the present study, birth weight had a strong influence on memory performance, significantly adding to the variance explained by PTB diagnosis. In contrast, prenatal stress and hospitalization time were not predictors for the observed negative outcomes. However, within the preterm group analyses, the prenatal stress marker was associated with emotional symptoms.
In exploratory analyses about the underlying conditions leading to PTB, we did not observe differential effects of them on memory, emotional problems and the stress system. In line with our findings, pregnancy-induced hypertension, which was one of the main causes of PTB in our study, was not associated with childhood development (Love et al., Citation2012). In contrast, Many et al. (Citation2003) found IQ differences between children born from mothers with preeclampsia and without preeclampsia, although no differences were found in a neurological exam. Seeing that our sample might be too small to detect the influence of PTB causes on offspring’s outcomes, larger studies are warranted.
Supporting our findings about sex differences in preterm children, animal and human studies suggest that females exposed to prenatal adversity or glucocorticoid therapy are more responsive to stressors later in life than males (Alexander et al., Citation2012; Glover & Hill, Citation2012). A recent study reported a link between higher maternal cortisol concentration in earlier gestation and larger right amygdala volume and affective problems in girls, but not in boys (Buss et al., Citation2012). Evidence suggests that 11β-hydroxysteroid dehydrogenase type 2 enzyme activity, a protecting barrier for the fetus from elevated maternal cortisol, is more affected by stress in the female than in the male fetus (Clifton, Citation2005), which might explain why girls appear to be more susceptible to early-life adversities than boys. Our findings are in line with recent evidence indicating that girls are more prone to become anxious and fearful in response to elevated maternal cortisol concentrations [reviewed by Sandman et al. (Citation2013)].
The strength of our study was the broad approach, investigating simultaneously the long-term consequences of PTB on the HPA axis and SNS functioning, different memory domains and internalizing/externalizing behavior. However, some limitations of our study need to be addressed as well. First, the retrospective nature of our study and the overlapping variables do not allow us to establish causal effects. The main causes for the later outcomes found in the preterm participants should be investigated in future longitudinal studies. Second, the preterm and full-term groups were not homogeneous in terms of father’s and mother’s education. To control for this limitation, these variables were included as covariates in the statistical analyses. Third, prenatal stress was based on retrospective maternal self-report, which could compromise the data accuracy. Fourth, we did not obtain any measure on maternal psychological function, neither prenatally nor at the time of our study. Fifth, the investigation of mother–offspring interaction would have also been informative. Last but not least a neuroimaging component would have been informative to investigate hippocampal integrity.
In sum, our results illustrate the long-term consequences of PTB on HPA axis functioning, internalizing behavior and memory later on in childhood. The HPA alterations were sexually dimorphic with girls showing more pronounced alterations than boys. Regarding memory functions, delayed recall and visual memory were the domains most affected. Such findings suggest that preterm individuals are a more vulnerable phenotype, being at an increased risk for cognitive impairments and psychiatric disorders throughout life. They also open windows for investigating the main causes of the observed outcomes.
Declaration of interest
The authors declare that they have no conflict of interest regarding this manuscript. The research was financially supported by a grant from the German Research Foundation (DFG). Furthermore, part of it was supported by a scholarship from Coordenação de Aperfeiçoamento de Pessoal (CAPES), Brazil. Both funding sources had no further role in the study design, the collection, analysis and interpretation of data, the writing of the report and the decision to submit the paper for publication.
Acknowledgements
We gratefully acknowledge Caroline N. Minchillo, Fernanda B. Vianna, Igor C. Carneiro, and Lucas B. D. Barbosa for being part of TSST-C committee (School of Medicine, University of Brasília). We are also grateful to Rosângela C. Marinho (neuropediatrician of Hospital Regional da Asa Sul – HRAS) and Mônica L. Lemos (physiotherapist of Hospital Regional da Asa Sul – HRAS and of Hospital da Universidade de Brasília- HUB) for their assistance in the recruitment of preterm children. Additionally we thank Eve-Mariek Hessas for language editing. Finally, we gratefully acknowledge all children and their parents for their engagement in the present study.
References
- Aarnoudse-Moens CSH, Duivenvoorden HJ, Weisglas-Kuperus N, Van Goudoever JB, Oosterlaan J. (2012). The profile of executive function in very preterm children at 4 to 12 years. Dev Med Child Neurol 54:247–53
- Alexander N, Rosenlöcher F, Stalder T, Linke J, Distler W, Morgner J, Kirschbaum C. (2012). Impact of antenatal synthetic glucocorticoid exposure on endocrine stress reactivity in term-born children. J Clin Endocrin Metab 97:3538–44
- Arpi E, Ferrari F. (2013). Preterm birth and behaviour problems in infants and preschool-age children: a review of the recent literature. Dev Med Child Neurol 55:788–96
- Baron IS, Litman FR, Ahronovich MD, Baker R. (2012). Late preterm birth: a review of medical and neuropsychological childhood outcomes. Neuropsychol Rev 22:438–50
- Blencowe H, Cousens S, Oestergaard MZ, Chou D, Moller A-B, Narwal R, Adler A, et al. (2012). National, regional, and worldwide estimates of preterm birth rates in the year 2010 with time trends since 1990 for selected countries: a systematic analysis and implications. Lancet 379:2162–72
- Bradley MM, Lang PJ. (1994). Measuring emotion: the self-assessment Manikin and the semantic differential. J Behav Ther Exp Psychiatry 25:49–59
- Buske-Kirschbaum A, Jobst S, Wustmans A, Kirschbaum C, Rauh W, Hellhammer D. (1997). Attenuated free cortisol response to psychosocial stress in children with atopic dermatitis. Psychosom Med 59:419–26
- Buske-Kirschbaum A, Krieger S, Wilkes C, Rauh W, Weiss S, Hellhammer DH. (2007). Hypothalamic-pituitary-adrenal axis function and the cellular immune response in former preterm children. J Clin Endocrinol Metab 92:3429–35
- Buss C, Davis EP, Muftuler LT, Head K, Sandman CA. (2010). High pregnancy anxiety during mid-gestation is associated with decreased gray matter density in 6–9-year-old children. Psychoneuroendocrinology 35:141–53
- Buss C, Davis EP, Shahbaba B, Pruessner JC, Head K, Sandman CA. (2012). Maternal cortisol over the course of pregnancy and subsequent child amygdala and hippocampus volumes and affective problems. Proc Natl Acad Sci USA 109:E1312–19
- Clifton VL. (2005). Sexually dimorphic effects of maternal asthma during pregnancy on placental glucocorticoid metabolism and fetal growth. Cell Tissue Res 322:63–71
- Cserjesi R, Van Braeckel KN, Butcher PR, Kerstjens JM, Reijneveld SA, Bouma A, Geuze RH, et al. (2012). Functioning of 7-year-old children born at 32 to 35 weeks’ gestational age. Pediatrics 130:838–46
- Davis EP, Buss C, Muftuler LT, Head K, Hasso A, Wing DA, Hobel C, et al. (2011a). Children’s brain development benefits from longer gestation. Front Psychol 2:1
- Davis EP, Waffarn F, Sandman CA. (2011b). Prenatal treatment with glucocorticoids sensitizes the hpa axis response to stress among full-term infants. Dev Psychobiol 53:175–83
- De Kieviet JF, van Elburg RM, Lafeber HN, Oosterlaan J. (2012). Attention problems of very preterm children compared with age-matched term controls at school-age. J Pediatr 161:824–9
- Entringer S, Buss C, Andersen J, Chicz-DeMet A, Wadhwa PD. (2011). Ecological momentary assessment of maternal cortisol profiles over a multiple-day period predicts the length of human gestation. Psychosom Med 73:469–74
- Entringer S, Kumsta R, Hellhammer DH, Wadhwa P, Wüst S. (2009). Prenatal exposure to maternal psychosocial stress and HPA axis regulation in young adults. Horm Behav 55:292–8
- Ford JH, Verfaellie M, Giovanello KS. (2010). Neural correlates of familiarity-based associative retrieval. Neuropsychologia 48:3019–25
- Glover V, Hill J. (2012). Sex differences in the programming effects of prenatal stress on psychopathology and stress responses: an evolutionary perspective. Physiol Behav 106:736–40
- Glover V, O’Connor TG, O’Donnell K. (2010). Prenatal stress and the programming of the HPA axis. Neurosci Biobehav Rev 35:17–22
- Goodman R. (1997). The strengths and difficulties questionnaire: a research note. J Child Psychol Psychiatry 38:581–6
- Grunau RE, Haley DW, Whitfield MF, Weinberg J, Yu W, Thiessen P. (2007). Altered basal cortisol levels at 3, 6, 8 and 18 months in infants born at extremely low gestational age. J Pediatr 150:151–6
- Grunau RE, Whitfield MF, Petrie-Thomas J, Synnes AR, Cepeda IL, Keidar A, Rogers M, et al. (2009). Neonatal pain, parenting stress and interaction, in relation to cognitive and motor development at 8 and 18 months in preterm infants. Pain 143:138–46
- Gustafsson PE, Janlert U, Theorell T, Hammarström A. (2010). Is body size at birth related to circadian salivary cortisol levels in adulthood? Results from a longitudinal cohort study. BMC Publ Health 10:346
- Holmes TH, Rahe RH. (1967). The social readjustment rating scale. J Psychosom Res 11:213–18
- Jongbloed-Pereboom M, Janssen AJ, Steenbergen B, Nijhuis-van der Sanden MW. (2012). Motor learning and working memory in children born preterm: a systematic review. Neurosci Biobehav 36:1314–30
- Jones A, Godfrey KM, Wood P, Osmond C, Goulden P, Phillips DI. (2006). Fetal growth and the adrenocortical response to psychological stress. J Clin Endocrinol Metab 91:1868–71
- Love ER, Crum J, Bhattacharya S. (2012). Independent effects of pregnancy induced hypertension on childhood development: a retrospective cohort study. Eur J Obstet Gynecol Reprod Biol 165:219–24
- Lupien SJ, McEwen BS, Gunnar MR, Heim C. (2009). Effects of stress throughout the lifespan on the brain, behaviour and cognition. Nat Rev Neurosci 10:434–45
- Luu TM, Ment L, Allan W, Schneider K, Vohr BR. (2011). Executive and memory function in adolescents born very preterm. Pediatrics 127:639–46
- Mangold D, Wand G, Javors M, Mintz J. (2010). Acculturation, childhood trauma and the cortisol awakening response in Mexican-American adults. Horm Behav 58:637–46
- Many A, Fattal A, Leitner Y, Kupferminc MJ, Harel S, Jaffa A. (2003). Neurodevelopmental and cognitive assessment of children born growth restricted to mothers with and without preeclampsia. Hypertens Pregnancy 22:25–29
- McClelland S, Korosi A, Cope J, Autumn I, Baram TZ. (2011). Emerging roles of epigenetic mechanisms in the enduring effects of early-life stress and experience on learning and memory. Neurobiol Learn Mem 96:79–88
- McElrath TF, Hecht JL, Dammann O, Boggess K, Onderdonk A, Markenson G, Harper M, et al. (2008). Pregnancy disorders that lead to delivery before the 28th week of gestation: an epidemiologic approach to classification. Am J Epidemiol 168:980–9
- Meaney MJ. (2010). Epigenetics and the biological definition of gene x environment interactions. Child Dev 81:41–79
- Nosarti C, Reichenberg A, Murray RM, Cnattingius S, Lambe MP, Yin L, MacCabe J, et al. (2012). Preterm birth and psychiatric disorders in young adult life. Arch Gen Psychiatry 69:1–8
- Oskis A, Loveday C, Hucklebridge F, Thorn L, Clow A. (2011). Anxious attachment style and salivary cortisol dysregulation in healthy female children and adolescents. J Child Psychol Psychiatry 52:111–18
- Pruessner JC, Wolf OT, Hellhammer DH, Buske-Kirschbaum A, von Auer K, Jobst S, Kaspers F, et al. (1997). Free cortisol levels after awakening: a reliable biological marker for the assessment of adrenocortical activity. Life Sci 61:2539–49
- Pyhälä R, Räikkönen K, Feldt K, Andersson S, Hovi P, Eriksson JG, Järvenpää A-L, et al. (2009). Blood pressure responses to psychosocial stress in young adults with very low birth weight: Helsinki study of very low birth weight adults. Pediatrics 123:731–4
- Quesada AA, Wiemers US, Schoofs D, Wolf OT. (2012). Psychosocial stress exposure impairs memory retrieval in children. Psychoneuroendocrinology 37:125–36
- Raz S, Debastos AK, Newman JB, Batton D. (2012). Intrauterine growth and neuropsychological performance in very low birth weight preschoolers. J Int Neuropsychol 18:200–11
- Rohleder N, Nater U. (2009). Determinants of salivary alpha-amylase in humans and methodological considerations. Psychoneuroendocrinology 34:469–85
- Rose SA, Feldman JF, Jankowski JJ. (2005). Recall memory in the first three years of life: a longitudinal study of preterm and term children. Dev Med Child Neurol 47:653–9
- Sandman CA, Davis EP, Buss C, Glynn LM. (2011). Prenatal programming of human neurological function. Int J Pept 2011:837596
- Sandman CA, Glynn LM, Davis EP. (2013). Is there a viability–vulnerability tradeoff? Sex differences in fetal programming. J Psychosom Res 75:327–35
- Sansavini A, Guarini A, Alessandroni R, Faldella G, Giovanelli G, Salvioli G. (2007). Are early grammatical and phonological working memory abilities affected by preterm birth? J Commun Disord 40:239–56
- Sheslow D, Adams W. (2003). Wide range assessment of memory and learning, 2nd ed. Administration and Technical Manual. Wilmington, DE: Wide Range, Inc
- Smith GC, Gutovich J, Smyser C, Pineda R, Newnham C, Tjoeng TH, Vavasseur C, et al. (2011). Neonatal intensive care unit stress is associated with brain development in preterm infants. Ann Neurol 70:541–9
- Strang-Karlsson S, Andersson S, Paile-Hyvärinen M, Darby D, Hovi P, Räikkönen K, Pesonen AK, et al. (2010). Slower reaction times and impaired learning in young adults with birth weight <1500 g. Pediatrics 125:74–82
- Tanskanen P, Valkama M, Haapea M, Barnes A, Ridler K, Miettunen J, Murray GK, et al. (2011). Is prematurity associated with adult cognitive outcome and brain structure? Pediatr Neurol 44:12–20
- Tzortzi Ch Proff P, Redlich M, Aframian DJ, Palmon A, Golan I, Muessig D, et al. (2009). Cortisol daily rhythm in saliva of healthy school children. Int Dent J 59:12–18
- Wadhwa PD, Entringer S, Buss C, Lu MC. (2011). The contribution of maternal stress to preterm birth: issues and considerations. Clin Perinatol 38:51–84
- Wolf OT. (2008). The influence of stress hormones on emotional memory: relevance for psychopathology. Acta Psychol (Amst) 127:513–31
- Wolf OT. (2009). Stress and memory in humans: twelve years of progress? Brain Res 1293:142–54
- Wüst S, Entringer S, Federenko IS, Schlotz W, Hellhammer DH. (2005). Birth weight is associated with salivary cortisol responses to psychosocial stress in adult life. Psychoneuroendocrinology 30:591–8