Abstract
The aim of this research was to develop pH-sensitive insulin-loaded NOCC (N,O-carboxymethyl chitosan) nanoparticles for the controlled release of insulin via the oral route. Thus, in this study, insulin-loaded NOCC nanoparticles were prepared by ionic gelation of NOCC with TPP (tripolyphosphate). NOCC nanoparticles were formed at conditions of 2 mg/ml of NOCC and 1 mg/ml of TPP. It was found that the encapsulation efficiency and process yield decreased with increasing NOCC to TPP weight ratio. Furthermore, the cumulative release of insulin from insulin-loaded NOCC nanoparticles decreased with decreasing NOCC-to-TPP weight ratio, but it increased with decreasing the initial concentration of insulin. The higher the pH of the phosphate buffered saline, the greater the amount of cumulative release of insulin-loaded NOCC nanoparticles, and thus they could protect insulin from acid.
Introduction
Insulin is the most commonly used drug for controlling plasma glucose levels. In this study, insulin-loaded NOCC (N,O-carboxymethyl chitosan) nanoparticles were prepared by very mild ionic gelation of NOCC with TPP (tripolyphosphate) involving the mixture of aqueous phases at room temperature (CitationCalvo et al., 1997; CitationFernández-Urrusuno et al., 1999). In addition, genipin-cross-linked NOCC/alginate, as a pH-sensitive hydrogel, was used for sustained protein drug delivery in the stomach (CitationChen et al., 2004). Insulin-loaded chitosan nanoparticles were prepared by ionotropic gelation of chitosan with TPP, and these proved to enhance the nasal absorption of insulin (CitationFernández-Urrusuno et al., 1999). Compared with an aqueous solution of chitosan, insulin-loaded chitosan nanoparticles prepared by ionotropic gelation of chitosan with TPP have been shown to improve the intestinal absorption of insulin in vivo (CitationPan et al., 2002). Therefore, the insulin-loaded NOCC nanoparticles could render insulin less susceptible to acid due to pH-sensitive NOCC, and enhance the intestinally stable absorption of insulin via the oral route because of nanoparticles.
Chitosan is a biopolymer that has been exploited for the controlled release of therapeutic drugs due to its excellent biocompatible characteristics (CitationShu & Zhu, 2000; CitationMi et al., 2002). Chitosan is the deacetylated form of chitin, which is itself a natural polymer extracted commercially from the economic exploitation of the exoskeletons of crustaceans, such as prawns, crabs, shrimps; and insects. In order to protect insulin from the harsh environment of gastric media in the stomach, pH-sensitive insulin-loaded NOCC nanoparticles have been developed for the controlled delivery of insulin via the oral route. NOCC is a water-soluble chitosan derivative having carboxymethyl substituents on some of both the amino and primary hydroxyl sites of the glucosamine units of the chitosan structure (CitationChen et al., 2004).
To avoid possibly undesirable effects, such as toxicity, drug release from NOCC may be controlled by exploiting reversible physical cross-links rather than chemical cross-links. For example, tripolyphosphate (TPP) is non-toxic and its multivalent anions are able to interact with the positively charged amino groups in chitosan via electrostatic forces (CitationShu & Zhu, 2000; CitationKo et al., 2002). Furthermore, the more-negative charges on TPP have shown a significant ability to ionically cross-link chitosan (CitationShu & Zhu, 2002).
Previously, hydrophilic chitosan nanoparticles as protein carriers were obtained by inducing the gelation of 5.0 mL of the chitosan solution (1.0–3.0 mg/mL) with 2.0 mL of the TPP solution (0.2–1.0 mg/mL) (CitationCalvo et al., 1997), and insulin-loaded chitosan nanoparticles were prepared by ionotropic gelation of chitosan with TPP (CitationFernández-Urrusuno et al., 1999; CitationPan et al., 2002). In this study, pH-sensitive insulin-loaded NOCC nanoparticles were prepared by ionic gelation of NOCC instead of chitosan with TPP. The objective of this research was to identify better/superior conditions (in terms of NOCC-to-TPP weight ratio and initial concentration of insulin) for the release behavior, encapsulation efficiency, and process yield of these pH-sensitive insulin-loaded NOCC nanoparticles which could protect insulin from acid in the stomach.
Materials and methods
Chitosan with 300 kDa molecular weight and a deacetylation percentage of ~95% was supplied by Primex Enterprises Company (Iceland). Isopropanol (purity: 95%) and chloroacetic acid were supplied by Fluka Enterprises Company (Switzerland) and J.T. Baker Enterprises Company (USA), respectively. TPP was supplied by Sigma Enterprises Company (USA), which also supplied the insulin used in the study.
NOCC was synthesized by slightly modifying the procedures described in previous literature (CitationHayes, 1986; CitationChen et al., 2004). A mass of 15 g of chitosan was suspended in 150 ml of isopropanol, and the resulting slurry was stirred at an agitation rate of 300–400 rpm and a temperature of 25°C. Next, 38 ml of 10 N aqueous NaOH solution were added in six equal portions at 4 min intervals. The alkaline slurry was stirred for an additional 45 min, then 18 g of chloroacetic acid were added in five equal portions at 3 min intervals. Subsequently, the mixture was heated to a temperature of 60°C, and stirring at this temperature was continued for 3 h. Finally, the reaction mixture was filtered and the filtered solid product was rinsed with 99.9% (v/v) methanol, then rinsed with pure de-ionized water, and subsequently oven-dried in air (50°C) for 3 days. Solid state nuclear magnetic resonance spectroscopy (SS-NMR: Bruker, Avance 400, Germany) was used to identify substitutions of carboxymethyl groups on the amino and primary hydroxyl sites on the NOCC.
NOCC nanoparticles were obtained by ionotropic gelation of NOCC with TPP. In order to determine the production zone of the nanoparticle formation, the following experiments were done. NOCC was dissolved in 1% (v/v) acetic acid aqueous solutions at different NOCC concentrations (1.0, 2.0, and 3.0 mg/ml) and TPP was dissolved in deionized water at various TPP concentrations (0.1, 0.5, and 1.0 mg/ml). Next, 2.0 ml of different TPP concentration solutions were added to 5.0 ml of various NOCC concentration solutions under magnetic stirring (600 rpm) at room temperature for 5 min and then samples were visually inspected. If an opalescent suspension could be found in a sample, it was assumed that NOCC nanoparticles had formed.
Insulin was dissolved in 0.01 M NaOH at different insulin concentrations (0.1, 0.5, and 1.0 mg/ml). Insulin pre-mixed with the TPP solution has a slightly higher association efficiency (AE) than that with the chitosan solution (CitationMa et al., 2002), and thus 1.0 ml of different insulin concentration solutions was pre-mixed with 2.0 ml of the TPP solution (1.0 mg/ml, forming an opalescent suspension) before 2.0 ml of the TPP solution were added to 5.0 ml of the NOCC solution (2.0 mg/ml, forming an opalescent suspension) under magnetic stirring (600 rpm) at room temperature for 5 min. Finally, in order to determine the encapsulation efficiency and process yield, insulin-loaded NOCC nanoparticles were separated from the aqueous suspension medium by centrifugation at 50,000 × g and 20°C for 20 min. Next, they were rinsed ultrasonically with 1.0 ml of de-ionized water, then frozen, subsequently vacuum freeze-dried (−53°C) for 3 days and weighed. The amount of free insulin in the clear supernatant solution was measured by RP-HPLC system equipped with a UV detector (Jasco, UV-1575, USA), a Waters Spherisorb column (ODS1, S10, 4.6 × 250 mm, Waters Corporation, Ireland), and pump (Jasco, PU-1580, USA) (CitationMa et al., 2002). The encapsulation efficiency and process yield were calculated according to the following equations:
where ITot = total amount of insulin and If = amount of free insulin.
where WNOCC = weight of insulin-loaded NOCC nanoparticles and WTot = total weight (NOCC + TPP + insulin).
Size and zeta potential for the NOCC nanoparticles prepared under different experimental conditions (different ratios (NOCC/TPP, w/w), insulin concentrations, and pHs of buffered solution) were evaluated. Sizes of nanoparticles were measured on freshly prepared samples by light scattering using a particle size analyzer system (90 Plus, Brookhaven Instruments Corporation, USA). Zeta potentials were measured on a Zeta Plus (Brookhaven Instruments Corporation, USA). Functional groups of chitosan and NOCC were analyzed by Fourier transform infrared spectroscopy (FTIR: Spectrum one, Perkin-Elmer Instrument, USA) using KBr pellets. Furthermore, the morphologies of NOCC nanoparticles and insulin-loaded NOCC nanoparticles were examined through a transmission electron microscope (TEM: JEOL JEM-2010, Japan) The samples were stained with 2% (w/v) phosphotungstic acid and placed on copper with carbon films for TEM observation.
Insulin-loaded NOCC nanoparticles were suspended in 10 ml of 0.01 M HCl solution (pH = 2.5) as well as PBS (phosphate buffered saline, pH = 7.4,) and then incubated on a shaking water bath (70 rpm) at 37°C. Aliquots of 1.0 ml were withdrawn at appropriate intervals and an equal volume of fresh medium was added to maintain a constant volume. The amount of released insulin was analyzed using HPLC. All the experiments were carried in triplicate.
Results and discussion
FTIR spectra of chitosan and NOCC are shown in , where the peak observed at 1029 cm−1 is the primary hydroxyl group (characteristic peak of –CH2–OH in primary alcohols, C–O stretch). From a comparison of the spectra of chitosan and NOCC, the peak (1029 cm−1) intensity of chitosan decreased as a consequence of the carboxymethylation of chitosan. This shows that a carboxymethyl group was substituted for –CH2–OH at the C6 position of NOCC. The spectrum of NOCC shows the peak of 1600 cm−1 for carboxylic acid salt (–COO− antiym stretch), hinting that there were carboxymethyl groups on NOCC. In contrast, in the spectrum of chitosan, the peak (1650 cm−1) represents the amino group (NH2 deformation). NMR spectra of chitosan and NOCC are shown in , respectively. From a comparison of the spectra of chitosan and NOCC, the peak (174 ppm) for –C=O of –NHCOCH3 on chitosan is shifted to 164 ppm for –C=O of –NHCOCH3 on NOCC and two new peaks (177 and 181 ppm) representing carboxymethyl groups at the O-position of C6, as well as the N-position of C2 of NOCC, respectively. This suggests that carboxymethyl groups were substituted to some of the primary hydroxyl and amino sites of NOCC. According to a method previously described in the literature (CitationSugimoto et al., 1998), the degrees of substitution of carboxymethyl groups on primary hydroxyl and amino sites were ~10.7% and 7.1%, respectively.
NOCC nanoparticles were obtained by inducing the gelation of NOCC with TPP. Then samples were visually inspected and three different systems were identified: clear solution, opalescent suspension, and aggregates. This opalescent suspension, which could correspond to a suspension of NOCC nanoparticles (formation conditions: 2 mg/ml of NOCC and 1 mg/ml of TPP), is listed in .
Table 1. NOCC nanoparticles were obtained by inducing the gelation of different concentrations of NOCC with different concentrations of TPP, then samples were visually inspected and three different systems (•: clear solution, ▴: opalescent suspension, and ♦: aggregates) were identified.
NOCC nanoparticles (NOCC: 2 mg/ml) displayed particle sizes from 242–275 nm and a positive zeta potential in the range of 22–38 mV, and the particle size as well as zeta potential of NOCC nanoparticles increased with increasing NOCC-to-TPP weight ratio. The reason behind this behavior may be that the lower the NOCC-to-TPP weight ratio, the higher the TPP concentration, and the higher the cross-linking density of NOCC nanoparticles, thus leading to more a compact structure of NOCC nanoparticles, and therefore decreasing the size of individual NOCC nanoparticles. TPP with multivalent anions can interact with positively charged amino groups in NOCC. Therefore, the lower the NOCC-to-TPP weight ratio, the higher the TPP concentration, and the higher the cross-linking density of NOCC nanoparticles, thus leading to more positively charged amino groups in NOCC ionically cross-linking with tripolyphosphoric groups in TPP, and therefore decreasing the zeta potential of the NOCC nanoparticles. In addition, the particle size of the NOCC nanoparticle with the aggregative polyhedron shape for the higher NOCC-to-TPP weight ratio (5/1) is larger than that of NOCC nanoparticles with spherical shapes for the lower NOCC to TPP weight ratio (3/1) (see ). This result confirms the above particle size distribution. The insulin-loaded NOCC nanoparticle possessed a typically spherical shape and very fine particles on its surface (see ), suggesting that insulin molecules were absorbed on the surface of the NOCC nanoparticle.
Figure 3. TEM (the scale bar = 100 nm) of NOCC nanoparticles (A) NOCC-to-TPP weight ratio = 3, NOCC: 2 mg/ml; (B) NOCC-to-TPP weight ratio = 5, NOCC: 2 mg/ml; (C) NOCC-to-TPP weight ratio = 3, NOCC: 2 mg/ml, insulin: 0.5 mg/ml.
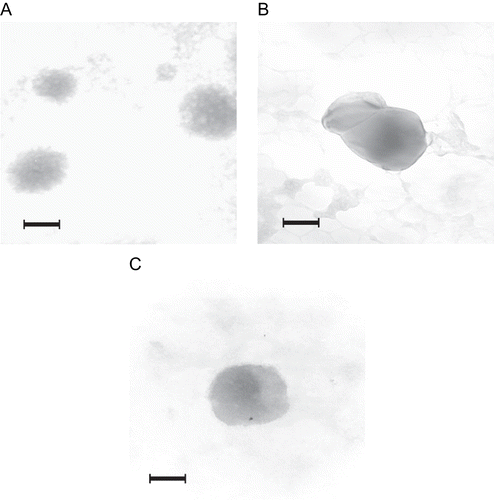
shows the influence of NOCC-to-TPP weight ratio on insulin encapsulation efficiency for insulin-loaded NOCC nanoparticles (NOCC: 2 mg/ml and insulin: 1 mg/ml). NOCC nanoparticles (NOCC: 2 mg/ml) displayed process yield in the range of 60–16% for NOCC-to-TPP weight ratio from 3 to 7. In general, the encapsulation efficiency and process yield decreased with increasing NOCC-to-TPP weight ratio. The reason behind this may be that the lower the NOCC-to-TPP weight ratio, the higher the TPP concentration, and the higher the cross-linking density of insulin-loaded NOCC microparticles, thus leading to a more compact structure of insulin-loaded NOCC nanoparticles. This then causes the particle size decrease of insulin-loaded NOCC nanoparticles and it is very likely that reticulation allowed smaller size NOCC nanoparticles to capture more insulin. A similar result has been published in previous literature (CitationGrenha et al., 2005). TPP with anions can interact with positively charged amino groups in NOCC. Therefore, the lower the NOCC-to-TPP weight ratio, the higher the TPP concentration, thus leading to more amino groups in NOCC ionically cross-linking with tripolyphosphoric groups in TPP, and therefore increasing process yield of the NOCC nanoparticles.
Figure 4. Influence of NOCC-to-TPP weight ratio on insulin encapsulation efficiency for insulin-loaded NOCC nanoparticles (NOCC: 2 mg/ml and insulin: 1 mg/ml) (mean ± SD, n = 3).
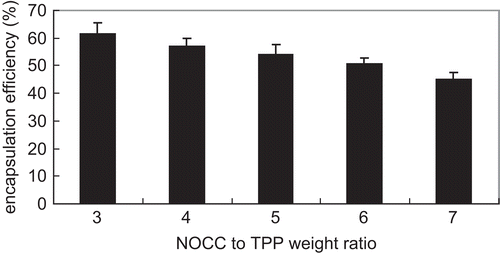
The zeta potential of insulin-loaded NOCC nanoparticles (NOCC-to-TPP weight ratio = 3, NOCC: 2 mg/ml, insulin: 0.5 mg/ml) increased with decreasing pH of solutions (see ). This can be explained because, at lower pHs, more protons are available to form NH3+ groups with amine groups in insulin-loaded NOCC nanoparticles. However, the particle size of insulin-loaded NOCC nanoparticles increased with increasing pH of solutions (see ). The reason behind this may be that the higher the pH of solutions, the greater the amount of carboxylic acid salt (–COO−), thus leading to increased electrostatic repulsion of inter and intra molecules, and then increasing the particle size of insulin-loaded NOCC nanoparticles.
Figure 5. Effect of pH of solutions on the particle size (♦) and zeta potential (▴) of insulin-loaded NOCC nanoparticles (NOCC-to-TPP weight ratio = 3, NOCC: 2 mg/ml, insulin: 0.5 mg/ml).
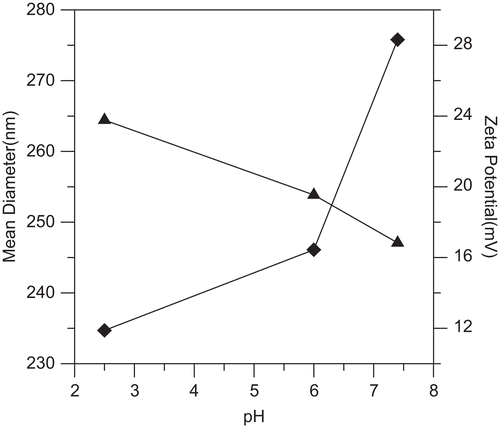
depict the effect of NOCC-to-TPP weight ratio and pH of PBS on the release profiles of insulin from insulin-loaded NOCC nanoparticles (NOCC: 2 mg/ml and insulin: 0.5 mg/ml). The results show insulin release was very rapid at an early stage and the maximum amounts of insulin being released were ~40–80%, as well as 70–90% for pH 2.5 as well as pH 7.4, respectively. It might be suggested that the burst release of insulin is associated with some insulin molecules dispersed at and near the surface of the NOCC nanoparticle (see ), which diffuse out in the initial incubation time. The higher the pH of the PBS, the greater the amount of carboxylic acid salt (–COO−), thus leading to increased electrostatic repulsion of inter- and intra-molecules, and therefore increasing the pore size and cumulative release of insulin-loaded NOCC nanoparticles. After a 660-min release at pH 7.4, the cumulative release of insulin-loaded NOCC nanoparticles still maintained at ~70–90%. This can be explained because some insulin molecules may be cross-linked with the nanopartice network directly by TPP. In addition, the cumulative release of insulin from insulin-loaded NOCC nanoparticles decreased with decreasing NOCC-to-TPP weight ratio. The reason behind this behavior may be that the lower the NOCC-to-TPP weight ratio, the higher the TPP concentration, the higher the cross-linking density of insulin-loaded NOCC nanoparticles, thus leading to a more compact structure of insulin-loaded NOCC nanoparticles, and therefore decreasing the diffusion as well as cumulative release of insulin from insulin-loaded NOCC nanoparticles.
Figure 6. Influence of NOCC-to-TPP weight ratio on cumulative release of insulin from insulin-loaded NOCC nanoparticles (NOCC: 2 mg/ml and insulin: 0.5 mg/ml) in (A) pH = 2.5, and (B) pH = 7.4 of PBS (mean ± SD, n = 3).
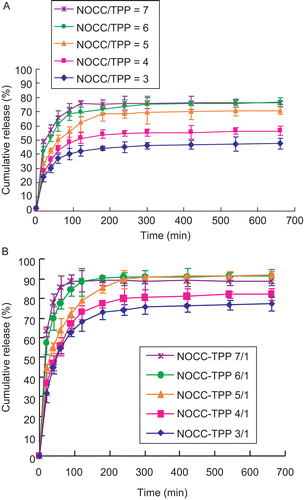
shows the effect of initial concentrations of insulin on cumulative release of insulin from insulin-loaded NOCC nanoparticles (NOCC: 2 mg/ml and NOCC to TPP weight ratio: 3) in pH = 7.4 of PBS. The cumulative release of insulin from insulin-loaded NOCC nanoparticles decreased with increasing the initial concentration of insulin. The reason behind this behavior may be that the higher the initial concentration of insulin, the higher the hydrophobic interactions to self aggregate into a critical nucleus as well as eventually into fibrils for insulin, thus decreasing the cumulative release of insulin from insulin-loaded NOCC nanoparticles (CitationMa et al., 2002).
Conclusions
From FTIR and NMR spectra of chitosan and NOCC, carboxymethyl groups were substituted to some primary hydroxyl and amino sites of NOCC. In addition, the opalescent suspension could correspond to a suspension of NOCC nanoparticles (formation conditions: 2 mg/ml of NOCC and 1 mg/ml of TPP). The particle size as well as zeta potential of NOCC nanoparticles increased with increasing NOCC-to-TPP weight ratio. The particle size was also confirmed by TEM. Furthermore, the particle size of insulin-loaded NOCC nanoparticles increased with increasing pH of solutions, but the zeta potential of insulin-loaded NOCC nanoparticles decreased with increasing pH of solutions.
Acknowledgements
Declaration of interest: The authors report no conflicts of interest. The authors alone are responsible for the content and writing of the paper.
References
- Calvo, P., Remuñán-López, C., Vila-Jato, J.L., Alonso, M.J. (1997). Novel hydrophilic chitosan-polyethylene oxide nanoparticles as protein carriers. J Appl Polym Sci. 63:125–32.
- Chen, S.C., Wu, Y.C., Mi, F.L., Lin, Y.H., Yu, L.C., Sung, H.W. (2004). A novel pH-sensitive hydrogel composed of N,O-carboxymethyl chitosan and alginate cross-linked by genipin for protein drug delivery. J Contr Rel. 96:285–300.
- Fernández-Urrusuno, R., Calvo, P., Remuñán-López, C., Vila-Jato, J.L., Alonso, M.J. (1999). Enhancement of nasal absorption of insulin using chitosan nanoparticles. Pharm Res. 16:1576–81.
- Grenha, A., Seijo, B., Remuñán-López, C. (2005). Microencapsulated chitosan nanoparticles for lung protein delivery. Eur J Pharm Sci. 25:427–37.
- Hayes, E.R. (1986). N,O-carboxymethyl chitosan and preparative method therefore. US Patent 4,619,995.
- Ko, J.A., Park, H.J., Hwang, S.J., Park, J.B., Lee, J.S. (2002). Preparation and characterization of chitosan microparticles intended for controlled drug delivery. Int J Pharm. 249:165–74.
- Ma, Z., Yeoh, H.H., Lim, L.Y. (2002). Formulation pH modulates the interaction of insulin with chitosan nanoparticles. J Pharm Sci. 91:1396–404.
- Mi, F.L., Shyu, S.S., Chen, C.T., Lai, J.Y. (2002). Adsorption of indomethacin onto chemically modified chitosan beads. Polymer. 43:757–65.
- Pan, Y., Li, Y.J., Zhao, H.Y., Zheng, J.M., Xu, H., Wei, G., Hao, J.S., Cui, F.D. (2002). Bioadhesive polysaccharide in protein delivery system: chitosan nanoparticles improve the intestinal absorption of insulin in vivo. Int J Pharm. 249:139–47.
- Shu, X.Z., Zhu, K.J. (2000). A novel approach to prepare tripolyphosphate/chitosan complex beads for controlled release drug delivery. Int J Pharm. 201:51–8.
- Shu, X.Z., Zhu, K.J. (2002). The influence of multivalent phosphate structure on the properties of ionically cross-linked chitosan films for controlled drug release. Eur J Pharm Biopharm. 54:235–43.
- Sugimoto, M., Morimoto, M., Sashiwa, H., Saimoto, H., Shigemasa, Y. (1998). Preparation and characterization of water-soluble chitin and chitosan derivatives. Carbohydr Polym. 36:49–59.