Abstract
Stress, an important aspect of modern life, has long been associated with an altered homeostatic state. Little is known about the effect of the life stress on the outcome of diabetes mellitus, especially related to the higher risk of infections. Here, we evaluate the effects of chronic mild stress (CMS) exposure on the evolution of type I diabetes induced by streptozotocin administration in BALB/c mice. Exposure of diabetic mice to CMS resulted in a significant reduction of survival and a sustained increase in blood glucose values. Concerning the immune response, chronic stress had a differential effect in mice with diabetes with respect to controls, showing a marked decrease in both T- and B-cell proliferation. No correlation was found between splenic catecholamine or circulating corticosterone levels and the proliferative response. However, a significant negative correlation was found between glucose levels and concanavalin A- and lipopolysaccharide-stimulated proliferative responses of T and B cells. A positive correlation between blood glucose and splenic catecholamine concentrations was found in diabetic mice but not in controls subjected to CMS. Hence, the present report shows that diabetic mice show a worse performance in immune function after stress exposure, pointing to the importance of considering life stress as a risk factor for patients with diabetes.
Introduction
Medical advances throughout the 20th century have resulted in the transformation of many acute and once incurable illnesses into chronic conditions. As a result, the prevalence of chronic diseases has increased rapidly. In addition, environmental factors such as pollutants and lifestyle changes such as sedentary habits and overeating have also contributed to the increase in chronic illnesses.
Diabetes mellitus is a group of disorders that share a common defect in the control of carbohydrate metabolism. There are two principal types of diabetes mellitus, type I or insulin-dependent diabetes and type II or non-insulin-dependent diabetes. Because type II diabetes is associated with ageing and obesity, this form of the disease is becoming more prevalent. Therefore, diabetes is one of the most challenging and burdensome chronic diseases of the 21st century, and it is a growing threat to the world's public health (King et al. Citation1995, Citation1998).
While data accumulate on the emotional issues that play an important role in the lives of people with diabetes (Cox and Gonder-Frederick Citation1992), little is known about the effect of life stress on the outcome of diabetes. Daily problems in living with diabetes can disrupt disease management and metabolic control, thus increasing the risk for developing complications of diabetes. Among these, an increased susceptibility to infection was described (Joshi et al. Citation1999; Peleg et al. Citation2007). Diabetes might be at a higher risk for moderate or severe infection-related morbidity caused by altered defence mechanisms. It was shown that individuals with diabetes show poorer outcomes after infection and increased incidence of nosocomial infections compared with normal subjects (Joshi et al. Citation1999; Bertoni et al. Citation2001). One potential mediator of the altered defence mechanisms is hyperglycaemia (McMahon and Bistrian Citation1995). Hyperglycaemia has been identified as the main factor contributing to the development of diseases associated with diabetes mellitus. Uncontrolled hyperglycaemia may be associated with increased risk of nosocomial infections in critically affected patients, even in those without a history of diabetes (Christiansen et al. Citation2004; Butler et al. Citation2005). Recently, we have demonstrated that high glucose concentrations can directly affect lymphoid cell growth leading to cell death (Rubinstein et al. Citation2008).
In this scenario, the aim of the present work was to evaluate the effects of chronic stress exposure on the evolution of the diabetic condition and in particular on the immune response. Considering that the adaptive response to stressors involves the activation of the hypothalamic–pituitary–adrenal (HPA) axis and the sympathetic nervous system (SNS), their participation was also evaluated. For this purpose, a streptozotocin (STZ)-induced model of type I diabetes in mice was used. The regimen of multiple doses of STZ is highly dependent on the mouse strain and age. We chose 2-month-old BALB/cByJ mice because this strain has an intermediate susceptibility to diabetes induction (Leiter et al. Citation1988) and displays a chronic stable mild hyperglycaemia without a requirement for insulin therapy and has a lifespan similar to normal mice. Hence, the conclusions emerging from our study could be important for both types of diabetes. To investigate the effects of chronic stress, we used the well-established chronic mild stress (CMS) model described by Willner et al. (Citation1992) in rats and by Monleon et al. (Citation1995) in mice, as previously detailed (Palumbo et al. Citation2007). The CMS model implies chronic low-grade stressors offering a realistic simulation of life stress. The mice were subjected to CMS after 1 week of diabetes induction, when the diabetic state is already established. We hypothesized that in vitro proliferative responses to stimulation of both T and B cells would be more impaired in mice with diabetes exposed to CMS.
Materials and methods
Animals
Inbred female BALB/c mice were purchased from Facultad de Veterinaria, Universidad de Buenos Aires. Sixty-day-old mice weighing between 20 and 25 g at the beginning of the experiments were used. Mice were maintained on a 12-h light/dark cycle (lights on at 08:00 h and off at 20:00 h) in a room with controlled temperature (18–22°C). Unless stated otherwise, food and water were freely available. The mice were killed by conscious decapitation.
Four experimental groups of 40 mice each were used in the experiment. Additional groups of 20 mice were used in a first step to study the evolution (glycaemia and survival) in the different experimental groups. One group was housed (five mice per cage) in normal conditions (CONTROL), a second group was singly housed and subjected to unpredictable CMS, a third group (five mice per cage) was treated with STZ to induce diabetes type I (DIAB) and a fourth group was subjected to CMS (individually housed) after 1 week of STZ treatment (DIAB+CMS). It is important to note that time of CMS exposure was always 1 week less than the time since diabetes induction.
Experimental diabetes
To induce the diabetic state, one daily dose of STZ (Sigma Chemical Co., St Louis, MO, USA; 40 mg/kg in 100 μl of citrate buffer, pH = 4.5) was administered intraperitoneally (i.p.) to the mice during five consecutive days (Like and Rossini Citation1976). Blood glucose measurements were performed with a One Touch Ultra test strip glucometer (Lifescan, Johnson-Johnson Co., Milpitas, CA, USA; useful range, 20–600 mg/dl) in blood samples obtained from mice through a small skin incision at the tip of the tail. Glycaemia was determined after 3 h of fasting. To ensure that all mice had a similar fasting time, food was removed at 10-min intervals between groups, and glycaemia was determined between 12:00 and 12:30 h once a week throughout the experiment. Mice injected with the vehicle at the same time were used as the respective controls.
CMS model
The stress scheme was slightly modified from the one previously used in rats by Willner et al. (Citation1992) and in mice by Monleon et al. (Citation1995) and consisted of 16 h (including 12 h overnight) of water deprivation, two periods of continuous overnight illumination, two periods (7 and 17 h) of 45° cage tilt, one 17-h period in a soiled cage (100 ml water in sawdust bedding), one period of food deprivation (8:4 h during the light and 4 h during the dark period) and one 17-h period of paired housing (mice were always housed in the same pairs, but the location alternated between the home cages of each member of the pair). All the individual stressors used have been classified as “mild” according to the Animals (Scientific Procedures) Act of 1986 (UK legislation). The stressors were applied alternately throughout the weeks, as previously described (Palumbo et al. Citation2007).
Experimental protocols were approved by the Internal Ethics Committee of the School of Medicine of the University of Buenos Aires, namely the Comite Institucional para Cuidado y Uso de los Animales de Laboratorio (CICUAL).
Cell suspensions and culture conditions
Lymphoid cell suspensions from lymph nodes (axillary, inguinal and mesenteric) or spleen were obtained as described previously (Silberman et al. Citation2002). The cell culture medium used was RPMI 1640 (Gibco™, Invitrogen, Carlsbad, CA, USA), containing 0.2 g% glucose (Sigma Chemical Co.). Briefly, lymphoid organs were aseptically removed and disrupted through a 1-mm metal mesh and the resulting cell suspensions were filtered through a 10-μm nylon mesh. After three washes in RPMI 1640 medium, cells were resuspended in RPMI 1640 supplemented with 10% of batch-tested non-stimulatory foetal bovine serum (Gibco™, Invitrogen), 2 mM glutamine (Gibco™, Invitrogen), 100 U/ml penicillin (Gibco™, Invitrogen) and 100 μg/ml streptomycin (Gibco™, Invitrogen). Cell viability was estimated according to the trypan blue exclusion criteria and was higher than 90%.
Mitogen assay
Cells (1.5 × 106 cells/ml) were settled at a final volume of 0.2 ml in 96-well flat-bottomed microtitre plates (Nunc™) in triplicate aliquots. The T-cell-selective mitogen concanavalin A (Con A; Sigma Chemical Co.) and the mitogen selective for B cells, lipopolysaccharide (LPS), were used. Mitogens were added to the microcultures and dose–response curves were performed to determine optimal mitogen concentrations. Cells were cultured at 37°C in a 5% CO2 atmosphere for different periods. Cells stimulated with the mitogens displayed the expected kinetics of proliferation with a peak on day 3 of culture (Cazaux et al. Citation1995). Proliferation was measured by adding 0.75 μCi [3H]-thymidine ([methyl-3H]-thymidine, specific activity: 20 Ci/mmol, NEN, Perkin-Elmer, Waltham, MA, USA) for the last 18-h period of culture. Thymidine incorporation was measured by scintillation counting after retention over GF/C glass-fibre filters (Whatman International Ltd, Kent, UK) of the acid-insoluble macromolecular fraction. The mean of triplicate determinations was calculated for each lectin concentration. The disintegrations per minute (d.p.m.) in basal cultures was subtracted from d.p.m. in stimulated cultures.
Hormone determinations
Trunk blood (500 μl) was collected after decapitation onto ice with 0.1 mol/l ethylenediaminetetraacetic acid (EDTA), and plasma was separated in a refrigerated centrifuge and stored at − 70°C. To avoid diurnal hormonal fluctuations, for plasma corticosterone determination, mice were bled at 12:00 h, and for insulin measurement, samples were obtained at 11:00 h in mice after 3 h of food deprivation. Corticosterone and insulin concentrations were determined by radioimmunoassay using commercial kits (MP Biomedicals, Solon, OH, USA and LINCO Research, Inc., Saint Charles, MO, USA, respectively). For plasma concentrations of corticosterone, the standard curve ranged between 1 and 250 mg/dl, and intra- and inter-assay coefficients of variation (CVs) were 5.4 ± 1.7 and 8.9 ± 0.7%, respectively. For plasma concentrations of insulin, the standard curve ranged between 0.1 and 10 ng/ml, and intra- and inter-assay CVs were 2.9 ± 1.5 and 9.0 ± 0.5%, respectively.
Noradrenaline and adrenaline (combined as catecholamines throughout the paper) concentrations were determined by fluorometric assays (Silberman et al. Citation2003). Briefly, spleens were homogenized in 12.5% sodium sulphite and 10% EDTA in 0.4 N perchloric acid. Homogenates were centrifuged, and supernatants were seeded in alumina columns. Eluates were oxidized with iodine in alkaline medium, and the fluorescence was recorded at 325/375 nm.
Statistical analysis
For survival analysis, Kaplan–Meier curves were constructed and compared using logrank tests. Group differences were tested by unpaired one-way analysis of variance (ANOVA) or by two-way ANOVA with treatment and time as main factors. When interaction was significant, simple effects analysis was made. Post hoc comparisons were applied to compare the data between the groups with the Student–Newman–Keuls (SNK) test. Pearson correlations were used to determine the relationships between glucose, corticosterone or catecholamines and lymphocyte reactivity. All tests were two-sided, and a p-value < 0.05 was considered statistically significant. Statistical and data analyses were performed using prism GraphPad Prism version 5.00 for Windows, GraphPad Software, San Diego, CA, USA, for the survival and correlation analysis, and Infostat Software, Cordoba University, Argentina, for the ANOVA.
Results
Animal survival
To determine the influence of stress and diabetes in the survival of the mice, the Kaplan–Meier plots were compared with logrank analysis; there were significant differences between curves (χ2 = 20.09, p = 0.0002). Mice with diabetes displayed a non-significant decrease in survival with respect to control mice (χ2 = 2.945, not significant). Stressed mice alone showed a survival similar to the control mice (χ2 = 0.8143, not significant); however, mice with diabetes exposed to CMS displayed a significant reduction of survival (vs. control mice: χ2 = 16.81, p < 0.0001; vs. diabetic mice: χ2 = 12.55, p = 0.0004), indicating a worse prognosis when CMS was combined with diabetes (, panel A).
Figure 1 Effect of stress and diabetes on survival and glycaemia in mice. (A) Survival is expressed as percent of survival against days of CMS. (B) Average glycaemia versus weeks of CMS. Data shown represent the mean ± SEM of 20 mice per group tested in two independent experiments performed with 10 mice in each group: CONTROL (mice without treatment), CMS (mice subjected to CMS), DIAB (diabetic mice) and DIAB+CMS (diabetic mice subjected to CMS). Statistical significance was determined with two-way repeated measures ANOVA with a 4 × 9 design followed by SNK post test. ***p < 0.001 vs. control mice, ****p < 0.0001 vs. control mice, # #p < 0.05 vs. diabetic mice and ###p < 0.001 vs. diabetic mice.
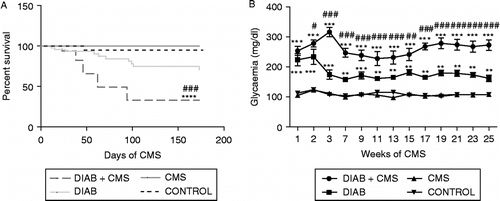
Glucose determination
Hyperglycaemia has been identified as the main factor contributing, either through direct or indirect mechanisms, to the diabetic pathogenesis, by producing biochemical and metabolic alterations that lead to both functional and structural alterations. Therefore, we evaluated whether hyperglycaemia could constitute one of the biological markers to correlate with poor prognosis in mice subjected to CMS. As shown in , panel B, plasma glucose concentrations did not increase in normal mice under CMS. As expected, diabetic mice displayed a significant increase in blood glucose concentrations. Additionally, when mice with diabetes were subjected to CMS, significantly higher glucose concentrations were observed with respect to non-stressed diabetic mice (two-way ANOVA paired by time; treatment F(3, 572) = 99.55, p < 0.0001; interaction treatment × weeks F(24, 572) = 3.659, p < 0.0001).
Lymphocyte reactivity to T- and B-cell mitogens
To investigate lymphocyte reactivity, the mitogen-induced T- and B-cell proliferation was analysed. Due to the well-known lymphoid tissue profiles, cell suspensions from lymph nodes were used for the T-selective mitogen Con A stimulation, while spleen lymphocyte suspensions were used for evaluating LPS (B-cell selective) mitogen effects. The maximal proliferation was reached at 1 μg/ml for Con A and 25 μg/ml of LPS for the four experimental groups at all times tested (data not shown). Results are shown in . Unstressed mice with diabetes did not show a significant difference from controls in the proliferative responses at any time. However, it is important to note that, as previously described (Rubinstein et al. Citation2008), the maximal mitogen-induced lymphocyte proliferation for both T and B cells was decreased in diabetic mice after 6 months of diabetes induction (data not shown). Also, after 5 weeks of CMS exposure, a diminution in T-cell proliferative response was observed as well as an increase in B-cell response. Interestingly, mice with diabetes under CMS showed a marked significant decrease in both T- and B-cell proliferation after 3 and 6 weeks of diabetes induction, or with 2 and 5 weeks under stress exposure, respectively; this effect was maintained throughout the experiment, that is, for 9 weeks (unpaired two-way ANOVA, T proliferative response—main effects: interaction treatment × weeks F(6, 48) = 6.24, p = 0.0001; simple effects analysis: 2nd week, F(3, 48) = 46.84, p < 0.0001; 5th week F(3, 48) = 29.24, p < 0.0001; 9th week F(3, 48) = 35.46, p < 0.0001; unpaired two-way ANOVA, B proliferative response—main effects: interaction treatment × weeks F(6, 24) = 26.26, p < 0.0001; simple effects analysis: 2nd week F(3, 24) = 15.92, p < 0.0001; 5th week F(3, 24) = 156.38, p < 0.0001; 9th week F(3, 24) = 168.41, p < 0.0001).
Figure 2 Mitogen-induced proliferative response in T and B cells. Lymphocytes were obtained from CONTROL (mice without treatment), CMS (mice subjected to CMS), DIAB (diabetic mice) and DIAB+CMS (diabetic mice subjected to CMS) groups at different times of CMS (2, 5 and 9 weeks). The cells were stimulated with optimal mitogen concentration of Con A (1 μg/ml, panel A) and of LPS (25 μg/ml, panel B). Data are the mean ± SEM of three independent experiments performed in triplicate (n = 5 mice for T and n = 3 mice for B proliferation assay). Statistical significance was determined with two-way ANOVA with a 4 × 3 design followed by SNK post test. **p < 0.01 vs. control and ###p < 0.01 vs. diabetic mice.
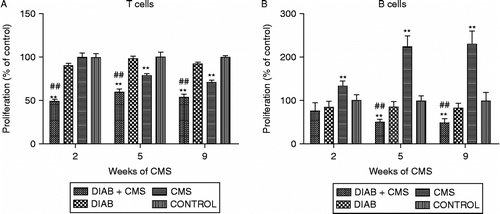
Evaluation of corticosterone and catecholamines
To investigate if the early activation of the HPA axis and SNS was involved in the worse evolution of diabetes, including the altered lymphocyte reactivity, in chronically stressed diabetic mice, both plasma corticosterone levels and splenic catecholamine content were determined.
Results shown in indicate that normal mice subjected to CMS show an increase in stress mediator levels during the first 3 weeks of CMS exposure, returning later to basal values as previously described (Silberman et al. Citation2003). In contrast, diabetic mice showed higher levels of corticosterone than controls at these times. Nevertheless, with CMS, the diabetic mice showed significantly lower corticosterone concentrations than diabetic mice not subjected to stress (; unpaired two-way ANOVA, main effect treatment: F(3, 84) = 24.01, p < 0.0001). Regarding catecholamines concentrations, there were no differences in diabetic mice with respect to controls, but a significant increase in diabetic mice under CMS was found (; unpaired two-way ANOVA, main effect treatment: F(3, 32) = 6.18, p = 0.002). These differences for both corticosterone and catecholamine concentrations were maintained throughout the duration of the experiment (data not shown).
Figure 3 Determination of corticosterone and catecholamines. Plasma and spleens from CONTROL (mice without treatment), CMS (mice subjected to CMS), DIAB (diabetic mice) and DIAB+CMS (diabetic mice subjected to CMS) were collected at different times of CMS (1–3 weeks), and plasma corticosterone (panel A) and splenic catecholamines (panel B) levels were determined. Data are mean ± SEM of at least eight and four mice in each group and each time for corticosterone and catecholamines, respectively. Statistical significance was determined with two-way ANOVA with a 4 × 3 design followed by SNK post test. *p < 0.05 vs. control values, **p < 0.01 vs. control values and ##p < 0.01 vs. diabetic mice.
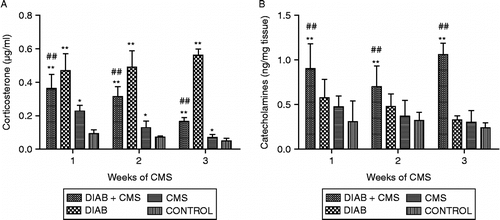
Correlations between blood glucose, corticosterone or catecholamines and lymphocyte reactivity
To analyse if the effect of stress on T- and B-cell reactivity observed in mice with diabetes was associated with changes in glucose, corticosterone or catecholamine levels, the correlation coefficient was calculated. As can be seen in , only a significant, and negative, correlation between T and B proliferative responses and glucose concentrations was observed (T proliferative response r = − 0.5754, p < 0.0001; B proliferative response r = − 0.5096, p = 0.0217).
Figure 4 Correlations between glycaemia and proliferation of T cells (panel A) and B cells (panel B) from CONTROL (mice without treatment), CMS (mice subjected to CMS), DIAB (diabetic mice) and DIAB+CMS (diabetic mice subjected to CMS) for 2, 5 and 9 weeks of CMS for T-cell proliferation and 5 and 9 weeks for B-cell proliferation. Negative correlations were significant: T cells, p < 0.0001; B cells, p = 0.022.
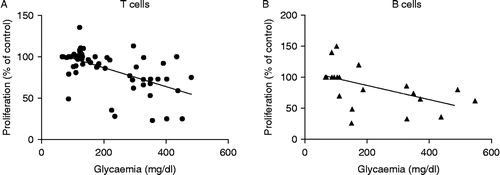
Correlation between corticosterone, catecholamines or insulin and glucose concentrations
Given that in normal mice, glucose blood concentrations are regulated by the secretion of insulin, insulin levels were determined in the four groups of mice. As can be seen in , plasma insulin concentrations were decreased in diabetic mice. However, stress exposure did not modify insulin levels either in control or in diabetic mice (one-way ANOVA F(3, 36) = 4.813, p = 0.0039). To ascertain if the increment in glycaemia observed in stressed diabetic mice could be associated to corticosterone or catecholamine changes, the correlation coefficient was determined. The results shown in indicate that glucose levels are positively correlated with changes in catecholamine levels in diabetic mice (r = 0.4432, p = 0.0342). Neither negative nor positive correlation was observed between glucose and corticosterone levels (r = 0.3207, not significant).
Figure 5 Correlation between insulin, catecholamine and glycaemia. (A) Plasma from CONTROL (mice without treatment), CMS (mice subjected to CMS), DIAB (diabetic mice) and DIAB+CMS (diabetic mice subjected to CMS) were collected on the 3rd week of CMS and plasma insulin concentrations determined. Each point represents one mouse, and the line represents the group mean (n = 14 per group). Statistical significance was determined with one-way ANOVA followed by SNK post test. *p < 0.05 vs. control values. (B) Positive correlation between splenic catecholamines and glycaemia is shown only for diabetic mice. p = 0.034.
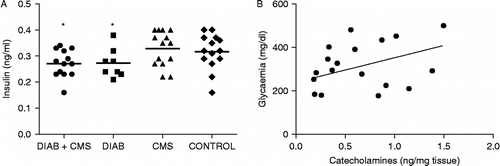
Discussion
Here, we show that chronic stress exposure of diabetic mice results in a significant reduction of survival and a greater sustained increase in blood glucose values than diabetes alone. However, stress did not increase blood glucose levels in normal mice as was previously reported (Harizi et al. 2007).
Concerning the immune system, no significant difference in mitogen-induced T- and B-cell proliferation was observed in diabetic mice at the time tested. However, mice with diabetes under chronic stress showed a marked decrease in both T- and B-cell proliferation after 2 and 5 weeks of stress exposure, respectively. According to our previous results (Silberman et al. Citation2002), exposure of control mice to stress significantly impaired T-cell proliferation but increased B-cell proliferation. These results suggest that stress has a different effect on the immune cell response in mice with diabetes compared to the controls.
Classically, it is considered that activation of both the HPA axis and the SNS plays a key role in the response to psychological stress (Munck and Guyre Citation1991; Elenkov et al. Citation2000), inducing an immunosuppression. We evaluated HPA activation by measuring plasma corticosterone levels and SNS activation by determining catecholamine levels in spleen, which received an important sympathetic innervation (Hori et al. Citation1995; Kim et al. Citation2004). In control mice, an increase in both corticosterone and catecholamines concentrations during the first 3 weeks of stress exposure was observed. However, inhibition of the T-cell response was observed after 5 weeks of exposure to stress. In addition, in diabetic mice, corticosterone levels in stressed mice were reduced with respect to non-stressed mice. Although a more detailed assessment of the HPA axis is needed, the present results indicate no correlation between corticosterone levels and changes in the immune response. However, it is important to note that a marked decrease in corticosterone-binding globulin (CBG) and unaffected total corticosterone concentrations were determined in loser males subjected to chronic social stress (Stefanski Citation2000). Future experiments must be conducted to determine whether in our model there is a decrease in CBG, hence freeing a greater amount of active corticosterone. Concerning catecholamines, splenic levels increased in diabetic mice under stress with respect to non-stressed diabetic mice, but there was no correlation between catecholamines levels and the lymphocyte proliferative response. However, a significant correlation was found between glucose levels and proliferative responses of both T and B cells. In a previous study (Rubinstein et al. Citation2008), we evaluated the influence of hyperglycaemia over the immune response in vitro. We observed that high glucose concentration diminished cell viability, increased apoptosis and induced oxidative stress in lymphocytes, indicating that high glucose concentrations can directly affect lymphoid cell growth (Rubinstein et al. Citation2008). Taking into account these results, it is possible to postulate that the increase in blood glucose levels induced by stress exposure could constitute an important factor that contributes to the marked decrease in the immune response.
Concerning the stress effect on blood glucose levels in diabetic mice, we investigated the participation of insulin and stress mediators in this phenomenon. It is known that insulin is the leading regulator of glucose metabolism. As expected, insulin levels were decreased in diabetic mice, but levels were not lower after stress exposure. Therefore, insulin does not seem to be the main factor involved in the stress-induced greater hyperglycaemia. However, stress hormones are referred to as “counter regulatory” because they generally show a hyperglycaemic effect. Glucocorticoids cause enhanced glucose production by the liver and diminished cellular glucose uptake. Catecholamines also play a role in the regulation of glucose metabolism (Nonogaki Citation2000). Bellush and Rowland (Citation1989) found that rats made diabetic with STZ had reliably higher norepinephrine levels than controls both before and after footshock training. Lee et al. (Citation1989) found similar results in diabetic rats subjected to acute stress. Our data indicated that there is a positive correlation between blood glucose and splenic catecholamine concentrations in diabetic mice but not in controls. These findings suggest that the increase in blood glucose values in diabetic mice under stress may depend on the level of catecholamines released during stressors exposure.
We have previously shown that glucose effects on immune responses are time and concentration dependent (Rubinstein et al. Citation2008). Stress, through an increase of glycaemia, anticipates similar deleterious effects on immune responses that occur in diabetic mice after a longer time. Normal mice show a different response to stress, which affects T-cell proliferation in the absence of hyperglycaemia. Finally, the present report shows, for the first time, that diabetic mice show a worse performance in immune function after stress exposure. Hyperglycaemia, and not increased corticosterone or catecholamines, may underlie these impaired immune cell responses. Nonetheless, increased catecholamines may contribute to hyperglycaemia in diabetes mellitus. These results point to the importance of considering life stress as a risk factor for increased susceptibility to infections suffered by patients with diabetes.
Acknowledgements
The authors thank Maria Rosa Gonzalez Murano for her technical assistance, Daniel Gonzalez for his invaluable help in the animal stress model and Mrs Noemí Capano for secretarial assistance. We thank LifeScan Argentina for supply of One Touch Ultra strips for the glucometer. This work was supported by grants from CONICET (PIP 6049 and 00281), UBACYT and Alberto J. Roemmers Foundation.
Declaration of interest: The authors report no conflicts of interest. The authors alone are responsible for the content and writing of the paper.
References
- Bellush LL, Rowland NE. 1989. Stress and behavior in streptozotocin diabetic rats: Biochemical correlates of passive avoidance learning. Behav Neurosci. 103 1: 144–150.
- Bertoni AG, Saydah S, Brancati FL. 2001. Diabetes and the risk of infection-related mortality in the US. Diabetes Care. 24:1044–1049.
- Butler SO, Btaiche IF, Alaniz C. 2005. Relationship between hyperglycaemia and infection in critically ill patients. Pharmacotherapy. 25:963–976.
- Cazaux CA, Sterin-Borda L, Gorelik G, Cremaschi GA. 1995. Down-regulation of beta-adrenergic receptors induced by mitogen activation of intracellular signaling events in lymphocytes. FEBS Lett. 364 2: 120–124.
- Christiansen C, Toft P, Jorgensen HS, Andersen SK, Tonnesen E. 2004. Hyperglycaemia and mortality in critically ill patients. A prospective study. Intensive Care Med. 30:1685–1688.
- Cox DJ, Gonder-Frederick L. 1992. Major developments in behavioral diabetes research. J Consult Clin Psychol. 60:628–638.
- Elenkov IJ, Wilder RL, Chrousos GP, Vizi ES. 2000. The sympathetic nerve—An integrative interface between two supersystem: The brain and the immune system. Pharmacol Rev. 52:595–638.
- Harizi H, Homo-Delarche F, Amrani A, Coulaud J, Mormède P. 2007. Marked genetic differences in the regulation of blood glucose under immune and restraint stress in mice reveals a wide range of corticosensitivity. J Neuroimmunol. 189:59–68.
- Hori T, Katafuchi T, Take S, Shimizu N, Niijima A. 1995. The autonomic nervous system as a communication channel between the brain and the immune system. Neuroimmunomodulation. 2 4: 203–215.
- Joshi N, Caputo GM, Weitekamp MR, Karchmer AW. 1999. Infections in patients with diabetes mellitus. N Engl J Med. 341 25: 1906–1912.
- Kim D, Seegal RF, Lawrence DA. 2004. Splenic norepinephrine and serum corticosterone level fluctuations associated with bacteria-induced stress. Neuroimmunomodulation. 11 5: 323–331.
- King H, Gruber W, Lander T. 1995. Implementing national diabetes programmes report of a WHO meeting. Geneva: World Health Organization Division of Non-communicable Diseases.
- King H, Aubert RE, Herman WH. 1998. Global burden of diabetes, 1995–2025: Prevalence, numerical estimates, and projections. Diabetes Care. 21:1414–1431.
- Lee JH, Konorska M, McCarty R. 1989. Physiological responses to acute stress in alloxan and streptozotocin diabetic rats. Physiol Behav. 45:483–489.
- Leiter EH, Le PH, Prochazka M, Worthen SM, Huppi K. 1988. Genetic and environmental control of diabetes induction by multi-dose streptozotocin in two BALB/c substrains. Diabetes Res. 9:5–10.
- Like AA, Rossini AA. 1976. Streptozotocin-induced pancreatic insulitis: New model of diabetes mellitus. Science. 193:415–417.
- McMahon MM, Bistrian BR. 1995. Host defenses and susceptibility to infection in patients with diabetes mellitus. Infect Dis Clin North Am. 9:1–9.
- Monleon S, D'Aquila P, Parra A, Simon VM, Brain PF, Willner P. 1995. Attenuation of sucrose consumption in mice by chronic mild stress and its restoration by imipramine. Psychopharmacology. 117:453–457.
- Munck A, Guyre PM. 1991. Glucocorticoids and immune function. In: Ader R, Felten DL, Cohen N, editors. Psychoneuroimmunology. Rochester: Academic Press. 447–475.
- Nonogaki K. 2000. New insights into sympathetic regulation of glucose and fat metabolism. Diabetologia. 43:533–549.
- Palumbo ML, Fosser NS, Rios H, Zorrilla Zubilete MA, Guelman LR, Cremaschi GA, Genaro AM. 2007. Loss of hippocampal neuronal nitric oxide synthase contributes to the stress-related deficit in learning and memory. J Neurochem. 102:261–274.
- Peleg AY, Weerarathna T, McCarthy JS, Davis TM. 2007. Common infections in diabetes: Pathogenesis, management and relationship to glycaemic control. Diabetes Metab Res Rev. 23:3–13.
- Rubinstein R, Genaro AM, Motta A, Cremaschi G, Wald MR. 2008. Impaired immune responses in streptozotocin-induced type I diabetes in mice. Involvement of high glucose. Clin Exp Immnunol. 154:235–246.
- Silberman DM, Wald M, Genaro AM. 2002. Effects of chronic mild stress on lymphocyte proliferative response. Participation of serum thyroid hormones and corticosterone. Int Immunopharmacol. 2:487–497.
- Silberman DM, Wald MR, Genaro AM. 2003. Acute and chronic stress exert opposing effects on antibody responses associated with changes in stress hormone regulation of T-lymphocyte reactivity. J Neuroimmunol. 144:53–60.
- Stefanski V. 2000. Social stress in laboratory rats: Hormonal responses and immune cell distribution. Psychoneuroendocrinology. 25 4: 389–406.
- Willner P, Muscat R, Papp M. 1992. Chronic mild stress-induced anhedonia: A realistic animal model of depression. Neurosci Biobehav Rev. 16:525–534.