Abstract
Acute restraint stress (ARS) for 3 h causes corticosterone (CORT) elevation in venous blood, which is accompanied by Fos up-regulation in the paraventricular nucleus (PVN) of male C57BL/6 mice. CORT elevation by ARS is attenuated in PACAP-deficient mice, but unaffected in PAC1-deficient mice. Correspondingly, Fos up-regulation by ARS is greatly attenuated in PACAP-deficient mice, but much less so in PAC1-deficient animals. We noted that both PACAP- and PAC1-deficiency greatly attenuate CORT elevation after ARS when CORT measurements are performed on trunk blood following euthanasia by abrupt cervical separation: this latter observation is of critical importance in assessing the role of PACAP neurotransmission in ARS, based on previous reports in which serum CORT was sampled from trunk blood. Seven days of chronic restraint stress (CRS) induces non-habituating CORT elevation, and weight loss consequent to hypophagia, in wild-type male C57BL/6 mice. Both CORT elevation and weight loss following 7-day CRS are severely blunted in PACAP-deficient mice, but only slightly in PAC1-deficient mice. However, longer periods of daily restraint (14–21 days) resulted in sustained weight loss and elevated CORT in wild-type mice, and these effects of long-term chronic stress were attenuated or abolished in both PACAP- and PAC1-deficient mice. We conclude that while a PACAP receptor in addition to PAC1 may mediate some of the PACAP-dependent central effects of ARS and short-term (<7 days) CRS on the hypothalamo-pituitary-adrenal (HPA) axis, the PAC1 receptor plays a prominent role in mediating PACAP-dependent HPA axis activation, and hypophagia, during long-term (>7 days) CRS.
Introduction
PACAP neurotransmission has been linked to brain circuits whose chronic activation is hypothesized to underlie HPA axis activation due to psychogenic stress (Agarwal et al., Citation2005; Dore et al., Citation2013; Grinevich et al., Citation1997; Norrholm et al., Citation2005). PACAP-deficient mice were first reported to be less anxious than their wild-type counterparts, based on elevated plus, emergence and novel object tests, in 2001 (Hashimoto et al., Citation2001). PACAP is also linked to the effects of chronic stress in triggering depressive and anxious behaviors. For example, increased forced swim test immobility and decreased social interaction occurring after 14 days of social defeat in wild-type C57BL/6 mice, indicative of a behaviorally depressed phenotype, are absent in PACAP-deficient mice (Lehmann et al., Citation2013). In the rat, a series of reports have highlighted a potential role of PACAP neurotransmission in anxious behavior emerging during a chronic (7-day) variate stress protocol (Hammack et al., Citation2009, Citation2010; Kocho-Schellenberg et al., Citation2014; Roman et al., Citation2014). Furthermore, while HPA axis activation following psychogenic stress [i.e. chronic social defeat, acute restraint stress (ARS), open field exploration] is decreased in PACAP-deficient mice, CORT elevation following acute systemic stressors (hypoglycemia, lipopolysaccharide administration or cold stress) is not (Lehmann et al., Citation2013; Stroth & Eiden, Citation2010; Stroth et al., Citation2011a,Citationb; Tsukiyama et al., Citation2011). These studies implicate PACAP and its receptor(s) as possible targets in therapy for stress-related depression, and along with recent clinical data (Ressler et al., Citation2011), potentially for depressive behavioral effects occurring in post-traumatic stress disorder (PTSD) (Hammack et al., Citation2012). However, more information derived from animal models for stress-related depression is required, along with additional clinical information, to fully implicate PACAP and its receptor(s) as drug targets for the treatment of depression or PTSD.
We sought to generalize the hypothesis that PACAPergic circuitry in the brain contributes to sustained activation of the HPA axis during chronic stress, by extending our studies of the effects of PACAP deficiency on chronic social stress (social defeat) to a second mild chronic psychogenic stressor, restraint (Hayashi et al., Citation2014; Kwon et al., Citation2006), that does not depend on social interaction. The PACAP receptor, PAC1, has been implicated in transducing the peripheral effects of PACAP on the adrenal medulla in both acute and chronic stress due to either psychogenic or systemic stressors in mice (Stroth & Eiden, Citation2010; Stroth et al., Citation2011a,Citationb, Citation2013). Both PACAP and the PAC1 receptor are also implicated in the anxiogenic effects of PACAP during chronic variate stress, mediated at the level of the extended amygdala, in the rat (Hammack et al., Citation2010; Roman et al., Citation2014). The studies reported here include an assessment of the effects of both PACAP and PAC1 deficiency on activation of the HPA axis following either acute or chronic restraint, and on the depressive behaviors induced by chronic restraint.
Materials and methods
Animals
All experiments were conducted using male C57BL/6N wild-type and C57BL/6N PACAP-deficient mice, and male C57BL/6 wild-type and PAC1-deficient C57BL/6 mice, 8–10 weeks of age, generated through an in-house breeding program in accordance with NIH guidelines and standards. PAC1-deficient mice were genotyped as approximately 82%N/18%J as assessed at multiple loci at which J and N sub-strains differ, and hence each deficient line (PACAP and PAC1) was paired with congenic control (wild-type) mice. All mice were individually housed 24 h (acute stress experiments) or 7 days (chronic stress experiments) under a 12-h light/12-h dark cycle (lights off at 6 p.m.) with food and water ad libitum prior to experimental testing during the light phase of the cycle. Mice were randomly assigned to each experimental group. All experiments were approved by the NIMH Institutional Animal Care and Use Committee and conducted in accordance with the NIH guidelines. Generation of PACAP-deficient mice, and PAC1-deficient, has been described previously, with each line back-crossed onto C57BL/6 as described above (Hamelink et al., Citation2002a; Otto et al., Citation2001).
Lipopolysaccharide administration
Lipopolysaccharide (LPS) challenge was conducted as previously described (Ait-Ali et al., Citation2010; Lehmann et al., Citation2013). Briefly, animals were transported to the test room in their home cages and individually housed for 12 h during the dark phase of the light cycle. The following day, 3 h into the light phase, mice were injected intraperitoneally with LPS (4 µl/g body weight, of a 0.25 mg/ml solution in saline) from Escherichia coli serotype 0.111:B4 (Sigma-Aldrich, St. Louis, MO), or an equivalent volume of saline. After 3 h, mice were transferred into a DecapiCone (DecapiCones®, Braintree Scientific Inc., Braintree, MA), rapidly killed by decapitation with sharp surgical scissors, and trunk blood collected and processed according to procedures described below.
Acute restraint stress
ARS was carried out as previously described (Stroth & Eiden, Citation2010). In brief, individually housed animals were transported to the test room in their home cages as described in the aforementioned methods. Mice were then restrained in a DecapiCone with all limbs positioned flat underneath the body to minimize physical pain. The large end of the tube was then rolled shut and secured with adhesive tape. The DecapiCones used were also previously perforated for adequate ventilation, with the tip of the cone removed to allow unobstructed breathing. The restrained mice were then placed back into the home cage for 3 h. The control, unstressed cohort of mice was left undisturbed in the test room throughout the entire duration of the experiments. Trunk blood was then collected according to the procedures described below, in a hood providing insulation of sound and odor from the rest of the room. For collection of tail blood, all mice were transported to the test room, allowed to acclimatize for 30 min and handled briefly (∼2 min) for a duration of 3 days, to achieve habituation to handling stress prior to restraint studies and blood sampling. The mice were then restrained for 3 h on day 4, as described above, released from the DecapiCone, and returned to the home cage prior to having tail vein blood sampled within 5 min of release. Unstressed control counterparts were subjected to identical handling and habituation procedures and remained undisturbed in their home cages for 3 h prior to tail blood collection. All experiments were carried out between 11 a.m. and 3 p.m. (between 5 and 9 h into the 12-h light phase).
Chronic restraint stress for 7 days
Three days prior to initiation of stress all mice were transported to the behavioral testing room, allowed to acclimatize for 30 min and habituated to handling for 3 days as described above. On the last day of habituation, all mice had tail vein blood sampled as described below. On the first day of restraint stress, mice were transported to the test room in their home cages, acclimatized for 30 min and restrained for 2 h according to the procedures described above. The mice were then released from the restraint, sampled for tail vein blood (within 5 min) or handled for sham blood collection (∼2 min) on the days that did not require blood collection. The mice were then returned to their home cages and transported to the colony room. The procedure followed on day 1 was continued for 7 consecutive days with blood collections made on 1, 4 and 7 days of restraint stress. All experiments were carried out between 11 a.m. and 3 p.m. (between 5 and 9 h into the 12-h light phase).
Chronic restraint stress for 21 days
Three days prior to initiation of stress, all mice were transported to the behavioral testing room, allowed to acclimatize for 30 min and habituated to handling for 3 days as described above. On the last day of habituation, all mice had tail vein blood sampled as described below. On the first day of restraint stress, all mice were transported to the test room in their home cages, acclimatized for 30 min and restrained (restraint group) or allowed to remain undisturbed in their home cages (unrestrained control group) for 2 h according to the procedures described above. The mice were then sampled for tail vein blood (within 5 min of release from restraint, or immediately upon removal from the home cage in the case of unrestrained controls). All mice were handled for sham blood collection (∼2 min) on the days that did not require blood collection. The mice were then returned to their home cages and transported to the colony room. The procedure followed on day 1 was continued for 21 consecutive days with blood collections made on 1, 7, 14 and 21 days of restraint stress. All experiments were carried out between 11 a.m. and 3 p.m. (between 5 and 9 h into the 12 h light phase).
Body weight and food consumption
All mice (control and stress group) were weighed daily throughout the entire duration of experimental testing. Percentage weight loss was calculated as the weight of the mouse on the indicated day following initiation of the chronic restraint protocol, divided by the weight of the mouse on day 0 prior to initiation of the chronic restraint protocol, times 100. Food consumption of standard chow was determined daily, in some experiments, by weighing the food pellets (in grams) and determining the amount of food consumed by each mouse over a 24 h period.
Blood sampling and determination of plasma corticosterone levels
For tail vein blood sampling, sharp surgical scissors were used to remove ∼1–2 mm of the most distal portion of the tail, while the mouse was loosely held but not restrained by the tail. The tail was gently massaged to stimulate blood flow, and blood (approximately 20 μl) was collected directly into heparinized 40 mm microhematocrit tubes (SafeCrit, IRIS International, Inc., Westwood, MA). Blood flow was stopped by cauterizing the wound with silver nitrate applicator swabs (GF Health Products, Atlanta, GA) and applying gentle finger pressure before the animal was returned to its home cage. Plasma was then collected by centrifugation (Micro-hematocrit centrifuge, IRIS International, Inc., Chatsworth, CA) and stored at −80 °C. For trunk blood collections, mice were decapitated, while still restrained or transferred rapidly from their home cages, placed into a DecapiCone and decapitated within 20 s with the use of a sharp heavy scissors. The collected trunk blood was allowed to coagulate at room temperature for 1 h, chilled on ice, centrifuged for 10 min, and the supernatant re-centrifuged for 5 min at 10,000g to collect serum. Corticosterone levels in serum were then determined using the DetectX corticosterone ELISA kit (Arbor Assays, Ann Arbor, MI) according to the manufacturer's instructions. In some experiments, corticosterone levels were measured by radioimmunoassay as previously described (Lehmann et al., Citation2013), and no systematic differences between the results obtained in either assay system were noted.
Home-cage monitoring of locomotor activity
Naïve mice individually housed for at least 24 h, and not previously subjected to any stress paradigms or behavioral testing, were transported to the behavioral testing room and placed into the home cage monitoring system (CleverSys Inc., Reston, VA). Mice were transferred to cages containing bedding from the home cage, and side capture video recording carried out for 23 h. Distance traveled per 30 min over a 23-h period was derived using the standard master settings file provided by the manufacturer.
Immunohistochemistry
Mice restrained according to the procedures described above, or left undisturbed in their home cages for 3 h, were overdosed with Avertin, and immediately transcardially perfused with ice-cold saline supplemented with 5 mM EGTA followed by ice-cold 4% paraformaldehyde (PFA) prepared in 0.1 M phosphate buffer (pH 7.4). Following the perfusion, the brains were removed, post-fixed for an additional 24 h with 4% PFA and then coronally sectioned with a vibratome at a thickness of 35 μm. Free-floating sections were then washed and permeabilized with Tris-buffered saline supplemented with 0.5% Triton X-100, blocked with 10% normal goat serum and incubated overnight at 4 °C under gentle agitation with primary Fos antibody (1:3000, Ab-5, Calbiochem, EMD Millipore, Billerica, MA). The sections were then incubated for a further 2 h at room temperature with Alexa 488-conjugated anti-rabbit-IgG (1:300; Invitrogen, Life Technologies, Grand Island, NY) secondary antibody, prior to being mounted in Vectashield mounting medium with DAPI (catalog number H-1200; Vector Laboratories). Representative sections of the PVN of the hypothalamus (2–4 sections, bregma −0.70 to −0.94) were then visualized for fluorescence using a confocal microscope (Zeiss LSM 510, Carl Zeiss Microscopy, LLC, Thornwood, NY). Images were converted to 16-bit greyscale and the number of Fos positive cells quantitated using the NIH ImageJ software (NIH, Bethesda, MD). Images were thresholded to highlight Fos positive nuclei and subjected to “Analyze Particles” by setting “size (pixel ○2)” as 10-infinity and “circularity” as 0.0–1.00. Fluorescence intensity for Fos immunoreactivity (IR) in PVN was also quantified and compared across the groups.
Statistical analysis
Data were analyzed for statistical significance by two-way ANOVA followed by Bonferroni post hoc test using SigmaPlot 11.0 (Systat Software, Inc., San Jose, CA). Repeated measures were conducted using STATISTICA (StatSoft, Inc., Tulsa, OH).
Results
PACAP but not PAC1 deficiency attenuates CORT elevation, with corresponding effect on PVN Fos induction, in ARS
Elevation in CORT levels upon ARS for 3 h, as previously reported, was significantly less in PACAP-deficient, compared to wild-type mice (, gene effect: F(1,37) = 17.414, p < 0.001; stress effect: F(1,37) = 160.521, p < 0.001; gene × stress: F(1, 37) = 5.294, p = 0.027; two-way ANOVA followed by Bonferroni post hoc test to compare PACAP+/ + vs. PACAP−/− within RS, p < 0.001), but has no effect on circulating CORT in PAC1-deficient mice (, gene effect: F(1,40) = 0.0071, p = 0.933).
Figure 1. Effects of PACAP (A) and PAC1 (B) deficiency on CORT elevation after ARS in mouse. CORT was sampled from the tail, after 3 h restraint in PACAP- and PAC1-deficient mice and wild-type male mice. ARS significantly decreases CORT levels in PACAP-deficient mice (***p < 0.001), but has no effect on CORT in PAC1-deficient mice (ns, not significant). Results are expressed as mean ± SEM (n = 9–11 per group). NRS, without restraint stress control; RS, with restraint stress.
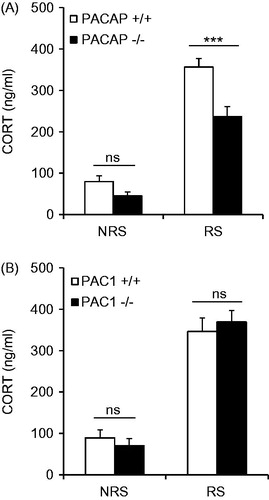
The blunting effect of PACAP deficiency on serum CORT elevation when sampled from the tail vein, while significant, was less than the effect on CORT elevation previously reported, when trunk blood was sampled (Lehmann et al., Citation2013; Stroth & Eiden, Citation2010; Tsukiyama et al., Citation2011). We repeated 3-h ARS in both PACAP- and PAC1-deficient mice, and their wild-type counterparts, with collection of trunk blood at time of euthanasia. Both PACAP- and PAC1-deficient mice showed a significant attenuation of CORT elevation, when sampled from trunk blood, compared to control mice (Supplementary Figure 1A, gene effect: F(1,37) = 66.757, p < 0.001; stress effect: F(1,37) = 137.51, p < 0.001; gene × stress: F(1,37) = 56.968, p < 0.001; two-way ANOVA followed by Bonferroni post hoc test to compare PACAP+/ + vs. PACAP−/− within RS, p < 0.001. Supplementary Figure 1B, gene effect: F(1,36) = 24.157, p < 0.001, stress effect: F(1,36) = 100.989, p < 0.001; gene × stress: F(1,36) = 5.829, p = 0.021; two-way ANOVA followed by Bonferroni post hoc test to compare PAC1+/ + vs. PAC1−/− within RS, p < 0.001). Furthermore, as previously reported for PACAP-deficient mice, PAC1-deficient mice showed no attenuation of CORT elevation after LPS challenge, even when trunk blood is collected following decapitation (data not shown). In the remaining experiments reported here, CORT levels were assessed following tail vein, rather than trunk blood, sampling.
Given the apparent lack of effect of PAC1 receptor utilization on CORT elevation following ARS, we focused on central effects of PACAP and PAC1 deficiency unlikely to be affected via the splanchnic nerve. Corticotrophin-releasing hormone (CRH)-elaborating neurons of the PVN of the hypothalamus transduce both systemic and psychogenic stress responses, whether acute or chronic, in mammals. Restraint stress increases Fos expression in CRH-expressing neurons of the PVN, and this increase is blunted in PACAP-deficient mice (Tsukiyama et al., Citation2011). Using Fos protein expression as an index of activation of these neurons, we examined PVN neuronal activation after ARS, in PACAP- and PAC1-deficient mice compared to their wild-type counterparts, after 3 h of restraint (). Both the number of Fos-positive neurons in the PVN, and the relative intensity of Fos expression within them, was dramatically increased by 3 h restraint (). Activation was decreased more than 50% in PACAP-deficient mice, but less than 25% in PAC1-deficient mice (, two-way ANOVA to compare the number of Fos-positive neurons, gene × stress: F(1,16) = 95.281, p < 0.001 for PACAP mice; F(1,20) = 12.117, p = 0.003 for PAC1 mice. For relative intensity of Fos expression, gene × stress: F(1,16) = 218.233, p < 0.001 for PACAP mice; F(1,20) = 12.662, p = 0.002 for PAC1 mice).
Figure 2. PACAP- and PAC1-deficient mice differentially affect ARS-induced Fos activation in PVN neurons. (A) Representative micrographs of Fos staining in PVN within the eight experimental groups. Fos activation was significant in wild-type mice after 3 h restraint stress. However, depletion of either PACAP or PAC1 attenuated Fos activation. Effect of PAC1 deficiency is much smaller than that of PACAP. Fos staining shown in right panels, and DAPI staining of same section shown in left panels, for each of the eight groups. WT, wild-type; KO, knock-out. 3V, third ventricle. Scale bars, 200 µm. (B) Quantification of Fos cellular immunostaining within PVN. Upper panels: cell counting of Fos + neurons; Lower panels: Relative Fos IR (normalized to corresponding non-restrained wild-type mice). Results are expressed as mean ± SEM (n = 4–6 per group). Two-way ANOVA followed by Bonferroni's post hoc test. Bonferroni's test: ***p < 0.001; ns, not significant.
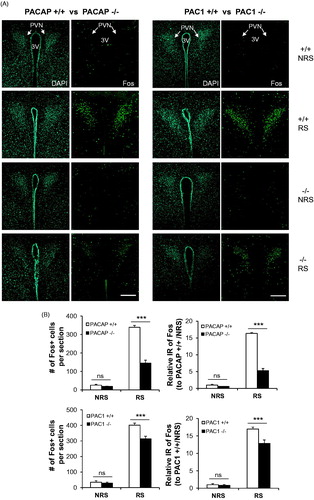
PACAP and PAC1 deficiency differentially affect CORT elevation and weight reduction as a function of chronic stress exposure across a 7-day period
If the effects of PACAP neurotransmission at the level of the PVN during stress responding are exerted largely on CRH biosynthesis subsequent to neuronal activation, as previously suggested by Stroth et al. (Citation2011b), the effects of PACAP deficiency on sustained CORT elevation during chronic stress would be predicted to become progressively more prominent, as initially reported by our laboratory in the context of chronic social defeat (Lehmann et al., Citation2013). We measured CORT levels prior to the onset of chronic restraint stress (CRS), and on days 1, 4 and 7 during 1 week of daily (2 h per day) restraint (see for experimental design). In PACAP-deficient mice, there was a significant diminution of CORT elevation measured immediately following cessation of the restraint session, which increased as a function of repeated restraint across the 7-day period (, PACAP+/ + vs. PACAP−/−, F(4,37) = 28.10, p < 0.001, repeated measures one-way ANOVA followed by Bonferroni post hoc test, p > 0.05 on day 0, however, p < 0.001 on day 1, or 4 or 7). In contrast, elevation of CORT following restraint was not significantly altered in PAC1-deficient mice until day 7 of chronic restraint (, PAC1+/ + vs. PAC1−/−, F(4,13) = 1.697, p = 0.210, repeated measures one-way ANOVA followed by Bonferroni post hoc test, p > 0.05 on day 0, 1 and day 4; on day 7, p = 0.043).
Figure 3. CORT elevation and body weight during 7-day (2 h/day) chronic restraint in PACAP- and PAC1-deficient mice. (A) Schematic representation of experimental design and timeline for 7-day chronic stress. Animals were single-housed for over a week and habituated to handling for 3 days prior to restraint stress. CRS was administered for a 2-h duration daily over 7 consecutive days. Tail blood was systemically collected on days 0, 1, 4 and 7 of CRS for CORT assay. Body weight and food consumption were determined daily. (B) CORT elevation during 7-day restraint-effect of PACAP deficiency. CORT was significantly elevated across 7 days for wild-type group upon CRS. PACAP deficiency significantly reduced this elevation. Repeated measures one-way ANOVA followed by Bonferroni's post hoc test, n = 21 per group; ***p < 0.001; ns, not significant. (C) CORT elevation during 7-day restraint-effect of PAC1 deficiency. CORT was significantly elevated across 7 days for wild-type group upon CRS. PAC1 deficiency significantly reduced this elevation only at day 7. Repeated measures one-way ANOVA followed by Bonferroni's post hoc test, n = 9 per group; *p < 0.05; ns, not significant. (D) Weight loss during 7-day restraint-effect of PACAP deficiency. CRS led to significant weight loss in wild-type restrained mice when measured on day 7 post-stress, but not in PACAP-deficient mice. Results are expressed as mean ± SEM (n = 20–21 for each group). Student's t-test: ***p < 0.001. (E) Weight loss during 7-day restraint-effect of PAC1 deficiency. CRS led to significant weight loss in both wild-type and PAC1-deficient restrained mice when measured on day 7 post-stress, and weight loss in wild-type was significantly greater than in PAC1-deficient mice. Results are expressed as mean ± SEM (n = 9 for each group). Student's t-test: *p < 0.05.
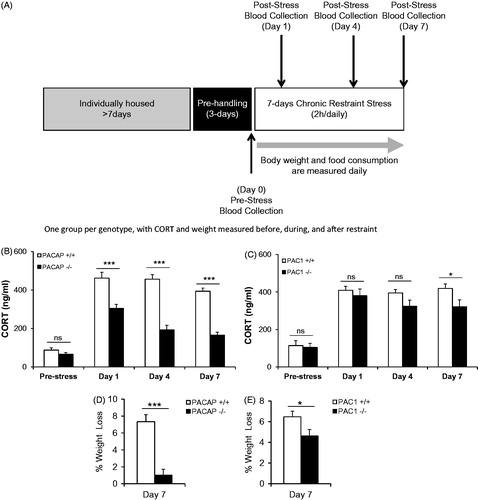
The effects of 7-day CRS on CORT elevation were paralleled by weight loss in wild-type, but not PACAP-deficient mice (, p < 0.001, Student's t-test), while in PAC1-deficient mice only a modest protection from the effects of restraint on weight loss was observed (, p = 0.039, Student's t-test).
In order to control for potential effects of differential locomotor activity, or metabolic effects, on body weight during the 7-day CRS period, locomotor activity was measured in both PACAP- and PAC1-deficient mice and their wild-type counterparts. As reported previously, PACAP-deficient mice were considerably more active during a 23-h period than wild-type mice (p < 0.001, Mann–Whitney rank sum test, ), while locomotor activity of PAC1-deficient mice was similar to their wild-type counterparts (p = 0.106). Thus, greater weight loss in PAC1-deficient, compared to PACAP-deficient mice, could not be attributed to a higher level of activity of these mice. Furthermore, direct measurement of food intake in wild-type mice subjected to stress demonstrated that weight loss was a direct consequence of hypophagia, and this stress-related decrease in food intake was not observed in PACAP-deficient animals (, gene effect: F(1,57) = 37.338, p < 0.001; stress effect: F(1,57) = 4.941, p = 0.03; two-way ANOVA followed by Bonferroni post hoc test to compare NRS vs. RS, p = 0.048 for PACAP+/+, p = 1 for PACAP−/−).
Figure 4. Locomotor activity in PACAP- and PAC1-deficient mice, and their wild-type counterparts. PACAP−/− mice were more hyperactive during a 23-h period (lights off during half-hour bins 14–38) than wild-type mice, while locomotor activity of PAC1−/− mice was similar to wild-types. N = 4–5 for each group.
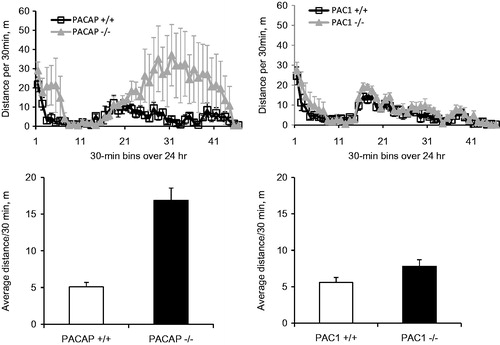
Figure 5. Lack of weight loss after chronic restraint in PACAP−/− mice is due to reversal of decreased food intake. Measurement of food intake in PACAP+/ + mice subjected to stress demonstrated that weight loss was a direct consequence of hypophagia, and this stress-related decrease in food intake was not observed in PACAP−/− (PACAP-deficient) mice. Results are expressed as mean ± SEM (n = 14–15 per group). Two-way ANOVA followed by Bonferroni's post hoc test. Bonferroni's test: *p < 0.05; ns, not significant.
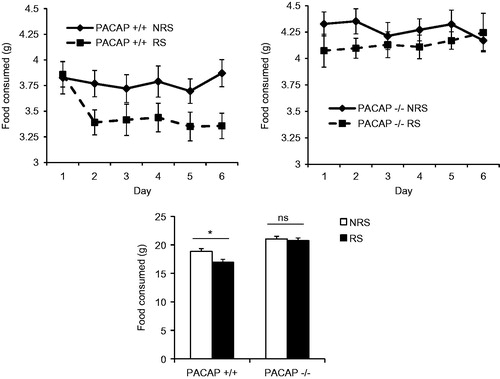
PACAP and PAC1 deficiency affect CORT elevation and weight reduction as a function of chronic stress exposure across a 21-day period
HPA axis elevation and weight loss as a consequence of 7 days of continuous daily restraint was not significantly attenuated in PAC1-deficient mice until day 7, and even at day 7 represented a significantly smaller effect of genotype for PAC1-deficient mice compared to PACAP-deficient mice (). Since previous reports indicate that some behavioral effects of chronic stress emerge more robustly at longer periods than 1 week in some rodent models (Strekalova et al., Citation2011), additional experiments were performed over a longer period of chronic stress, and the effects of genotype (PACAP and PAC1 deficiency) were examined (). In these experiments, unrestrained mice of all genotypes were maintained under identical conditions (daily transport to behavioral stimulus room, but without restraint) so that comparisons of both body weight and CORT levels would be tightly temporally controlled. By day 14 of restraint, and continuing for an additional week, the effect of PACAP deficiency on CORT elevation progressed from a significant decrease compared to CORT levels in wild-type mice, but still a significant elevation compared to unrestrained mice, to a mild elevation upon restraint that was not significantly greater than CORT levels in unrestrained mice (; repeated measures two-way ANOVA, gene effect: F(3,12) = 37.631, p < 0.001; stress effect: F(3,12) = 90.280, p < 0.001; gene × stress: F(3,12) = 41.642, p < 0.001. Bonferroni post hoc test, PACAP+/ + vs. PACAP−/− within RS, p < 0.001 on day 7, day 14 and day 21; RS vs. NRS within PACAP−/−, p = 0.082 on day 7, p = 0.135 on day 14, p = 1 on day 21).
Figure 6. CORT elevation and body weight during 21-day (2 h/day) chronic restraint in PACAP- and PAC1-deficient mice and wild-type male mice. (A) Schematic representation of experimental design and timeline for 21-day chronic stress. Animals were single-housed for over a week and habituated to handling for 3 days prior to restraint stress. CRS was administered for a 2-h duration daily over 21 consecutive days. Control cohort of animals was subjected to identical conditions except that they remained undisturbed in their home cages in the test room for the entire duration of CRS. Tail blood collection and weight measurement were conducted on days 7, 14 and 21 post restraint stress. Body weight and food consumption were determined daily. (B) CORT elevation during 21-day restraint-effect of PACAP deficiency. CORT was significantly elevated on days 7, 14 and 21 for wild-type group upon CRS, and PACAP-deficient restrained group was both significantly different than control restraint group, and not significantly different from either the wild-type or the PACAP-deficient unrestrained group. Repeated measures two-way ANOVA followed by Bonferroni's post hoc test, n = 4–5 per group; ***p < 0.001; ns, not significant. (C) CORT elevation during 21-day restraint-effect of PAC1 deficiency. CORT was significantly elevated on days 7, 14 and 21 for wild-type group upon CRS; and PAC1-deficient restrained group was significantly different than wild-type restrained group, and significantly different from unrestrained groups. Repeated measures two-way ANOVA followed by Bonferroni's post hoc test, n = 9–10 per group; ***p < 0.001; **p < 0.01; ns, not significant. (D) Weight loss during 21-day restraint-effect of PACAP deficiency. CRS led to significant weight loss in wild-type restrained mice when measured on days 7, 14 and 21 post-stress, but not in PACAP-deficient mice. Results are expressed as mean ± SEM (n = 8–9 for each group). Repeated measures two-way ANOVA followed by Bonferroni's post hoc test: ***p < 0.001; **p < 0.01; ns, not significant. (E) Weight loss during 21-day restraint-effect of PAC1 deficiency. CRS led to significant weight loss in both wild-type and PAC1-deficient restrained mice when measured on days 7, 14 and 21 post-stress, with weight loss in restrained PAC1-deficient mice significantly less than in restrained wild-type mice. Results are expressed as mean ± SEM (n = 9–10 for each group). Repeated measures two-way ANOVA followed by Bonferroni's post hoc test: ***p < 0.001.
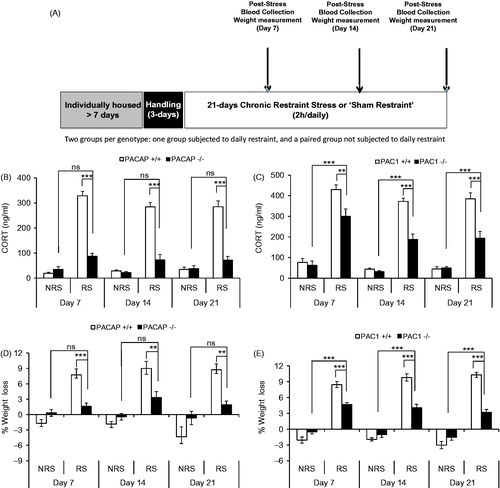
PAC1-deficient mice also showed a progressive attenuation of CORT elevation, in response to restraint, that was significantly less than CORT elevation following restraint in wild-type mice, but still significantly greater than CORT levels in unrestrained (control) wild-type mice maintained under otherwise identical conditions of husbandry during the 3-week period of chronic restraint (; repeated measures two-way ANOVA, gene effect: F(3,33) = 15.234, p < 0.001; stress effect: F(3,33) = 92.972, p < 0.001; gene × stress: F(3,33) = 13.335, p < 0.001. Bonferroni post hoc test, PAC1+/ + vs. PAC1−/− within RS, p < 0.01 on day 7, p < 0.001 on day 14 and day 21; RS vs. NRS within PAC1−/−, p < 0.001 on day 7, day 14 and day 21).
Both PACAP- and PAC1-deficient mice showed significant resistance to weight loss as a consequence of restraint over the last 2 weeks of the 3-week chronic restraint period, albeit the effect of PAC1-deficiency was quantitatively less than that of PACAP deficiency (, repeated measures two-way ANOVA, gene effect: F(3,28) = 1.984, p = 0.139; stress effect: F(3,28) = 20.688, p < 0.001; gene × stress: F(3,28) = 9.332, p < 0.001; Bonferroni post hoc test PACAP+/ + vs. PACAP−/− within RS, p < 0.001 on day 7, p < 0.01 on day 14 and day 21; RS vs. NRS within PACAP−/−, p > 0.05 on day 7, day 14 and day 21. , repeated measures two-way ANOVA, gene effect: F(3,33) = 8.213, p < 0.001; stress effect: F(3,33) = 104.153, p < 0.001; gene × stress: F(3,33) = 18.119, p < 0.001. Bonferroni post hoc test, PAC1+/ + vs. PAC1−/− within RS, p < 0.001 on day 7, day 14 and day 21; RS vs. NRS within PAC1−/−, p < 0.001 on day 7, day 14 and day 21).
Discussion
A large body of evidence implicates the neurotransmitter PACAP in both peripheral and central neurotransmission underlying stress responding. The data presented in this report provide important new features of PACAP neurotransmission in stressor responding mediated centrally and peripherally, and in stressor responding initiated either by systemic stressors, or by psychogenic stressors.
The main findings of our report are that there is a requirement for PACAP, but not for PAC1 expression, in mediating HPA axis activation during ARS, and a major role for PACAP, with progressively greater involvement of PAC1, in mediating HPA axis activation, and hypophagia, during CRS. Hypophagia is considered an index of anhedonia/depression in mouse models (Lim et al., Citation2012), along with sucrose preference which could not, however, be measured in our study due to potential secondary effects on caloric intake and body weight.
Previous studies have convincingly demonstrated that PACAP is the “stress transmitter” released by the splanchnic nerve during high-frequency stimulation occasioned by increased sympathetic outflow secondary to either systemic or psychogenic stress, and that the peripheral neurotransmitter actions of PACAP at the adrenomedullary synapse occur via engagement of the PAC1 receptor (Kuri et al., Citation2009; Stroth & Eiden, Citation2010; Stroth et al., Citation2013). Our results demonstrate that PAC1 receptor deficiency does not affect CORT secretion during ARS. The data do, however, uncover a mechanism for a PAC1-dependent, PACAP-dependent agonal effect on serum CORT elevation after ARS mediated peripherally by massive splanchnic disinhibition upon decapitation (Mravec et al., Citation2012; Popper et al., Citation1977). This must be accounted for in assessing both PACAP- and PAC1-dependence of the overall stress response in vivo. Specifically, we hypothesize that a PACAP- and PAC1-dependent increase in the biosynthesis of CORT within the adrenal cortex, and its release upon splanchnic disinhibition during decapitation, may cause misattribution of central versus peripheral mediation of stress responses within the HPA axis. Numerous experiments in both rats and mice are carried out via trunk blood sampling for CORT, on the assumption that agonal effects on CORT secretion, if they occurred, would be manifest in both stressed and unstressed cohorts (see, e.g. Barnum et al., Citation2007). Since this does not appear to be the case, blood sampling by tail vein rather than via collection of trunk blood in experiments examining the effects of neuropeptides including PACAP on both acute and chronic stress responding, may be preferred to avoid conflation of effects occurring during life, and agonal effects, on CORT secretion in such experiments.
The data presented here, in our view, argue for a major role of PACAP neurotransmission, likely at the level of the PVN, in supporting sustained HPA axis activation during chronic stress, and that this action is mediated by multiple PACAP receptors, with PAC1 being the dominant receptor for PACAP during prolonged chronic psychogenic stress. Determining which additional receptors are involved in earlier phases of PACAP-dependent stress response will require parallel studies with VPAC1- and VPAC2-deficient mice. It must also be remarked that the evidence in favor of a role of PACAP transmission at extrahypothalamic sites, via PAC1 receptor activation, in mediating anxiety associated with chronic variate stress, adduced mainly in rat experimental models, is quite strong (Hammack et al., Citation2009, Citation2010). It will be imperative, in the near future, to establish whether the effects of chronic stress on anhedonia and anxiety, both associated with depression as clinically diagnosed, can be dissociated based on dependence on PAC1 receptor utilization, and on the region of the brain mediating these effects. PACAP effects on depression-related behaviors, such as hypophagia, may well be independent, both in terms of causality and circuitry, of PACAP effects on the HPA axis. However, the observed fact that PACAP and PAC1 receptor neurotransmission affect both offers a new avenue of opportunity to perform genetically-based regional lesions in PACAPergic circuitry in mouse brain to determine whether this is the case. Such studies may have the added virtue of defining more precisely what effects of PACAP neurotransmission are more likely to be adaptive, or maladaptive, in response to a given stressor, and thus provide a biological guide to the development of PACAP-related therapeutic approaches to depression and anxiety disorders.
Finally, we wish to provide a caveat, based on the results herein, of the hypothesis put forward by ourselves and others that PACAP neurotransmission is important in mediating the behavioral effects of psychogenic stress, but not systemic stress, because of a differential utilization of PACAPergic and non-PACAPergic circuits in activation of the HPA axis by each stressor modality (Hamelink et al., Citation2002b; Lehmann et al., Citation2013; Tsukiyama et al., Citation2011). The differential effect of PACAP on CORT elevation following an acute psychogenic (restraint), compared to a systemic (LPS) stressor is maintained whether CORT is measured in peripheral blood, or by sampling of trunk blood. However, this differential effect is far more modest when apparent agonal effects of trunk blood sampling are taken into account. Thus, it will be of considerable interest, in future experiments, to compare the robust dependence of chronic psychogenic stress on PACAP neurotransmission, to that of chronic, rather than acute systemic stress.
In summary, we have provided evidence that PACAP mediates the central effects of stress on sustained CORT elevation across the period of application of CRS, and that the PAC1 receptor plays an increasingly important role in transduction of PACAP signaling with longer periods of chronic stress. We believe these findings are of great translational significance for two reasons. First, the finding of a requirement for PACAP expression in the depressogenic effects of chronic stress in both chronic social defeat and CRS increases the likelihood that PACAP neurotransmission might be required for the depressive effects of chronic stress in humans. CRS presents a maximally robust and efficient test for in vivo screening of PACAP antagonists in treatment of behavioral disorders associated with chronic stress. Second, the requirement for PAC1 receptor expression in HPA responsiveness to chronic, but not acute psychogenic stress, and the apparently more penetrant genotypic effect of PACAP than of PAC1 deficiency, argues for further inquiry into the role(s) of the VPAC1 and VPAC2 receptors in mediating the neurotransmitter effects of PACAP during chronic psychogenic stress.
Declaration of interest
We acknowledge support from the National Institute of Health (NIMH) Intramural Research Program (1ZIAMH002386); the Brain and Behavior Foundation (NARSAD Young Investigator Award to Tomris Mustafa). The authors declare no conflicts of interest.
Supplementary material available online Supplementary Figure 1
Supplemental Material.pdf
Download PDF (79.3 KB)Acknowledgements
We thank David Huddleston and Chang-Mei Hsu for assistance with management of mouse colonies, genotyping and conducting quantitative PCR.
References
- Agarwal A, Halvorson LM, Legradi G. (2005). Pituitary adenylate cyclase-activating polypeptide (PACAP) mimics neuroendocrine and behavioral manifestations of stress: evidence for PKA-mediated expression of the corticotropin-releasing hormone (CRH) gene. Brain Res Mol Brain Res 138:45–57
- Ait-Ali D, Stroth N, Sen JM, Eiden LE. (2010). PACAP-cytokine interactions govern adrenal neuropeptide biosynthesis after systemic administration of LPS. Neuropharmacology 58:208–14
- Barnum CJ, Blandino P Jr, Deak T. (2007). Adaptation in the corticosterone and hyperthermic responses to stress following repeated stressor exposure. J Neuroendocrinol 19:632–42
- Dore R, Iemolo A, Smith KL, Wang X, Cottone P, Sabino V. (2013). CRF mediates the anxiogenic and anti-rewarding, but not the anorectic effects of PACAP. Neuropsychopharmacology 38:2160–9
- Grinevich V, Fournier A, Pelletier G. (1997). Effects of pituitary adenylate cyclase-activating polypeptide (PACAP) on corticotropin-releasing hormone (CRH) gene expression in the rat hypothalamic paraventricular nucleus. Brain Res 773:190–6
- Hamelink C, Lee H-W, Hsu C-M, Eiden LE. (2002a). Role of protein kinases in neuropeptide gene regulation by PACAP in chromaffin cells – a pharmacological and bioinformatic analysis. Ann NY Acad Sci 971:474–90
- Hamelink C, Tjurmina O, Damadzic R, Young WS, Weihe E, Lee H-W, Eiden LE. (2002b). Pituitary adenylate cyclase activating polypeptide is a sympathoadrenal neurotransmitter involved in catecholamine regulation and glucohomeostasis. Proc Natl Acad Sci USA 99:461–6
- Hammack SE, Cheung J, Rhodes KM, Schutz KC, Falls WA, Braas KM, May V. (2009). Chronic stress increases pituitary adenylate cyclase-activating peptide (PACAP) and brain-derived neurotrophic factor (BDNF) mRNA expression in the bed nucleus of the stria terminalis (BNST): roles for PACAP in anxiety-like behavior. Psychoneuroendocrinology 34:833–43
- Hammack SE, Cooper MA, Lezak KR. (2012). Overlapping neurobiology of learned helplessness and conditioned defeat: implications for PTSD and mood disorders. Neuropharmacology 62:565–75
- Hammack SE, Roman CW, Lezak KR, Kocho-Shellenberg M, Grimmig B, Falls WA, Braas K, May V. (2010). Roles for pituitary adenylate cyclase-activating peptide (PACAP) expression and signaling in the bed nucleus of the stria terminalis (BNST) in mediating the behavioral consequences of chronic stress. J Mol Neurosci 42:327–40
- Hashimoto H, Shintani N, Tanaka K, Mori W, Hirose M, Matsuda T, Sakaue M, et al. (2001). Altered psychomotor behaviors in mice lacking pituitary adenylate cyclase-activating polypeptide (PACAP). Proc Natl Acad Sci USA 98:13355–60
- Hayashi T, Ikematsu K, Abe Y, Ihama Y, Ago K, Ago M, Miyazaki T, Ogata M. (2014). Temporal changes of the adrenal endocrine system in a restraint stressed mouse and possibility of postmortem indicators of prolonged psychological stress. Leg Med (Tokyo) 16:193–6
- Kocho-Schellenberg M, Lezak KR, Harris OM, Roelke E, Gick N, Choi I, Edwards S, et al. (2014). PACAP in the BNST produces anorexia and weight loss in male and female rats. Neuropsychopharmacology 39:1614–23
- Kuri BA, Chan SA, Smith CB. (2009). PACAP regulates immediate catecholamine release from adrenal chromaffin cells in an activity-dependent manner through a protein kinase C-dependent pathway. J Neurochem 110:1214–25
- Kwon MS, Seo YJ, Shim EJ, Choi SS, Lee JY, Suh HW. (2006). The effect of single or repeated restraint stress on several signal molecules in paraventricular nucleus, arcuate nucleus and locus coeruleus. Neuroscience 142:1281–92
- Lehmann ML, Mustafa T, Eiden AM, Herkenham M, Eiden LE. (2013). PACAP-deficient mice show attenuated corticosterone secretion and fail to develop depressive behavior during chronic social defeat stress. Psychoneuroendocrinology 38:702–15
- Lim BK, Huang KW, Grueter BA, Rothwell PE, Malenka RC. (2012). Anhedonia requires MC4R-mediated synaptic adaptations in nucleus accumbens. Nature 487:183–9
- Mravec B, Bodnar I, Uhereczky G, Kvetnansky R, Palkovits M. (2012). Effect of lesions of A5 or A7 noradrenergic cell group or surgical transection of brainstem catecholamine pathways on plasma catecholamine levels in rats injected subcutaneously by formalin. Gen Physiol Biophys 31:247–54
- Norrholm SD, Das M, Legradi G. (2005). Behavioral effects of local microinfusion of pituitary adenylate cyclase activating polypeptide (PACAP) into the paraventricular nucleus of the hypothalamus (PVN). Regul Pept 128:33–41
- Otto C, Martin M, Wolfer DP, Lipp HP, Maldonado R, Schutz G. (2001). Altered emotional behavior in PACAP-type-I-receptor-deficient mice. Brain Res Mol Brain Res 92:78–84
- Popper CW, Chiueh CC, Kopin IJ. (1977). Plasma catecholamine concentrations in unanesthetized rats during sleep, wakefulness, immobilization and after decapitation. J Pharmacol Exp Ther 202:144–8
- Ressler KJ, Mercer KB, Bradley B, Jovanovic T, Mahan A, Kerley K, Norrholm SD, et al. (2011). Post-traumatic stress disorder is associated with PACAP and the PAC1 receptor. Nature 470:492–7
- Roman CW, Lezak KR, Hartsock MJ, Falls WA, Braas KM, Howard AB, Hammack SE, May V. (2014). PAC1 receptor antagonism in the bed nucleus of the stria terminalis (BNST) attenuates the endocrine and behavioral consequences of chronic stress. Psychoneuroendocrinology 47:151–65
- Strekalova T, Couch Y, Kholod N, Boyks M, Malin D, Leprince P, Steinbusch HM. (2011). Update in the methodology of the chronic stress paradigm: internal control matters. Behav Brain Funct 7:9--26
- Stroth N, Eiden LE. (2010). Stress hormone synthesis in mouse hypothalamus and adrenal gland triggered by restraint is dependent on pituitary adenylate cyclase-activating polypeptide signaling. Neuroscience 165:1025–30
- Stroth N, Holighaus Y, Ait-Ali D, Eiden LE. (2011a). PACAP: a master regulator of neuroendocrine stress circuits and the cellular stress response. Ann NY Acad Sci 1220:49–59
- Stroth N, Kuri BA, Mustafa T, Chan SA, Smith CB, Eiden LE. (2013). PACAP controls adrenomedullary catecholamine secretion and expression of catecholamine biosynthetic enzymes at high splanchnic nerve firing rates characteristic of stress transduction in male mice. Endocrinology 154:330–9
- Stroth N, Liu Y, Aguilera G, Eiden LE. (2011b). Pituitary adenylate cyclase-activating polypeptide (PACAP) controls stimulus-transcription coupling in the hypothalamic-pituitary-adrenal axis to mediate sustained hormone secretion during stress. J Neuroendocrinol 23:944–55
- Tsukiyama N, Saida Y, Kakuda M, Shintani N, Hayata A, Morita Y, Tanida M, et al. (2011). PACAP centrally mediates emotional stress-induced corticosterone responses in mice. Stress 14:368–75