Abstract
Non-invasive methods to quantify components of stress in non-human animals rely typically on the use of physiological or behavioural measures. At the physiological level, stress is usually measured non-invasively in terms of faecal or urinary glucocorticoid output. A common group of behavioural measures used are self-directed behaviours (SDBs), which have been shown to be linked to anxiety, a subset of stress, although a number of authors have explicitly linked SDBs to stress more generally. Whether increased rates of SDBs are likely to be associated with increased faecal glucocorticoid ouput in wild mammals remains unclear. Here, for wild female olive baboons, we show no association between day-to-day changes in levels of SDB and cortisol metabolite excretion. We also show no relationship between long-term mean levels of these variables. We discuss several possible interpretations of our results, including the possibility that SDBs represent a behavioural coping mechanism, helping to ameliorate the physiological stress response.
Introduction
The development of non-invasive methods to assess different components of stress has provided a powerful tool for zoologists in a broad range of fields. Non-invasive techniques have been applied most frequently in studies of captive, free-ranging or wild mammals, for example to explore the stress related costs of group living (Sands and Creel Citation2004), to investigate the anxiety reducing benefits of conflict management (Schino Citation1998) or to assess the fitness impacts of elevated stress (Pride Citation2005). In addition, given the clear link between animals' well-being and the stress levels they experience, these approaches have also been used to address key applied questions relating to captive animal welfare (Honess and Marin Citation2006; Lane Citation2006). To date, two main techniques have been used to quantify stress non-invasively; these involve quantification of behavioural or physiological measures (Honess and Marin Citation2006).
There is evidence that self-directed behaviours (SDBs), such as self-scratching, self-grooming, yawning and body shaking, can provide an index of a subset of stress, namely anxiety (Maestripieri et al. Citation1992). While some of these behaviours, particularly self-grooming or scratching, may ordinarily have hygienic functions (Maestripieri et al. Citation1992; Ventura et al. Citation2005), a range of studies have found that they increase markedly above baseline levels in anxiety-inducing situations. For example, long-tailed macaques (Macaca fascicularis) given anxiogenic drugs show increased SDBs (Schino et al. Citation1996). Rates of SDB have also been shown to increase in domestic goats (Capra hircus) following aggression (Schino Citation1998) and among female olive baboons (Papio hamadryas anubis) when in close proximity to a dominant individual (Castles et al. Citation1999). Some authors have, however, gone beyond linking SDBs to anxiety only, and have explicitly linked SDBs to stress more generally (e.g. Troisi Citation2002). As a consequence, SDB measures are now often taken to be indicative of a wider and more general level of stress (Elder and Menzel Citation2001; Owen et al. Citation2004; Fraser et al. Citation2008).
Studies that include non-invasive physiological measures of stress most commonly assess faecal or urinary glucocorticoid output. While glucocorticoid levels can be affected by non-stress related events (Lane Citation2006), validations of this approach, in which known stressors such as anesthetisation are shown to cause direct increases in glucocorticoid output, show that these methods can be used to measure this aspect of the stress response (Heistermann et al. Citation2006). In addition, biological stressors experienced by animals in the wild have been shown to affect faecal glucocorticoid (FGC) levels in a range of species. For example, Engh et al. (Citation2006) found that FGC levels of lactating female chacma baboons (Papio hamadryas ursinus) rose when potentially infanticidal males entered the troop, and that actual infanticide caused a further rise in these levels. Similarly, elevated FGC output has been seen among wild European pine martens, Martes martes, exposed to high tourist pressure (Barja et al. Citation2007) and European rabbits, Oryctolagus cuniculus, exposed to the odour of a predator (Monclús et al. Citation2006).
One might expect that elevated levels of anxiety would ultimately be manifest as physiological stress, and that SDB and FGC measures would therefore be positively correlated in studies of wild mammals. The clinical literature provides some evidence for anxiety leading to a stress response involving cortisol secretion: administration of an anxiogenic benzodiazepine receptor antagonist to chair restrained rhesus macaques (Macaca mulatta) was associated with increased rates of SDBs (‘picking behaviour’), as well as increased circulating levels of both catecholamines and cortisol (Ninan et al. Citation1982). Clinical conditions such as these, however, provide little information about how behavioural and physiological measures might be related under natural circumstances. Moreover, a number of authors have proposed that SDBs may represent a coping strategy to reduce aspects of the physiological stress response (Maestripieri et al. Citation1992; Watson et al. Citation1999), such that SDB and faecal cortisol measures may be negatively associated, or unrelated.
To our knowledge there have been no extensive tests of the relationship between SDBs and glucocorticoid output in wild mammals. The few studies investigating the specific link between these two non-invasive measures in captivity have been conducted primarily with non-human primates, and have produced contradictory results. Peel et al. (Citation2005), for example, found a positive association between self-grooming rates and FGC concentration in a captive western lowland gorilla (Gorilla gorilla gorilla). By contrast, Ulyan et al. (Citation2006) found that imposition of an unpredictable feeding regime among captive brown capuchins (Cebus apella) was associated with an increase in faecal cortisol concentrations, but no change in rates of SDBs.
Working with wild female olive baboons (P. h. anubis) in Nigeria, we quantify rates of a number of different SDBs, and faecal concentrations of cortisol metabolites. We explore first the day-by-day relationship between these two measures, both across all females and within each female separately, and then investigate the association between mean levels over time. Although the captive environment affords a number of advantages when it comes to studying aspects of stress in non-human animals, studies carried out in the wild arguably allow a more appropriate investigation of a species' natural behavioural and physiological responses. A number of interesting parallels can be drawn between wild baboons and humans in the physiological mechanisms underlying stress responses and the impact of stress on health measures (Sapolsky Citation2005). Moreover, as there is growing interest in the use of SDBs as indicators of emotional state in humans (Troisi Citation2002), and as recent studies have begun to investigate the relationship between physiological and behavioural indices of stress and anxiety in humans (Pico-Alfonso et al. Citation2007), analogous data from non-human primates provide a comparative context in which the results of such studies can be interpreted.
Methods
We studied two troops of olive baboons at Gashaka-Gumti National Park (GGNP), Nigeria (Sommer et al. Citation2004), from April 2004 to April 2005. Kwano and Gamgam troops have been monitored since 2000, and all individuals are identifiable. Kwano troop consisted of 28 individuals during the study period, with behavioural data presented for all six adult females and one subadult cycling female. Gamgam troop consisted of 19 individuals during the study period, with behavioural data presented for three out of four adult females and one subadult cycling female. The following classification was used to assess reproductive state daily for each female (CitationHigham et al. 2007, 2008): Lactating—suckling an infant; pregnant—not cycling and showing reddening of the ano-genital area (AGA); S0—cycling with no swelling; S1—cycling with a small swelling of the AGA; S2—cycling with a medium-large swelling of the AGA and a small swelling of the para-callosal skin (PCS); S3—cycling with a large swelling of the AGA and full swelling of the PCS. This scheme was used to enable the consideration of different stages of the cycle independently, and to control for any cycle phase effects when creating female mean glucocorticoid values.
Faecal collection, hormone assays and interpretation
We collected a total of 890 faecal samples, aiming to collect samples every 1–2 days from individual females (Higham et al. Citation2008). Samples were collected directly after defecation in the early morning according to protocols in Hodges and Heistermann (Citation2003), with 81% of samples collected before 10:00 am. Although there was no cut-off point for sample collection, a paired t-test analysis of 19 occasions where we collected samples before 10:00 am and after 10:00 am from the same female on the same day, showed that samples collected later in the day did not differ significantly in glucocorticoid concentration from those collected before 10:00 am (t = 0.782, df = 18, p = 0.444). As such, all samples were used in analyses. Samples were homogenised on collection, and approximately 2 g (wet weight) of faeces were placed into 10 ml of >95% ethanol. Laboratory analyses were undertaken at Roehampton University. Faecal samples were extracted into 99.5% methanol using a double extraction method (based on Ziegler et al. Citation2000). The efficacy of the extraction procedure, determined by the recovery of tritiated estradiol added to 12 samples (not used elsewhere) prior to extraction, was 97.5% ± 2.9. Faecal extracts were analysed for 5β-androstane-3α, 11β-diol-17-one cortisol metabolites (3α,11β-dihydroxy-CM, hereafter FGCs), using an enzyme immuno-assay previously applied to monitor glucocorticoid output in other species of primates (e.g. Barbary macaque, Macaca sylvanus: Heistermann et al. Citation2006; Shutt et al. Citation2007; Assamese macaque, Macaca assamensis: Ostner et al. Citation2008; douc langur, Pygathrix nemaeus: Heistermann et al. Citation2004; Verraux's sifaka, Propithecus verrauxi: Fichtel et al. Citation2007), and validated for use in olive baboons using an ACTH challenge test (A. Daspre, M. Heistermann, L. Rosetta and P.C. Lee personal communication 2007). The assay was carried out on microtitre plates according to the procedure described in detail by Heistermann et al. (Citation2004). Sensitivity of the assays at 90% binding was 1.2 pg/well. Intra-assay variation, calculated from repeated measures of high and low concentration quality controls, was 7.5% (high) and 13.7% (low) while measures of inter-assay variation were 11.9% (high) and 16.5% (low). All assay results were standardised for differences in extraction volume and faecal weight, and are presented as nanograms of hormone per gram dry faecal weight.
Measuring behaviour
A total of 848 continuous focal behavioural observations, each lasting 45 min, were undertaken on separate females by two observers on Psion Workabout (www.psionteklogix.com) handheld hardware units, loaded with Noldus Observer software (www.noldus.com). A number of 45 min observations (range 2–9) were taken for a given female on a given day; these were spaced by a minimum 15 min intervening period, and considered discrete observations. Although observational data were collected by two different observers, comparisons of four pairs of observations undertaken on the same focal animal at the same time, showed a high degree of inter-observer reliability (all r ≥ 0.99, all p < 0.001), and observations were combined for analyses. All activity was recorded, including all SDB, which was also broken down into four constituent categories; self-grooming (autogrooming), self-scratching, shaking and yawning (following definitions by Schino et al. (Citation1988)). To assess dominance rank of the study females, we used data on aggressive and submissive interactions to construct a dyadic interaction table of ‘winners’ and ‘losers’. However, the linearity of rank, tested in MatMan (www.noldus.com), was not significant (Kwano troop, p = 0.70; Gamgam troop, p = 0.75; based on randomisation tests using 10,000 randomisations). As behavioural data did not reveal an order of dominance relationships within either troop, dominance rank was not included in any analyses.
Data treatment and analysis
Observation start times were skewed towards the earlier morning and later afternoon. However, this skew was not biased with respect to female ID, reproductive state, troop or observer. We averaged across all observations for a given female for a given day, to create a dataset of 298 ‘female days’. These female days were analysed for their relationships with FGC values using general linear mixed models, which controlled for multiple observations of the same female, and multiple observations of females from the same troops, by including both ‘Female ID’ and ‘Troop’ as random factors in all models. We investigated relationships between behavioural and physiological measures of stress in several ways as it is unclear whether the two measures might temporally coincide, or whether one might precede and initiate the other. In addition, there is also an excretion lag between blood hormone values, and values of hormone metabolites subsequently measured in faecal samples. In baboons, steroid hormone metabolites have been measured as first appearing around 18 h after infusion with tritiated forms, peaking in concentration between 24 and 48 h (with a mean peak at 36 h), and dropping to 40–60% of peak after 72 h (Wasser et al. Citation1994). We followed common practice in studies of non-invasive primate endocrinology, and used an excretion lag of 2 days (Heistermann et al. Citation1996; Engelhardt et al. Citation2005; Higham et al. Citation2008). FGC values were first investigated with respect to behavioural values using three combinations of relative timing of data collection for the two types of stress variables, taking the excretion lag into account in all cases: (1) behavioural data were assessed with respect to FGC values measured on that morning or the following morning only (i.e. within 1 day of the behaviour data). This assumes that higher plasma cortisol values precede higher SDB values; (2) behavioural data were assessed with respect to FGC values measured 2 days later, so assuming that increased levels of plasma cortisol and SDBs temporally coincide; (3) behavioural data were assessed with respect to FGC values measured 3–4 days later. This assumes that higher values of SDBs precede higher values of plasma cortisol. Following these analyses across all females, we investigated plots of FGC levels against SDB levels for each female separately under all three alignments, and looked for relationships between the two using two-tailed Pearson correlations. We also looked at plots of both FGC levels and all five measures of SDBs for each female, to see whether data might correlate if aligned in any other way, when taking into account both an excretion lag, and any delay in response of changes of one measure to the other. Finally, we also looked at subsets of the data, in which each subset contained only one female swelling state (S0–S3), so eliminating any potential effect of variation in female cycle stage.
In addition, we investigated female mean values of SDBs against mean values of FGCs. To get these mean values, we first averaged across all values for each female in each of the four swelling states (i.e. for each female, averaging across all her values for states S0–S3 separately). We then averaged across these four values to produce overall female means. Three females that were present in the dataset in only one or two of the four swelling states were excluded from the analysis of female means. For the remaining eight females, we used two-tailed Pearson correlations to compare mean FGC values against mean percentage time spent in total SDBs, and against mean percentage time spent self-grooming, self-scratching, yawning and shaking. Analyses were performed in R 2.2.1. (GLMMs) and SPSS 15.0. (Pearson correlations).
Results
There was marked variation in both SDBs and FGCs, both within () and between () females. None of the 15 GLMM analyses testing the three different alignments of FGC levels and the five measures of SDBs (total SDB, self-grooming, self-scratching, yawning or shaking) produced any significant relationships (all analyses p>0.2). Plots for individual females of the three different alignments showed no relationships between FGCs and any measure of SDBs (all analyses p>0.1; ). Graphical investigation of the day-by-day changes in FGC levels and all five measures of SDBs similarly failed to reveal any potential alignments that might produce correlations between the two types of measure. Analysis of data subsets for each swelling state (S0–S3) separately also revealed no significant relationships, indicating that cycle phase was not a confounding variable in the larger analyses. Female mean FGC values were similarly not related to mean values for any of the five SDB measures (total SDB, r = 0.391, p = 0.338, n = 8; self-scratching, r = 0.363, p = 0.377, n = 8; self-grooming, r = 0.394, p = 0.333, n = 8; yawning, r = − 0.312, p = 0.452, n = 8; shaking, r = − 0.209, p = 0.619, n = 8; ).
Figure 1 (A) Plot of FGC concentrations against total levels of SDB (Total SDB) for one female with FGC concentrations aligned to 2 days after Total SDB values to take into account an excretion lag into faeces and (B) FGC concentrations and Total SDB measured on each day over a 1-month period for the same female.
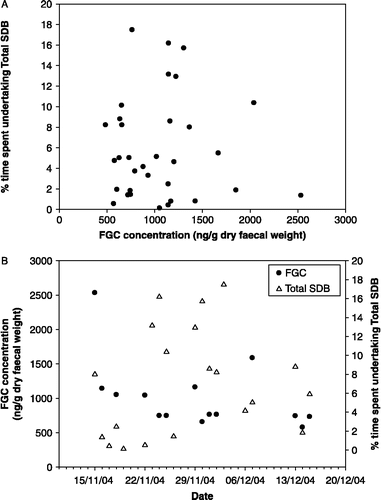
Figure 2 The relationship between individual female mean FGC concentrations and individual female mean: (A) Total SDB and (B) self-scratching. Relationships are not significant in two-tailed Pearson's correlations (Total SDB, r = 0.391, p = 0.338, n = 8; self-scratching, r = 0.363, p = 0.377, n = 8).
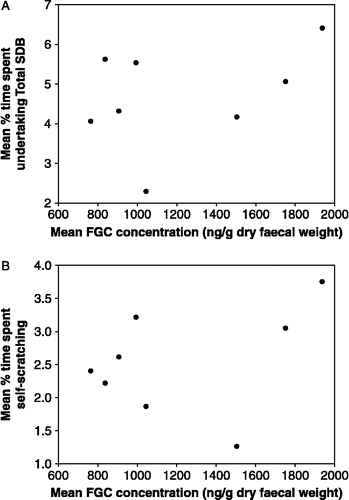
Discussion
In this study of wild female olive baboons we found no associations between day-by-day changes in FGC concentrations and changes in rates of total SDBs, self-grooming, self-scratching, shaking or yawning. Moreover, there were also no associations between mean values over time of FGC levels and any of the five measures of SDB. Thus, we find no evidence that behavioural indices of anxiety are related to a physiological measure of stress in our study animals.
Some authors explicitly describe SDB as an indicator of stress (e.g. Elder and Menzel Citation2001; Troisi Citation2002; Owen et al. Citation2004; Fraser et al. Citation2008). Our results suggest that caution should be exercised when interpreting patterns of variation in SDBs as necessarily being indicative of stress in terms of glucocorticoid production. This point is particularly pertinent to studies using SDBs as a measure of welfare (Powell et al. Citation2006; Carder and Semple Citation2008) or to studies using SDBs and glucocorticoid measures as alternatives, with study constraints determining the preferred approach (Fernström et al. Citation2008). Our findings indicate that in our study population at least, behavioural indices of anxiety cannot be used as a simple surrogate for glucocorticoid levels.
We can envisage four potential explanations for our results; these explanations are not mutually exclusive. First, SDBs may be related specifically to anxiety (Maestripieri et al. Citation1992), or to low levels of stress, rather than to high-impact or chronic stress, and they are not therefore associated with significant and/or prolonged elevations in glucocorticoid output. If this is the case, then it may be that assessing FGCs, as an integrated measure of glucocorticoid production, is not a method with sufficient sensitivity to detect any acute cortisol response associated with SDBs. Second, SDBs may be more associated with activation of the adrenal medulla rather than the adrenal cortex, and therefore increases in these behaviours may be linked to production of catecholamines rather than glucocorticoids. It is interesting to note in this regard that proximity of a dominant individual is known to lead to elevated heart rate (which is closely linked to catecholamine secretion) in macaques (Aureli et al. Citation1999) and increased SDB in baboons (Castles et al. Citation1999), although no studies have simultaneously assessed both of these measures.
A third potential explanation for our findings is that displacement activities such as SDBs may represent a successful behavioural coping strategy to reduce the physiological stress response. Evidence to support this latter hypothesis comes from work by Watson et al. (Citation1999), who studied scent marking as a displacement activity in the small eared bushbaby (Otolemur garnettii). These authors found that individuals exhibiting higher scent marking rates when exposed to a novel environment showed lower cortisol responses to subsequent restraint, indicating that scent marking may provide a mechanism for mediation of the physiological stress response. Further support for the idea that SDBs may act as coping behaviours is provided by a study of humans, in which it was found that women displaying higher levels of displacement behaviour during a stressful interview showed lower heart rate increments, and less marked vagal suppression, during the recovery period (Pico-Alfonso et al. Citation2007). Finally, the lack of association between SDB and FGC concentrations may reflect the fact that rates of expression of SDBs in this instance are more related to their hygienic (or other) function than they are to underlying emotional state. Variation between individuals in the use of SDBs for these purposes (e.g. differences between animals in self-grooming related to differences in their ectoparasite loads) may obscure any relationship between SDBs and glucocorticoid output. While this last type of explanation is particularly pertinent to comparisons involving mean SDB levels per individual, it is less likely to explain the absence of relationships at the intra-individual level where day-by-day changes in SDB and FGC were explored. Nevertheless, it is likely that measuring much shorter term changes from baseline in SDBs, rather than day-by-day changes or mean levels over time, may provide the most appropriate index of emotional state.
Our results highlight the value of considering carefully the aspect of stress that authors are trying to identify and measure, and making sure that the methods used are appropriate to reveal information about those aspects of an individual's state. Moreover, as studies highlighting the long-term fitness impacts of physiological stress focus on the effects of glucocorticoids, our findings indicate that animals exhibiting high levels of SDBs may not be as at risk of experiencing such impacts as may have been thought. Further studies quantifying both SDBs and stress hormones in diverse taxa are required to test the generality of our results across mammal species.
Acknowledgements
The authors would like to thank Nigerian National Parks Service for permission to undertake research in GGNP. NCF/WWF-UK provided field logistical support. Bobbo Buba, Halidu Ilyusu, Helen Cross and Alejandra Pascual Garrido helped collect data in the field. Balbir Singh Josen provided lab assistance at Roehampton University. Changiz Mohiyeddini provided valuable discussion, in addition to advice on statistics. Lauren Brent, John Russell and five anonymous reviewers provided constructive criticism of the manuscript. Funding came from Roehampton University and The Leakey Trust. The Chester Zoo Nigeria Biodiversity Programme provided core funding for key Gashaka Primate Project infrastructure Baboon faecal samples were imported into the UK under license from DEFRA (license number AHZ/2537/2004/1). This is GPP publication number 69.
Declaration of interest: The authors report no conflicts of interest. The authors alone are responsible for the content and writing of the paper.
References
- Aureli F, Preston SD, de Waal FBM. 1999. Heart rate responses to social interactions in free moving rhesus macaques (Macaca mulatta): A pilot study. J Comp Psychol. 113:59–65.
- Barja I, Silván G, Rosellini S, Piñeiro A, González-Gil A, Camacho L, Illera JC. 2007. Stress physiological responses to tourist pressure in a wild population of European pine marten. J Steroid Biochem Mol Biol. 104:136–142.
- Carder G, Semple S. Visitor effects on anxiety in two captive groups of western lowland gorillas. Appl Anim Behav Sci. 2008 115:211-210.
- Castles DL, Whiten A, Aureli F. 1999. Social anxiety, relationships and self-directed behaviour among wild female olive baboons. Anim Behav. 58:1207–1215.
- Elder CM, Menzel CR. 2001. Dissociation of cortisol and behavioural indicators of stress in an orang-utan (Pongo pygmaeus) during a computerised task. Primates. 42:345–357.
- Engelhardt A, Hodges JK, Niemitz C, Heistermann M. 2005. Female sexual behavior, but not sex skin swelling, reliably indicates the timing of the fertile phase in wild long-tailed macaques (Macaca fasicularis). Horm Behav. 47:195–204.
- Engh AE, Beehner JC, Bergman TJ, Whitten PL, Hoffmeier RR, Seyfarth RM, Cheney DL. 2006. Behavioural and hormonal responses to predation in female chacma baboons (Papio hamadryas ursinus). Proc R Soc Lond B. 273:707–712.
- Fernström AL, Sutian W, Royo F, Westlund K, Nilsson T, Carlsson HE, Paramastri P, Pamungkas J, Sajuthi D, Schapiro SJ, Hau J. Stress in cynomolgus monkeys (Macaca fascicularis) subjected to long-distance transport and simulated transport housing conditions. Stress. 2008 11:467-476.
- Fichtel C, Kraus C, Ganswindt A, Heistermann M. 2007. Influence of reproductive season and rank on fecal glucocorticoid levels in free-ranging male Verreaux's sifakas (Propithecus verreauxi). Horm Behav. 51:640–648.
- Fraser ON, Stahl D, Aureli F. 2008. Stress reduction through consolation in chimpanzees. Proc Natl Acad Sci USA. 105:8557–8562.
- Heistermann M, Möhle U, Vervaecke H, van Elsacker L, Hodges JK. 1996. Application of urinary and fecal steroid measurements for monitoring ovarian function and pregnancy in the bonobo (Pan paniscus) and evaluation of perineal swelling patterns in relation to endocrine events. Biol Reprod. 55:844–853.
- Heistermann M, Ademmer C, Kaumanns W. 2004. Ovarian cycle and effect of social changes on adrenal and ovarian function in Pygathrix nemaeus. Int J Primatol. 25:689–708.
- Heistermann M, Palme R, Ganswindt A. 2006. Comparison of different enzymeimmunoassays for assessment of adrenocortical activity in primates based on fecal analysis. Am J Primatol. 68:257–273.
- Higham JP, Ross C, Warren Y, Heistermann M, MacLarnon AM. 2007. Reduced reproductive function in wild olive baboons (Papio hamadryas anubis) related to natural consumption of the African black plum (Vitex doniana). Horm Behav. 52:384–390.
- Higham JP, MacLarnon AM, Ross C, Heistermann M, Semple S. 2008. Baboon sexual swellings: Information content of size and color. Horm Behav. 53:452–462.
- Hodges JK, Heistermann M. 2003. Field endocrinology: Monitoring hormonal changes in free-ranging primates. In: Setchell JCurtis DField and laboratory methods in primatology. Cambridge: Cambridge University Press. 282–294.
- Honess PE, Marin CM. 2006. Behavioural and physiological aspects of stress and aggression in nonhuman primates. Neurosci Biobehav Rev. 30:390–412.
- Lane J. 2006. Can non-invasive glucocorticoid measures be used as reliable indicators of stress in animals?. Anim Welf. 15:331–342.
- Maestripieri D, Schino G, Aureli F, Troisi A. 1992. A modest proposal: Displacement activities as an indicator of emotions in primates. Anim Behav. 44:967–979.
- Monclús R, Rödel HG, Palme R, Von Holst D, de Miguel J. 2006. Non-invasive measurement of the physiological stress response of wild rabbits to the odour of a predator. Chemoecology. 16:25–29.
- Ninan PT, Insel TM, Cohen RM, Cook JM, Skolnick P, Paul SM. 1982. Benzodiazepene receptor-mediated experimental ‘anxiety’ in primates. Science. 218:1332–1334.
- Ostner J, Heistermann M, Schülke O. Dominance, aggression and physiological stress in wild male Assamese macaques (Macaca assamensis). Horm Behav. 2008 54:613-619.
- Owen MA, Swaisgood RR, Czekala NM, Steinman K, Lindburg DG. 2004. Monitoring stress in captive giant pandas (Ailuropoda melanoleuca): Behavioral and hormonal responses to ambient noise. Zoo Biol. 23:147–164.
- Peel AJ, Vogelnest L, Finnigan M, Grossfeldt L, O'Brien JK. 2005. Non-invasive fecal hormone analysis and behavioral observations for monitoring stress responses in captive western lowland gorillas (Gorilla gorilla gorilla). Zoo Biol. 24:431–445.
- Pico-Alfonso MA, Mastorci F, Ceresini G, Ceda GP, Manghi M, Pino O, Troisi A, Sgoifo A. 2007. Acute psychosocial challenge and cardiac autonomic response in women: The role of estrogens, corticosteroids, and behavioral coping styles. Psychoneuroendocrinology. 32:451–463.
- Powell DM, Carlstead K, Tarou LR, Brown JL, Monfort SL. 2006. Effects of construction noise on behavior and cortisol levels in a pair of captive giant pandas (Ailuropoda melanoleuca). Zoo Biol. 25:391–408.
- Pride RE. 2005. High faecal glucocorticoid levels predict mortality in ring-tailed lemurs (Lemur catta). Biol Lett. 1:60–63.
- Sands J, Creel S. 2004. Social dominance, aggression and faecal glucocorticoid levels in a wild population of wolves, Canis lupus. Anim Behav. 67:387–396.
- Sapolsky RM. 2005. The influence of social hierarchy on primate health. Science. 308:648–652.
- Schino G. 1998. Reconciliation in domestic goats. Behaviour. 135:343–356.
- Schino G, Scucchi S, Maestripieri D, Turillazzi PG. 1988. Allogrooming as a tension-reduction mechanism: A behavioural approach. Am J Primatol. 16:43–50.
- Schino G, Perretta G, Taglioni A, Monaco V, Troisi A. 1996. Primate displacement activities as an ethopharmacological model of anxiety. Anxiety. 2:186–191.
- Shutt K, MacLarnon A, Heistermann M, Semple S. 2007. Grooming in Barbary macaques: Better to give than receive?. Biol Lett. 3:231–233.
- Sommer V, Adanu J, Faucher I, Fowler A. 2004. Nigerian chimpanzees (Pan troglodytes vellerosus) at Gashaka: Two years of habituation efforts. Folia Primatol. 75:295–316.
- Troisi A. 2002. Displacement activities as a behavioral measure of stress in nonhuman primates and human subjects. Stress. 5:47–54.
- Ulyan M, Burrows A, Buzzell C, Raghanti M, Marcinkiewicz J, Phillips K. 2006. The effects of predictable and unpredictable feeding schedules on the behavior and physiology of captive brown capuchins (Cebus apella). Appl Anim Behav Sci. 101:154–160.
- Ventura R, Majolo B, Schino G, Hardie S. 2005. Differential effects of ambient temperature and humidity on allogrooming, self-grooming, and scratching in wild Japanese macaques. Am J Phys Anthropol. 126:453–457.
- Wasser SK, Monfort SL, Southers J, Wildt DE. 1994. Excretion rates and metabolites of oestradiol and progesterone in baboon (Papio cynocephalus cynocephalus) faeces. J Reprod Fertil. 101:213–220.
- Watson SL, Ward JP, Davis KB, Stavisky RC. 1999. Scent-marking and cortisol response in the small-eared bushbaby (Otolemur garnettii). Physiol Behav. 66:695–699.
- Ziegler T, Hodges K, Winkler P, Heistermann M. 2000. Hormonal correlates of reproductive seasonality in wild female Hanuman langurs (Presbytis entellus). Am J Primatol. 51:119–134.