Abstract
Physiological functions of oxytocin released during stress are not well understood. We have (1) investigated the release of oxytocin during chronic stress using two long-term stress models and (2) simulated stress-induced oxytocin secretion by chronic treatment with oxytocin via osmotic minipumps. Plasma oxytocin levels were significantly elevated in rats subjected to acute immobilization stress for 120 min, to repeated immobilization for 7 days and to combined chronic cold stress exposure for 28 days with 7 days immobilization. To simulate elevation of oxytocin during chronic stress, rats were implanted with osmotic minipumps subcutaneously and treated with oxytocin (3.6 μg/100 g body weight/day) or vehicle for 2 weeks. Chronic subcutaneous oxytocin infusion led to an increase in plasma oxytocin, adrenocorticotropic hormone, corticosterone, adrenal weights and heart/body weight ratio. Oxytocin treatment had no effect on the incorporation of 5-bromo-2-deoxyuridine into DNA in the heart ventricle. Mean arterial pressure response to intravenous phenylephrine was reduced in oxytocin-treated animals. Decrease in adrenal tyrosin hydroxylase mRNA following oxytocin treatment was not statistically significant. Oxytocin treatment failed to modify food intake and slightly increased water consumption. These data provide evidence on increased concentrations of oxytocin during chronic stress. It is possible that the role of oxytocin released during stress is in modulating hypothalamic—pituitary–adrenocortical axis and selected sympathetic functions.
Introduction
Despite its known effects on myoepithelial and myometrial cell function, oxytocin remains an orphan hormone in respect to its peripheral effects, especially in males. Oxytocin is released into the circulation in response to various types of acute stress stimuli (Jezova et al. Citation1995). These include physical, emotional, metabolic and osmotic stressors (Hashiguchi et al. Citation1997; Jezova and Skultetyova Citation1997; Nishioka et al. Citation1998; Wotjak et al. Citation1998). However, the information on increased oxytocin secretion in response to repeated or chronic stress situations is limited. Elevated concentrations of plasma oxytocin were found following 3-day forced swimming (Wotjak et al. Citation1998), 3-day shaker stress (Dubovicky et al. Citation2007), isolation stress for 60 days (Grippo et al. Citation2007) or chronic inflammatory/nociceptive stress (Suzuki et al. Citation2009).
The physiological function of oxytocin released into the circulation during stress is not clear. Oxytocin has been postulated to facilitate mammalian reproduction, such as parturition and lactation (Russell et al. Citation2003). Apparently, these effects are not relevant to stress-induced oxytocin release in males and non-pregnant females. Several other actions of oxytocin can be important for coping with stress situations.
Thus, oxytocin participates in the control of body fluid homeostasis (Gimpl and Fahrenholz Citation2001). It is likely that oxytocin, similarly as his closely related hormone vasopressin, participates in the regulation of fluid balance during conditions of stress (Hew-Butler et al. Citation2008).
Oxytocin released during stress may contribute to the control of other hormones involved in the stress response. It is well known that oxytocin present and acting in the brain inhibits basal and stress-induced activity of the hypothalamic–pituitary–adrenocortical (HPA) axis, particularly in female rats (Neumann et al. Citation2000; Windle et al. Citation2004). However, little information is available on HPA axis activity in response to a rise in circulating oxytocin. Peripherally injected oxytocin was described both to stimulate and to inhibit adrenocorticotropic hormone (ACTH) release (Gibbs Citation1986; Jezova et al. Citation1995). As to other stress hormones, oxytocin was found to influence catecholamine release (Gibbs Citation1986; Mahata and Ghosh Citation1991). In this respect, oxytocin may act at the level of the adrenal medulla because an enhanced number of chromaffin cells accompanied by an increase in catecholamine content in the adrenal medulla were observed in rats treated repeatedly with oxytocin (Plecas et al. Citation1989). A direct influence of oxytocin on catecholamine synthesizing enzymes has not been studied yet.
Another function of oxytocin released during stress may be the control of blood pressure. Oxytocin is considered to be a cardiovascular hormone (Jankowski et al. Citation1998; Gutkowska et al. Citation2000). Single peripheral administration of oxytocin resulted in increases (Costa-E-Sousa et al. Citation2005; Bakos et al. Citation2008) in the mean arterial pressure (MAP), while repeated treatment with oxytocin led to a reduction in the MAP (Petersson et al. Citation1996). Recent observations obtained by our research group indicate that oxytocin may induce cardioprotective effects (Ondrejcakova et al. Citation2009). Moreover, oxytocin concentrations in the heart were found to decrease in response to voluntary wheel running (Bakos et al. Citation2007), and oxytocin receptors in the heart were upregulated by endurance training (Gutkowska et al. Citation2007).
In the present study, we have attempted (1) to investigate the release of oxytocin during chronic stress using two long-term stress models and (2) to simulate stress-induced oxytocin secretion by chronic treatment with oxytocin via osmotic minipumps to evaluate possible effects of oxytocin on hormones of the HPA axis, blood pressure responses to vasoactive drugs and gene expression of catecholamine-synthesizing enzymes in the adrenal gland.
Materials and methods
Animals
Adult male Wistar (350–480 g) or Sprague Dawley (acute and chronic stress experiments; 250–270 g) rats (AnLab s.r.o., Prague, Czech Republic) were used. The animals were kept four per cage, unless stipulated otherwise. They were kept under standard housing conditions with a constant 12:12 h light/dark cycle (lights on at 06.00 h) and temperature at 22°C ± 2°C. Food and water were available ad libitum. Principles of laboratory animal care and all procedures were approved by the Animal Health and Animal Welfare Division of the State Veterinary and Food Administration of the Slovak Republic.
Chronic stress exposure
The rats (n = 7–8 per group) were exposed to one of the stress paradigms or remained undisturbed in their home cages (controls). The first chronic stress model consisted of repeated immobilization for 120 min daily for 7 days. The second chronic stress model consisted of permanent exposure to cold (4°C, 28 days) combined with 120 min immobilization on the last day of cold exposure. For comparison, one group of animals was exposed to an acute immobilization stress (120 min) at room temperature. The rats were killed by decapitation and the trunk blood was collected for measurements of oxytocin concentrations. EDTA was used as an anticoagulant.
Treatment with oxytocin
The animals were randomly assigned to vehicle- and oxytocin-treated rats. Oxytocin (H-2510, Bachem, Switzerland) or vehicle (saline) was continuously administered via osmotic minipumps (Model 2002, Alzet, Alza Corp., Palo Alto, CA, USA) for 2 weeks. Osmotic minipumps were implanted subcutaneously as described previously (Hlavacova and Jezova Citation2008). The concentration of oxytocin used to fill the pumps was calculated based on the mean pump infusion rate provided by the manufacturer (0.5 μl/h, 14 days), the body weight of animals and the dose intended. The minipumps delivered oxytocin at a dose of 3.6 μg/100 g body weight/day. Oxytocin was dissolved in isotonic saline. A total of 220 μl were loaded into each minipump. Control animals received minipumps that contained vehicle only. After implantation, rats were housed individually.
Cannulations
Polyethylene cannulas (Smiths Medical International, Ltd, Hythe, Kent, UK) were inserted into the tail artery and jugular vein under the combined ketamine (Narkamon 5% – 1.2 ml/kg) and xylazine (Rometar 2% – 0.4 ml/kg) anaesthesia. The cannulas were filled with heparinized saline (300 IU/ml), tunnelled under the skin of the back, protected with steel springs and exteriorized through the top of the cage, allowing the rats free movements. The incision was subsequently treated with an antibiotic. After the cannulation procedure was completed, the animals were housed individually. Administrations of vasoactive peptides and blood pressure measurements were performed in conscious, freely moving rats 24 h later.
Experimental protocols
Experiment 1
On the 14th day following minipumps implantation, vehicle- (n = 8) and oxytocin-treated (n = 8) rats were killed by decapitation in the morning between 10.00 and 12.00 h. The trunk blood was collected into cooled polyethylene tubes containing EDTA as anticoagulant and centrifuged immediately at 4°C to separate plasma, which was then stored at − 20°C until analysed. Organs such as thymus, heart ventricles and adrenal glands were quickly removed and immediately weighed. The tissues were kept frozen at − 70°C until use. In additional groups of rats treated with vehicle (n = 7) or oxytocin (n = 7), the water consumption and food intake were measured daily at 10.00 h.
Experiment 2
In a group of vehicle- (n = 4) and oxytocin-treated (n = 7) animals, 5-bromo-2-deoxyuridine (BrdU, Roche, Mannheim, Germany), an indicator of cell proliferation, was injected twice at a dose of 150 mg/kg intraperitoneally on the 14th day following minipumps implantation. The time difference between the first and the second injection was around 5 h. Rats were decapitated 3 days after the BrdU injections.
Experiment 3
Cannulations were performed in 17 rats (8 vehicle- and 9 oxytocin-treated) on the 13th day following minipump implantation. On the 14th day, the measurements of MAP and heart rate were performed using a computerized direct blood pressure system (DBP001, Kent Scientific Corporation, Torrington, CT, USA) that was configured with a MacIntosh IIvx personal computer. This methodology has been used in stress research repeatedly (e.g. Kvetnansky et al. Citation1997; Moncek et al. Citation2003), as stress hormones are not elevated during the measurements. Arterial cannulas were connected to transducers and basal MAP parameters were registered for 10 min. Thereafter, the α1-adrenergic receptor agonist phenylephrine (Phenylephrine, Sigma, St Louis, MO, USA) at a dose of 200 μg/kg/ml was injected intravenously via the canula in the jugular vein without interruption of the recordings which were taken at 10-s intervals. Fifteen minutes later, when arterial pressure came back to normal values, a solution of angiotensin II (Angiotensin II, Sigma, St Louis, MO, USA) at a dose of 500 ng/kg/ml was infused within 2 min and the MAP was recorded for another 10 min.
Measurements
Hormone assays
Oxytocin and vasopressin concentrations in plasma were determined by specific radioimmunoassays (RIAs) described previously (Jezová and Michajlovskij Citation1992; Bakos et al. Citation2007). Plasma concentrations of ACTH were determined by RIA (Jezová et al. Citation1987), using double antibody technique to separate free and bound fractions. Plasma corticosterone levels were analysed by RIA after dichloromethane extraction as described earlier (Jezová et al. Citation1994).
DNA isolation
Left heart ventricle tissue was homogenized (Heidolph Homogenizer DIAX 900, Burladingen, Germany) in homogenization Tris buffer (50 mM NaCl, 10 mM Tris–HCl, pH 7.5) and then sonicated for 60 s using MSE sonicator (Scientific Instruments, Darwen, Lancashire, England) with an intensity setting of 5 μm amplitude. The final concentration was 0.25 mg tissue/1 μl buffer. DNA was extracted from 20 mg of sonicated tissue using a commercially available nucleic acid purification kit (Wizard DNA Purification Kit, Promega, Madison, WI, USA), following the manufacturer's instructions. DNA concentration was analysed spectrophotometrically (GeneQuant Pro, Biochrom Ltd, Cambridge, UK) at 260 nm and stored at − 4°C.
Analysis of BrdU incorporation into DNA
BrdU incorporation into DNA was measured by sandwich enzyme-linked immunosorbent assay (ELISA) developed by Behl et al. (Citation2006). Primary monoclonal anti-BrdU antibody (formalin grade; Roche) was purified on high-trap affinity columns (1 ml rProtein A FF, Amersham Biosciences, Uppasala, Sweden). The BrdU ELISA was performed in 96-well microtitre plates (Maxisorb, Nunc, Rochester, NY, USA). The plates were coated overnight with a solution of the anti-BrdU antibody (5 μg/ml, 100 μl/well) in coating buffer (0.05 M sodium carbonate buffer, pH 9.6). This was followed by blocking with 1% bovine serum albumin in phosphate-buffered saline for 60 min and washing with Tris-buffered saline with Tween 20 (TBST; 20 mM Tris–HCl, 150 mM NaCl, 0.05% Tween 20, pH 7.6) five times. Then the extracts of DNA with incorporated BrdU (100 μl/well) were edited and incubated for 120 min. Then the plates were washed with TBST buffer five times followed by 120 min incubation with the secondary anti-BrdU antibody conjugated with peroxidase (Roche) and washed with TBST buffer five times. Peroxidase activity was determined by adding 100 μl/well of 3,3′,5,5′-tetramethylbenzidine substrate (Roche) for 15 min. The reaction was stopped with 50 μl of 1 M H2SO4 and the reaction product was determined by absorption at 450 nm.
Isolation of RNA and measurement of gene expression
Reverse transcription polymerase chain reaction (RT-PCR) was used for measurements of adrenomedullary tyrosine hydroxylase (TH), dopamine β-hydroxylase (DBH) and phenylethanolamine N-methyl transferase (PNMT) mRNA levels. Total RNA was prepared from frozen rat adrenal tissue homogenized in 500 μl of the RNAzol™ reagent (Tel-Test, Friendwood, TX, USA) according to the manufacturer's instructions. RT was performed from 1.5 μg of total RNA using Ready-To-Go You-Prime First-Strand Beads (Amersham Biosciences) and pd(N)6 primer according to the manufacturer's protocol. To determine mRNA levels in adrenals of rats, the primers used are shown in . After the initial denaturation at 94°C for 5 min, PCR program included 25 cycles of denaturation at 94°C for 1 min, annealing at 60–63°C for 30 s and polymerization at 72°C for 1 min. All reactions were performed in the linear phase of amplification, where the efficiency between samples was comparable and did not reach a plateau. PCR products were separated on 2% agarose/ethidium bromide gels. Intensity of individual bands was evaluated by analysis system STS 6220I (Ultra-Lum, Inc., Claremont, CA, USA) and relative expression values were calculated by analysis of band intensities using PCBAS 2.08e software (Raytest, Inc., Wilmington, NC, USA). Semi-quantitative values were expressed relatively to the housekeeping gene, glyceraldehyde-3-phosphate dehydrogenase (GAPDH). GAPDH was chosen for normalization because it was not affected by oxytocin treatment (as compared to saline).
Table I. Sequences of used primers and conditions of PCR reaction.
Statistical analysis
The data on oxytocin, corticosterone, ACTH and vasopressin levels, as well as BrdU incorporation, concentration of mRNA of the catecholamine enzymes and relative organ weights were analysed using a one-way analysis of variance (ANOVA) with treatment as variable. Post hoc analysis was performed using Tukey tests. Statistical analysis of changes in MAP, heart rate, food and water intake was done by ANOVA for repeated measures with treatment and time as variables. The incremental areas under the curve (iAUC) were calculated using the trapezoidal rule. Results are expressed as means or percentage of pretreatment values ± SEM. The overall level of statistical significance was defined as p < 0.05.
Results
Plasma concentrations of oxytocin increased in response to both acute and chronic stress stimuli. One-way ANOVA showed a significant main effect for factor stress (F(3,26) = 7.9970, p < 0.001). Tukey post hoc test revealed significant increases in plasma oxytocin in response to acute immobilization (p < 0.01), repeated immobilization (p < 0.01) and combined chronic cold exposure and immobilization (p < 0.001) compared to hormone levels in the control, unstressed group ().
Figure 1. Plasma oxytocin in response to acute and chronic stress stimuli. 1 × IMO, single immobilization for 120 min; 7 × IMO, immobilization for 120 min daily for 7 days; 28 days cold stress+1 × IMO, cold stress for 28 days+120 min of immobilization on the 28th day (means ± SEM, n = 7–8). Statistical significance **p < 0.01, ***p < 0.001, stress vs. control.
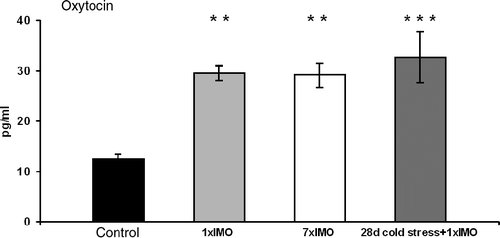
Prolonged release of oxytocin via osmotic minipumps resulted in a significant increase in oxytocin concentrations in plasma, as revealed by one-way ANOVA (F(1,14) = 26.961, p < 0.001). Treatment with oxytocin led to a mild elevation in plasma ACTH as well as corticosterone levels (). As determined by one-way ANOVA, plasma ACTH (F(1,12) = 8.34, p < 0.05) and corticosterone (F(1,12) = 5.65, p < 0.05) were significantly higher in oxytocin-treated animals compared to those in controls. Comparison of the organ weights showed that oxytocin-treated rats had higher relative weights of adrenal glands (F(1,14) = 5.7371, p < 0.05, ) but not absolute adrenal or thymus weights () than the vehicle-treated animals. The initial body weight measured before minipumps implantation was slightly lower in rats of oxytocin (441 ± 4 g) compared to those of the control (459 ± 8 g) group, and this difference became significant following the treatments (). Treatment with oxytocin had no effect on absolute weight of heart ventricles () but resulted in an increase in the heart/body weight ratio (F(1,14) = 5.38, p < 0.05, ). Measurement of the incorporation of BrdU into DNA in the left heart ventricle failed to reveal any differences between oxytocin- and vehicle-treated rats (). Oxytocin treatment had no effect on plasma concentrations of vasopressin (VEH: 0.78 ± 0.02 pg/ml; OXY: 0.81 ± 0.03 pg/ml).
Figure 2. Effects of oxytocin treatment on plasma levels of oxytocin, ACTH, corticosterone, relative adrenal and heart weights and on the incorporation of BrdU into DNA of the left ventricle. Values are expressed as means ± SEM (n = 4–8).
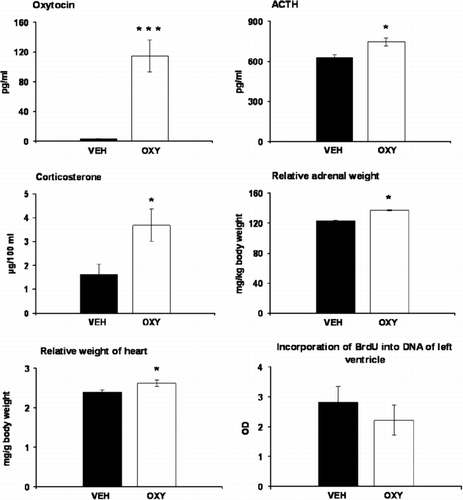
Table II. Absolute body and organ weights at the end of vehicle or oxytocin treatment for 14 days. The values are expressed as means ± SEM (eight rats per group).
Basal values of heart rate (OXY: 310 ± 10 beats/min; VEH: 297 ± 9 beats/min) and MAP (OXY: 101 ± 2 mmHg; VEH: 98 ± 3 mmHg) were similar in oxytocin- and vehicle-treated rats. MAP rose in response to injection of phenylephrine and the rise was less pronounced in oxytocin-treated rats compared to vehicle-treated controls (). The analysis of values (at 1-min intervals) by ANOVA for repeated measures showed significant main effects for factor treatment (F(1,15) = 4.49, p = 0.05) and time (F(9,135) = 68.648, p < 0.001). The fall of heart rate in response to phenylephrine-induced rise in blood pressure has a similar pattern in both treatment groups (data not shown). MAP rose also in response to angiotensin II infusion (factor time: F(9,135) = 56.037, p < 0.001), but there were no differences between the treatment groups ().
Figure 3. MAP responses to phenylephrine and angiotensin II in oxytocin-treated animals compared to those in controls. Values are expressed as percentage of appropriate pre-treatment values (means ± SEM, n = 8–9). Bar charts represent the values of iAUC. *p = 0.05, oxytocin vs. vehicle.
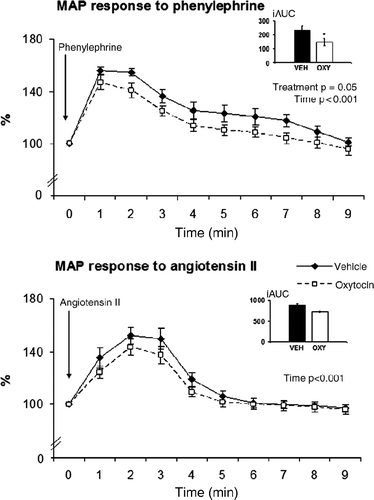
Concentrations of mRNA of the different catecholamine biosynthetic enzymes in adrenal medulla are shown in . TH mRNA levels were decreased slightly in oxytocin-treated group and the difference approached statistical significance (VEH: 0.94 ± 0.04 a.u.; OXY: 0.84 ± 0.03 a.u.; p = 0.07). Oxytocin treatment failed to modify mRNA levels coding for DBH or PNMT.
Table III. Concentrations of mRNA of the different catecholamine biosynthetic enzymes in adrenal medulla in oxytocin-treated compared to those in vehicle-treated rats. The values are expressed in arbitrary units as means ± SEM (eight rats per group).
To evaluate potential effects of oxytocin on ingestive behaviour, food and water consumption were measured in additional groups of rats with implanted osmotic minipumps. The implantation of the minipumps performed under anaesthesia in the afternoon of day 0 resulted in attenuated food intake the following night as measured on day 1 of the treatment (). Thereafter, the food intake increased gradually (F(14,168) = 9.3464, p < 0.001 for factor time) without any significant changes for factors treatment or treatment × time interaction. The statistical analysis of water consumption data did not reveal significant main effects for factors treatment but there was a significant factor time (F(14,154) = 2.0833, p < 0.05) and time × treatment interaction (F(14,154) = 1.8129, p < 0.05). Post hoc comparisons showed that the increased water intake in oxytocin-treated rats was significant on the 2nd to 4th day of treatments.
Discussion
The results of the present study provide evidence that chronic stressors increase oxytocin levels and demonstrate that prolonged increase in circulating oxytocin leads to activation of the HPA axis as demonstrated by elevated plasma ACTH and corticosterone as well as enlarged adrenal glands. Next, prolonged oxytocin treatment failed to modify cell proliferation in the heart ventricle, but led to a reduction in blood pressure in response to the α1-adrenergic drug phenylephrine. An influence of oxytocin on the sympathetic-adrenomedullary system cannot be excluded.
Present experiments confirmed our previous findings on the activation of oxytocin release during acute immobilization stress (Jezová et al. Citation1993; Jezova and Skultetyova Citation1997). This is consistent with the view that oxytocin is a stress hormone released into the circulation in response to various types of acute stress stimuli (Jezova et al. Citation1995). The present results show for the first time that immobilization is inducing oxytocin release in rats after a long-term exposure for at least 7 days. A significant rise in plasma oxytocin was observed when the rats were exposed to chronic cold stress combined with immobilization on the last day. The latter finding can be of significant physiological importance, as it allows us to suggest that even if an adaptation to a homozygous stressor may happen (Hashiguchi et al. Citation1997), the responses to other, heterozygous stress stimuli occurring in the life could be maintained.
The administration of oxytocin via osmotic minipumps at the dose rate used resulted in plasma oxytocin levels approaching those which may be observed during intensive stress situations such as injection of hypertonic saline (Jezova et al. Citation1995) or immobilization (present study). Continuous treatment with this mild dose of oxytocin exerted a stimulatory action on ACTH and corticosterone release. This is consistent with previous observation of a stimulatory effect of single injection of oxytocin (Gibbs Citation1984; Petersson et al. Citation1999). To our knowledge, no information on plasma ACTH or corticosterone concentrations in response to chronic treatment with oxytocin is available. Petersson et al. (Citation1999) used repeated treatment with relatively high doses of oxytocin and evaluated HPA axis hormones 1–10 days after the last injection (when plasma oxytocin levels were apparently no more increased). They observed a decrease in plasma corticosterone but not ACTH levels. The present data show that chronic elevation of plasma oxytocin is associated with increased activity of the HPA axis manifested by elevated concentrations of plasma ACTH and corticosterone as well as enlarged adrenals. It remains to be elucidated whether the enlargement of the adrenals was due to an increase in cell number or hypertrophy of the cells in the adrenal cortex and/or medulla.
Changes in stress hormone concentrations observed in oxytocin-treated rats do not seem to be related to alterations in food intake. Brain oxytocinergic system is considered to play a role in the regulation of ingestive behaviour, and central administration of oxytocin results in food inhibition (Arletti et al. Citation1990; Olson et al. Citation1991; Valassi et al. Citation2008). There are limited data on the inhibition of feeding behaviour induced by single peripheral administration of high doses of oxytocin (Arletti et al. Citation1990). To our knowledge, no information is available on chronic peripheral treatment with oxytocin, which failed to modify the food intake in the present study. Oxytocin receptors within the central nervous system appear to mediate also an inhibition of drinking responses (Fitts et al. Citation2003). In the present experiments, oxytocin induced a mild increase in water intake particularly during the first week of chronic peripheral treatment. These data are consistent with an increased water intake induced by intermittent subcutaneous administration of oxytocin in food-deprived rats for 3 days (Bernal et al. Citation2007).
We failed to observe any effects of oxytocin treatment on plasma vasopressin concentrations. Vasopressin is a peptide similar to oxytocin in structure as well as in some physiological effects. A chronic increase in plasma oxytocin levels, as induced in the present study, does not seem to modify vasopressin release into the circulation.
Recent studies using cell cultures have shown that oxytocin is involved in cell differentiation and proliferation, usually stimulating the growth of primary cells and inhibiting the proliferation of cancer cells (Reversi et al. Citation2005; Cattaneo et al. Citation2008). Among these effects of oxytocin, positive influence on cardiogenesis by promoting the differentiation of cardiomyocytes was described (Paquin et al. Citation2002; Matsuura et al. Citation2004). An increase in heart/body weight ratio induced by chronic treatment with oxytocin in the present experiments substantiated the prediction that oxytocin might have influenced cell proliferation in the heart. However, the quantification of incorporated BrdU, as a measure of cell proliferation in vivo, failed to reveal any changes in heart ventricles in response to oxytocin treatment. It is possible that the increase in heart/body weight ratio was due to an increase in the size of cardiomyocytes that, however, needs further verification.
Chronic mild elevation in peripheral, circulating oxytocin achieved in the present study did not modify resting blood pressure or reflex fall of the heart rate. These results are consistent with the data of Petersson et al. (Citation1996) demonstrating a decrease in blood pressure following repeated treatment with high but not low doses of oxytocin. Interestingly, a reduction in basal blood pressure and heart rate was observed also in knockout mice with oxytocin deficiency (Rigatto et al. Citation2003; Bernatova et al. Citation2004). Moreover, lack of oxytocin in these animals was not associated with the modulation of the pressor response to vasoactive agents, such as centrally administered angiotensin II (Rigatto et al. Citation2003).
The blood pressure response to intravenous phenylephrine, an α1-adrenergic agonist, was reduced by oxytocin treatment. Under the conditions of present experiments, the elevation of peripheral oxytocin had no influence on the pressor response to intravenous infusion of angiotensin II. The reduced pressor response to phenylephrine does not seem to be mediated by the mild increase in corticosterone concentrations in oxytocin-treated rats as glucocorticoids were shown to potentiate responses to vasoactive substances (Scheuer and Bechtold Citation2001; Van Acker et al. Citation2002). Based on our preliminary measurements in the heart (unpublished data), it is possible that the effects of chronically elevated oxytocin levels are mediated by other systems, such as atrial natriuretic peptide which is known to mediate cardiovascular actions of oxytocin (Gutkowska et al. Citation2000).
The decrease in gene expression of the TH in the adrenals, another parameter related to catecholaminergic system measured in the present study, was not statistically significant. The available knowledge on possible effect of oxytocin on catecholamines or sympathetic nervous system is very limited. An indirect evidence of the relationship between oxytocin and sympathetic activity in humans is the observation that oxytocin might be a partial mediator of the attenuating effect of partner support on resting levels of circulating noradrenaline in women (Grewen et al. Citation2005). In another study, intranasal administration of oxytocin was related to a lower sympathetic activity in human males (Goganau et al. Citation2008). Thus, further investigation of the involvement of oxytocin in sympathetic and adrenomedullary systems is warranted.
A limitation of the presented studies is that the administration of oxytocin in osmotic minipumps led to a constant increase in peripheral oxytocin, while this hormone exerts a daily rhythm and/or a rhythm of responsiveness to stressful stimuli under physiological conditions (Forsling Citation2000; Dubovicky et al. Citation2007). We cannot exclude that the chronic increase in circulating oxytocin induced a downregulation of oxytocin receptors or other compensations which may, or may not, occur under chronic stress conditions. Future studies are needed with respect to possible gender and species differences.
In conclusion, plasma oxytocin concentrations are increased in rats subjected to two types of chronic stress situations, and the induction of chronic elevation in circulating oxytocin leads to an activation of the HPA axis, does not influence cell proliferation in heart ventricles and results in a reduction in MAP response to α1-receptor stimulation. It is possible that the role of oxytocin released during stress is in modulating the HPA axis and selecting sympathetic functions.
Acknowledgements
The study was supported by the grants of APVV (LPP-0194-06) and CENDO. The authors wish to thank Dr Natasa Hlavacova as well as Ludmila Zilava and Jana Blaskova for their kind help.
Declaration of interest: The authors report no conflicts of interest. The authors alone are responsible for the content and writing of the paper.
References
- Arletti R, Benelli A, Bertolini A. 1990. Oxytocin inhibits food and fluid intake in rats. Physiol Behav. 48:825–830.
- Bakos J, Hlavacova N, Makatsori A, Tybitanclova K, Zorad S, Hinghofer-Szalkay H, Johansson BB, Jezova D. 2007. Oxytocin levels in the posterior pituitary and in the heart are modified by voluntary wheel running. Regul Pept. 139:96–101.
- Bakos J, Bobryshev P, Tillinger A, Kvetnanský R, Jezova D. 2008. Phenylethanolamine N-methyltransferase gene expression in the heart and blood pressure response to oxytocin treatment in rats exposed to voluntary wheel running. Ann N Y Acad Sci. 1148:302–307.
- Behl B, Klos M, Serr M, Ebert U, Janson B, Drescher K, Gross G, Schoemaker H. 2006. An ELISA-based method for the quantification of incorporated BrdU as a measure of cell proliferation in vivo. J Neurosci Methods. 158:37–49.
- Bernal A, Mahía J, Puerto A. 2007. Oxytocin, water intake, and food sodium availability in male rats. Horm Behav. 52:289–296.
- Bernatova I, Rigatto KV, Key MP, Morris M. 2004. Stress-induced pressor and corticosterone responses in oxytocin-deficient mice. Exp Physiol. 89:549–557.
- Cattaneo MG, Chini B, Vicentini LM. 2008. Oxytocin stimulates migration and invasion in human endothelial cells. Br J Pharmacol. 153:728–736.
- Costa-E-Sousa RH, Pereira-Junior PP, Oliveira PF, Olivares EL, Werneck-de-Castro JP, Mello DB, Nascimento JH, Campos-de-Carvalho AC. 2005. Cardiac effects of oxytocin: Is there a role for this peptide in cardiovascular homeostasis?. Regul Pept. 132:107–112.
- Dubovicky M, Mach M, Key M, Morris M, Paton S, Lucot JB. 2007. Diurnal behavioral and endocrine effects of chronic shaker stress in mice. Neuro Endocrinol Lett. 28:846–853.
- Fitts DA, Thornton SN, Ruhf AA, Zierath DK, Johnson AK, Thunhorst RL. 2003. Effects of central oxytocin receptor blockade on water and saline intake, mean arterial pressure, and c-Fos expression in rats. Am J Physiol Regul Integr Comp Physiol. 285:R1331–R1339.
- Forsling ML. 2000. Diurnal rhythms in neurohypophysial function. Exp Physiol. 85:179S–186S.
- Gibbs DM. 1984. Dissociation of oxytocin, vasopressin and corticotropin secretion during different types of stress. Life Sci. 35:487–491.
- Gibbs DM. 1986. Oxytocin inhibits ACTH and peripheral catecholamine secretion in the urethane-anesthetized rat. Regul Pept. 14:125–132.
- Gimpl G, Fahrenholz F. 2001. The oxytocin receptor system: Structure, function, and regulation. Physiol Rev. 81:629–683.
- Goganau I, Zahiu D, Ioan S, Nastase A, Braga RI, Zagrean AM. 2008. Oxytocin induces variable changes in pain perception in human males. FENS Abstract. 4:18913.
- Grewen KM, Girdler SS, Amico J, Light KC. 2005. Effects of partner support on resting oxytocin, cortisol, norepinephrine, and blood pressure before and after warm partner contact. Psychosom Med. 67:531–538.
- Grippo AJ, Cushing BS, Carter CS. 2007. Depression-like behavior and stressor-induced neuroendocrine activation in female prairie voles exposed to chronic social isolation. Psychosom Med. 69:149–157.
- Gutkowska J, Jankowski M, Mukaddam-Daher S, McCann SM. 2000. Oxytocin is a cardiovascular hormone. Braz J Med Biol Res. 33:625–633.
- Gutkowska J, Paquette A, Wang D, Lavoie JM, Jankowski M. 2007. Effect of exercise training on cardiac oxytocin and natriuretic peptide systems in ovariectomized rats. Am J Physiol Regul Integr Comp Physiol. 293:R267–R275.
- Hashiguchi H, Ye SH, Morris M, Alexander N. 1997. Single and repeated environmental stress: effect on plasma oxytocin, corticosterone, catecholamines, and behavior. Physiol Behav. 61:731–736.
- Hew-Butler T, Noakes TD, Soldin SJ, Verbalis JG. 2008. Acute changes in endocrine and fluid balance markers during high-intensity, steady-state, and prolonged endurance running: unexpected increases in oxytocin and brain natriuretic peptide during exercise. Eur J Endocrinol. 159:729–737.
- Hlavacova N, Jezova D. 2008. Chronic treatment with the mineralocorticoid hormone aldosterone results in increased anxiety-like behavior. Horm Behav. 54:90–97.
- Jankowski M, Hajjar F, Kawas SA, Mukaddam-Daher S, Hoffman G, McCann SM, Gutkowska J. 1998. Rat heart: A site of oxytocin production and action. Proc Natl Acad Sci USA. 95:14558–14563.
- Jezova D, Skultetyova I. 1997. Neuroendocrine response in stress – a specific activation by individual stressors. Arch Physiol Biochem. 105:233–237.
- Jezova D, Skultetyova I, Tokarev DI, Bakos P, Vigas M. 1995. Vasopressin and oxytocin in stress. Ann N Y Acad Sci. 771:192–203.
- Jezová D, Michajlovskij N. 1992. N-methyl-d-aspartic acid injected peripherally stimulates oxytocin and vasopressin release. Endocr Regul. 26:73–75.
- Jezová D, Kvetnanský R, Kovács K, Oprsalová Z, Vigas M, Makara GB. 1987. Insulin-induced hypoglycemia activates the release of adrenocorticotropin predominantly via central and propranolol insensitive mechanisms. Endocrinology. 120:409–415.
- Jezová D, Michajlovskij N, Kvetnanský R, Makara GB. 1993. Paraventricular and supraoptic nuclei of the hypothalamus are not equally important for oxytocin release during stress. Neuroendocrinology. 57:776–781.
- Jezová D, Guillaume V, Juránková E, Carayon P, Oliver C. 1994. Studies on the physiological role of ANF in ACTH regulation. Endocr Regul. 28:163–169.
- Kvetnanský R, Pacák K, Tokarev D, Jeloková J, Jezová D, Rusnák M. 1997. Chronic blockade of nitric oxide synthesis elevates plasma levels of catecholamines and their metabolites at rest and during stress in rats. Neurochem Res. 22:995–1001.
- Mahata SK, Ghosh A. 1991. Neural influence on oxytocin-induced changes of adrenomedullary catecholamines in the pigeon. Regul Pept. 33:183–190.
- Matsuura K, Nagai T, Nishigaki N, Oyama T, Nishi J, Wada H, Sano M, Toko H, Akazawa H, Sato T, Nakaya H, Kasanuki H, Komuro I. 2004. Adult cardiac Sca-1-positive cells differentiate into beating cardiomyocytes. J Biol Chem. 279:11384–11391.
- Moncek F, Aguilera G, Jezova D. 2003. Insufficient activation of adrenocortical but not adrenomedullary hormones during stress in rats subjected to repeated immune challenge. J Neuroimmunol. 142:86–92.
- Neumann ID, Wigger A, Torner L, Holsboer F, Landgraf R. 2000. Brain oxytocin inhibits basal and stress-induced activity of the hypothalamo–pituitary–adrenal axis in male and female rats: partial action within the paraventricular nucleus. J Neuroendocrinol. 12:235–243.
- Nishioka T, Anselmo-Franci JA, Li P, Callahan MF, Morris M. 1998. Stress increases oxytocin release within the hypothalamic paraventricular nucleus. Brain Res. 781:56–60.
- Olson BR, Drutarosky MD, Chow MS, Hruby VJ, Stricker EM, Verbalis JG. 1991. Oxytocin and an oxytocin agonist administered centrally decrease food intake in rats. Peptides. 12:113–118.
- Ondrejcakova M, Ravingerova T, Bakos J, Pancza D, Jezova D. 2009. Oxytocin exerts protective effects on in vitro myocardial injury induced by ischemia and reperfusion. Can J Physiol Pharmacol. 87:137–142.
- Paquin J, Danalache BA, Jankowski M, McCann SM, Gutkowska J. 2002. Oxytocin induces differentiation of P19 embryonic stem cells to cardiomyocytes. Proc Natl Acad Sci USA. 99:9550–9555.
- Petersson M, Alster P, Lundeberg T, Uvnäs-Moberg K. 1996. Oxytocin causes a long-term decrease of blood pressure in female and male rats. Physiol Behav. 60:1311–1315.
- Petersson M, Hulting AL, Uvnäs-Moberg K. 1999. Oxytocin causes a sustained decrease in plasma levels of corticosterone in rats. Neurosci Lett. 264:41–44.
- Plećas B, Ugresić N, Hristić M, Popović A, Jovović D. 1989. The response of rat adrenal medulla to oxytocin. Arch Int Physiol Biochim. 97:303–308.
- Reversi A, Cassoni P, Chini B. 2005. Oxytocin receptor signaling in myoepithelial and cancer cells. J Mammary Gland Biol Neoplasia. 10:221–229.
- Rigatto K, Puryear R, Bernatova I, Morris M. 2003. Salt appetite and the renin–angiotensin system: Effect of oxytocin deficiency. Hypertension. 42:793–797.
- Russell JA, Leng G, Douglas AJ. 2003. The magnocellular oxytocin system, the fount of maternity: Adaptations in pregnancy. Front Neuroendocrinol. 24:27–61.
- Scheuer DA, Bechtold AG. 2001. Glucocorticoids potentiate central actions of angiotensin to increase arterial pressure. Am J Physiol Regul Integr Comp Physiol. 280:R1719–R1726.
- Suzuki H, Onaka T, Kasai M, Kawasaki M, Ohnishi H, Otsubo H, Saito T, Hashimoto H, Yokoyama T, Fujihara H, Dayanithi G, Murphy D, Nakamura T, Ueta Y. 2009. Response of arginine vasopressin-enhanced green fluorescent protein fusion gene in the hypothalamus of adjuvant-induced arthritic rats. J Neuroendocrinol. 21:183–190.
- Valassi E, Scacchi M, Cavagnini F. 2008. Neuroendocrine control of food intake. Nutr Metab Cardiovasc Dis. 18:158–168.
- Van Acker SA, Oitzl MS, Fluttert MF, de Kloet ER. 2002. Centrally regulated blood pressure response to vasoactive peptides is modulated by corticosterone. J Neuroendocrinol. 14:56–63.
- Windle RJ, Kershaw YM, Shanks N, Wood SA, Lightman SL, Ingram CD. 2004. Oxytocin attenuates stress-induced c-fos mRNA expression in specific forebrain regions associated with modulation of hypothalamo–pituitary–adrenal activity. J Neurosci. 24:2974–2982.
- Wotjak CT, Ganster J, Kohl G, Holsboer F, Landgraf R, Engelmann M. 1998. Dissociated central and peripheral release of vasopressin, but not oxytocin, in response to repeated swim stress: New insights into the secretory capacities of peptidergic neurons. Neuroscience. 85:1209–1222.