Abstract
In the present investigation, testosterone (T) was evaluated as a targeting ligand to direct the site-specific delivery of 5-Fluorouracil (5-FU) bearing liposomes to the androgen receptor (ARs) positive tumors and other organs like prostate, brain, and testis. The testosterone was conjugated with the distearoyl phosphatidyl ethanolamine (DSPE) and then this lipid conjugate, Testosterone-DSPE (T-DSPE) was used as one of the components of the liposome. The liposomes were prepared by cast film method using T-DSPE, egg PC, and cholesterol. Further these liposomes were characterized for vesicle shape, average size, polydispersity index, drug entrapment, and in vitro drug release. It was observed that the prepared liposomes were spherical in shape with an average size of 232 ± 21 nm and 0.181 ± 0.064 polydispersity index. The in vitro drug release study showed 79.50 ± 2.81 percent drug release in 24 h. In vivo performance of the developed liposomes was evaluated using organ distribution study in male albino rats. Moreover the fluorescent microscopy was also performed using 6-Carboxyfluorescein (6-CF) as a fluorescent marker. The organ distribution and fluorescent uptake studies confirm that T-DSPE coupled liposomes were effectively taken up by various ARs expressing tissues. Thus, it may be concluded that the testosterone may be used as an effective ligand for the site-specific delivery of anti-cancer agents to various ARs positive carcinomas.
Introduction
Prostate cancer may be hormone responsive or non-responsive in nature. Hormonal therapy is used for the reduction of serum androgen level in hormone responsive prostate cancer results in inhibition of the androgen receptors (ARs) in prostate cancer cells (CitationDenis & Grifiths, 2000). However, in androgen irresponsive prostate cancer the hormonal therapy fails and cancer progresses to a hormone refractory state. Other alternate approaches such as radiation therapy and surgical removal of the diseased organ may have severe side-effects including the possibility of impotence, incontinence, or both. In these conditions chemotherapy remains the treatment of choice.
5-FU is one of the chemotherapeutic agents used in prostate cancers. It interferes with the nucleic acid synthesis and eventually inhibits the cell growth due to its structural resemblance with natural pyrimidines (CitationLangenbach et al., 1972; CitationParker & Cheng, 1990). The oral bioavailability of the 5-FU is incomplete and erratic, therefore it is administered as an intravenous bolus injection or continuous infusion modulated with folinic acid (leucovorin) (CitationHahn et al., 1975; CitationVan et al., 1999; CitationLabianca et al., 2001). After intravenous administration, 5-FU may cause severe systemic toxic effects including gastrointestinal, hematological, neural, cardiac, and dermatological when the drug reached the various superfluous sites (CitationDiasio & Harris, 1989). One more limitation associated with this agent is its hydrophillicity, as it remains in plasma for a very short time duration, thus repeated IV bolus injection is required for effective plasma drug concentration.
Site-specific delivery of various chemotherapeutic agents using nanocarriers may provide safe and effective means for cancer chemotherapy and also may reduce the systemic side-effects associated with these agents. The strategy may be instrumental to increase the accumulation of the drug in diseased organs due to enhanced permeability and retention effect (CitationMaeda et al., 2000). After administration of chemotherapeutic agent encapsulated in liposomes the pharmacokinetic behavior of the drug is replaced with that of liposomes. This may result in higher chemotherapeutic potential of cytotoxic agents (CitationGabizon et al., 1990). The liposomes may also be used to achieve active or passive targeted drug delivery as its surface membrane may be modified easily using various cell-specific targeted ligands (CitationSchuber, 1995).
The cancer chemotherapy via ligand receptor targeting is a potential approach for enhancing the therapeutic potential of several anti-neoplastic agents including 5-FU (CitationGupta et al., 2007). Various receptors which are over-expressed at tumor cells are epidermal growth factor receptor (EGFR) (CitationTorp et al., 1991; CitationRusch et al., 1996), folate receptor (FRs) (CitationWeitman et al., 1992), transferrin receptor (TRs) (CitationRecht et al., 1990), fucose receptor CitationSeymour, 1994), estrogen receptors (ERs) (CitationHarding et al., 1990; CitationLoven et al., 1990), and androgen receptor (ARs) (CitationKampa et al., 2002; CitationDas et al., 2006), etc. These ligands have been proposed for site-specific delivery of different imaging agents or therapeutic agents via conjugated drug delivery. Being endogenous in nature, steroid and peptide ligands may have the advantage of higher affinity to the respective receptors as compared to synthetic ligands. Steroidal ligands have been investigated for toxin/gene/imaging agents in different steroid receptors positive carcinomas related to the endocrine cancer. The receptor-specific and selective steroidal ligands are trafficked to the target site where the receptors for these ligands are present or over-expressed, especially in the case of endocrinal cancer (CitationHager et al., 1998).
Nuclear steroidal receptors are promising pharmacological targets as they bind with small lipophillic molecules and may be modified by drug design. SRs diffuse easily via cell membranes and thus their lipophillic conjugates may also diffuse via cell membrane and result in the cytosolic or nuclear delivery of the drug conjugates or carriers. Steroidal ligands, especially androgen (CitationBrix et al., 1990), estrogen (CitationSwamy et al., 2002), Vitamin D3 (CitationMolnar et al., 2004), and corticoids (CitationKhandare et al., 2005), etc. have been investigated as bioconjugates showing sufficient selectivity towards SRs positive tumor. Sex steroids receptors have also been evaluated for the same purposes in a number of studies (CitationHoffmann & Sommer, 2005; CitationLeuschner & Hansel, 2005). Targeted delivery of different chemotherapeutic or imaging agent in these SRs positive cancer can be of vital significance over other therapies. Recently, liposomes have also been implicated for the site-specific gene/drug delivery using estrogen as a targeting ligand (CitationReddy & Banerjee, 2005; CitationRai et a., 2008). ARs are the members of nucleic receptor family which are over-expressed at the tumor surface in various cancer cells like DU-145 (CitationDas et al., 2006), and LNCaP cells (CitationKampa et al., 2002; CitationKidwai et al., 2004). Testosterone, a primary male sex hormone ligand, regulates the sexual and other regulatory function in male. Various conjugates of testosterone have been evaluated for immunological assay, ARs imaging/targeting purposes as these receptors are over-expressed at the tumor surface (CitationKatzenellenbogen et al., 1995; CitationKuduk et al., 2000; CitationHoffmann & Sommer, 2005; CitationDas et al., 2006). Thus, the androgen receptor-specific testosterone lipid conjugate (T-DSPE) present at the liposomes membrane may direct the drug delivery to the ARs target organs and ARs over-expressed tumors.
In the present approach we have prepared testosterone conjugated lipid (T-DSPE) as a constituent of a liposome membrane to direct the liposomes encapsulated 5-FU to the ARs positive organs. Thus, the T-DSPE coupled liposomes carrying 5-FU may result in site-specific drug release at the target organs, simultaneously avoiding systemic adverse effect related to the agent.
Materials and method
Materials
L-α Egg phosphatidylcholine (PC) and distearoyl phosphatidylethanolamine (DSPE) were purchased from Avanti Polar Lipids (Alabaster, AL). Testosterone, carboxy methoxylamine hemihydrochloride, Dicyclohexylcarbodiimide (DCC), Sephadex G-50, Triton X-100, triethylamine (TEA), and 6-carboxyfluorescein (6-CF) were purchased from Sigma Chemical Co. (St. Louis, MO). 5-flurouracil was provided as gift sample by M/s Khandelwal Labs. (Mumbai, India). All other reagents and solvents unless specified were either of analytical or HPLC grade.
Preparation of testosterone-coupled (T-DSPE) liposomes
The testosterone-coupled liposomes were prepared in two steps, i.e. synthesis of testosterone coupled lipid (T-DSPE) and preparation of T-DSPE coupled liposomes.
Synthesis of testosterone lipid conjugate (T-DSPE)
First of all testosterone was modified for the attachment with lipid (DSPE) at the C-3 carbonyl group and not at C-17 hydroxyl group of the steroid which is necessary for hormone ligand receptor binding. The testosterone was coupled with DSPE in two steps using the methods reported by CitationDas et al. (2006) and CitationHwang et al. (1992), with slight modifications. Briefly, 25 mg (0.087 mmol) of testosterone and 45 mg (0.413 mmol) carboxy methoxylamine hemihydrochloride were dissolved in 2.1 ml mixture of pyridine/methanol/water (1:4:1 v/v). The solution was refluxed for 2 h, stirred overnight at room temperature, and the solvent was removed with the help of a vacuum dryer. The resulting powdered mass, i.e. carboxy derivative of testosterone (18.1 mg, 0.05 mmol) was further reacted with n-hydroxyl succinimide (0.15 g, 1.3 mmol) in the presence of dicyclohexyl carbodimide (0.268 g, 1.3 mmol) in dimethyl formamide (DMF). The reaction was carried out at 4°C for 18 h which resulted in active ester derivative. The ester derivative of testosterone-3-(-O-carboxy methoxyl) amine was subsequently reacted with DSPE to form the testosterone-lipid (T-DSPE) conjugate. In this step, the solution of DSPE (0.239 g, 0.32 mmol) in chloroform and ethyl amine (30:1) was added drop-wise in the flask containing testosterone-3-(-O-carboxy methoxyl) amine with continuous stirring. The mixture was stirred at 40–50°C in nitrogen stream for 72 h. IR (Perkin Elmer 783 Spectrophotometer) and mass spectroscopic (Micromass Quattro II triple quadrupole mass spectrometer) methods were used for the confirmation of formation of T-DSPE conjugate. IR spectroscopy was carried out using KBr pellet method after adsorption of a small amount of conjugate on KBr. The mass spectroscopy was carried out by introduction of samples dissolved in methanol into the ESI source through a syringe pump at the rate of 5 μl/min. The ESI capillary was set at 3.5 KV and the cone voltage was 40 V. The spectra were collected in 6S scans and the printouts are averaged spectra of six-to-eight scans. Spectra recorded at high mass unit are computerized decovoluted. The synthesis of T-DSPE is shown in .
Preparation of liposomes
The 5-FU bearing liposomes were prepared using cast film hydration method with slight modification (CitationBangham et al., 1965). T-DSPE lipid conjugate was added in certain extent in egg PC (the molar ratio of egg PC:cholesterol:T-DSPE was taken as 7:3:1.0). Briefly egg PC, T-DSPE, and cholesterol were dissolved in minimum quantity of chloroform and methanol mixture (4:1) in a round bottom flask. A thin film of the lipids was casted at the inner surface of the round bottom flask by evaporating the solvent under reduced pressure using a flash rotary evaporator (Stereoglass Rotavap, Italy). The flask was rotated continuously under nitrogen atmosphere until the film was dried. Final traces of solvents were removed under vacuum and dried overnight. The dried lipid film was hydrated with 10 ml of PBS (pH 7.4) having 5-FU (50 mg) followed by continuous vortexing of the flask for ∼ 1 h to get MLV. Liposomal suspension was allowed to stand for a further 3–4 h in the dark at room temperature to allow complete swelling of the vesicles and then the liposomal suspension was sonicated for 5 min at 4 ± 1°C using a probe sonicator (Lark Innovative Technology India Ltd, Chennai, India) to form smaller vesicles. The liposomal formulations were passed through the Sephadex G-50 column to remove unentrapped drug and vesicles were stored in the dark at cold temperature.
Characterization of the formulations
The T-DSPE coupled and plain liposomes were characterized for average size, shape, and polydispersity index, drug entrapment, and in vitro drug release.
Shape and size
The prepared liposomes were examined under Transmission Electron Microscopy (TEM analysis) (Philips Morgagni, Netherlands) for its shape. To prepare the sample for TEM study a drop of liposomes suspension was put on the copper grids which were coated with a solution of collodion and left for 15–20 min in contact with the copper grids. Finally grids were picked up, blotted with filter paper, left for drying for 3 min, and then observed under TEM (CitationManosroi et al., 2004). The TEM of the T-DSPE coupled liposomes is shown in . Photon correlation spectroscopy (Zetasizer, ZS 90, Malvern, UK) was used to determine the average vesicle size expressed as volumetric diameter for the plain and T-DSPE coupled liposomes ().
Table 1. Characterization of uncoupled and testosterone (T-DSPE) coupled liposome formulations.
Entrapment efficiency
Entrapment efficiency (%EE) was determined using the method reported by CitationFry et al. (1978), briefly, ∼ 0.2 ml of liposomes suspension was eluted with PBS (pH 7.4) through Sephadex G-50 column to separate unentrapped drug from liposomes. The liposomes suspension (undiluted) was applied drop-wise on the top of the gel bed in the center. Columns were centrifuged at 3000 rpm for 3 min to remove the unentrapped drug from liposomes suspension. Elute was removed and 0.25 ml saline was applied to each column, and centrifuged as previously. The collected liposomes were then disrupted using 0.1% TritonX-100 solution. The absorbance of sample was measured at 266.5 nm using a UV-spectrophotometer (Cintra-10, Japan). Entrapment efficiency was then calculated applying the following equation:
In vitro drug release
The in vitro drug release from liposomes free from unentrapped drug was studied using a dialysis bag (CitationGupta et al., 2005). One milliliter of liposomal suspension was taken into a dialysis bag (molecular cut-off point 3500, Sigma, MO) and placed in a beaker containing 20 ml of PBS (pH 7.4). The beaker was placed over a magnetic stirrer and the temperature of the assembly was maintained at 37 ± 1°C throughout the study. Samples were withdrawn at pre-determined time intervals and replaced with the same volume of PBS (pH 7.4). The withdrawn samples were assayed for drug content by measuring absorbance at 266.5 nm against a blank (PBS, pH 7.4) using a UV-Visible spectrophotometer (Cintra-10, Japan).
In vivo studies
Healthy male albino rats (wistar strain) of weight ∼ 130–150 g were selected for the in vivo qualitative (Fluorescence) and quantitative (Biodistribution) studies. The animals were kept at normal conditions for one week before starting the experiment, fed with Purina chops, and allowed water ad libitum. The in vivo studies were carried out as per the guidelines compiled by CPCSEA (Committee for the Purpose of Control and Supervision of Experiments on Animal, Ministry of Culture, Government of India). The Institutional Animal Ethics Committee (Dr H. S. Gour University, Sagar M. P., India) had approved the study.
Qualitative biodistribution study (fluorescence microscopy)
The qualitative biodistribution study was performed in male albino rats using 6- carboxyfluorescein (6-CF) as a fluorescent marker. Both the liposomes formulation encapsulating 6-CF were prepared using the same method as followed in the case of liposomes encapsulating 5-FU by replacing the 5-FU with 6-CF. Plain and T-DSPE-coupled liposomes encapsulating 6-CF were administered to the rats intravenously through tail vein. The rats were sacrificed after 1 h and different tissues were excised. These tissues were fixed in 10% formaldehyde saline solution. Dehydration of these tissues was done using 60% alcohol for 1 h, in 80% alcohol for 1.5 h, in 90% alcohol for 24 h, and then in absolute alcohol for 1 h. The tissues were transferred to the mixture of absolute alcohol:xylene (1:1) for 30 min, after which the tissues were transferred to xylene and kept for 30 min, and then this step was repeated again for the next 30 min before removing the alcohol from the tissues. The tissues were then transferred to molten matured wax in first infiltration pan kept in an incubator at 62–64°C for paraffin embedding. The same procedure was repeated twice. Then, the blocks were prepared using the lid of cuffling’s jar. Finally the microtomy was done with the help of microtome and ribbons of section obtained were fixed on slides using egg albumin solution as fixative. The sections were observed under fluorescent microscope (Nikon ES200). The fluorescent photomicrographs are shown in .
Figure 3. Fluorescent uptake after 1 h administration of 6-CF bearing uncoupled liposomes (alphabetical) and T-DSPE coupled liposomes (alphabetical artistic) in various androgen target organs. (A) Liver, (B) Prostate, (C) Testis, (D) Brain, (E) Spleen, (F) Kidney. The photographs clearly reveal that after administration of T-DSPE coupled liposomes the fluorescence of testosterone targeted organs is higher as compared to uncoupled liposomes, i.e. liver, prostate, testis, and brain, while non-target organs, i.e. spleen and kidney, show lesser fluorescence after administration of T-DSPE liposomes. It is clear that T-DSPE liposomes have better selectivity towards ARs targeted organs.
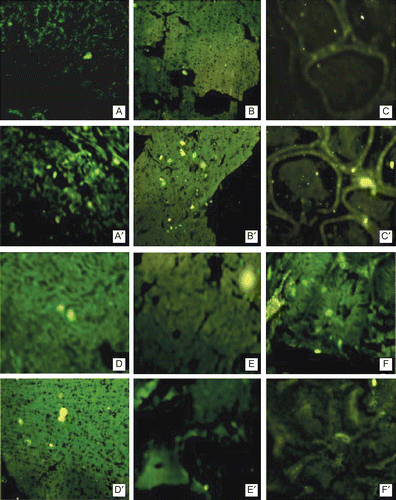
Estimation of 5-FU in blood and various tissues/biodistribution studies
Sixty male albino rats (wistar strain) of weight ∼ 130–150 g were divided into four groups of 15 rats each. The rats were fasted overnight before drug administration. The first group animals were given 5-FU drug solution in PBS (pH 7.4) intravenously at a dose equivalent to 5 mg drug/kg body weight through tail vein. The second and third group animals were given intravenous injection of plain liposomes and T-DSPE coupled liposomes, respectively at a dose equivalent to 5 mg drug/kg body weights in PBS (pH 7.4). The fourth group of animals acted as control and did not receive any drug or formulation. The animals (n = 3) from each group were sacrificed after a definite time period for the collection of visceral organs like spleen, liver, kidney, brain, prostate, and testis. The organs were excised and isolated. These organs were washed with ringer’s solution to remove any adhered debris and dried using tissue paper. These visceral organs were stored at −22°C until assay (CitationGupta et al., 2007). Different organs (spleen, liver, kidney, brain, prostate, and testis) were weighed separately and cut into small pieces. One gram of each organ was homogenized with 2 ml PBS (pH 7.4). In the case of organs weighing less than 1 g, the whole organ was used. Blood samples were collected by cardiac puncture. The blood and tissues were homogenized (Lark, India) in 0.8 ml of citric acid buffer (pH 6) for 3 min at a speed of 3000 rpm. The homogenate was transferred to a centrifuge tube containing 3.0 ml mixture of chloroform and methanol (1/4, v/v). The tubes were vortexed for 10 min and centrifuged at 3000 rpm for 10 min. After centrifugation, liquid was filtered through a 0.2 μm membrane filter and injected into an HPLC (Shimadzu LC-10, C-18 Column, Japan) column system. The detection wavelength was 266.5 nm, and the mobile phase consisted of HPLC-grade water. The flow rate was maintained at 0.8 ml/min, and the analytical column used was a reverse phase C18 column (4.6 × 250 mm, 5 μm) (CitationKrishnaiah et al., 2003).
Statistical analyses
Statistical analysis was performed for the data obtained by one-way analysis of variance (ANOVA) with Tukey–Kramer multiple comparisons post test using Graph Pad InStatTM software. Throughout, the level of significance chosen was less than 0.05 (i.e. p < 0.05).
Results and discussion
Ligand targeted liposomes provide a better opportunity for site-specific sustained drug release at the tumor tissues more precisely as compared to drug solution and/or plain liposomal formulation. Plain liposomes may display lower toxicity and sustained release characters but may have limited application as they may not provide desired concentration as the tumor sites. Testosterone is the endogenous ligand investigated for the imaging (CitationDas et al., 2006), targeted drug delivery (CitationBetsch, 1990; CitationRoth et al., 1995; CitationKuduk et al., 2000), or immunological study (CitationRajkowski et al., 1997), via various testosterone ligand conjugates, so far as androgen receptors are present at most of the prostate tumor surface/membrane, e.g. DU-145 and LNCaP, cells. Hence, in the present study, testosterone (T-DSPE) coupled liposomes bearing 5-FU were prepared which may possess selectivity towards the ARs over-expressed at tumor surface. T-DSPE lipid conjugate was synthesized and incorporated at the liposomal surface encapsulated with 5-FU. Thus, the object of the present study was to prepare 5-FU liposomes coupled with the endogenous ligand testosterone and assure the preferential uptake of the prepared liposomes in androgen receptor (ARs) target organs.
Preparation, characterization, and in vitro drug release
T-DSPE was synthesized in two steps using the procedure reported previously (CitationHwang et al., 1992; CitationDas et al., 2006), with slight modifications. 3-carbonyl group of Testosterone ligand was selected for the derivatization of carboxylic acid group in testosterone, as this position of the ligand can be modified to retain the significant binding properties of this testosterone conjugate towards ARs (CitationAutenrieth et al., 1981). The derivative viz. testosterone-3-(-O-carboxy methoxyl) amine was further modified for the formation of amide linkage in between the carboxylic acid group of testosterone-3-(-O-carboxy methoxyl) amine and primary amine group of DSPE. The carboxyl derivative of the testosterone and final lipid conjugate, i.e. T-DSPE conjugate, was characterized by IR and mass spectroscopic analysis for the structural confirmation. Different peaks in IR spectrum shows () the structural changes in testosterone and identification of new broad peaks viz. C=O absorption peak at 1737 cm−1 and no absorption at 1670–1640 cm−1 (conjugated C=O). Thus, middle compound showed the derivation of caroboxylic acid group in testosterone. The IR spectrum of the final lipid conjugate (T-DSPE) shows broad peak at 3430.32 cm−1 for OH, 3327.32 cm−1 of N-H stretching, 1573.97 cm−1 of primary amide bond, 1469.81 cm−1 of C-N stretching, and 663.53 cm−1 of out of plane NH wagging. The identification of the mass peak at 362 and 1094 in the mass spectrum shows that the molecular weight of the derivative were very close to the testosterone-3-(-O-carboxy methoxyl) amine and T-DSPE, respectively. These studies confirm the synthesis of T-DSPE conjugate. Plain liposomes and T-DSPE coupled liposomes were prepared by film hydration method. In order to obtain liposomes with a reduced mean size, the liposomes suspension was sonicated under an ice bath for 5 min using a probe sonicator. The sonication reduced the size of liposomes to the nanometric range and also allowed the formation of liposomes suspensions with a very narrow size distribution. These liposomal formulations were characterized for various attributes viz; size, shape, polydispersity index, entrapment efficiency, and in vitro drug release. The mean size of a colloidal drug carrier is an important requisite that influences the in vivo performance of delivery systems (CitationKarajgi et al., 1993). The TEM analysis of the prepared plain and T-DSPE coupled liposomes showed spherical and multilamellar nature of the liposomes (). The size of plain and T-DSPE coupled liposomes was found to be 188 ± 16 and 232 ± 21 nm, respectively. The polydispersity index of both the formulations was found to be 0.203 ± 0.043 and 0.181 ± 0.064 (). The polydispersity index (< 0.3 in both cases) for plain and T-DSPE coupled liposomes showed uniformity of preparation. Entrapment efficiency of 5-FU in both liposomal formulations was found to be 29.4 ± 1.34% and 27.3 ± 1.82% (). The entrapment of the 5-FU may be low in liposomes as its hydrophilic nature and small molecular weight. Thus, the entrapment in both cases was found to be > 27% ().
In vitro drug release profile of the drug was determined in PBS (pH 7.4) up to a period of 24 h from initial, i.e. zero, time. The release rate of the T-DSPE coupled liposomes was significantly lesser than plain liposomes. This might be due to coupling of T-DSPE at the liposomes surface which may create an additional barrier to drug diffusion from the liposomal surface. Thus, the targeted liposomal preparations may be more stable as compared to plain liposomes and exhibiting slow drug release. The cumulative percent drug release from plain and T-DSPE coupled liposomes was found to be 87.2 ± 3.54% and 79.50 ± 2.81%, respectively, in 24 h ().
In vivo studies
The efficiency of plain and T-DSPE coupled liposomes to target the ARs target organs was studied by replacing the drug with fluorescent marker (6-CF). As shown in , the T-DSPE coupled liposomes formulation showed much greater accumulation in androgen target organs, especially in prostate and brain, as compared to plain liposomes carrying 6-CF as a fluorescent marker. The study also showed lesser accumulation in non-target organs like kidney and spleen, which may increase the selectivity to the target organs as compared to non-targeted ones. The enhanced uptake of 6-CF by testosterone coupled (T-DSPE) liposomes could be due to higher interaction with the cellular ARs which are over-expressed at the membrane of the majority of prostate cancer cells, i.e. LNCaP and DU-145 cell lines (CitationKampa et al., 2002; CitationDas et al., 2006). Thus, the T-DSPE coupled liposomes may be more efficacious in various ARs positive carcinomas. In vivo organ distribution study of testosterone (T-DSPE) coupled liposomes formulation was conducted in male albino rats and the results were compared with plain liposomes and the free drug after intravenous administration via the tail vein. The studies show that the drug level in the blood after 1 h administration of free drug, plain liposomes, and T-DSPE coupled liposomes was 13.3 ± 1.12, 18.4 ± 1.43, and 16.8 ± 1.16 μg/ml, respectively. However, after 6 h of administration the blood drug level was reduced to 2.6 ± 0.23, 6.3 ± 1.32, and 10.8 ± 1.03 μg/ml, respectively, and after 24 h no drug was detected in blood in case of free drug; however, 3.4 ± 1.1 and 4.9 ± 1.23 μg/ml drug level was found with plain and T-DSPE coupled liposomes but no drug was detected after 8 h administration of free drug (). 5-FU blood level after administration of T-DSPE coupled liposomes and plain liposomes in the blood was found to be sufficiently higher than administration of free 5-FU for a longer time due to slow release of the drug from the liposomes with longer circulation while free drug administered, excreted out in a short period due to its hydrophilic nature and no release barrier. The blood drug level after administration of T-DSPE coupled liposomes was higher as compared to the plain liposomes. The possible reason for this may be the presence of endogenous ligand testosterone coupled to the DSPE lipid at the liposomal surface that may oppose the opsonization of T-DSPE coupled liposomes because of its endogenous nature. Drug recovered in different organs at various time intervals, as shown in . It may also be concluded that the drug was significantly accumulated in androgen targeted organs like prostate, testis, and brain tissues. In the case of prostate tissue 3.72 ± 0.24 μg/g 5-FU was recovered after administration of T-DSPE coupled liposomes as compared to 1.51 ± 0.22 μg/g and 2.68 ± 0.34 μg/g after administration of the equivalent dose of free drug solution and plain liposomes in 1 h, respectively. After 4 h the amount of the 5-FU in prostate tissue was 12.74 ± 0.34 μg/g when compared with 3.66 ± 0.72 μg/g of the plain liposomes and 2.14 ± 0.42 μg/g of the free drug. The higher accumulation of 5-FU to prostate tissue using T-DSPE coupled liposomes proves the selectivity to the ARs present at the target organs. Thus, cytotoxic drug delivery to prostate cancer using ARs-specific liposomal carriers could be a potential carrier for prostate cancer treatment. A significant amount of the drug (p < 0.05) was recovered even after 24 h using T-DSPE liposomes in various target organs ().
Figure 6. Plasma drug level after administration of 5-FU drug solution and various liposomal formulations (n = 3).
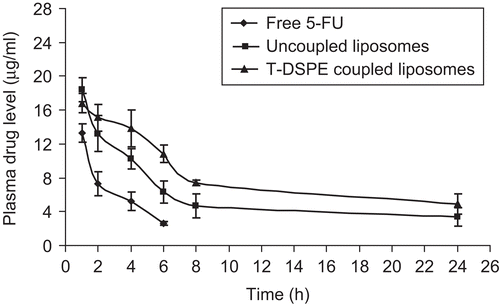
Table 2. Drug recovered in various organs after various time intervals.
Figure 7. Accumulation of 5-FU in prostate tissues after administration of 5-FU drug solution, 5-FU bearing uncoupled liposomes and T-DSPE coupled liposomes (n = 3).
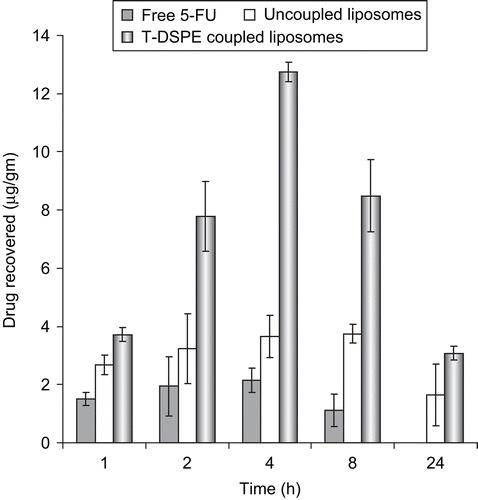
The accumulation of free 5-FU in non-target tissues can be attributed to the wide volume of distribution of free 5-fluorouracuil, which may be the cause of drug induced toxicity to the normal tissues and low drug level in the tumor. The accumulation of the drug in prostate tissue was found higher after administration of T-DSPE-coupled liposomes as compared to free drug and plain liposomes due to higher expression of androgen receptors in prostate tissues as compared to other tissues as these receptors having high selectivity to the testosterone ligand. The results are shown in . The findings of the biodistribution experiment indicate that when injected into the blood stream plain liposomes and T-DSPE coupled liposomes both can alter tissue distribution of 5-fluorouracuil. The concentration of 5-FU in liver was higher after administration of plain liposomes and T-DSPE-coupled liposomes than free drug at corresponding time points. This point indicates the passive targeting of both liposomal formulation in liver and also the presence of ARs which may further direct the T-DSPE liposomes towards liver. The lower concentration of plain and T-DSPE coupled liposomal 5-FU in the kidney and spleen tissues as compared to free 5-FU may provide a platform for lower toxicity of the drug to these tissues. Biodistribution study on prostate, testis, and brain revealed greater accumulation of 5-FU after administration of T-DSPE coupled liposomes as compared to plain liposomes and free 5-FU. The altered biodistribution of liposomes might be due to differential expression of androgen receptors for the coupled testosterone ligand over the liposomes membrane which may direct the T-DSPE coupled liposomes to these organs. These organs are testosterone responsive organs, and endogenous ligand testosterone might bind with the cognate receptors present over them. Difference in the concentration of 5-fluorouracuil in prostate, testis, and brain reflects the difference in the expression level of receptors at these tissues.
Conclusion
The use of targeting ligand for over-expressed tumor cell receptors is a widely accepted approach for selective delivery of therapeutic agents to these cells while sparing non-target healthy cells. Novel ARs targeted liposomes carrying 5-FU were developed and the formulation exhibited uniform shape and size with desired sustained release profile and shows the sufficient stability in biofluid for upto 24 h of administration. The formulations also retained their specificity towards the ARs. Thus, it can be concluded that ARs-targeted liposomes formulation of anti-neoplastic agents could be potentially useful for treatment of ARs positive tumors such as prostate or testicular cancer and the approach may open the new possibility for non-immunogenic, site-specific delivery of bioactive(s) at SRs positive carcinomas.
Acknowledgement
The authors are thankful to Khandelwal Labs. (Mumbai, India) for providing 5-FU as a gift sample, the sophisticated analytical instrument facility, AIIMS (New Delhi) for performing TEM, CDRI Lucknow for spectroscopic analysis and University grant commission (UGC) for providing financial assistance (JRF) to one of the authors (PKM).
Declaration of interest: The authors report no conflicts of interest. The authors alone are responsible for the content and writing of the paper.
References
- Autenrieth, D., Kan, D., Van Lier, J.E. (1981). Synthesis of 3- and. 6-substituted steroidal heterocycles as potential anticancer agents. Eur J Med Chem. 16:525–8.
- Bangham, A.D., Stansfield, M.M., Walkins, J.C. (1965). Diffusion of univalent ions across the lamellar of swollen phospholipids. J Mol Biol. 13:238–52.
- Betsch, B. (1990). The pharmacokinetic model and distribution pattern of new sexual-steroid-hormone-linked anticancer agents. J Cancer Res Clin Oncol. 116:467–9.
- Brix, H.P., Berger, M.R., Schneider, M.R., Tang, W.C., Eisenbrand, G. (1990). Androgen linked alkylating agents: biological activity in methylnitrosourea- induced rat mammary carcinoma. J Cancer Res Clin Oncol. 116:538–49.
- Das, T., Banerjee, S., Samuel, G., Bapat, K., Subramanian, S., Pillai, M.R.A., Venkatesh, M. (2006). A novel 99mTc-labeled testosterone derivative as a potential agent for targeting androgen receptors. Bioorg Med Chem Lett. 16:5788–92.
- Denis, L.J., Grifiths, K. (2000). Endocrine treatment in prostate cancer. Sem Surg Oncol. 18:52–74.
- Diasio, R.B., Harris, B.E. (1989). Clinical pharmacology of 5-fluorouracil. Clin Pharmacokinet. 16:215–37.
- Fry, D.W., White, J.C., Goldman, I.D. (1978). Rapid separation of low molecular weight solutes from liposomes without dilution. J Anal Biochem. 90:809–15.
- Gabizon, A., Amselem, S., Goren, D., Cohen, R., Druckmann, S., Fromer, I., Chisin, R., Peretz, T., Sulkes, A., Barenholz, Y. (1990). Preclinical and clinical experience with a doxorubicin-liposome preparation. J Liposome Res. 1:491–502.
- Gupta, Y., Jain, A., Jain, P., Jain, S.K. (2007). Design and development of folate appended liposomes for enhanced delivery of 5-FU to tumor cells. J Drug Target. 15:231–40.
- Gupta, Y., Soni, V., Chourasia, M.K., Jain, A., Khare, P., Jain, S.K. (2005). Targeted drug delivery to the brain via transferrin coupled liposomes. Drug Deliv Tech. 5:66–71.
- Hager, G.L., Smith, C.L., Fragoso, G., Wolford, R., Walker, D., Barsony, J., Htun, H. (1998). Intranuclear traffcking and gene targeting by members of the steroid/nuclear receptor superfamily. J Steroid Biochem Molec Biol. 65:25–132.
- Hahn, R.G., Moertel, C.G., Schutt, A.J., Bruckner, H.W. (1975). A double-blind comparison of intensive course 5-fluorouracil by oral vs. intravenous route in the treatment of colorectal carcinoma. Cancer. 35:1031–5.
- Harding, M., Cowan, S., Hole, D., Cassidy, L., Kitchener, H., Davis, J., Leake, R. (1990). Estrogen and progesterone receptors in ovarian cancer. Cancer. 65:486–91.
- Hoffmann, J., Sommer, A. (2005). Steroid hormone receptors as targets for the therapy of breast and prostate cancer—recent advances, mechanisms of resistance, and new approaches. J Steroid Biochem Mol Biol. 93:191–200.
- Hwang, D.R., Scott, M.E., Hedaya, E. (1992). Phospholipids conjugates and their preparation. US patent, 5,106,963.
- Kampa, M., Papakonstanti, E.A., Hatzoglou, A., Tathopoulos, E.N., Stournaras, C., Castanas, E. (2002). The human prostate cancer cell line LNCaP bears functional membrane testosterone receptors that increase PSA secretion and modify actin cytoskeleton. FASEB J. 16:1429–31.
- Karajgi, J., Jain, N.K., Vyas, S.P. (1993). Passive vectoring of a colloidal carrier system for sodium stibogluconate: preparation, characterization and performance evaluation. J Drug Target. 1:197–206.
- Katzenellenbogen, J.A., Coleman, R.E., Kins, R.A., Krohan, K.A., Larson, S.M., Mendelsohn, J., Osborne, C.K., Piwnica-Worms, D., Reba, R.C., Siegel, B.A. (1995). Tumor receptor imaging: proceedings of the national cancer institute workshop, review of current work, and prospective for further investigations. Clin Cancer Res. 921:921–32.
- Khandare, J., Kolhe, P., Pillai, O., Kannan, S., Lieh-Lai, M., Kannan, R.M. (2005). Synthesis, cellular transport, and activity of polyamidoamine dendrimer methylprednisolone conjugates. Bioconjug Chem. 16:330–7.
- Kidwai, N., Gong, Y., Sun, X., Deshpande, C.G., Yeldandi, A.V., Rao, M.S., Badve, S. (2004). Expression of androgen receptor and prostate-specific antigen in male breast carcinoma. Breast Cancer Res. 6:18–23.
- Krishnaiah, Y.S.R., Satyanarayana, V., Kumar, D.B., Karthikeyan, R.S., Bhaskar, P. (2003). In vivo pharmacokinetics in human volunteers: oral administered guar gumbased colon-targeted 5-fluorouracil tablets. Eur J Pharm Sci. 19:355–62.
- Kuduk, S.D., Harris, C.R., Zheng, F.F., Sepp-Lorenzino, L., Ouerfelli, Q., Rosen, N., Danishefsky, S.J. (2000). Synthesis and evaluation of geldanamycin-testosterone hybrids. Bioorg Med Chem Lett. 10:1303–6.
- Labianca, R.F., Beretta, G.D., Pessi, M.A. (2001). Disease management consideration. Drugs. 61:1751–64.
- Langenbach, R.J., Dancenberg, P.V., Heidelberger, C. (1972). Thymidylate synthetase: mechanism of inhibition of 5-fluorouracil-2-deoxyuridylate. Biochem Biophys Res Commun. 48:1565–71.
- Leuschner, C., Hansel, W. (2005). Targeting breast and prostate cancers through their hormone receptors. Biol Reprod. 73:860–5.
- Loven, D., Rakowsky, E., Geier, A., Lunenfeld, B., Rubinstein, A., Klein, B., Lurie, H. (1990). A clinical evaluation of nuclear estrogen receptors combined with cytosolic estrogen and progesterone receptors in breast cancer. Cancer. 66:341–6.
- Maeda, H., Wu, J., Sawa, T., Matsumura, Y., Hori, K. (2000). Tumor vascular permeability and the EPR effect in macromolecular therapeutics: a review. J Contr Rel. 65:271–84.
- Manosroi, A., Kongkaneramit, L.L., Manosroi, J. (2004). Characterization of amphotericin B liposome formulation. Drug Dev Ind Pharm. 30:535–43.
- Molnar, I., Kute, T., Willingham, M.C., Schwartz, G.G. (2004). 19-Nor-1alpha, 25- dihydroxyvitamin D2 (paricalcitol) exerts anticancer activity against HL-60 cells in vitro at clinically achievable concentrations. J Steroid Biochem Mol Biol. 89–90:39–43.
- Parker, W.B., Cheng, Y.C. (1990). Metabolism and mechanism of action of 5- fluorouracil. Pharmacol Ther. 48:381–95.
- Rai, S., Paliwal, R., Vaidya, B., Khatri, K., Gupta, P.N., Goyal, A.K., Vyas, S.P. (2008). Targeted delivery of doxorubicin via estrone appended liposomes. J Drug Target. 16:455–63.
- Rajkowski, K.M., Cittanova, N., Desfosses, B., Jayle, M.F. (1997). The conjugation of testosterone with horseradish peroxidase and a sensitive enzyme assay for the conjugate. Steroids. 29:701–13.
- Recht, L., Torres, C.O., Smith, T.W., Raso, V., Griffin, T.W. (1990). Transferrin receptor in normal and neoplastic brain tissue: Implications for brain-tumor immunotherapy. J Neurosurg. 72:941–5.
- Reddy, B.S., Banerjee, R.K. (2005). 17ß-Estradiol-associated stealth-liposomal delivery of anticancer gene to breast cancer cells. Angew Chem Int Ed. 44:6723–7.
- Roth, T., Tang, W., Eisenbrand, G. (1995). Synthesis of novel androgen-linked phosphoramide mustard prodrugs and growth inhibitory activity in human breast cancer cells. Anticancer Drug Des. 10:655–6.
- Rusch, V., Mendelsohn, J., Dmitrovsky, E. (1996). The epidermal growth factor receptor and its ligands as therapeutic targets in human tumors. Cytokine Growth Factor Rev. 7:133–41.
- Schuber, F. (1995). Chemistry of ligand coupling to liposomes. In: Philippot, J.R., Schuber, F. (Eds.), Liposomes as tools in basic research and industry. Boca Raton, FL: CRC Press. P. 21.
- Seymour, L.W. (1994). Soluble polymer for lectin-mediated targeting. Adv Drug Del Rev. 14:89–111.
- Swamy, N., James, D.A., Mohr, S.C., Hanson, R.N., Ray, R. (2002). An estradiolporphyrin conjugate selectively localizes into estrogen receptor positive breast cancer cells. Bioorg Med Chem. 10:3237–43.
- Torp, S.H., Helseth, E., Dalen, A., Unsgaard, G. (1991). Epidermal growth factor receptor expression in human gliomas. Cancer Immunol Immunother. 33:61–4.
- Van, C.E., Peeters, M., Verslype, C., Filez, L., Haustermans, K., Janssens, J. (1999). The medical treatment of colorectal cancer: actual status and new developments. Hepatogastroenterology. 46:709–16.
- Weitman, S.D., Lark, R.H., Coney, L.R., Fort, D.W., Frasca, V., Zurawski, V.R., Kamen, B.A. (1992). Distribution of the folate receptor GP38 in normal and malignant cell lines and tissues. Cancer Res. 52:3396–401.