Abstract
The effect of emotional stress on the spontaneous baroreceptor reflex (sBRR) in freely moving rats was investigated. Six male Wistar rats equipped with an intra-arterial polyethylene catheter were exposed to a 2-min air-jet stress. For time course analysis of the sBRR response to stress, the records of systolic blood pressure (SBP) and pulse interval (PI) were divided into five regions: baseline (BASELINE), acute exposure to air-jet stress (STRESS), immediate recovery (IMMED. RECOVERY), remaining recovery (RECOVERY), and delayed response (DELAYED RESPONSE). In addition to sBRR sensitivity and effectiveness, we introduce the sequence coverage area and its median for evaluation of the sBRR operating range and set point. During exposure to STRESS and IMMED. RECOVERY, sBRR sensitivity was preserved, its effectiveness was decreased, its operating range was enlarged, and the set point was shifted towards higher SBP and lower PI values. According to the joint symbolic dynamics analysis, the SBP and PI relationship became less predictable hence more prone to respond to stress. In RECOVERY the parameters regained baseline values and DELAYED RESPONSE occurred during which re-setting of sBRR was noted. It follows that emotional stress modulates sBRR differentially during the time course of stress and recovery, affecting both linearity and unpredictability of the BP and PI relationship.
Introduction
The baroreceptor reflex (BRR) is a major negative feedback regulator of arterial blood pressure (BP) that keeps BP in a homeostatic range. Cardiovascular disease is associated with the alterations in BRR activity, and reduced BRR sensitivity (BRS) has been found to be an indicator of the severity of cardiovascular morbidity. For instance, in moderate to severe chronic heart failure (Mortara et al. Citation1997), obstructive sleep apnea (Ryan et al. Citation2007), and myocardial infarction (La Rovere et al. Citation2001), depressed sensitivity of the BRR (cardiac vagal branch) were found to parallel deterioration of the clinical status and to predict poor survival. Reduction in baroreflex sensitivity is also an independent marker of the risk of mortality and major adverse cardiovascular events in hypertensive patients (Ormezzano et al. Citation2008, Narkiewicz and Grassi Citation2008).
It is well established that cardiovascular morbidity and mortality can be triggered by emotional stress (Brotman et al. Citation2007). Acute and chronic emotional stress can cause development of arterial hypertension, either as an independent cardiovascular disease (Meyer et al. Citation2004), or as a part of the metabolic syndrome (Raikkonen et al. Citation2002). Therefore elucidating, and indeed clarifying, the effects of emotional stress on the functioning of the BRR are highly pertinent for shedding light on the development of different pathophysiological states including hypertension.
The recent development of computer-based techniques for the analysis of the spontaneous BRR (sBRR) allows for the analysis of the cardiac sBRR without the use of vasoactive drugs. In this study the functioning of the sBRR was analyzed, for the first time, during the time course of the stress response and recovery in a comprehensive manner, using both linear (Laude et al. Citation2004) and nonlinear (Baumert et al. Citation2005) analytical tools.
We hypothesized that the sBRR of healthy, awake rats exposed to emotional stress changes differentially during the time course of the stress and recovery, and that both the linear and non-linear BP and HR relationships are modulated. Moreover, we introduce new parameters for the estimation of the sBRR operating range and the sBRR set point. Establishing the normal sBRR response during the time course of stress and recovery will open new perspectives for defining unfavorable cardiovascular profiles. Preliminary results of this study were presented at the fifth Conference of the ESGCO in Parma, Italy (Bajić et al. Citation2008).
Methods
Animals
Out-bred male Wistar rats (n = 6, imported from the University of Bristol's animal facility and bred at the animal facility of the Institute of Pharmacology School of Medicine University of Belgrade) weighing (mean ± SEM) 330 ± 10 g were used. Rats were housed individually in Plexiglas cages (25 × 25 × 25 cm) with food pellets (Veterinarski Zavod, Subotica, Serbia) and tap water ad libitum, in controlled laboratory conditions (temperature 21 ± 1°C, relative humidity 60 ± 5%, 07.00–19.00 h light and 19.00–07.00 h dark cycle). The number of animals per experimental group was calculated according to the variability of the parameters in the control group of rats, using statistical software ‘Power Sample Size Calculation’ available at http://biostat.mc.vanderbilt.edu/twiki/bin/view/Main/PowerSampleSize, for power of 90% and type I error probability of 0.05. At the end of the experiment, the rats were euthanized by an overdose of thiopentone sodium (200 mg/kg, i.p.).
All experimental procedures in this study conformed to European Communities Council Directive of November 24, 1986 (86/609/EEC) and the School of Medicine University of Belgrade Guidelines on Animal Experimentation, 2004.
Surgery
Under halothane anesthesia (4% for induction and 2% via mask, equilibrated with air) using an Univentor 400 anesthesia unit (TSE Systems, Bad Homburg, Germany) a polyethylene catheter (PE, ED 0.90 mm, ID 0.58 mm) pre-filled with heparinized (50 IU/ml) saline was inserted in the left femoral artery of a rat and its distal end tunneled subcutaneously to exit at the back of the animal's neck. In order to minimize the effects of stress induced by surgery, we made a small skin incision in the inguinal area ( < 10 mm-long) and at the back of the neck ( < 3 mm-long). To reduce pain and common complications we gave the non-steroidal anti-inflammatory drug metamizol (200 mg/kg i.m.), injected penicillin on the day of surgery (30 IU, i.m.), and sprayed the sutured wounds with bacitracin neomycin combination (Enbecin® spray). Rats were fully recovered 48 h after surgery as judged by their spontaneous behavior, the concentration of serum corticosterone as well as the cardiac sympathetic activity assessed by the basal HR (Shimokawa et al. Citation1998; Maignan et al. Citation2000; Akine et al. Citation2001) and the HR spectral analysis method (Stojičić et al. Citation2008).
Experimental protocol
Each day the experiments started at 11.00 h in a quiet environment, separate from the housing room to avoid disturbances of other rats. To avoid direct animal manipulation during the experiment the femoral arterial catheter was connected to a pressure transducer (IsotecTM, Harvard Apparatus, HSE, Freiburg, Germany) for direct continuous measurement of arterial BP 1 h before the experiment started. The total length of the arterial line was 60 cm with 54 Hz undamped resonance frequency at its tip (Japundzic et al. Citation1990). Rats were then exposed to air-jet stress. Based on a previously published protocol (CitationBarrès et al. 2004), this acute stress was initiated by blowing air (1 bar) for 2 min through a tube (ID 4 mm) directed to the top of the head, avoiding the animal's snout. Although rats were moving freely in their cages, they were equally affected in all parts of the cage since the tube was positioned centrally. For a time course analysis of spontaneous baroreflex response to stress, the records of systolic BP (SBP) and pulse interval (PI) were divided into five regions (): 3 min baseline (BASELINE), 2 min acute exposure to air-jet stress (STRESS), 2 min of immediate recovery (IMMED. RECOVERY) and a remaining 8 min recovery period (RECOVERY), during which the baseline values of SBP and PI were restored. Occurrences of second, delayed concomitant increases of SBP and shortening of PI during the recovery period were analyzed separately (DELAYED RESPONSE).
Figure 1 Time course of SBP and PI changes in a rat exposed to air-jet stress. Exposure to air-jet induces simultaneous increases in SBP and shortening of PI followed by three distinct dynamic regions of SBP and PI changes during recovery: fast (immediate recovery), slow (recovery), and delayed response.
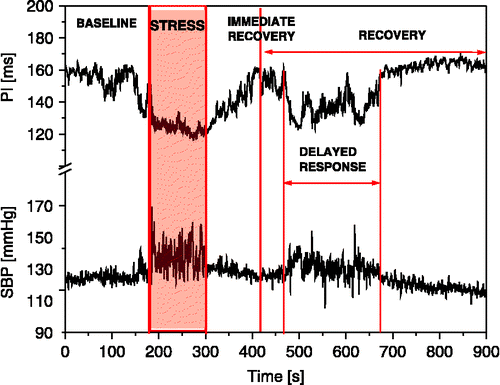
Cardiovascular signal processing and analysis
The arterial BP signal from the IsotecTM transducer was directed to a TAM A amplifier (Harvard Apparatus, Freiburg, Germany), digitized at 1000 Hz and relayed to a PC equipped with Anapres® software (Notocord, Croissy-Sur-Seine, France) for acquisition and analysis of cardiovascular signals. SBP and PI were derived from the arterial BP using software Anapres®. Artifacts were identified visually and the mismatching values were replaced manually offline. The greatest number of artifacts was found during STRESS (5.1%), then during DELAYED RESPONSE (4.7%), then in IMMED. RECOVERY (2.8%). BASELINE and RECOVERY had less than 1.5% of erroneous beats. In order to filter out respiration-induced fluctuations, SBP and PI beat-to-beat series were low-pass filtered by a moving average on 10 cardiac cycles (Oosting et al. Citation1997; Waki et al. Citation2006). This was done because the respiration-induced fluctuations in SBP are non-neural in nature (Japundzic et al. Citation1990) and because BRR has only a minor effect in the genesis of respiratory sinus arrhythmia. Filtering improved the sensitivity of the sequence method: increased the number of sequences and increased the correlation coefficient of sBRS sequences, while sBRS remained unaffected.
Evaluation of the sBRR by the sequence method
This analysis was performed by computer scanning of SBP and PI time series and identification of consecutive beats in which SBP and PI changed in the same direction. As suggested by Iellamo (Citation2001) and Waki et al. (Citation2006), using PI is more appropriate than HR for studying the reflex neural control of the sinus node, primarily because the relationship between the frequency of stimulation of vagal efferent nerves and PI interval responses is linear, while the relationship between HR and vagal stimulation is hyperbolic (Parker et al. Citation1984; Daly Citation1997). Baroreflex time delay from a change in SBP to a reflex response in PI was estimated previously in rats by Oosting et al. (Citation1997). They used pharmacological tools and reported that delay over 3–5 beats is linked to the sBRR. We used the cross-correlation method between SBP and PI of rats under baseline conditions and found that a time shift in the low frequency spectral range (0.2–0.8 Hz) was between 0.61 and 1.51 s, which corresponds to 3–5 beats delay. We also found that the number of sequences was maximal for time lags of 3–5 beats. Therefore, parameters for sBRR evaluation were calculated separately for each delay (3–5 beats) and then averaged for each experimental condition (Waki et al. Citation2006). A chain of SBP–PI pairs was considered a sBRR sequence if it consisted of a minimum of three beats (four SBP–PI pairs). Differences between the successive SBP and PI samples were reduced due to the moving average filter, so no threshold (minimal difference value) was set. We did not consider setting the threshold for coefficient of correlation either, as setting it to 0.5, as suggested by Oosting et al. (Citation1997) would remove less than 0.5% of the original sequences which is of no statistical significance. However a minimal number of sequences within an observation interval was set to three (Laude et al. Citation2004). All spontaneous increases and decreases in SBP (ramps) were calculated and the number of SBP ramps as well as the numbers of sBRR sequences (NS) were normalized and expressed per minute (NR). The following parameters were calculated to evaluate sBRR functioning ():
• | BRS (sBRR sensitivity): assessed as a mean linear regression coefficient (PI = BRS × SBP+const), in (ms/mmHg), where fitting of the line is done in a least square sense; | ||||
• | BEI (sBRR effectiveness index): ratio of number of sBRR sequences vs. number of SBP ramps, | ||||
• | sBRR duration: mean number of SBP–PI pairs (beats) in one sequence; | ||||
• | sBRR operating range (sequence coverage area, SCA) is a rectangle region in SBP PI plane between the lowest and the highest sequence points in both dimensions without 5% outlier points; | ||||
• | sBRR set point: median value of all SBP–PI sequence points. |
Figure 2 Features of the sBRR under baseline conditions and during exposure to stress. Note sBRR sensitivity expressed as a mean regression of all sequences, sBRR operating range (rectangle surface in SBP PI plane between the lower and upper limits), and sBRR set point (calculated as median value of all SBP–PI sequence points).
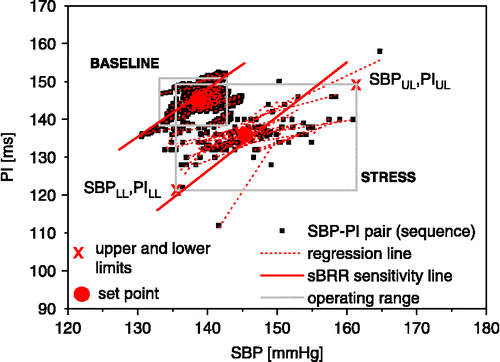
Surrogate data
To validate the sequence method we created two sets of surrogate data. The first set of ‘isospectral’ surrogate data was created by randomizing the phase of Fourier transformed time series. The second set of ‘isodistributional’ surrogate data was created by randomly shuffling the time series (Blaber et al. Citation1995; Porta et al. Citation2000, Citation2002). Surrogate data mimic statistical properties of the original data but not the property that is tested for. Temporal dependence is tested using scrambled ‘isodistributional’ surrogates and phase dependence using ‘isospectral’ surrogates, with mean values of SBP and PI remaining the same.
Evaluation of the sBRR by the method of joint symbolic dynamics (JSD, Baumert et al. Citation2005)
From the matrix X comprising SBP
and PI
time series, a new matrix of new binary symbols 0 and 1 is formed,
, comprising SBP
and PI
symbol series where:
is formed obeying the same rule as in (1). The increase of successive SBP or PI values is coded with ‘1’, while decrease and equilibrium are coded with ‘0’. The three successive symbols form a word. Since the symbols are binary and the length of word is 3, there are 23 = 8 different types of words, i.e. all combinations from [0 0 0] to [1 1 1]. Since four heart beats define three PI intervals, words are able to map dynamics within four consecutive heart beats. The words from the sSBP symbol series are then paired with the words from the sPI symbol series shifted by 3–5 symbols (Varga et al. Citation2007). The total number of different pairs of words is equal to 64 (23 × 23 = 64). A relative frequency for each pair of words is counted from the sSBP and sPI symbol series and averaged over 3–5 shifts. Finally, the relative frequencies in (%) for each of the 8 × 8 SBP and PI words pairs are mapped into the word distribution density matrix W:
To estimate the baroreflex response, we calculated the relative frequencies of word pairs with symmetric (diagonal of the matrix W starting at [SBP000, PI000] to [SBP111, PI111]) and diametric patterns (diagonal of the matrix W starting at [SBP111, PI000] to [SBP000, PI111]). Symmetric word types (JSDsym) refer to baroreflex activation (decreasing SBP is associated with shortening of PI and increasing SBP is associated with lengthening of PI). Diametric word types (JSDdiam) imply suppressed baroreflex activity (Baumert et al. Citation2005). All other word types reflect nonlinear relationship between SBP and PI changes (Varga et al. Citation2007).
Statistics
Results are expressed as mean ± standard error of the mean (SEM). Statistical significance in the same experimental group was assessed using Kruskal Wallis and Dunn's method for multiple comparisons in GraphPad Prism® 4 software (GraphPad Software Inc., San Diego, CA, USA). Statistical significance was considered at p < 0.05.
Results
All rats exposed to air-jet stress exhibited a complex behavioral response involving emotional (fear), motor (startle, escape), and autonomic (micturition, defecation) components. This was accompanied by an immediate and simultaneous increase in SBP and shortening of PI. The maximum SBP and PI responses were reached simultaneously before the end of the exposure time (). After cessation of exposure to the stressor, SBP and PI returned to baseline in about 5 min, exhibiting a time course of recovery that consisted of three characteristic regions: (1) immediate recovery characterized by a fast decline of SBP and lengthening of PI followed by; (2) slower decline of SBP and lengthening of PI, recovery, during which and (3) another short-lasting delayed increase of SBP and shortening of PI occurred.
Mean values of BP and HR during the time course of stress and recovery are shown in . sBRR evaluation was performed at all time points (baseline, stress, immediate recovery, recovery, delayed response) of the cardiovascular response to stress.
Table I. BP and heart rate during the time course of air-jet stress and recovery.
Surrogate data analysis
Isodistributional data show significant decreases in sBRS, number of sequences per minute, sequence length, BEI, and correlation coefficient. Isospectral surrogates show slight decreases in sequence length and correlation coefficient and no significant changes in any other parameter ().
Table II. sBRR evaluation in original and surrogate data.
sBRR during exposure to stress
illustrates changes in parameters for the evaluation of the sBRR by the sequence method during exposure to stress. In , it can be noted that, during stress, the sBRR sensitivity (BRS, slope of SBP PI line) is not changed, although the duration of one sequence is reduced () due to the augmentation of PI (from 2.8 ms ± 0.5 to 5.2 ms ± 0.8, p < 0.01) and SBP (from 2.9 mmHg ± 0.4 to 5.8 mmHg ± 0.4, p < 0.01) swings (mean difference between highest and lowest SBP or PI value in one sequence, Parati et al. Citation2000). Exposure to stress also reduces sBRR effectiveness () as a consequence of a decreased number of sBRR sequences (20.1 ± 3.2 to 13.7 ± 1.4, p < 0.05) without changes in the number of SBP ramps. shows that the sBRR operating range is enlarged while the sBRR set point is shifted to higher SBP and lower PI values, indicating functional resetting of the sBRR.
Figure 3 The sequence method parameters during exposure to air-jet stress. Air-jet stress induces no change in sBRR sensitivity, (a) reduces sBRR effectiveness index (b), shortens sBRR duration (c), enhances sBRR operating range, and displaces the sBRR set point towards higher SBP and lower PI values (d). n = 6 rats; *p < 0.05 versus baseline.
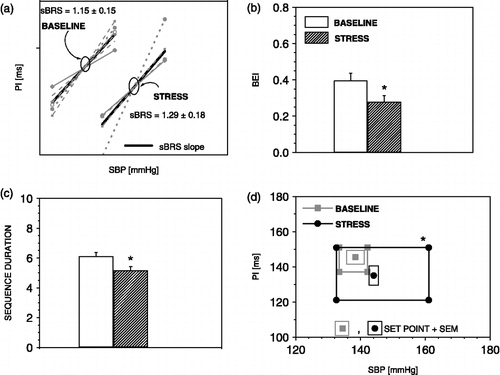
JSD analysis parameters applied to four consecutive SBP and PI word pairs during exposure to stress are summarized in . During exposure to stress, symmetric words, particularly SBP000 and PI000 and SBP111 and PI111, were found to decrease (). An increased occurrence of some JSD diametric words, as well as of the residual words (neither symmetric nor diametric), is shown in . This is associated with statistically significant decrease in deviation from uniform distribution of all JSD symbols during exposure to stress ().
Figure 4 JSD parameters during exposure to air-jet stress. During exposure to stress, JSD symmetric words decreased (a), as well as the deviation of all JSD words from uniform distribution (b). Note a prominent decrease of SBP000 and PI000, and, SBP111 and PI111, word pairs (c). n = 6 rats; *p < 0.05, **p < 0.01, ***p < 0.001 versus baseline.
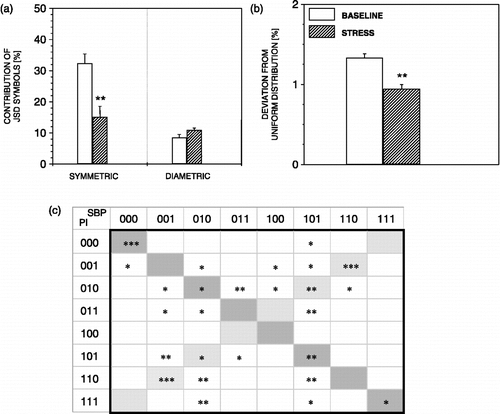
sBRR during immediate recovery
During immediate recovery, when the SBP steeply decreases and PI steeply increases (), the sBRR sensitivity (), and sBRR duration () regain baseline values. However, the sBRR effectiveness () still remains decreased due to decreased number of sBRR sequences (from 20.1 ± 3.2 to 13.6 ± 2, p < 0.05) and unchanged number of SBP ramps. The sBRR operating range is still significantly enlarged (), and sBRR set point is shifted to the lower PI and higher SBP values indicating a resetting of the sBRR during immediate recovery.
Figure 5 The sequence method parameters during immediate recovery from air-jet stress. During immediate recovery, there was no change in sBRR sensitivity (a), sBRR effectiveness index was still reduced (b), sBRR duration has recovered (c), sBRR operating range remained enhanced while the sBRR set point was displaced towards higher SBP and lower PI values (d). n = 6 rats; *p < 0.05 versus baseline.
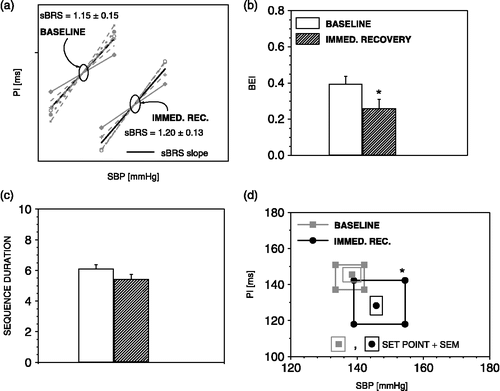
Moreover, during immediate recovery, JSD symmetric words were decreased (). At the same time, non-symmetric and non-diametric words were increased (), causing statistically significant overall symbol deviation from the uniform distribution ().
sBRR during recovery
Later in the recovery period, when moderate decrease of SBP and increase of PI values were recorded, all parameters of the sBRR, recovered (). illustrates that all JSD analysis parameters recovered also. However, during this phase of recovery, all rats exhibited a second, delayed increase of SBP and decrease of PI ().
sBRR during delayed response
Application of the sequence method during the delayed response reveals a significant shift of the sBRR set point ().
Figure 9 The sequence method parameters during delayed response to air-jet stress. During delayed response, the sBRR operating range was again enlarged and the sBRR set point displaced towards higher SBP and lower PI values (d). n = 6 rats; **p < 0.01 versus baseline.
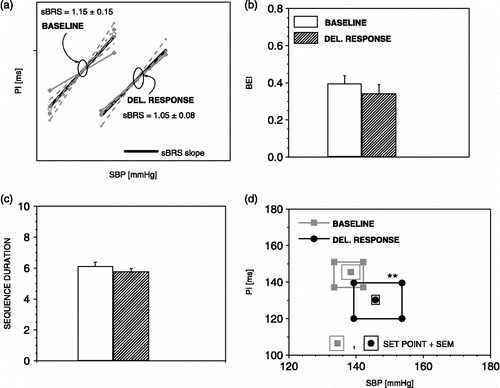
JSD detected no change in overall symbols dynamics during the delayed response in comparison with baseline values (), except for a prominent decrease of SBP111 and PI111 symmetric words ().
Discussion
Air-jet stress is a model of experimental emotional stress. When exposed to it, rats exhibit a complex behavioral response integrated at the hypothalamic level, involving fear originating from amygdala, motor manifestations escape or startle, and autonomic reactions, micturition, defecation and a vigorous cardiovascular response (Stojičić et al. Citation2008). Exposure of rats to air-jet stress induced an essentially instantaneous and abrupt increase in BP and shortening of PI followed by the reverse changes during immediate recovery and a more gradual decline of BP and PI until normalization. During recovery, we observed a second, unexpected and short-lasting increase of BP and shortening of PI that was unassociated with direct exposure to the stressor, so we termed it ‘delayed response’ (Stojičić et al. Citation2008). In the ascending part of the SBP record and the descending part of the PI record, depicting the response to direct exposure to the air-jet, the sequence method revealed that sBRR sensitivity is not inhibited nor enhanced and that it does not differ from baseline conditions (). However, fine analysis of the sBRR sequence uncovered that reduction of sBRR duration coupled to enhanced SBP and PI swings occur, possibly as a consequence of concomitant increase in peripheral resistance and cardiac vagal response. Along with these changes, we noted a reduction of BEI resulting from a decreased number of sBRR sequences. At the same time, the sBRR operating range was enlarged while the sBRR set point was re-set towards higher SBP and lower PI values (). The results show that during stress the sBRR operates with preserved sensitivity, less effectively over a wider operating range, re-set towards higher SBP and lower PI values.
Accumulated evidence indicates that the cardiovascular response to emotional stress is governed from the dorsomedial hypothalamic area—dorsomedial hypothalamus (DMH, defense area). However, the modulation of the BRR during exposure to emotional stress remains to be clarified. Mifflin et al. (Citation1988) have shown that electrical stimulation of the DMH of anesthetized cats increased BP and heart rate, and that the stimulation of DMH shunted excitatory synaptic potentials evoked from arterial baroreceptors in neurons of the nucleus tractus solitarii (NTS). Subsequently, they found that hypothalamic stimulation evoked inhibitory postsynaptic potentials in these baroreceptor-activated NTS neurons (Silva-Carvalho et al. Citation1995), which were gamma-amino-butyric acid mediated (Jordan et al. Citation1988; Mifflin et al. Citation1988). Therefore, they proposed that the BRR is inhibited during stress. However, in urethane anesthetized rats, McDowall et al. (Citation2006) noted that disinhibition of DMH, by microinjections of a GABAA receptor antagonist, increased sympathetic vasomotor discharge, cardiac activity, the BRR operating range, and reset the BRR to higher SBP values without affecting BRR sensitivity, challenging the view of Jordan et al. (Citation1988), Mifflin et al. (Citation1988), and Silva-Carvalho et al. (Citation1995). Experiments in conscious animals by Schadt and Hasser (Citation1998) in rabbits, and Kanbar et al. (Citation2007) in rats confirmed that the BRR during stress is functional. Schadt and Hasser (Citation1998) showed that exposure of rabbits to air-jet stress resets cardiac BRR without affecting its gain and the operating range. However, Kanbar et al. (Citation2007) found that during air-jet stress the BRR sensitivity of renal sympathetic nervous activity is increased, operates over a wider range and is reset towards higher BP values.
Apart from differences in species, the use or otherwise of anesthesia, homotypic (Schadt and Hasser Citation1998; Kanbar et al. Citation2007) or heterotypic (Hatton et al. Citation1997) air-jet stressors, that can certainly account for differences in these findings, it is important to mention that aforementioned studies employed the pharmacological method for the evaluation of the BRR. The main disadvantage of the use of pharmacological agents (NO donors and α-adrenergic vasoconstrictors) is that they modulate the functioning of the cardiovascular system, and thus interfere with BRR analysis (Bertinieri et al. Citation1988; Oosting et al. Citation1997; Casadei and Peterson Citation2000; Paton et al. Citation2001). In addition, the HR response to vasoactive drug infusions is delayed (hysteresis) during air-jet stress as the heart rate remains elevated regardless of drug-induced changes in BP, precluding proper analysis of the cardiac component of the BRR (Kanbar et al. Citation2007). In contrast, the sequence analysis calculates the sBRS as slope of SBP versus PI linear regression in spontaneously occurring BP ramps (Fritsch et al. Citation1986; Bertinieri et al. Citation1988), thus providing data on dynamic BRR changes in the physiological range. It can be applied on non-stationary as well as stationary SBP and PI recordings. The sBRS obtained this way is reliable both in rats (Oosting et al. Citation1997; Waki et al. Citation2006) and humans (Parlow et al. Citation1995; Parati et al. Citation2000), shows a high correlation to the reference pharmacological method, and gives a zero value following surgical and chemical interruption of the BRR arch (Bertinieri et al. Citation1988; Parlow et al. Citation1995; Oosting et al. Citation1997). It is also important to emphasize that this method does not arouse the rats, and does not interfere with stress-induced effects.
Infusion of vasoactive drugs demands prolonged stimulation of animals with air-jet during which BP and HR partially decrease indicating that habituation occurs (Hatton et al. Citation1997; Schadt and Hasser Citation1998). Our experimental protocol rules out habituation as exposure to air-jet stress is short-lasting and the analysis involves only the ascending part of the SBP recording and the corresponding descending part of the PI tracing (). Also, the short-lasting exposure to stress allowed us to define the sBRR changes during the whole time course of recovery. Using the sequence method, we found that during immediate recovery when SBP steeply decreases and PI steeply increases, the sBRR, BEI, operating range, and the set point of the sBRR do not differ from exposure to stress. Hence, the system is still prone to respond adequately to the stressor in this phase of recovery. During late recovery, when all parameters return to basal values, we observed occurrences of abrupt and short-lasting SBP and PI changes, resembling in shape the response during exposure to air-jet stress. In this phase of recovery, we found re-setting and increase in the operating range of the sBRR. In our previous study (Stojičić et al. Citation2008) we noted that rats pre-treated with anxiolytic drugs or with a new class of antidepressant drugs, vasopressin V1b receptor antagonists, recovered faster and did not exhibit a ‘delayed response’ suggesting that central mechanisms play an important role in cardiovascular regulation during late recovery also.
Although SBP and PI have complex interactions (Wagner and Persson Citation1998), neither the classical Oxford method nor the method of sequences provides information on the non-linear part of their relationship. In order to retrieve information on SBP PI non-linear relationships, we applied the method of JSD. As suggested by Baumert et al. (Citation2005), we applied the concept of symbolic dynamics that enables a simplified description of the SBP PI dynamics of the cardiovascular system with a limited number of words. This method has been successfully applied to uncover pathophysiological cardiovascular control in dilated cardiomyopathy (Baumert et al. Citation2005). In our experiments, JSD analysis uncovered that the cardiovascular systems gain complexity during exposure to air-jet stress and immediate recovery, as indicated by the deviation of SBP and PI symbol dynamics from uniform distribution (). The reduction of sBRR associated events, as denoted by JSDsym, is in agreement with BEI decrease observed with the sequence method. Reduction of sBRR associated events is compensated by increased complexity of SBP PI dynamics (). During the delayed response, although JSDsym did not change significantly we observed SBP111, PI111 word pair decreases, suggesting reduction in sBRR associated events. In physiological terms, the cardiovascular system benefits from increased dynamic complexity during exposure to air-jet stress. It becomes less susceptible to destabilization by stressor-induced perturbations, less conditioned by the initial state, and it can make large changes with minimum expenditure of energy. All this makes the cardiovascular system more able to respond to the stressor (Wagner and Persson Citation1998). Reduction of complexity can be an important parameter in assessing functional pathology and has been found to be associated with poor prognosis in cardiovascular disease (Goldstein et al. Citation1998; Kennedy Citation1998). Although air-jet stress is associated with behavioural responses similar to panic reaction in humans, it is not an experimental model for panic attacks because they are not triggered by external stimuli. Nevertheless it is worth mentioning that panic disorder in humans is associated with unfavorable cardiovascular profiles (Yeragani et al. Citation2004), increased incidence of cardiovascular co-morbidity and sudden death (Shioiri et al. Citation2005).
In conclusion, the sequence method analysis of the sBRR during exposure of rats to air-jet stress and immediate recovery reveals that the sBRR is operating with preserved sensitivity, decreased effectiveness and enlarged range re-set towards higher SBP and PI values. According to JSD analysis, the SBP and PI relationship becomes less predictable and more able to respond. In spite of the resemblance in the shape of SBP and PI response during the delayed response with exposure to stress, no changes in sBRR functioning could be noted except for re-setting. It follows that emotional stress modulates the sBRR differentially during the time course of stress and recovery.
Acknowledgements
This work was supported by Wellcome Trust (UK) Grant No. 074392 and the Ministry of Science (RS) Grant No. OI145062 and No. TR11022.
Declaration of interest: There is no conflict of interest for the authors. The authors alone are responsible for the content and writing of the article.
References
- Akine A, Suzuka H, Hayashida Y, Kato Y. 2001. Effects of ketamine and propofol on autonomic cardiovascular function in chronically instrumented rats. Auton Neurosci. 87:201–208.
- Bajić D, Stojičić S, Šarenac O, Lončar-Turukalo T, Bojić T, Japundžić-Žigon N. 2008 Temporal analysis of spontaneous baroreceptor reflex during emotional stress in freely moving rats. [Proceeding] 5th Conference of the European Study Group on Cardiovascular Oscillations ESGCO. 7–9 April, Parma, Italy O12: 5–7
- Barrès C, Cheng Y, Julien C. Steady-state and dynamic responses of renal sympathetic nerve activity to air-jet stress in sinoaortic denervated rats. Hypertension. 43:629–635.
- Baumert M, Baier V, Truebner S, Schirdewan A, Voss A. 2005. Short- and long-term joint symbolic dynamics of heart rate and blood pressure in dilated cardiomyopathy. IEEE Trans Biomed Eng. 52:2112–2115.
- Bertinieri G, Di Rienzo M, Cavallazzi A, Ferrari AU, Pedotti A, Mancia G. 1988. Evaluation of baroreceptor reflex by blood pressure monitoring in unanesthetized cats. Am J Physiol. 254:377–383.
- Blaber AP, Yamamoto Y, Hughson RL. 1995. Methodology of spontaneous baroreflex relationship assessed by surrogate data analysis. Am J Physiol Heart Circ Physiol. 268:H1628–H1687.
- Brotman DJ, Golden SH, Wittstein IS. 2007. The cardiovascular tool of stress. Lancet. 370:1089–1100.
- Casadei B, Paterson DJ. 2000. Should we still use nitrovasodilators to test baroreflex sensitivity?. J Hypertens. 18:3–6.
- Daly M. 1997. Peripheral Arterial Chemoreceptors and Respiratory Cardiovascular Integration. Monographs of the Physiological Society No. 46, Oxford Medical Publications, UK.
- Di Rienzo M, Parati G, Castiglioni P, Tordi R, Mancia G, Pedotti A. 2001. Baroreflex effectiveness index: An additional measure of baroreflex control of heart rate in daily life. Am J Physiol Regul Integr Comp Physiol. 280:744–751.
- Fritsch JM, Eckberg DL, Graves LD, Wallin BG. 1986. Arterial pressure ramps provoke linear increases of heart period in humans. Am J Physiol. 251:R1086–R1090.
- Goldstein B, Fiser DH, Kelly MM, Mickelsen D, Ruttimann U, Pollack MM. 1998. Decomplexification in critical illness and injury: Relationship between heart rate variability, severity of illness, and outcome. Crit Care Med. 26:352–357.
- Hatton DC, Brooks V, Qi Y, McCarron DA. 1997. Cardiovascular response to stress: Baroreflex resetting and hemodynamics. Am J Physiol. 272:1588–1594.
- Iellamo F. 2001. Neural mechanisms of cardiovascular regulation during exercise. Auton Neurosci. 90:66–75.
- Japundzic N, Grichois ML, Zitoun P, Laude D, Elghozi JL. 1990. Spectral analysis of blood pressure and heart rate in conscious rats: Effects of autonomic blockers. J Auton Nerv Syst. 30:91–100.
- Jordan D, Mifflin SW, Spyer KM. 1988. Hypothalamic inhibition of neurons in the nucleus tractus solitarius of the cat is GABA mediated. J Physiol. 399:389–404.
- Kanbar R, Orea V, Barres C, Julien C. 2007. Baroreflex control of renal sympathetic nerve activity during air-jet stress in rats. Am J Physiol Regul Integr Comp Physiol. 292:362–367.
- Kennedy HL. 1998. Heart rate variability-a potential, noninvasive prognostic index in the critically ill patients. Crit Care Med. 26:213–214.
- La Rovere MT, Pinna GD, Hohnloser SH, Marcus F, Mortara A, Nohara R, Bigger JTJr, Camm AJ, Schwartz PJ. 2001. Baroreflex sensitivity and heart rate variability in the identification of patients at risk for life-threatening arrhythmias: Implications for clinical trials. Circulation. 103:2072–2077.
- Laude D, Elghozi JL, Girard A, Bellard E, Bouhaddi M, Castiglioni P, Cerutti C, Cividjian A, Di Rienzo M, Fortrat JO, Janssen B, Karemaker JM, Lefthériotis G, Parati G, Persson PB, Porta A, Quintin L, Regnard J, Rüdiger H, Stauss HM. 2004. Comparison of various techniques used to estimate spontaneous baroreflex sensitivity (the EuroBaVar study). Am J Physiol Regul Integr Comp Physiol. 286:R226–R231.
- Maignan E, Dong WX, Legrand M, Safar M, Cuche JL. 2000. Sympathetic activity in the rat: Effects of anaesthesia on noradrenaline kinetics. J Auton Nerv Syst. 80:46–51.
- McDowall LM, Horiuchi J, Killinger S, Dampney RA. 2006. Modulation of the baroreceptor reflex by the dorsomedial hypothalamic nucleus and perifornical area. Am J Physiol Regul Integr Comp Physiol. 290:1020–1026.
- Meyer CM, Armenian HK, Eaton WW, Ford DE. 2004. Incident hypertension associated with depression in the baltimore epidemiologic catchment area follow-up study. J Affect Disord. 83:127–133.
- Mifflin SW, Spyer KM, Withington-Wray DJ. 1988. Baroreceptor inputs to the nucleus tractus solitarius in the cat: Modulation by hypothalamus. J Physiol. 399:369–387.
- Mortara A, La Rovere MT, Pinna GD, Prpa A, Maestri R, Febo O, Pozzoli M, Opasich C, Tavazzi L. 1997. Arterial baroreflex modulation of heart rate in chronic heart failure. Clinical and hemodynamic correlates and prognostic implications. Circulation. 96:3450–3458.
- Narkiewicz K, Grassi G. 2008. Impaired baroreflex sensitivity as a potential marker of cardiovascular risk in hypertension. J Hypertens. 26:1303–1304.
- Oosting J, Struijker-Boudier HAJ, Janssen BJA. 1997. Validation of a continuous baroreceptor reflex sensitivity index calculated from spontaneous fluctuations of blood. J Hypertens. 15:391–399.
- Ormezzano O, Cracowski JL, Quesada JL, Pierre H, Mallion JM, Baguet JP. 2008. EVAluation of the prognostic value of BARoreflex sensitivity in hypertensive patients: The EVABAR study. J Hypertens. 26:1373–1378.
- Parati G, Di Rienzo M, Mancia G. 2000. How to measure baroreflex sensitivity: From the cardiovascular laboratory to daily life. J Hypertens. 18:7–19.
- Parker P, Celler BG, Potter EK, McCloskey DI. 1984. Vagal stimulation and cardiac slowing. J Auton Nerv Syst. 11:226–231.
- Parlow J, Viale JP, Annat G, Hughson R, Quintin L. 1995. Spontaneous cardiac baroreflex in humans. Comparison with drug-induced responses. Hypertension. 25:1058–1068.
- Paton JF, Deuchars J, Ahmad Z, Wong LF, Murphy D, Kasparov S. 2001. Adenoviral vector demonstrates that angiotensin I induced depression of the cardiac baroreflex is mediated by endothelial nitric oxide synthase in the nucleus tractus solitarii of the rat. J Physiol. 531:445–458.
- Porta A, Baselli G, Rimoldi O, Malliani A, Pagani M. 2000. Assessing baroreflex gain from spontaneous variability in conscious dogs: Role of causality and respiration. Am J Physiol Heart Circ Physiol. 279:H2558–H2567.
- Porta A, Furlan R, Rimoldi O, Pagani M, Malliani A, van de Borne P. 2002. Quantifying the strength of the linear causal coupling in closed loop interacting cardiovascular variability signals. Biol Cybern. 86:241–251.
- Raikkonen K, Matthews KA, Kuller LH. 2002. The relationship between psychological risk attributes and the metabolic syndrome in healthy women: Antecedent or consequence?. Metabolism. 51:1573–1577.
- Ryan S, Ward S, Heneghan C, McNicholas WT. 2007. Predictors of decreased spontaneous baroreflex sensitivity in obstructive sleep apnea syndrome. Chest. 131:1100–1107.
- Schadt JC, Hasser EM. 1998. Hemodynamic effects of acute stressors in the conscious rabbit. Am J Physiol Regul Integr Comp Physiol. 274:814–821.
- Shimokawa A, Kunitake T, Takasaki M, Kannan H. 1998. Differential effects of anesthetics on sympathetic nerve activity and arterial baroreceptor reflex in chronically instrumented rats. J Auton Nerv Syst. 72:46–54.
- Shioiri T, Kojima-Maruyama M, Hosoki T, Kitamura H, Tanaka A, Yoshizawa M, Bando T, Someya T. 2005. Disfunctional baroreflex regulation of sympathetic nerve activity in remitted patients with panic disorder. Eur Arch Psychiatry Clin Neurosci. 255:293–298.
- Silva-Carvalho L, Dawid-Milner MS, Spyer KM. 1995. The pattern of excitatory inputs to the nucleus solitarii evoked on stimulation in the hypothalamic defence area in the cat. J Physiol. 487:727–737.
- Stojičić S, Milutinović-Smiljanić S, Šarenac O, Milosavljević S, Paton JFR, Murphy D, Japundžić-Žigon N. 2008. Blockade of central vasopressin receptors reduces the cardiovascular response to acute stress in freely moving rats. Neuropharmacology. 54:824–836.
- Varga D, Lončar-Turukalo T, Bajić D, Milutinović S, Japundžić-Žigon N. 2007. Joint symbolic dynamics of cardiovascular time series of rats. IFMBE Proceedings MEDICON. 16:773–776.
- Wagner CD, Persson PB. 1998. Chaos in the cardiovascular system: An update. Cardiovasc Res. 40:257–264.
- Waki H, Katahira K, Polson JW, Kasparov S, Murphy D, Paton JF. 2006. Automation of analysis of cardiovascular autonomic function from chronic measurements of arterial pressure in conscious rats. Exp Physiol. 91:201–213.
- Yeragani VK, Mallavarapu M, Radhakrishna KA, Tancer M, Uhde T. 2004. Linear and nonlinear measures of blood pressure variability: Increased chaos of blood pressure time series in patients with panic disorder. Depress Anxiety. 19:85–95.