Abstract
Either pre- or post-natal environmental factors seem to play a key role in brain and behavioral development and to exert long-term effects. Increasing evidence suggests that exposure to prenatal stress (PS) leads to motor and learning deficits and elevated anxiety, while enriched environment (EE) shows protective effects. The dopaminergic system is also sensitive to environmental life circumstances and affects attention functioning, which serves as the preliminary gate to cognitive processes. However, the effects of methylphenidate (MPH) on the dopaminergic system and attentional functioning, in the context of these life experiences, remain unclear. Therefore, we aimed to examine the effects of EE or PS on distinct types of attention, along with possible effects of MPH exposure. We found that PS impaired selective attention as well as partial sustained attention, while EE had beneficial effects. Both EE and MPH ameliorated the deleterious effects of PS on attention functioning. Considering the possible psychostimulant effect of MPH, we examined both anxiety-like behavior as well as motor learning. We found that PS had a clear anxiogenic effect, whereas EE had an anxiolytic effect. Nevertheless, the treatment with both MPH and/or EE recovered the deleterious effects of PS. In the motor-learning task, the PS group showed superior performance while MPH led to impaired motor learning. Performance decrements were prevented in both the PS + MPH and EE + MPH groups. This study provides evidence that peripubertal exposure to EE (by providing enhanced sensory, motor, and social opportunities) or MPH treatments might be an optional therapeutic intervention in preventing the PS long-term adverse consequences.
Introduction
Environmental factors seem to play a key role in brain and behavioral development, both in humans and in animals. Different environmental manipulations, either pre- or post-natal, have been shown to exert long-term physiological and behavioral effects.
It is well documented that prenatal stress (PS) exerts a strong impact on fetal brain development in experimental animal models (Weinstock, Citation2001). In the last few years, numerous studies have demonstrated that exposure to PS leads to a delay in motor development, impaired adaptation to stressful conditions, altered sexual behavior, and learning deficits (Darnaudery et al., Citation2008; Huizink et al., Citation2004; Weinstock, Citation2001).
In contrast to the deleterious effects of PS, accumulated data on environmental enrichment (EE) showed the rehabilitative or protective effects of EE in different animal models (Guilarte et al., Citation2003; Hellemans et al., Citation2004; Ilin et al., Citation2009).
The EE comprises an alteration to the animal’s home cage or secondary exploratory area, which provides enhanced sensory, motor, cognitive, and social opportunities. The EE large space, within which the animal experiences exploration and introduction to a variety of objects, varying in shape, size, weight, smell, and texture, renders stimulation of the various sensory modalities. In addition, EE animals are kept as a group and have the opportunity for complex social interaction as well as have ample space for exercise (Cymerblit-Sabba et al., Citation2013; Nithianantharajah et al., Citation2006).
EE has been shown to increase dendritic arborization (Greenough & Volkmar, Citation1973), enhance performance of learning and memory tasks (Fares et al., Citation2013; Pamplona et al., Citation2009), improve encoding of information related to object recognition and spatial maps (Nithianantharajah et al., Citation2006), improve motor function (Seo et al., Citation2013), exert neuroprotective effects (Wright et al., Citation2008), and decrease levels of anxiety-like behavior (Fares et al., Citation2013; Urakawa et al., Citation2013). EE was also found to rescue abnormal behaviors, such as emotional reactivity and spatial learning, as well as motor skills deficits, all induced by PS (Chapillon et al., Citation2002; Lemaire et al., Citation2006; Morley-Fletcher et al., Citation2003).
Dopamine (DA) is the predominant catecholamine neurotransmitter in the mammalian brain, where it controls a variety of functions including locomotor activity, cognition, emotion, positive reinforcement, food intake, and endocrine regulation (Missale et al., Citation1998). Evidence provided by animal research, as well as retrospective studies in humans, indicates that the exposure to adverse events in early life can alter adult behaviors and neurochemical indicators of DA activity. This suggests that the development of the DA system is sensitive to disruption by exposure to early life experiences. PS increases DA D2 receptors in limbic areas, increases DA-stimulated release in the nucleus accumbens, while decreases it in cortical areas. PS also exerts an inhibitory effect on general levels of DA at some unknown stage of DA biosynthesis and disrupts the DA-glutamate balance (see Baier et al., Citation2012 for review).
However, EE has been shown to decrease basal DA concentration in the striatum (Bowling et al., Citation1993), increase dopamine clearance from the medial prefrontal cortex (Neugebauer et al., Citation2004), and enhance dopaminergic neuron migration from the midbrain to the striatum (Urakawa et al., Citation2007). Thus, the basal tone of dopaminergic system can be significantly influenced by environmental variables.
In humans, dysfunction of dopaminergic system is associated attention-deficit hyperactivity disorder (ADD/ADHD) (Baier et al., Citation2012). Methylphenidate (MPH; Ritalin©) is commonly prescribed for the treatment of ADD/ADHD, a psychiatric disorder that is more common during early life, i.e. childhood and early adulthood. MPH inhibits DA and norepinephrine (NE) transporters, thereby increasing the extra-cellular concentration of these neurotransmitters (Anderson et al., Citation2006; Hannestad et al., Citation2010; Volkow et al., Citation1998). Although there are several reports investigating the effects of the acute administration of MPH on animals reared under different life circumstances (Perry et al., Citation2008; Wooters et al., Citation2011), the effects of chronic treatment remain largely unexplored. Moreover, the vast majority of MPH literature is focused on ADD/ADHD, while less attention has been provided regarding life circumstances and types of attention.
Considering both early life propitious and adverse experiences, in the current study, we aimed to examine the effects of positive (i.e. environmental enrichment) or negative (i.e. PS) early life conditions on distinct types of attention. We also examined the possible mediating and/or moderating effects of MPH on the consequences of the aforementioned life experiences.
Materials and methods
Animals
Thirty pregnant female Wistar rats (at day 8 of pregnancy weighing between 260 and 290 g) were purchased from Harlan (Jerusalem, Israel). Rats were housed in standard plastic cages (30 cm × 30 cm × 18 cm) with sterilized sawdust bedding. All offspring were weaned at post natal day (PND) 21 and only male rats were included in the experiment. It should be emphasized that in order to maintain genetic variability, male siblings were randomly allocated to different experimental groups.
Room temperature was maintained at 23 ± 1 °C with 67% humidity at 12:12 d/night cycle (lights on at 07:00). Food and water access was allowed ad libitum. This study was carried out in strict accordance with the recommendations of the Guide for the Care and Use of Laboratory Animals of the National Institutes of Health. The protocol was approved by the Institutional Animal Care and Use Committee. All efforts were made to minimize animal suffering.
Procedure
At days 13–15 of pregnancy, half of the females were exposed to stress and their offspring were included in the PS groups. The other females remained in their home cages and their offspring were included in all other groups. At PND 21, male offspring were separated from their dams and reared in standard conditions (four rats per cage in the same conditions described in the above-mentioned “Animals” section).
The experiment included eight groups: control, PS, enriched environment (EE), PS + EE, MPH, PS + MPH, EE + MPH, and PS + EE + MPH (N = 12 in each group). At PND 30–60, rats in the EE groups (i.e. EE, PS + EE, EE + MPH, and PS + EE + MPH) were housed in EE cages. At PND 60–90, rats in the MPH groups (i.e. MPH, PS + MPH, EE + MPH, and PS + EE + MPH) were treated with MPH. Five days later (at PND 95), following the clearance of MPH from the CNS (Aoyama et al., Citation1990; Devilbiss et al., Citation2006; Wargin et al., Citation1983), rats were tested for two types of attention: selective attention tested in the object recognition (OR) task (Braida et al., Citation2013; Levin et al., Citation2011) and partial sustained attention tested in the pre-pulse inhibition (PPI) test (Zubedat et al., Citation2014). In order to monitor changes in anxiety-like behaviors, rats were tested in the open field (OF) and startle response tests. Since MPH may exert effects on locomotion and motor abilities, we examined the various groups for total activity in the OF test as well as for motor learning in the rotor-rod test. All behavioral procedures and tests were carried in a dimly lit room (50 lux), except otherwise mentioned, and in the order as described below. A schematic diagram of the experimental design and procedures is presented (Scheme 1).
Prenatal stress
PS was composed of three separate procedures delivered at days 13–15 of pregnancy (one procedure each day, between 9:00 and 12:00 AM), in the following sequence:
Swim stress: At day 13, rats were allowed to swim for 5 min in a squared water tank: 38 × 30 cm2, water depth: 60 cm. The temperature of water was maintained at 23 ± 1 °C (Avital et al., Citation2011).
Mirror stress: At day 14, rats were placed in a novel octagon shaped mirror box (20 cm sides) for 5 min under high light (2500 l×) conditions (for more details, see Supplementary materials).
Restraint stress: At day 15, rats were placed in a radial-shaped restrainer (6 cm height), for three times for 30 min each time with 1 h spent in a resting cage between exposures to the restrainer (Avital et al., Citation2011).
Enriched environment
At PND 30, rats were placed in a custom-made EE cage (100 L × 50 W × 50 H cm3) until PND 60. The cage consisted of various objects (e.g. running wheel, stairs, tubes, lego, and wood parts) which were weekly replaced (to maintain novelty) and cleaned. The cage also consisted of an extra elevated (25 cm above the main surface) surface (20 L × 50 W) that was accessible via stairs. In addition, a sandbox (20 L × 10 W) was included in the main floor, in order to diversify the cage texture. Eight rats were housed in each EE cage, in order to maintain social enrichment, without over-crowding. During their stay in the cage, rats had free access to food and water. Finally, in order to enrich their diet, cornflakes (20 g) were scattered once a week on the main floor. Cages were located in a temperature and humidity controlled room, under day/night cycle (lights on between 07:00 and 19:00) (Cymerblit-Sabba et al., Citation2013).
MPH treatment
At PND 60–90, rats were treated orally with 2.7 mg/kg MPH (Sigma-Aldrich, St. Louis, MO). MPH was dissolved in 1000 μL 10% sucrose solution and was stirred for 2 min (unheated) using a magnetic stirring rod until completely dissolved. In order to simplify dosing, the concentration of MPH was prepared so that the dose could easily be controlled by the volume of solution administered (e.g. a 300 g animal received 300 μL of solution). The solution was administered with a micropipette. We placed the filled tip in or near the animal’s mouth and slowly discharged the suspension to allow the animal to drink (Wheeler et al., Citation2007). The control rats were administrated with vehicle comprising 10% sucrose solution, in their appropriate volume (e.g. a 300 g animal received 300 μL of solution).
Behavioral tests
Open field (OF) test
The open field is made of a black lusterless Perspex box (100 L × 100 W × 40 H cm3). Rats were placed in the corner of the open field (facing the wall). Their behavior (i.e. locomotor activity and immobility duration) was videotaped for 5 min by a CC TV Panasonic camera with post-recording analysis performed using Ethovision XT software (Noldus, Wageningen, The Netherlands) (Avital et al., Citation2011).
Object recognition
The test occurred in a black lusterless Perspex box (50 × 50 cm2). Rats were acclimated to the test arena for 10 min per day during two successive days (i.e. they were allowed to explore the arena without any objects). Initially, the rats were placed in the distal part of the box, facing the wall. On the third day, rats were allowed to explore the arena for 10 min, with the presence of two identical objects (Lego cubes). Following the first exploration session, rats were removed back to their home cages. Subsequently, after 1 h, rats were placed back in the arena (which was cleaned) for a 5 min test trial with two objects, with one object replaced by a new one (a cone, located as in the exploration session). Their behavior (i.e. total exploration and percent novel object exploration) in the test trial was videotaped by a CC TV Panasonic camera (Panasonic Corporation, Osaka, Japan) and post-recording analysis with Ethovision XT software (Noldus, The Netherlands) (Cymerblit-Sabba et al., Citation2013).
Rotor-rod
The rotor-rod (San Diego Instruments, San Diego, CA) apparatus is used to assess motor functions, motor learning, coordination, and equilibrium. It is made of black lusterless Perspex, comprising a rotating rod with four lanes separated by opaque black Perspex. The apparatus contains a sawdust cabin (45.7 cm height) for safe landing and automatic recording of latencies (in sec) via a red-beams’ system. Each learning session consists of four daily trials on four consecutive days. Rats were acclimated to the apparatus for 30 s on the first day. Each trial starts at five rpm for 15 s with constant acceleration at 0.1 rpm per sec (max speed 50 rpm after 460 s) and the latency until the rat falls from the rod was measured (Zubedat et al., Citation2013).
Pre-pulse inhibition (PPI) and startle response
The test was held in a ventilated soundproof box (Campden Instruments, Silebym, UK). The session (a total of 90 trials) started with a 3 min acclimatization period with a 57 dB background noise level that was delivered continuously throughout the test session. To evaluate the startle response (in Newtons), each of the first 10 trials consisted of a single 40 ms 120 dB “pulse alone” startle stimuli (Inter-Trial-Interval (ITI) 1 min). The rest of the 80 trials (10 s ITI) consisted of random delivery of 10 “no stimuli” trials, during which no stimuli was delivered, fourteen “pre” stimuli (at 59, 61, 65, 69, 73, 78, or 85 dB), and 56 “pre-pulse” trials that consisted of a single 120 dB pulse preceded (80 ms interval) by a 20 ms pre pulse of 2, 4, 8, 12, 16, 21, or 28 dB above background (i.e. 59, 61, 65, 69, 73, 78, or 85 dB). Finally, pre-pulse inhibition (PPI) was calculated as the percent of the habituated response as follows: [100 − (max response to “pre-pulse” trial/max response to “pulse alone” trial × 100)] (Avital et al., Citation2011).
Statistical analysis
In order to examine the effects of life circumstances and pharmacological treatment, we used 3-way ANOVA (2 × 2 × 2). Interaction effects were further analyzed with one-way ANOVA, followed by the Post-Hoc Tukey test or Student's t-tests for independent samples. Moreover, we also used 4-way ANOVA for mixed design, with the three groups (PS, EE, and MPH) as between subject's factors and PPI pre-intensity/rotor-rod training day, as within subject's factor.
A result was significant when p < 0.05. All tests were calculated as two-tailed with SPSS V17.0 (IBM, New York, NY). Results are presented as means ± standard error of the means (S.E.M).
Results
Attention performance
Measuring the total duration of objects exploration in the object recognition test (), we calculated a 3-way ANOVA (2 × 2 × 2) for the PS, EE, and MPH treatment. We found significant effects for the exposure to PS [F(1, 76) = 7.22, p < 0.009], the exposure to EE [F(1, 76) = 7.28, p > 0.008], with no significant effect of MPH treatment [F(1, 76) = 2.51, p > 0.117]. A significant EE × MPH interaction [F(1, 76) = 9.013, p < 0.004] was found, while all other interactions were not significant. Compared with the controls level, the PS group showed the shortest exploration time (p < 0.001), while the EE + MPH showed the longest exploration time, compared with either the control (p < 0.013) or MPH (p < 0.006) groups.
Figure 1. Selective and partial sustained attention. (A) In the object recognition test, the PS group decreased total exploration time, while the EE + MPH led to an increase, compared with the control group (*p < 0.013, **p < 0.0001). (B) The PS group showed the shortest novel object exploration duration compared with the control group. MPH and/or EE recovered the impaired performance of the PS group, compared with the control group (*p < 0.04, **p < 0.001) and compared with the PS group (##p < 0.001). (C) In the prepulse inhibition test, the PS group showed the lowest PPI along all pre-intensities, while EE showed the highest PPI compared with all other groups. PS + EE, PS + MPH, and PS + EE + MPH groups recovered the impaired performance of the PS (*p < 0.001).
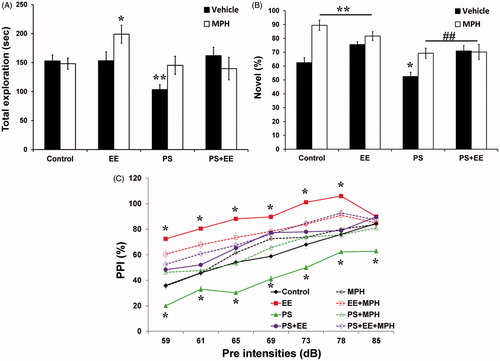
The proportion of time spent exploring the novel object versus the familiar object considered to reflect selective attention in the novel object recognition test (). Significant main effects were found for PS [F(1, 76) = 18.39, p < 0.0001], for EE [F(1, 76) = 5.24, p < 0.025], and for MPH [F(1, 76) = 20.67, p < 0.0001]. A significant EE × MPH interaction [F(1, 76) = 12.682, p < 0.001] was observed, while all other interactions were not significant. The PS group exhibited the lowest percent of novel object exploration (p < 0.04) compared with the control group. EE, MPH, and EE + MPH groups showed similar performance, with substantially elevated novel object exploration compared with the control group (p < 0.004; p < 0.0001; p < 0.001, respectively). MPH, EE, and their combination significantly increased the percent of novel object exploration compared with the PS group (p < 0.002, p < 0.001, p < 0.008, respectively).
In the pre-pulse inhibition test (), we calculated a 4-way ANOVA (2 × 2 × 2 × 7) for the PS, EE, and MPH treatment as between-subjects factors, and pre-pulse intensity as within-subjects factor. A significant main effect of pre-pulse intensity was observed [F(6, 83) = 296.4, p < 0.0001], along with all two-way interactions (i.e. pre-pulse intensity × PS, pre-pulse intensity × EE and pre-pulse intensity × MPH), three-way interactions (pre-pulse intensity × PS × EE, pre-pulse intensity × PS × MPH and pre-pulse intensity × EE × MPH), and four-way interaction (pre-pulse intensity × PS × EE × MPH) (p < 0.0001). Examining the between-subjects effects, we found a significant effects for PS [F(1, 88) = 98.19, p < 0.0001], EE [F(1, 88) = 367.17, p < 0.0001], and MPH [F(1, 88) = 16.18, p < 0.0001]. We also found significant two-way interactions for PS × MPH [F(1, 88) = 63.97, p < 0.0001] and EE × MPH [F(1, 88) = 61.56, p < 0.0001], but not for the PS × EE [F(1, 88) < 1].
Similar to the selective attention findings, the PS group showed the lowest performance along all pre-intensities (p < 0.0001). In contrast, the EE group showed the highest performance (except the last pre-intensity level) compared with all other groups (p < 0.0001). MPH led to elevated PPI in the intermediate pre-pulse intensities (65 dB, p < 0.009; 69 dB, p < 0.0001). MPH and/or EE increased PPI level along all pre-intensities, compared with the PS group (p < 0.0001).
Evaluation of anxiety-like behaviors
In order to monitor possible anxiolytic or anxiogenic effects following different life circumstances and pharmacological treatment, we measured both startle reactivity and immobility behavior in the open field. A significant main effect on startle reactivity () was found for the exposure to EE [F(1, 88) = 35.14, p < 0.0001], but not for PS [F(1, 88) < 1]and MPH treatment [F(1, 88) = 2.08, p > 0.153]. Significant effects for PS × MPH [F(1, 88) = 18.31, p < 0.0001] and EE × MPH [F(1, 88) = 13.507, p < 0.0001] interactions were found, as well as for the PS × EE × MPH interaction [F(1, 88) = 21.22, p < 0.0001]. The PS group exhibited the highest startle reactivity (p < 0.0001). Both EE and MPH recovered the anxiogenic effect of PS to a level lower than the control group (p < 0.013, p < 0.049, respectively). In addition, while the MPH group showed similar startle reactivity to that of the control, the EE group showed a decreased reactivity, compared with the control group (p < 0.013).
Figure 2. Evaluation of anxiety-like behaviors. (A) In the startle reflex test, the PS group showed the highest startle reactivity, while PS + EE, PS + MPH, and PS + EE + MPH groups significantly reduced this elevation to a level lower than the control group (*p < 0.049, **p < 0.0001). (B) In the open-field test, the PS group showed the highest immobility duration (***p < 0.0001 compared with the control group). The hyper-immobility of PS was not observed in the PS + EE, PS + MPH, and PS + EE + MPH groups (#p < 0.0001: compared with the PS group), which were indistinguishable from controls. In addition, while MPH increased immobility, EE (with or without MPH) decreased it (*p < 0.031, **p < 0.028, compared with the control group).
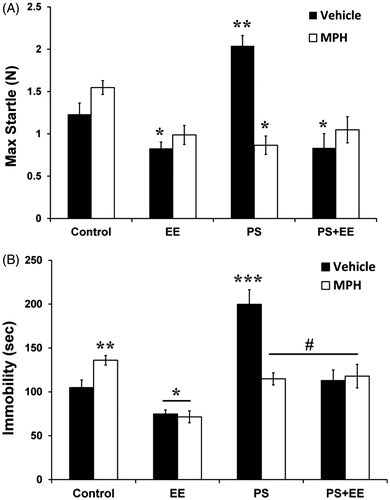
Similar results were obtained while measuring immobility behavior in the open field test (). Significant effects of PS [F(1, 88) = 40.41, p < 0.0001], EE [F(1, 88) = 51.305, p < 0.0001], and MPH treatment [F(1, 88) = 4.38, p < 0.039] were observed. Significant PS × MPH [F(1, 88) = 18.7, p < 0.0001] and EE × MPH [F(1, 88) = 4.97, p < 0.028] interactions were found, as well as a PS × EE × MPH interaction [F(1, 88) = 24.92, p < 0.0001]. The PS group exhibited the highest immobility duration (p < 0.0001). The anxiogenic effect of PS was not observed in the PS + EE, PS + MPH, and PS + EE + MPH groups (p < 0.0001), which were indistinguishable from controls. In addition, while the MPH group showed higher immobility duration compared with the control group (p < 0.028), immobility was decreased in EE and EE + MPH groups relative to controls (p < 0.031, p < 0.011, respectively).
Motor evaluation
Finally, we examined locomotor activity in the open field, as well as motor learning performance in the rotor-rod. In the open field (), we found significant effects of PS [F(1, 88) = 46.52, p < 0.0001], EE [F(1, 88) = 4.12, p < 0.045], whereas no effect of MPH treatment was observed [F(1, 88) < 1]. Significant PS × EE [F(1, 88) = 4.88, p < 0.030] and EE × MPH [F(1, 88) = 22.89, p < 0.0001] interactions were found, but there was no PS × EE × MPH interaction [F(1, 88) = 3.1, p < 0.081]. The PS group exhibited the lowest activity relative to controls (p < 0.0001). Although the PS + MPH group showed a trend toward increasing hypo-activity observed in the PS, the PS + EE and PS + EE + MPH showed a significant increase of locomotor activity relative to the PS activity level (p < 0.0001, p < 0.019, respectively), returning control group levels. In addition, both MPH and EE were hyperactive relative to the control group (p < 0.003, p < 0.002, respectively).
Figure 3. Locomotor activity and motor learning. (A) In the open-field test, the PS group showed the lowest activity (***p < 0.0001) compared with the control group, while PS + EE and PS + EE + MPH recovered this hypo-activity (#p < 0.019, ##p < 0.0001: compared with the PS group) back to the control group level. In addition, MPH or EE increased activity (*p < 0.003, **p < 0.002, compared with the control group). (B) In the rotor-rod test, both PS and PS + EE showed the highest motor learning ability compared with the control group, while the MPH group showed the lowest performance (*p < 0.024, **p < 0.001, ***p < 0.0001: compared with the control group; #p < 0.0001: compared with the PS group).
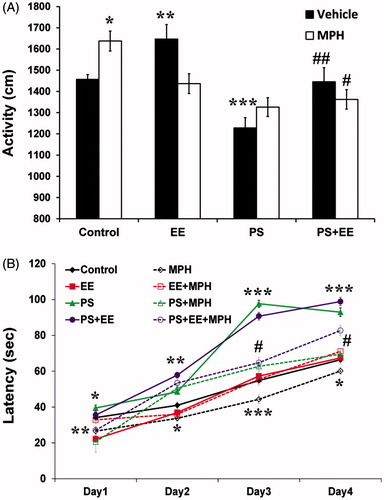
In the rotor-rod motor learning task (), we used a four-way ANOVA (2 × 2 × 2 × 4) for the PS, EE, and MPH treatment as between-subject's factors, and day of training as within-subject's factor. A significant effect was found for days of learning [F(3, 86) = 1207.95.4, p < 0.0001], with all two-way interactions (i.e. days × PS, days × EE, and days × MPH) and three-way interactions (days × PS × EE, days × PS × MPH and days × EE × MPH) found to be significant (p < 0.0001). The four-way interaction (days × PS × EE × MPH) was not significant.
Examining the between-subjects effects, we found significant main effects for PS [F(1, 88) = 455.46, p < 0.0001], EE [F(1, 88) = 15.79, p < 0.0001], and MPH [F(1, 88) = 160.68, p < 0.0001]. Significant PS × MPH [F(1, 88) = 87.06, p < 0.0001] and EE × MPH [F(1, 88) = 28.72, p < 0.0001] interactions were observed. Neither PS × EE [F(1, 88) < 1] nor PS × EE × MPH interactions were significant [F(1, 88) = 3.82, p > 0.054].
During all 4 d of learning, the MPH group showed the lowest motor performance compared with the control group (days 1–4: p < 0.001, p < 0.002, p < 0.0001, p < 0.022). Overall, both PS and PS + EE showed the highest motor-learning performance (compared with the control group, on days 1–4: p < 0.024, p < 0.001, p < 0.0001, p < 0.0001). However, treating these groups with MPH (i.e. PS + MPH and PS + EE + MPH) moderated their elevated performance towards the performance of the control group on days 3–4 (p < 0.0001).
Discussion
The development of the nervous system is known to be highly dependent on the interactions between the organism and its environment. The dopaminergic system, which is thought to play a fundamental role in psychiatric disorders such as ADHD, is known to be particularly sensitive to environmental life circumstances. Moreover, the dopaminergic system affects various cognitive processes as well as attentional function, which serves as the preliminary gate for cognition.
In the current study, we examined the effects of positive (i.e. environmental enrichment) or negative (i.e. PS) early life circumstances on distinct types of attention. We also examined the possible mediating and/or moderating effects of MPH on the consequences of the aforementioned life circumstances.
We have focused on two types of attention: in the object recognition test, we have examined selective attention, and in the prepulse inhibition (PPI) test, we have examined partial sustained attention. Recently, it was shown by us (Avital et al., Citation2011) and by others (Annic et al., Citation2014; Scholes et al., Citation2010; Weiss et al., Citation2001) that PPI performance is modulated by attention and is suggested as partial sustained attention. We found that PS impaired selective attention as well as partial sustained attention. Both the “behavioral” (EE) and the pharmacological (MPH) treatments ameliorated the deleterious effects of PS on attentional functioning.
MPH is a well-known pharmacological treatment for ADD/ADHD, exerting its effects mainly through DA activity in the prefrontal cortex (Schmeichel et al., Citation2013).While there are reports in humans regarding the beneficial effects of MPH on attention deficits (Brodeur et al., Citation2001; Paton et al., Citation2014), only a few studies (both in humans and in animal models) have examined the effects of MPH without the presence of ADD/ADHD (Avital et al., Citation2011; Brodeur et al., Citation2001). Similar to our previous study (Avital et al., Citation2011), we found that MPH increased PPI performance (i.e. naive rats that were treated with MPH) only in some of the prepulse intensities tested. However, we found that MPH had a clear beneficial effect on selective attention, which was tested in the object recognition task. In support of our results, Jonkman et al. (Citation1997) showed that MPH affects selective attentional processing even when attentional deficits are absent.
It has been also suggested that EE may exert advantageous effects on selective attention in the object recognition test (Doulames et al., Citation2014; Mesa-Gresa et al., Citation2013). However, previous studies showed conflicting results in which EE decreased (Pena et al., Citation2009), had no effect (Varty et al., Citation2000), or had increased (Avital et al., Citation2011; Chen et al., Citation2010) PPI. Indeed, our results indicated that EE increased both PPI and OR performance.
It was previously reported that MPH may induce an anxiogenic effect (Heredia et al., Citation2014). Therefore, in our study, we conducted an anxiety evaluation of the various experimental groups, measuring startle reactivity and immobility behavior. Indeed, MPH led to increased immobility duration and tended to increase startle reactivity. As shown in previous studies (Fares et al., Citation2013; Urakawa et al., Citation2013), we found that EE by itself had an anxiolytic effect while PS had an anxiogenic effect. Nevertheless, the treatment with both MPH and/or EE (i.e. MPH, EE, and MPH + EE groups) decreased the anxiogenic effect of PS.
Since MPH, as a psychostimulant, may exert effects on locomotion and motor abilities, we utilized the rotor-rod and open field tests, examining motor learning and locomotor activity, respectively. We found that while MPH impaired motor learning in the rotor-rod, it led to increased locomotion in the open field test. Since locomotion requires exploration of a featureless environment, the stimulant effect of MPH seems to be straightforward, as shown previously (Heredia et al., Citation2014). However, when the motor task is more demanding, like during the 4 d of learning in the rotor-rod, we assumed that the stimulant effect of MPH interfered with the ability to stay on the rod. Surprisingly, opposed to the adverse effects of PS as aforementioned, the PS group showed superior performance in the motor learning task and decreased locomotor activity in the open field. While PS + EE performed similar to the PS group, the PS + MPH group showed motor-learning performance similar to the control group.
Thus, while MPH led to impaired motor learning, both PS + MPH and EE + MPH groups recovered this decrement. The dopaminergic system is known to be particularly sensitive to environmental circumstances. Many reports have indicated that PS affects the DA system in various brain regions (Baier et al., Citation2012; Gill et al., Citation2013). However, the prefrontal cortex (PFC) is specifically considered to be crucial for the expression of the highest-order cognitive abilities. Moreover, the PFC is also sensitive to the detrimental effects of exposure to stress, which can impair higher order abilities such as working memory and attention (Arnsten, Citation2009). Berger et al. (Citation2002) found that PS increased DA D2 receptors in the dorsal frontal cortex and medial prefrontal cortex. In addition, Carboni et al. (Citation2010) reported that PS reduced NA concentration in the PFC. Thus, our findings suggest that MPH exerts beneficial effects on PS adverse consequences, presumably mediated by increasing mostly DA, but also NA, activity in the PFC.
The DA system is known to be sensitive to is EE. Similar to the above-mentioned effects of PS, there is evidence that EE increases dopamine clearance from the medial prefrontal cortex (Neugebauer et al., Citation2004). Therefore, we postulate that the positive effects of EE on PS may be explained by a non-DA mechanism. Specifically, PS was shown to reduce spine density and dendritic length, accompanied by reduced complexity of dendritic trees in the prefrontal cortex (Murmu et al., Citation2006). In contrast, EE was shown to increase brain weight and cortex thickness (Percaccio et al., Citation2007), dendrocyte branches, synapses (Gelfo et al., Citation2009; Johansson, Citation2004; Lewis, Citation2004) and oligodendrocytes in the cortex (Zhao et al., Citation2011). Finally, as a possible shared mechanism for the beneficial effects of both MPH and/or EE on PS, previous reports showed that MPH can enhance neurotrophic factor expression (Connor et al., Citation1999, Citation2001; Cohen et al., Citation2003), as can EE (Gelfo et al., Citation2011). Thus, the neurotrophic effects of MPH and EE might be advantageous because it could render neurons resistance to the harmful consequences of PS. The positive effects of EE on the deleterious consequences of PS may stem from the opposite effects of these two life circumstances. Additionally, the effects of PS or EE on PFC are sparse in the literature, thus, further investigation is needed to better clarify these opposite processes.
To conclude, this study provides evidence that peripubertal exposure to EE, as a behavioral treatment (i.e. providing enhanced sensory, motor, and social opportunities), or MPH as a pharmacological treatment, might be an optional therapeutic intervention in preventing the long-term adverse consequences of PS.
Supplementary material available online
Supplemental Material.pdf
Download PDF (34.9 KB)Declaration of interest
The authors declare no conflict of interest.
This study was founded by US ARO grant W911NF-12-1-0002 awarded to A. A.
References
- Anderson VR, Scott LJ. (2006). Methylphenidate transdermal system: in attention-deficit hyperactivity disorder in children. Drugs 66:1117–26
- Annic A, Bocquillon P, Bourriez JL, Derambure P, Dujardin K. (2014). Effects of stimulus-driven and goal-directed attention on prepulse inhibition of the cortical responses to an auditory pulse. Clin Neurophysiol: Off J Int Feder Clin Neurophysiol 125:1576–88
- Aoyama T, Kotaki H, Iga T. (1990). Dose-dependent kinetics of methylphenidate enantiomers after oral administration of racemic methylphenidate to rats. J Pharmacobio-Dyn 13:647–52
- Arnsten AF. (2009). Stress signalling pathways that impair prefrontal cortex structure and function. Nat Rev Neurosci 10:410–22
- Avital A, Dolev T, Aga-Mizrachi S, Zubedat S. (2011). Environmental enrichment preceding early adulthood methylphenidate treatment leads to long term increase of corticosterone and testosterone in the rat. PLoS One 6:e22059
- Baier CJ, Katunar MR, Adrover E, Pallares ME, Antonelli MC. (2012). Gestational restraint stress and the developing dopaminergic system: an overview. Neurotox Res 22:16–32
- Berger MA, Barros VG, Sarchi MI, Tarazi FI, Antonelli MC. (2002). Long-term effects of prenatal stress on dopamine and glutamate receptors in adult rat brain. Neurochem Res 27:1525–33
- Bowling SL, Rowlett JK, Bardo MT. (1993). The effect of environmental enrichment on amphetamine-stimulated locomotor activity, dopamine synthesis and dopamine release. Neuropharmacology 32:885–93
- Braida D, Donzelli A, Martucci R, Ponzoni L, Pauletti A, Langus A, Sala M. (2013). Mice discriminate between stationary and moving 2D shapes: application to the object recognition task to increase attention. Behav Brain Res 242:95–101
- Brodeur DA, Pond M. (2001). The development of selective attention in children with attention deficit hyperactivity disorder. J Abnorm Child Psychol 29:229–39
- Carboni E, Barros VG, Ibba M, Silvagni A, Mura C, Antonelli MC. (2010). Prenatal restraint stress: an in vivo microdialysis study on catecholamine release in the rat prefrontal cortex. Neuroscience 168:156–66
- Chapillon P, Patin V, Roy V, Vincent A, Caston J. (2002). Effects of pre-and postnatal stimulation on developmental, emotional, and cognitive aspects in rodents: a review. Dev Psychobiol 41:373–87
- Chen Y, Mao Y, Zhou D, Hu X, Wang J, Ma Y. (2010). Environmental enrichment and chronic restraint stress in ICR mice: effects on prepulse inhibition of startle and Y-maze spatial recognition memory. Behav Brain Res 212:49–55
- Cohen AD, Tillerson JL, Smith AD, Schallert T, Zigmond MJ. (2003). Neuroprotective effects of prior limb use in 6-hydroxydopamine-treated rats: possible role of GDNF. J Neurochem 85:299–305
- Connor B, Kozlowski DA, Schallert T, Tillerson JL, Davidson BL, Bohn MC. (1999). Differential effects of glial cell line-derived neurotrophic factor (GDNF) in the striatum and substantia nigra of the aged Parkinsonian rat. Gene Therapy 6:1936–51
- Connor B, Kozlowski DA, Unnerstall JR, Elsworth JD, Tillerson JL, Schallert T, Bohn MC. (2001). Glial cell line-derived neurotrophic factor (GDNF) gene delivery protects dopaminergic terminals from degeneration. Exp Neurol 169:83–95
- Cymerblit-Sabba A, Lasri T, Gruper M, Aga-Mizrachi S, Zubedat S, Avital A. (2013). Prenatal enriched environment improves emotional and attentional reactivity to adulthood stress. Behav Brain Res 241:185–90
- Darnaudery M, Maccari S. (2008). Epigenetic programming of the stress response in male and female rats by prenatal restraint stress. Brain Res Rev 57:571–85
- Devilbiss DM, Berridge CW. (2006). Low-dose methylphenidate actions on tonic and phasic locus coeruleus discharge. J Pharmacol Exp Ther 319:1327–35
- Doulames V, Lee S, Shea TB. (2014). Environmental enrichment and social interaction improve cognitive function and decrease reactive oxidative species in normal adult mice. Int J Neurosci 124:369–76
- Fares RP, Belmeguenai A, Sanchez PE, Kouchi HY, Bodennec J, Morales A, Georges B, et al. (2013). Standardized environmental enrichment supports enhanced brain plasticity in healthy rats and prevents cognitive impairment in epileptic rats. PLoS One 8:e53888
- Gelfo F, De Bartolo P, Giovine A, Petrosini L, Leggio MG. (2009). Layer and regional effects of environmental enrichment on the pyramidal neuron morphology of the rat. Neurobiol Learn Mem 91:353–65
- Gelfo F, Cutuli D, Foti F, Laricchiuta D, De Bartolo P, Caltagirone C, Petrosini L, Angelucci F. (2011). Enriched environment improves motor function and increases neurotrophins in hemicerebellar lesioned rats. Neurorehabil Neural Repair 25:243–52
- Gill KE, Beveridge TJ, Smith HR, Porrino LJ. (2013). The effects of rearing environment and chronic methylphenidate administration on behavior and dopamine receptors in adolescent rats. Brain Res 1527:67–78
- Greenough WT, Volkmar FR. (1973). Pattern of dendritic branching in occipital cortex of rats reared in complex environments. Exp Neurol 40:491–504
- Guilarte TR, Toscano CD, McGlothan JL, Weaver SA. (2003). Environmental enrichment reverses cognitive and molecular deficits induced by developmental lead exposure. Ann Neurol 53:50–6
- Hannestad J, Gallezot JD, Planeta-Wilson B, Lin SF, Williams WA, van Dyck CH, Malison RT, et al. (2010). Clinically relevant doses of methylphenidate significantly occupy norepinephrine transporters in humans in vivo. Biol Psychiatry 68:854–60
- Hellemans KG, Benge LC, Olmstead MC. (2004). Adolescent enrichment partially reverses the social isolation syndrome. Brain Res Dev Brain Res 150:103–15
- Heredia L, Torrente M, Colomina MT, Domingo JL. (2014). Assessing anxiety in C57BL/6J mice: a pharmacological characterization of the open-field and light/dark tests. J Pharmacol Toxicol Methods 69:108–14
- Huizink AC, Mulder EJ, Buitelaar JK. (2004). Prenatal stress and risk for psychopathology: specific effects or induction of general susceptibility? Psychol Bull 130:115–42
- Ilin Y, Richter-Levin G. (2009). Enriched environment experience overcomes learning deficits and depressive-like behavior induced by juvenile stress. PLoS One 4:e4329
- Johansson BB. (2004). Functional and cellular effects of environmental enrichment after experimental brain infarcts. Restor Neurol Neurosci 22:163–74
- Jonkman LM, Kemner C, Verbaten MN, Koelega HS, Camfferman G, vd Gaag RJ, Buitelaar JK, van Engeland H. (1997). Effects of methylphenidate on event-related potentials and performance of attention-deficit hyperactivity disorder children in auditory and visual selective attention tasks. Biol Psychiatry 41:690–702
- Lemaire V, Lamarque S, Le Moal M, Piazza PV, Abrous DN. (2006). Postnatal stimulation of the pups counteracts prenatal stress-induced deficits in hippocampal neurogenesis. Biol Psychiatry 59:786–92
- Levin ED, Bushnell PJ, Rezvani AH. (2011). Attention-modulating effects of cognitive enhancers. Pharmacol Biochem Behav 99:146–54
- Lewis MH. (2004). Environmental complexity and central nervous system development and function. Ment Retard Dev Disabil Res Rev 10:91–5
- Mesa-Gresa P, Perez-Martinez A, Redolat R. (2013). Environmental enrichment improves novel object recognition and enhances agonistic behavior in male mice. Aggress Behav 39:269–79
- Missale C, Nash SR, Robinson SW, Jaber M, Caron MG. (1998). Dopamine receptors: from structure to function. Physiol Rev 78:189–225
- Morley-Fletcher S, Rea M, Maccari S, Laviola G. (2003). Environmental enrichment during adolescence reverses the effects of prenatal stress on play behaviour and HPA axis reactivity in rats. Eur J Neurosci 18:3367–74
- Murmu MS, Salomon S, Biala Y, Weinstock M, Braun K, Bock J. (2006). Changes of spine density and dendritic complexity in the prefrontal cortex in offspring of mothers exposed to stress during pregnancy. Eur J Neurosci 24:1477–87
- Neugebauer NM, Cunningham ST, Zhu J, Bryant RI, Middleton LS, Dwoskin LP. (2004). Effects of environmental enrichment on behavior and dopamine transporter function in medial prefrontal cortex in adult rats prenatally treated with cocaine. Brain Res Dev Brain Res 153:213–23
- Nithianantharajah J, Hannan AJ. (2006). Enriched environments, experience-dependent plasticity and disorders of the nervous system. Nat Rev Neurosci 7:697–709
- Pamplona FA, Pandolfo P, Savoldi R, Prediger RD, Takahashi RN. (2009). Environmental enrichment improves cognitive deficits in Spontaneously Hypertensive Rats (SHR): relevance for Attention Deficit/Hyperactivity Disorder (ADHD). Prog Neuro-Psychopharmacol Biol Psychiatry 33:1153–60
- Paton K, Hammond P, Barry E, Fitzgerald M, McNicholas F, Kirley A, Robertson IH, et al. (2014). Methylphenidate improves some but not all measures of attention, as measured by the TEA-Ch in medication-naive children with ADHD. Child Neuropsychol: J Normal Abnormal Dev Childhood Adoles 20:303–18
- Pena Y, Prunell M, Rotllant D, Armario A, Escorihuela RM. (2009). Enduring effects of environmental enrichment from weaning to adulthood on pituitary–adrenal function, pre-pulse inhibition and learning in male and female rats. Psychoneuroendocrinology 34:1390–404
- Percaccio CR, Pruette AL, Mistry ST, Chen YH, Kilgard MP. (2007). Sensory experience determines enrichment-induced plasticity in rat auditory cortex. Brain Res 1174:76–91
- Perry JL, Stairs DJ, Bardo MT. (2008). Impulsive choice and environmental enrichment: effects of d-amphetamine and methylphenidate. Behav Brain Res 193:48–54
- Schmeichel BE, Berridge CW. (2013). Neurocircuitry underlying the preferential sensitivity of prefrontal catecholamines to low-dose psychostimulants. Neuropsychopharmacology: Off Publ Am Coll Neuropsychopharmacol 38:1078–84
- Scholes KE, Martin-Iverson MT. (2010). Disturbed prepulse inhibition in patients with schizophrenia is consequential to dysfunction of selective attention. Psychophysiology 47:223–35
- Seo JH, Yu JH, Suh H, Kim MS, Cho SR. (2013). Fibroblast growth factor-2 induced by enriched environment enhances angiogenesis and motor function in chronic hypoxic-ischemic brain injury. PLoS One 8:e74405
- Urakawa S, Takamoto K, Hori E, Sakai N, Ono T, Nishijo H. (2013). Rearing in enriched environment increases parvalbumin-positive small neurons in the amygdala and decreases anxiety-like behavior of male rats. BMC Neurosci 14:13
- Urakawa S, Hida H, Masuda T, Misumi S, Kim TS, Nishino H. (2007). Environmental enrichment brings a beneficial effect on beam walking and enhances the migration of doublecortin-positive cells following striatal lesions in rats. Neuroscience 144:920–33
- Varty GB, Paulus MP, Braff DL, Geyer MA. (2000). Environmental enrichment and isolation rearing in the rat: effects on locomotor behavior and startle response plasticity. Biol Psychiatry 47:864–73
- Volkow ND, Wang GJ, Fowler JS, Gatley SJ, Logan J, Ding YS, Hitzemann R, Pappas N. (1998). Dopamine transporter occupancies in the human brain induced by therapeutic doses of oral methylphenidate. Am J Psychiatry 155:1325–31
- Wargin W, Patrick K, Kilts C, Gualtieri CT, Ellington K, Mueller RA, Kraemer G, Breese GR. (1983). Pharmacokinetics of methylphenidate in man, rat and monkey. J Pharmacol Exp Ther 226:382–6
- Weinstock M. (2001). Alterations induced by gestational stress in brain morphology and behaviour of the offspring. Prog Neurobiol 65:427–51
- Weiss IC, Domeney AM, Moreau JL, Russig H, Feldon J. (2001). Dissociation between the effects of pre-weaning and/or post-weaning social isolation on prepulse inhibition and latent inhibition in adult Sprague–Dawley rats. Behav Brain Res 121:207–18
- Wheeler TL, Eppolito AK, Smith LN, Huff TB, Smith RF. (2007). A novel method for oral stimulant administration in the neonate rat and similar species. J Neurosci Methods 159:282–5
- Wooters TE, Bardo MT, Dwoskin LP, Midde NM, Gomez AM, Mactutus CF, Booze RM, Zhu J. (2011). Effect of environmental enrichment on methylphenidate-induced locomotion and dopamine transporter dynamics. Behav Brain Res 219:98–107
- Wright RL, Conrad CD. (2008). Enriched environment prevents chronic stress-induced spatial learning and memory deficits. Behav Brain Res 187:41–7
- Zhao YY, Shi XY, Qiu X, Zhang L, Lu W, Yang S, Li C, et al. (2011). Enriched environment increases the total number of CNPase positive cells in the corpus callosum of middle-aged rats. Acta Neurobiol Exp 71:322–30
- Zubedat S, Aga-Mizrachi S, Cymerblit-Sabba A, Shwartz J, Leon JF, Rozen S, Varkovitzky I, et al. (2014). Human–animal interface: the effects of handler's stress on the performance of canines in an explosive detection task. Appl Anim Behav Sci 158:69–75
- Zubedat S, Freed Y, Eshed Y, Cymerblit-Sabba A, Ritter A, Nachmani M, Harush R, et al. (2013). Plant-derived nanoparticle treatment with cocc 30c ameliorates attention and motor abilities in sleep-deprived rats. Neuroscience 253:1–8