Abstract
In the present study, we evaluated cardiac baroreflex responses of rats submitted to acute restraint stress. The baroreflex was tested: immediately before, during a 30 min exposure to restraint stress, as well as 30 and 60 min after ending the stress session (recovery period). Restraint increased both mean arterial pressure (MAP) and heart rate (HR). The magnitude of tachycardiac responses evoked by intravenous infusion of sodium nitroprusside was higher during restraint stress, whereas that of bradycardiac responses evoked by intravenous infusion of phenylephrine was decreased. Restraint-evoked baroreflex changes were still observed at 30 min into the recovery period, although MAP and HR values had already returned to control values. The baroreflex was back to control values at 60 min of the recovery period. Intravenous administration of the selective β1-adrenoceptor antagonist atenolol blocked the restraint-evoked increase in the tachycardiac baroreflex response, but did not affect the effects on the bradycardiac response. In conclusion, the present results suggest that psychological stresses, such as those resulting from acute restraint, affect the baroreflex. Restraint facilitated the tachycardiac baroreflex response and reduced the bradycardiac response. Restraint-related effects on baroreflex persisted for at least 30 min after ending restraint, although MAP and HR had already returned to control levels. The cardiac baroreflex returned to control values 60 min after the end of restraint, indicating non-persistent effects of acute restraint on the baroreflex. Results also indicate that the influence of restraint stress on the baroreflex tachycardiac response is mainly dependent on cardiac sympathetic activity, whereas the action on the bradycardiac response is mediated by the cardiac parasympathetic component.
Introduction
The baroreflex is an important mechanism for moment-to-moment regulation of cardiovascular parameters (Sved and Gordon Citation1994; Michelini Citation2007). Baroreceptors sense systemic blood pressure through the extent of receptor stretch within the walls of the carotid arteries and aorta. Thus, increases in blood pressure activate baroreceptor afferents, increasing cardiac parasympathetic activity and decreasing sympathetic activity, which results in heart rate (HR), cardiac contractility, vascular resistance and venous return decreases (Michelini Citation1994). The blood pressure decrease reduces baroreceptor afferent discharge, causing the opposite effects (Michelini Citation1994, Citation2007; Sved and Gordon Citation1994).
It is well known that exposure to stressful stimuli evokes blood pressure and HR increases (Lawler et al. Citation1975; Kvetnansky et al. Citation1979; Hatton et al. Citation1997; Irvine et al. Citation1997; Barres et al. Citation2004; Carrive Citation2006; Tavares and Correa Citation2006). Hilton (Citation1963) was the first to address the question as to how these parallel increases in blood pressure and HR occur during stress. It was first suggested that baroreflex activity could be inhibited during stimulation of the hypothalamic defense area in cats (Coote et al. Citation1979). However, further investigation on baroreflex activity during emotional stress yielded inconsistent results. A variety of baroreflex changes which included facilitation, inhibition or a shift in the set point without changes in reflex gain were reported following exposure to different stressors or defense area activation (Evans Citation1978; Shammas et al. Citation1988; Schenberg et al. Citation1993; Hatton et al. Citation1997; Schadt and Hasser Citation1998). These contradictory reports may result from methodological limitations in baroreflex evaluation or analysis of limited portions of the HR response range. Also, post-stress baroreflex activity recovery was never evaluated.
Although pressor and tachycardiac responses are a common denouement of stress exposure, their patterns were shown to vary among exposure to different stressors, such as exposure to air-jet, handling, a swimming test or restraint (Sgoifo et al. Citation1999; McDougall et al. Citation2005). Restraint stress is well accepted in the literature as a model characterized by unconditioned and unavoidable stress-elicited neuroendocrine and cardiovascular responses, the latter being characterized by sustained blood pressure and HR increases, which last through the restraint period (Barron and Van Loon Citation1989; Irvine et al. Citation1997; McDougall et al. Citation2005; Tavares and Correa Citation2006). Although restraint-induced cardiovascular responses are well documented, there is no information about baroreflex changes associated with this model of psychological stress.
In the present study, we tested the hypothesis that acute restraint stress modulates the cardiac baroreflex response and that this effect is reversed after ending the stress session. To test this hypothesis, the baroreflex cardiac response was examined before, during restraint stress and 30 and 60 min after ending restraint, through nonlinear analysis, which generates sigmoid curves, and linear analysis of the baroreflex that allows separate evaluation of the baroreflex bradycardiac and tachycardiac components.
Materials and methods
Animals
Experimental procedures were carried out following protocols approved by the Ethical Review Committee of the School of Medicine of Ribeirão Preto, which complies with the Guiding Principles for Research Involving Animals and Human Beings of the American Physiological Society.
Male Wistar rats weighing approximately 250 g were used in the present experiments. Rats were housed in plastic cages (5 rats per cage) in a temperature-controlled room (25°C) in the Animal Care Unit of the Department of Pharmacology, School of Medicine of Ribeirão Preto. Rats were kept under a 12:12 h light–dark cycle (lights on between 06:00 am and 6:00 pm) and had free access to water and standard laboratory food, except during the experimental period.
Surgical preparation
One day before the trial, rats were anesthetized with tribromoethanol (250 mg/kg, i.p.) and a catheter was inserted into the abdominal aorta through the femoral artery, for blood pressure and HR recording. A second catheter was implanted into the femoral vein for infusion of phenylephrine or sodium nitroprusside (SNP) to evoke baroreflex changes. Both catheters were tunneled under the skin and exteriorized on the rat's dorsum. After surgery, the rats received a poly-antibiotic (i.m. 0.27 mg/kg, Pentabiotico®, Fort Dodge, Brazil), with streptomycins and penicillins, to prevent infection, and a nonsteroidal anti-inflammatory (i.m. 0.025 mg/kg, Flunixine Meglumine, Banamine®, Schering Plough, Brazil) for post-operative analgesia. After surgery, rats were kept in individual cages that were later used for transport rats to the experimental room.
Measurement of cardiovascular responses
On the day of the experiment, the arterial cannula was connected to a pressure transducer and pulsatile arterial pressure (PAP) was recorded using an HP-7754A pre-amplifier (Hewlett Packard, Palo Alto, CA, USA) and an acquisition board (MP100A, Biopac Systems Inc, Goleta, CA, USA) connected to a personal computer. PAP was recorded with a sampling rate of 100 Hz. Mean arterial pressure (MAP) and HR values were derived from PAP and were processed online. Experiments were performed during the morning period in order to minimize possible circadian rhythm interferences.
Baroreflex assessment
The ‘ramp’ method was chosen to assess the baroreflex because MAP changes are gradual, thus avoiding abrupt responses (Farah et al. Citation1999). Therefore, the baroreflex was tested by giving intravenous phenylephrine (Sigma, St. Louis, MO, USA; 50 μg/ml/kg at 0.34 ml/min) or SNP (Sigma; 50 μg/ml/kg at 0.8 ml/min) infusion using an infusion pump (K.D. Scientific, Holliston, MA, USA; Resstel et al. Citation2004). Infusions of vasoactive drugs were randomized and the interval between infusions was approximately 5 min. Infusions lasted for 30–40 s, resulting in the injection of a total dose of 8–10 μg/kg of phenylephrine and of a total dose of 20–25 μg/kg of SNP.
Experimental protocols
Rats were brought to the experimental room in their own home cages. Rats were allowed one hour to adapt to the conditions of the experimental room, such as sound and illumination before starting arterial pressure and HR recording. The experimental room was temperature controlled (25°C) and was acoustically isolated from the other rooms. Constant background noise was generated by an air exhauster to minimize sound interference within the experimental room.
The experiments began with a 30 min recording of MAP and HR of freely moving rats. After that, rats received infusions of phenylephrine or SNP to determine control baroreflex parameters (control group). Fifteen minutes later, rats were submitted to 30 min of restraint, which was initiated by placing rats into small plastic cylindrical restraint tubes (diameter = 6.5 cm and length = 15 cm), ventilated by holes of 0.5 cm of diameter which comprised approximately 20% of the tube surface. Twenty minutes after restraint onsets, when MAP and HR were stable, infusions of vasoactive drugs were repeated to determine baroreflex activity (restraint group). The rats were then returned to their home cages and the baroreflex was evaluated 30 (group: 30 min after) and 60 min (group: 60 min after) after the ending of restraint. Each rat was submitted to one session of restraint to prevent habituation.
To study the role of cardiac sympathetic activity on the cardiac baroreflex response, the rats were intravenously treated with atenolol (1 mg/kg, Head and McCarty Citation1987), a selective β1-adrenoceptor antagonist. Infusions of phenylephrine or SNP were made before (control group) and 10 min after (control + atenolol group) administration of atenolol to determine control baroreflex parameters. In the sequence, the protocol to study baroreflex activity during (restraint + atenolol group) and after (30 min after + atenolol and 60 min after + atenolol groups) acute restraint stress in atenolol-treated rats was conducted as described above.
Method of baroreflex evaluation
Baroreflex curves were constructed matching MAP changes with HR responses. Paired MAP and HR values were plotted to generate sigmoid curves (Head and McCarty Citation1987) for each rat and baroreflex curves were generated using commercial software (Prism, GraphPad, San Diego, CA, USA).
Linear regression analysis was further performed for the separate analysis of bradycardiac and tachycardiac baroreflex responses. For that, HR values matching 10, 20, 30 and 40 mmHg of MAP changes were calculated. Resulting points were plotted to create linear regression curves for each rat (Resstel et al. Citation2004; Scopinho et al. Citation2007) and their slopes were compared to verify changes in baroreflex gain. The delay in reflex HR responses was about 1.2 s because of the time for baroreflex synapse processing, which is 700 ms according to Su et al. (Citation1992), and the integration factor of the recording system, which was about 500 ms.
Statistical analysis
The baroreflex was analyzed using sigmoid curves which were characterized by four parameters: (i) P1 (bpm) lower HR plateau and P2 (bpm) upper HR plateau; (ii) HR range (bpm), i.e. difference between upper and lower plateau levels; (iii) median blood pressure (BP50, mmHg), which is the MAP at 50% of the HR range; (iv) average gain (G, bpm/mmHg), which is the average slope of the curves; and maximum gain (Gmax, bpm/mmHg), which is the maximum gain obtained from the calculation of the baroreflex gain across the full range of MAP variations (Head and McCarty Citation1987; Hatton et al. Citation1997). Significant differences between sigmoid curves and linear regression parameters and MAP and HR responses to restraint stress were analyzed using one-way ANOVA followed by Bonferroni's post-test, assuming P < 0.05 as significant. Student's t-test was used to compare basal values of MAP and HR before and after administration of atenolol.
Results
Baroreflex cardiac response during acute restraint stress
Acute restraint stress (n = 6) caused significant increases in MAP (96 ± 5 vs. 115 ± 4 mmHg, F(3,20) = 6, P < 0.01) and HR (341 ± 11 vs. 408 ± 8 bpm, F(3,20) = 10, P < 0.01). A representative recording illustrating the cardiovascular responses from one rat submitted to acute restraint stress is presented in .
Figure 1 Recording from one rat illustrating the effect of acute restraint stress on pulsatile arterial pressure (PAP), MAP and HR. Note the sustained arterial pressure and HR increase during the entire restraint period.
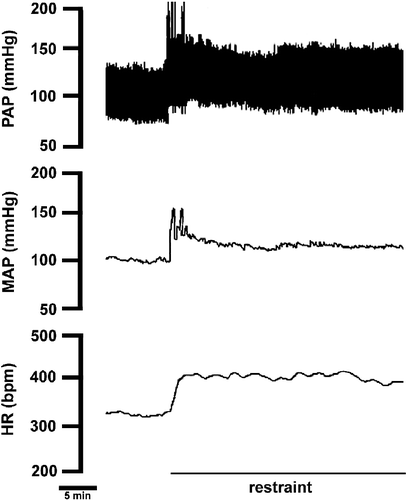
Baroreflex curves were constructed matching MAP changes with HR responses. Sigmoid nonlinear regression analyses of curves constructed using MAP and HR changes demonstrated that acute restraint affected all the baroreflex parameters. Baroreflex changes were characterized by increased upper plateau (P2, bpm), HR range (bpm), average gain (G, bpm/mmHg) and maximum gain (Gmax, bpm/mmHg); decreased lower plateau (P1, bpm) and shift toward the left in the mean blood pressure value (BP50, mmHg; and ). Baroreflex linear regression curves were generated for separate analysis of bradycardiac and tachycardiac baroreflex responses (). Linear regression analysis indicated that restraint stress increases the curve slope of tachycardiac responses ( − 1.8 ± 0.2 vs. − 3.5 ± 0.4 bpm/mmHg, F(3,20) = 11, P < 0.005, n = 6) without affecting the x-intercept value ( − 4 ± 3 vs. − 1 ± 4 mmHg, F(3,20) = 1, P>0.05). The acute restraint stress caused a rightward displacement of the regression lines of bradycardiac responses. The x-intercept was significantly increased (0.1 ± 2 vs. 10 ± 1 mmHg, F(3,20) = 9, P < 0.05, n = 6) and no changes were observed in the curve slope ( − 1.7 ± 0.3 vs. − 1.2 ± 0.4 bpm/mmHg, F(3,20) = 1, P>0.05).
Figure 2 (A) Sigmoid baroreflex curves (n = 6) correlating changes in mean arterial pressure (ΔMAP) and heart rate (ΔHR) of responses before (control, ○; r2 = 0.80) or during (restraint, •; r2 = 0.86) acute restraint stress. Symbols indicate the curve BP50. (B) Baroreflex linear regression curves (n = 6) correlating ΔMAP and ΔHR of control (dashed lines) or restraint (solid lines) groups. Correlation r2 values for bradycardiac regression curves were 0.65 for control and 0.78 for restraint. Correlation r2 values for tachycardiac regression curves were 0.50 for control and 0.59 for restraint. (C) Sigmoid baroreflex curves (n = 6) correlating absolute values of MAP and HR of control (○; r2 = 0.65) and restraint (•; r2 = 0.76) groups. Symbols indicate the curve BP50. (D) Linear regression curves (n = 6) correlating absolute values of MAP and HR for infusion of SNP (▪) and phenylephrine (Phenyl, ○) of control (dashed lines) and restraint (solid lines) groups. Correlation r2 values for bradycardiac regression curves were 0.60 for control and 0.68 for restraint. Correlation r2 values for tachycardiac regression curves were 0.50 for control and 0.55 for restraint. One-way ANOVA followed by Bonferroni's post-test was used to compare differences in cardiac baroreflex responses (See Results): Restraint stress increased the tachycardiac baroreflex response and evoked a rightward displacement in the bradycardiac response, P < 0.005.
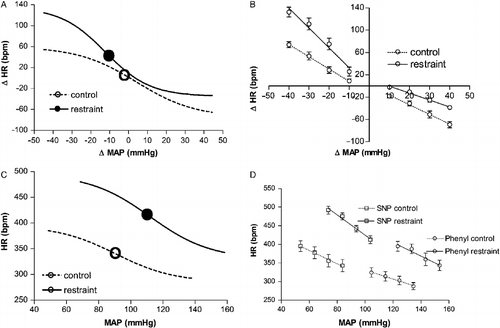
Table I. Parameters derived from sigmoidal baroreflex curves constructed using MAP and HR changes.
Nonlinear regression analysis of curves generated using absolute values of MAP and HR demonstrated that restraint stress increased upper plateau (P2, bpm), HR range (bpm), average gain (G, bpm/mmHg) and maximum gain (Gmax, bpm/mmHg); decreased lower plateau (P1, bpm) and shift toward the right in the mean blood pressure value (BP50, mmHg; and ). Linear analysis indicated that restraint stress increased the curve slope of tachycardiac responses ( − 1.7 ± 0.3 vs. − 2.9 ± 0.2 bpm/mmHg, F(3,20) = 7, P < 0.005) and decreased the x-intercept value (276 ± 4 vs. 248 ± 6 mmHg, F(3,20) = 28, P < 0.001; ). The x-intercept value of the bradycardiac response was significantly increased (383 ± 6 vs. 480 ± 7 mmHg, F(3,20) = 78, P < 0.001) and no changes were observed in the curve slope ( − 1.2 ± 0.3 vs. − 1.3 ± 0.2 bpm/mmHg, F(3,20) = 1, P>0.05; ).
Table II. Parameters derived from sigmoidal baroreflex curves constructed using absolute values of MAP and HR.
Baroreflex cardiac activity at 30 and 60 min during recovery from acute restraint stress
Thirty minutes after the end of acute restraint the MAP (96 ± 5 vs. 91 ± 4 mmHg, P>0.05) and HR (349 ± 11 vs. 361 ± 9 bpm, P>0.05, n = 6) had returned to basal values. However, nonlinear regression analysis of curves constructed using MAP and HR changes indicated that at 30 min of the recovery period the baroreflex parameters had values similar to those observed during restraint ( and ). Linear regression analysis also indicated that the slope of the tachycardiac baroreflex response was still increased ( − 1.8 ± 0.2 vs. − 3.3 ± 0.3 bpm/mmHg, F(3,20) = 11, P < 0.05). No effects were observed in the x-intercept ( − 4 ± 3 vs. − 1 ± 2 mmHg, F(3,20) = 1, P>0.05), when compared with control values (). The bradycardiac response still displayed rightward displacement. The x-intercept was significantly increased (0.1 ± 2 vs. 9 ± 2 mmHg, F(3,20) = 9, P < 0.05) but did not affect the slope of the bradycardiac response ( − 1.7 ± 0.3 vs. − 1.4 ± 0.2 bpm/mmHg, F(3,20) = 1, P>0.05), when compared with control values ().
Figure 3 (A) Sigmoid baroreflex curves (n = 6) correlating changes in mean arterial pressure (ΔMAP) and heart rate (ΔHR) of responses before (control, ▪; r2 = 0.80) or 30 (30 min after, •; r2 = 0.76) and 60 min after (60 min after, ○; r2 = 0.86) ending acute restraint stress. Symbols indicate the curve BP50. (B) Linear regression curves (n = 6) correlating ΔMAP and ΔHR of control (dotted lines) or 30 min after (solid lines) and 60 min after (dashed lines) groups. Correlation r2 values for bradycardiac regression curves were 0.65 for control and 0.78 or 0.64, respectively, 30 and 60 min after ending restraint. Correlation r2 values for tachycardiac regression curves were, respectively, 0.50 for control and 0.59 or 0.78 at 30 or 60 min after ending restraint. (C) Sigmoid baroreflex curves (n = 6) correlating absolute values of MAP and HR of control (▪; r2 = 0.70), 30 min after (•; r2 = 0.65) and 60 after (○; r2 = 0.58) groups. Symbols indicate the curve BP50. (D) Linear regression curves (n = 6) correlating absolute values of MAP and HR for infusion of SNP (▪) and phenylephrine (Phenyl, ○) of control (dotted lines), 30 min after (solid lines) and 60 min after (dashed lines) groups. Correlation r2 values for bradycardiac regression curves were 0.69 for control and 0.68 or 0.54, respectively, 30 and 60 min after ending restraint. Correlation r2 values for tachycardiac regression curves were, respectively, 0.53 for control and 0.67 or 0.68 at 30 or 60 min after ending restraint. One-way ANOVA followed by Bonferroni's post-test was used to compare differences in cardiac baroreflex responses (See Results): restraint-evoked baroreflex changes were still observed at 30 min of the recovery period (P < 0.05), and had returned to control values at 60 min of the recovery period (P>0.05).
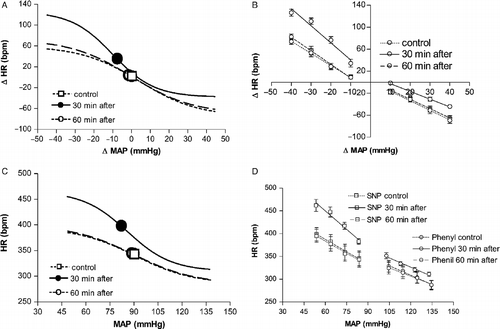
Sixty minutes after ending acute restraint the nonlinear curve parameters returned to control values ( and ). Linear regression also indicated that the baroreflex response had returned to control values (). The x-intercept ( − 4 ± 3 vs. − 3 ± 3 mmHg, P>0.05) and the slope ( − 1.8 ± 0.2 vs. − 1.7 ± 0.3 bpm/mmHg, P>0.05) of the reflex tachycardiac response was similar to control values. The x-intercept (0.1 ± 2 vs. 1 ± 2 mmHg, P>0.05) and the slope ( − 1.7 ± 0.3 vs. − 1.7 ± 0.2 bpm/mmHg, P>0.05) values of the bradycardiac response also returned to control values at 60 min of the recovery period.
Nonlinear regression analysis of curves generated using absolute values of MAP and HR also indicated that at 30 min of the recovery period the baroreflex parameters had values similar to those observed during restraint ( and ). Linear analysis indicated that restraint stress increases the curve slope of tachycardiac responses ( − 1.7 ± 0.3 vs. − 2.9 ± 0.2 bpm/mmHg, F(3,20) = 7, P < 0.05) and decreases the x-intercept value (276 ± 4 vs. 248 ± 4 mmHg, F(3,20) = 28, P < 0.001; ). The x-intercept of the bradycardiac response was significantly increased (383 ± 6 vs. 405 ± 8 mmHg, F(3,20) = 78, P < 0.05) and no changes were observed in the curve slope ( − 1.2 ± 0.3 vs. − 1.3 ± 0.4 bpm/mmHg, P>0.05; ).
Nonlinear regression analysis of curves generated using absolute values of MAP and HR indicated that at 60 min of the recovery period the baroreflex parameters returned to control values ( and ). The x-intercept (276 ± 4 vs. 279 ± 4 mmHg, P>0.05) and the slope ( − 1.7 ± 0.3 vs. − 1.8 ± 0.3 bpm/mmHg, P>0.05) of the reflex tachycardiac response was similar to control values (). The x-intercept (383 ± 6 vs. 380 ± 4 mmHg, P>0.05) and the slope ( − 1.2 ± 0.3 vs. − 1.4 ± 0.5 bpm/mmHg, P>0.05) values of the bradycardiac response also returned to control values at 60 min of the recovery period ().
Representative recordings showing the effect of acute restraint stress on reflex bradycardia and tachycardia are presented, respectively, in . Moreover, a scatter plot showing MAP–HR data of one representative rat, together with the fitted sigmoid curve before and during restraint stress is presented in .
Figure 4 Typical recording from one representative rat illustrating reflex bradycardia in response to a blood pressure increase caused by intravenous phenylephrine (phenyl) infusion before, during (Restraint) or 30 min after ending (30 min after restraint) the acute restraint stress. Vertical lines (a) indicate an increase of 10 mmHg in MAP and the corresponding reflex bradycardia showing rightward displacement of the response during and 30 min after acute restraint stress. PAP, pulsatile arterial pressure and HR, heart rate.
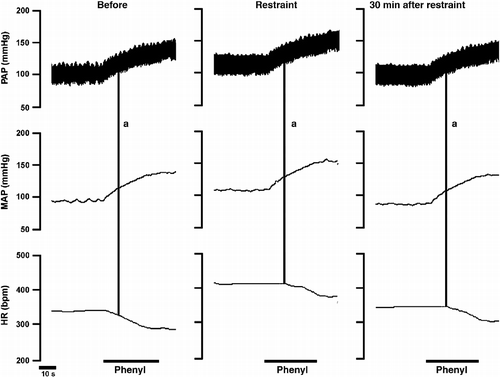
Figure 5 Typical recording from one representative rat illustrating reflex tachycardia in response to blood pressure decreases caused by intravenous SNP infusion before, during (Restraint) or 30 min after ending (30 min after restraint) the acute restraint stress. PAP, pulsatile arterial pressure; MAP, mean arterial pressure; HR, heart rate.
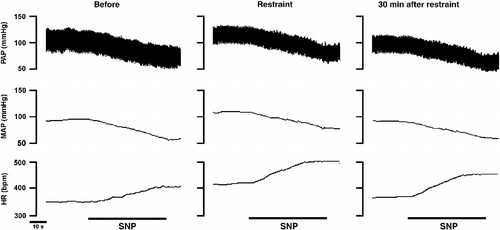
Figure 6 Scatter plot of MAP–HR data from one representative rat before (control) or during acute restraint stress (restraint) measured during phenylephrine (Phenyl, ○) and SNP (▪) infusions, with the fitted sigmoid curves (control: r2 = 0.97; restraint: r2 = 0.95). Curves were constructed by plotting the HR value observed at each subsequent 5 mmHg of increase or decrease in MAP.
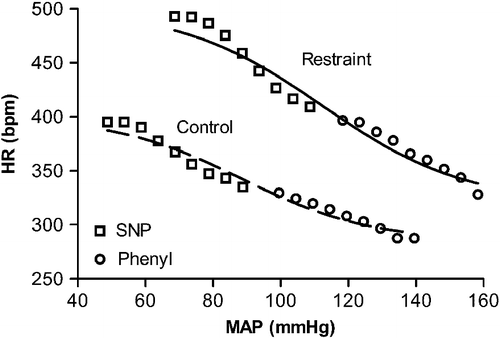
Baroreflex cardiac activity in atenolol-treated rats before, during or 30 and 60 min after acute restraint stress
Intravenous treatment with the selective β1-adrenoceptor antagonist atenolol (n = 5) did not affect the baseline MAP (95 ± 4 vs. 94 ± 3 mmHg; t = 0.2, P>0.05) or HR (357 ± 9 vs. 353 ± 10 bpm; t = 0.6, P>0.05). However, atenolol significantly reduced the restraint-evoked increase in heart rate (ΔHR: 61 ± 4 vs. 14 ± 2 bpm; t = 8, P < 0.001), without affecting the increase in MAP (ΔMAP: 16 ± 2 vs. 14 ± 2 mmHg; t = 0.4, P>0.05).
Sigmoid nonlinear regression curve analyses demonstrated that treatment with atenolol significantly decreased the upper plateau (P2, bpm), HR range (bpm), average gain (G, bpm/mmHg) and maximum gain (Gmax, bpm/mmHg); and the shift toward the right in the mean blood pressure value (BP50, mmHg), when compared with values obtained before treatment ( and ). Linear regression curves indicated that atenolol significantly decreased the slope ( − 2.3 ± 0.3 vs. − 0.4 ± 0.1 bpm/mmHg, F(4,20) = 16, P < 0.001) and did not affect the x-intercept ( − 7 ± 1 vs. 2 ± 3 mmHg, F(4,20) = 2, P < 0.05) of the tachycardiac response (). The slope ( − 1.5 ± 0.2 vs. − 1.4 ± 0.2 bpm/mmHg, F(4,20) = 1, P>0.05) and the x-intercept (1 ± 2 vs. 2 ± 2 mmHg, P>0.05) of the bradycardiac baroreflex responses were similar before and after treatment with atenolol ().
Figure 7 (A) Sigmoid baroreflex curves (n = 5) correlating changes in MAP (ΔMAP) and HR (ΔHR) of responses before (control, ▪; r2 = 0.78) or after intravenous treatment with the selective β1-adrenoceptor antagonist atenolol (control + atenolol, ○; r2 = 0.81) and during restraint stress in atenolol-treated rats (restraint + atenolol, •; r2 = 0.80). Symbols indicate the curve BP50. (B) Linear regression curves (n = 5) correlating ΔMAP and ΔHR of control (dotted lines), control + atenolol (dashed lines) and restraint + atenolol (solid lines) groups. Correlation r2 values for bradycardiac regression curves were 0.69 for control; 0.75 for control + atenolol and 0.74 for restraint + atenolol. Correlation r2 values for tachycardiac regression curves were, 0.50 for control; 0.59 for control + atenolol and 0.78 for restraint + atenolol. One-way ANOVA followed by Bonferroni's post-test was used to compare differences on cardiac baroreflex response in atenolol-treated rats (See Results): Restraint stress evoked a rightward displacement of the bradycardiac response (P < 0.005) with no changes in the tachycardiac baroreflex response in atenolol-treated rats.
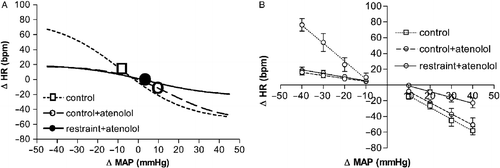
Table III. Parameters derived from sigmoidal baroreflex curves constructed using MAP and HR changes in atenolol-treated animals.
Baroreflex cardiac response during acute restraint stress
Comparison of nonlinear regression curves before and during restraint stress in atenolol-treated rats demonstrated that acute restraint affected the baroreflex activity. Baroreflex changes were characterized by decreased lower plateau (P1, bpm), HR range (bpm) and average gain (G, bpm/mmHg; and ), when compared with the control + atenolol group. Linear regression analysis indicated that restraint stress did not affect the slope ( − 0.4 ± 0.2 vs. − 0.5 ± 0.2 bpm/mmHg, P>0.05) or the x-intercept (2 ± 2 vs. 3 ± 3 mmHg, F(4,20) = 2, P>0.05) of the tachycardiac bradycardiac response (). However, acute restraint caused a rightward displacement of the regression lines of the bradycardiac response (). The x-intercept was significantly increased (2 ± 2 vs. 11 ± 1 mmHg, F(4,20) = 8, P < 0.05) and no changes were observed in the curve slope ( − 1.4 ± 0.3 vs. − 0.8 ± 0.3 bpm/mmHg, F(4,20) = 1, P>0.05).
Baroreflex cardiac activity at 30 and 60 min during recovery from acute restraint stress
Thirty minutes after the end of acute restraint the MAP (94 ± 6 vs. 90 ± 4 mmHg, P>0.05) and HR (344 ± 12 vs. 359 ± 8 bpm, P>0.05) had returned to basal values in the atenolol-treated rats. However, nonlinear regression analysis indicated that at 30 min of the recovery period the baroreflex parameters had values similar to those observed during restraint ( and ). Linear regression analysis also indicated that the bradycardiac response still displayed rightward displacement (). The x-intercept of bradycardiac responses was significantly increased (2 ± 2 vs. 11 ± 2 mmHg, F(4,20) = 8, P < 0.05) and no changes were observed in the slope ( − 1.4 ± 0.3 vs. − 1.1 ± 0.2 bpm/mmHg, F(4,20) = 1, P>0.05). The slope value ( − 0.4 ± 0.2 vs. − 0.6 ± 0.1 bpm/mmHg, P>0.05) and the x-intercept value (2 ± 2 vs. − 5 ± 5 mmHg, F(4,20) = 2, P>0.05) of the tachycardiac responses were similar to the control + atenolol group.
Figure 8 (A) Sigmoid baroreflex curves (n = 5) correlating changes in MAP (ΔMAP) and HR (ΔHR) in rats treated intravenously with the selective β1-adrenoceptor antagonist atenolol before (control + atenolol, ▪; r2 = 0.71) or 30 (30 min after + atenolol, •; r2 = 0.79) and 60 min after (60 min after + atenolol, ○; r2 = 0.82) ending the acute restraint stress. Symbols indicate the curve BP50. (B) Linear regression curves (n = 5) correlating ΔMAP and ΔHR in atenolol-treated rats of control + atenolol (dotted lines), 30 min after + atenolol (solid lines) and 60 min after + atenolol (dashed lines) groups. Correlation r2 values for bradycardiac regression curves were 0.69 for control + atenolol and 0.88 or 0.75, respectively, 30 and 60 min after ending restraint. Correlation r2 values for tachycardiac regression curves were, 0.72 for control + atenolol and 0.79 or 0.86 at 30 or 60 min after ending restraint. One-way ANOVA followed by Bonferroni's post-test was used to compare differences in cardiac baroreflex responses (See Results): restraint-evoked baroreflex changes in atenolol-treated rats were still observed at 30 min of the recovery period (P < 0.05), and had returned to control values at 60 min of the recovery period (P>0.05).
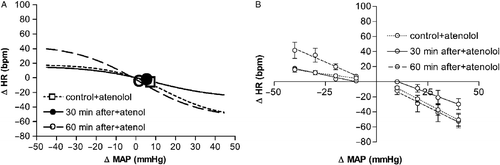
Sixty minutes after ending acute restraint nonlinear analysis of the baroreflex indicated increase of the upper plateau (P2, bpm), HR range (bpm) and average gain (G, bpm/mmHg), when compared with control + atenolol group ( and ). Linear regression analysis indicated that the slope of the tachycardiac response was increased ( − 0.4 ± 0.2 vs. − 1.2 ± 0.2 bpm/mmHg, F(4,20) = 16, P < 0.05) and no changes were observed in the x-intercept (2 ± 2 vs. − 3 ± 2 mmHg, F(4,20) = 2, P>0.05), when compared with the control + atenolol group (). The x-intercept (2 ± 2 vs. 0 ± 3 mmHg, P>0.05) and the slope ( − 1.4 ± 0.3 vs. − 1.3 ± 0.3 bpm/mmHg, P>0.05) of the bradycardiac response returned to control values at 60 min of the recovery period ().
Discussion
The present work demonstrates that stress evoked by acute restraint increases the baroreflex tachycardiac response and decreases the baroreflex bradycardiac response. These effects on the baroreflex could be observed 30 min after the end of restraint and were normalized 60 min after the end of stress. Moreover, intravenous treatment with atenolol blocked the restraint-evoked increase in the baroreflex tachycardiac response, but did not affect restraint effects on the baroreflex bradycardiac response.
Cardiac baroreflex activity was determined during exposure to acute restraint stress and during the post-stress recovery period. The baroreflex was first evaluated by nonlinear regression analysis of sigmoid curves generated by plotting HR changes in response to blood pressure increase or decrease evoked by, respectively, i.v. infusion of phenylephrine or SNP. Analysis of sigmoid curves generated when the cardiac reflex was evaluated during restraint indicated a decrease in the magnitude of the lower plateau (P1) and an increase of the upper plateau (P2). We also observed a significant increase in curve gain and HR range index. The HR range increase was due mainly to an increase in the upper HR plateau. The increased upper plateau and decreased lower plateau resulted in a BP50 shift to the left on the x-axis in the curves constructed using MAP and HR change. In the case of the curves constructed using absolute values, the effect on the BP50 was mainly because of the restraint-evoked increase in MAP. Therefore, our results suggest a facilitatory influence of acute restraint stress on the tachycardiac response of the baroreflex and an inhibitory action on the bradycardiac response.
To further study the restraint stress effect on the baroreflex cardiac response, we used linear regression for separate analysis of the bradycardiac and tachycardiac baroreflex limbs (Resstel et al. Citation2004; Crestani et al. Citation2006; Pelosi et al. Citation2007; Scopinho et al. Citation2007). Baroreflex linear regression indicated that the tachycardiac response gain increased during restraint stress, whereas that of the bradycardiac response was not affected. This observation indicates that changes in baroreflex gain observed after nonlinear analysis were due to a change in gain of baroreflex tachycardiac responses. However, despite the absence of an effect of acute restraint on the gain of the bradycardiac response, we observed a rightward displacement of its regression line, suggesting a reset of the bradycardiac response onset toward upper values during restraint. This suggests that the baroreflex is not inhibited during stress and instead bradycardiac responses to baroreflex activation are reset toward higher blood pressure values.
A similar reset in baroreflex activity toward higher blood pressure values was reported when animals were exposed to air-jet or shock stresses (Hatton et al. Citation1997; Shammas et al. Citation1988; Schadt and Hasser Citation1998). Although previous results suggest that different stressors may cause differential cardiovascular responses (Sgoifo et al. Citation1999; McDougall et al. Citation2005), baroreflex modulation may be a common effect during different stress situations. Moreover, the reset of the reflex bradycardiac response toward higher blood pressure values allows parallel increases in sympathetic nerve activity, MAP and HR (Nosaka Citation1996).
The present report is the first description of the baroreflex activity during recovery from a stress session. We observed that 30 min after ending restraint, although MAP and HR had returned to control values, the baroreflex activity was still similar to that observed during acute restraint stress and only returned to control values at 60 min of the recovery period, indicating non-persistent effects of acute restraint on the baroreflex.
Physical exercise evokes changes in arterial pressure, HR, sympathetic nerve activity and baroreflex activity which are similar to those observed during stress (Rowell and O'Leary Citation1990; DiCarlo et al. Citation1996). After arterial baroreceptor denervation, it was observed that MAP fell sharply after ending exercise before returning to resting values (Krasney et al. Citation1974; Ludbrook and Graham Citation1985), indicating that the baroreflex could be important to counteract excessive activation of depressor mechanisms during the recovery period. Previous results suggested that endothelin-A receptors and a nitrergic mechanism in the brain are activated during the recovery period from stress and mediate the decrease in arterial pressure after ending stress (Yip and Krukoff Citation2002). Thus, the persistence of the restraint effects on baroreflex activity, observed in the present study after ending exposure to the stressor, could be important to counteract excessive activation of depressor mechanisms during the recovery period, thus allowing adequate return of blood pressure to normal values without abrupt falls during the recovery period.
To study the role of cardiac sympathetic activity on the cardiac baroreflex response, the rats were intravenously treated with the selective β1-adrenoceptor antagonist atenolol. The dose of atenolol presently used (1 mg/kg, i.v.) did not affect basal MAP and HR values, but already interfered in the baroreflex response (Head and McCarty Citation1987). Intravenous treatment with atenolol significantly decreased the upper HR plateau of the baroreflex sigmoid curves without affecting the lower plateau, when compared with values before treatment. Moreover, linear regression analysis indicated a decrease in the tachycardiac response with no changes in the bradycardiac responses. Thus, although some studies suggest that baroreflex responses are due to reciprocal variations in sympathetic and parasympathetic activity (Wang and Borison Citation1947; Abdel-Rahman Citation1999), the present results corroborate other studies which suggest that the baroreflex bradycardiac response is mainly mediated by cardiac parasympathetic stimulation, whereas the tachycardiac response is predominantly affected by cardiac sympathetic activity (Glick and Braunwald Citation1965; Sullebarger et al. Citation1990). It has been documented that the baroreflex response using a ‘steady state’ method of baroreflex assessment (blood pressure changes caused in steps) was affected by both sympathetic and vagal blockade, whereas the responses through ‘ramp’ (brief infusion of vasoactive drugs) or in bolus (injection of single doses) methods were unaffected by sympathetic blockade, but were inhibited by treatment with a vagal antagonist (Weinstock and Rosin Citation1984; Sullebarger et al. Citation1990). Thus, differences in the involvement of autonomic components in baroreflex responses among different studies could result from methodological differences in baroreflex stimulation or its analysis.
Nonlinear and linear analyses of the baroreflex indicated that treatment with atenolol blocked restraint-evoked changes in the tachycardiac response of the baroreflex, without affecting restraint effects on the bradycardiac response. Differences in the response of atenolol-treated rats at 60 min of the recovery period when compared with the control + atenolol group are a result of the partial reversibility of atenolol β1-adrenoceptor blockade. Therefore, data suggest that the facilitatory influence of acute restraint stress on the tachycardiac response of the baroreflex is mainly dependent on cardiac sympathetic activity, whereas the inhibitory action on the bradycardiac response is mediated by the cardiac parasympathetic component. This idea is reinforced by data indicating that baroreflex control of the renal sympathetic nerve activity is sensitized and operates over a larger range during air-jet stress (Kanbar et al. Citation2007).
Several central nervous system structures may mediate stress-related modulation of baroreflex cardiac activity. Baroreflex modulation has been observed after stimulation or inhibition of several limbic forebrain structures involved with autonomic and behavioral responses to threat situations, including the medial prefrontal cortex (Verberne et al. Citation1987; Resstel and Correa Citation2006; Resstel et al. Citation2006), anterior (Simon et al. Citation1985) or posterior hypothalamus (Bauer et al. Citation1988), hypothalamic paraventricular nucleus (Jin and Rockhold Citation1989), periaqueductal gray matter (Pelosi et al. Citation2007), bed nucleus of the stria terminalis (Crestani et al. Citation2006; Crestani et al. Citation2008a, Citation2009), septal lateral area (Scopinho et al. Citation2007), amygdala (Schlor et al. Citation1984) and diagonal band of Broca (Crestani et al. Citation2008b). The time course of c-fos mRNA expression in the above structures of animals submitted to acute restraint stress indicated that 30 min after ending restraint expression was still high and returned to basal values only at 90 min of the recovery period (Cullinan et al. Citation1995). This observation agrees with the present report that baroreflex changes are still observable 30 min after ending the restraint session. In addition, restraint-related baroreflex modulation may also be mediated, at least in part, by stress hormones such as glucocorticoids or angiontensin II (DiBona et al. Citation1996; Scheuer and Mifflin Citation2001; Scheuer and Bechtold Citation2002). Intravenous administration of mifepristone, a glucocorticoid type II receptor antagonist, or of the AT1 receptor antagonist losartan shifted the baroreflex and renal sympathetic nerve activity curves to the left (DiBona et al. Citation1996; Scheuer and Mifflin Citation2001), suggesting that physiological levels of glucocorticoids and angiotensin II may modulate the baroreflex.
To summarize, the present results suggest that exposure to restraint stress affects the cardiac baroreflex response, facilitating the tachycardiac response and shifting the set point for the bradycardiac response toward higher BP values. The facilitatory influence of acute restraint stress on the tachycardiac baroreflex response is mainly dependent on cardiac sympathetic activity, whereas the action on the bradycardiac response is mediated by the cardiac parasympathetic component. Results also indicate that these effects persist for at least 30 min after ending restraint and returned to normal values at 60 min of the recovery period, indicating non-permanent baroreflex changes after acute restraint stress. Considering that cardiovascular and baroreflex changes are important allostasis mediators (McEwen and Wingfield Citation2003), but might precipitate cardiovascular dysfunction (Brotman et al. Citation2007), efficient reversibility of stress-evoked autonomic changes is important to reduce chances of inducing pathologies associated with long-term cardiovascular changes.
Acknowledgements
The authors wish to thank I.A.C. Fortunato and I.I.B. Aguiar and S.S. Guilhaume for technical help. Crestani has a FAPESP PhD fellowship (06/57670-4); Tavares has a CNPq post-doctoral fellowship (151388/2007-5) and Alves has a CNPq PhD fellowship (870307/1997-5). The present research was also supported by grants from the CNPq (306381/2003-6 and 505394/2003-0), FAPESP and FAEPA.
Declaration of interest: The authors report no conflicts of interest. The authors alone are responsible for the content and writing of the paper.
References
- Abdel-Rahman RA. 1999. Gender difference in baroreflex-mediated bradycardia in young rats: Role of cardiac sympathetic and parasympathetic components. Can J Physiol Pharmacol. 77:358–366.
- Barres C, Cheng Y, Julien C. 2004. Steady-state and dynamic responses of renal sympathetic nerve activity to air-jet stress in sinoaortic denervated rats. Hypertension. 43 3: 629–635.
- Barron BA, Van Loon GR. 1989. Role of sympathoadrenomedullary system in cardiovascular response to stress in rats. J Auton Nerv Syst. 28 2: 179–187 (doi: doi:10.1016/0165-1838(89)90090-8).
- Bauer RM, Vela MB, Simon T, Waldrop TG. 1988. A GABAergic mechanism in the posterior hypothalamus modulates baroreflex bradycardia. Brain Res Bull. 20 5: 633–641.
- Brotman DJ, Golden SH, Wittstein IS. 2007. The cardiovascular toll of stress. Lancet. 370 9592: 1089–100.
- Carrive P. 2006. Dual activation of cardiac sympathetic and parasympathetic components during conditioned fear to context in the rat. Clin Exp Pharmacol Physiol. 33 12: 1251–1254.
- Coote JH, Hilton SM, Perez-Gonzales JF. 1979. Inhibition of the baroreceptor reflex on stimulation in the brain stem defence centre. J Physiol. 288:549–560.
- Crestani CC, Alves FH, Resstel LB, Correa FM. 2006. The bed nucleus of the stria terminalis modulates baroreflex in rats. Neuroreport. 17 14: 1531–1535.
- Crestani CC, Alves FH, Resstel LB, Correa FM. Bed nucleus of the stria terminalis alpha(1)-adrenoceptor modulates baroreflex cardiac component in unanesthetized rats. Brain Res. 2008a; 1245:108–115.
- Crestani CC, Tavares RF, Alves FH, Resstel LB, Correa FM. Diagonal band of Broca modulates the cardiac component of the baroreflex in unanesthetized rats. Neurosci Lett. 2008b; 448 2: 189–193.
- Crestani CC, Alves FH, Tavares RF, Correa FM. 2009. Role of the bed nucleus of the stria terminalis in the cardiovascular responses to acute restraint stress in rats. Stress. 12 3: 268–278.
- Cullinan WE, Herman JP, Battaglia DF, Akil H, Watson SJ. 1995. Pattern and time course of immediate early gene expression in rat brain following acute stress. Neuroscience. 64 2: 477–505.
- DiBona GF, Jones SY, Sawin LL. 1996. Effect of endogenous angiotensin II on renal nerve activity and its arterial baroreflex regulation. Am J Physiol. 271 2 Pt 2: R361–R367.
- DiCarlo SE, Chen CY, Collins HL. 1996. Onset of exercise increases lumbar sympathetic nerve activity in rats. Med Sci Sports Exerc. 28 6: 677–684.
- Evans MH. 1978. Potentiation of a cardioinhibitory reflex by hypothalamic stimulation in the rabbit. Brain Res. 154:331–343.
- Farah VMA, Moreira ED, Pires MD, Irigoyen MCC, Krieger EM. 1999. Comparison of three methods for the determination of baroreflex sensitivity in conscious rats. Braz J Med Biol Res. 32:361–369.
- Glick G, Braunwald E. 1965. Relative roles of the sympathetic and parasympathetic nervous systems in the reflex control of heart rate. Circ Res. 16:363–375.
- Hatton DC, Brooks V, Qi Y, McCarron DA. 1997. Cardiovascular response to stress: Baroreflex resetting and hemodynamics. Am J Physiol. 272 5 Pt 2: R1588–R1594.
- Head GA, McCarty R. 1987. Vagal and sympathetic components of the heart rate range and gain of the baroreceptor–heart rate reflex in conscious rats. J Auton Nerv Syst. 21 2–3: 203–213.
- Hilton SM. 1963. Inhibition of baroreceptor reflexes on hypothalamic stimulation. J Physiol. 165:56–57.
- Irvine RJ, White J, Chan R. 1997. The influence of restraint on blood pressure in the rat. J Pharmacol Toxicol Methods. 38 3: 157–162.
- Jin CB, Rockhold RW. 1989. Effects of paraventricular hypothalamic microinfusions of kainic acid on cardiovascular and renal excretory function in conscious rats. J Pharmacol Exp Ther. 251 3: 969–975.
- Kanbar R, Orea V, Barres C, Julien C. 2007. Baroreflex control of renal sympathetic nerve activity during air-jet stress in rats. Am J Physiol. 292 1: R362–R367.
- Krasney JA, Levitzky MG, Koehler RC. 1974. Sinoaortic contribution to the adjustment of systemic resistance in exercising dogs. J Appl Physiol. 36 6: 679–685.
- Kvetnansky R, McCarty R, Thoa NB, Lake CR, Kopin IJ. 1979. Sympatho-adrenal responses of spontaneously hypertensive rats to immobilization stress. Am J Physiol. 236 3: H457–H462.
- Lawler JE, Obrist PA, Lawler KA. 1975. Cardiovascular function during pre-avoidance, avoidance, and post-avoidance in dogs. Psychophysiology. 12 1: 4–11.
- Ludbrook J, Graham WF. 1985. Circulatory responses to onset of exercise: Role of arterial and cardiac baroreflexes. Am J Physiol. 248 4 Pt 2: H457–H467.
- McDougall SJ, Lawrence AJ, Widdop RE. 2005. Differential cardiovascular responses to stressors in hypertensive and normotensive rats. Exp Physiol. 90 1: 141–150.
- McEwen BS, Wingfield JC. 2003. The concept of allostasis in biology and biomedicine. Hormones and Behavior. 43:2–15.
- Michelini LC. 1994. Vasopressin in the nucleus tractus solitarius: A modulator of baroreceptor reflex control of heart rate. Braz J Med Biol Res. 27 4: 1017–1032.
- Michelini LC. 2007. Differential effects of vasopressinergic and oxytocinergic pre-autonomic neurons on circulatory control: Reflex mechanisms and changes during exercise. Clin Exp Pharmacol Physiol. 34 4: 369–376.
- Nosaka S. 1996. Modifications of arterial baroreflexes: Obligatory roles in cardiovascular regulation in stress and poststress recovery. Jpn J Physiol. 46 4: 271–288.
- Pelosi GG, Resstel LB, Correa FM. 2007. Dorsal periaqueductal gray area synapses modulate baroreflex in unanesthetized rats. Auton Neurosci. 131 1–2: 70–76.
- Resstel LB, Correa FM. 2006. Medial prefrontal cortex NMDA receptors and nitric oxide modulate the parasympathetic component of the baroreflex. Eur J Neurosci. 23 2: 481–488.
- Resstel LB, Fernandes KB, Correa FM. 2004. Medial prefrontal cortex modulation of the baroreflex parasympathetic component in the rat. Brain Res. 1015 1–2: 136–144.
- Resstel LB, Joca SR, Guimaraes FG, Correa FM. 2006. Involvement of medial prefrontal cortex neurons in behavioral and cardiovascular responses to contextual fear conditioning. Neuroscience. 143 2: 377–385.
- Rowell LB, O'Leary DS. 1990. Reflex control of the circulation during exercise: Chemoreflexes and mechanoreflexes. J Appl Physiol. 69 2: 407–418.
- Schadt JC, Hasser EM. 1998. Hemodynamic effects of acute stressors in the conscious rabbit. Am J Physiol. 274 3 Pt 2: R814–R821.
- Schenberg LC, Vasquez EC, da Costa MB. 1993. Cardiac baroreflex dynamics during the defence reaction in freely moving rats. Brain Res. 621 1: 50–58.
- Scheuer DA, Bechtold AG. 2002. Glucocorticoids modulate baroreflex control of heart rate in conscious normotensive rats. Am J Physiol. 282 2: R475–R483.
- Scheuer DA, Mifflin SW. 2001. Glucocorticoids modulate baroreflex control of renal sympathetic nerve activity. Am J Physiol. 280 5: R1440–R1449.
- Schlor KH, Stumpf H, Stock G. 1984. Baroreceptor reflex during arousal induced by electrical stimulation of the amygdala or by natural stimuli. J Auton Nerv Syst. 10 2: 157–165.
- Scopinho AA, Crestani CC, Alves FH, Resstel LB, Correa FM. 2007. The lateral septal area modulates the baroreflex in unanesthetized rats. Auton Neurosci. 137 1–2: 77–83.
- Sgoifo A, Koolhaas JM, Musso E, De Boer SF. 1999. Different sympathovagal modulation of heart rate during social and nonsocial stress episodes in wild-type rats. Physiol Behav. 67 5: 733–738.
- Shammas RA, Denison AL, Pfennig TW, Hemker DP, Stephenson RB. 1988. Baroreflex unimpaired by operant avoidance or classical aversive conditioning in dogs. Am J Physiol 254(6 Pt. 2:R1025–R1034.
- Simon OR, Basuray BN, West WL, Copeland R. 1985. Interaction between the baroreflex and anterior hypothalamic stimulation. Demonstration of a noradrenergic involvement. Neuropharmacology. 24 7: 665–675.
- Su DF, Cerutti C, Barres C, Julien C, Vincent M, Paultre C, Sassard J. 1992. Arterial baroreflex control of heart period is not related to blood pressure variability in conscious hypertensive and normotensive rats. Clin Exp Pharmacol Physiol. 19 11: 767–776.
- Sullebarger JT, Liang CS, Woolf PD, Willick AE, Richeson JF. 1990. Comparison of phenylephrine bolus and infusion methods in baroreflex measurements. J Appl Physiol. 69 3: 962–967.
- Sved AF, Gordon FJ. 1994. Amino acids as central neurotransmitter in the baroreceptor reflex pathway. News Physiol Sci. 9:243–246.
- Tavares RF, Correa FM. 2006. Role of the medial prefrontal cortex in cardiovascular responses to acute restraint in rats. Neuroscience. 143 1: 231–240.
- Verberne AJ, Lewis SJ, Worland PJ, Beart PM, Jarrott B, Christie MJ, Louis WJ. 1987. Medial prefrontal cortical lesions modulate baroreflex sensitivity in the rat. Brain Res. 426 2: 243–249.
- Wang SC, Borison HL. 1947. An analysis of the carotid sinus cardiovascular reflex mechanisms. Am J Physiol. 750:712–721.
- Weinstock M, Rosin AJ. 1984. Relative contributions of vagal and cardiac sympathetic nerves to the reflex bradycardia induced by a pressor stimulus in the conscious rabbit: Comparison of ‘steady state’ and ‘ramp’ methods. Clin Exp Pharmacol Physiol. 11 2: 133–141.
- Yip AW, Krukoff TL. 2002. Endothelin-A receptors and NO mediate decrease in arterial pressure during recovery from restraint. Am J Physiol. 282 3: R881–R889.