Abstract
The objectives of this research were to prepare ribavirin niosomes and evaluate the influence of niosomal encapsulation on drug liver targeting in rats. Ribavirin niosomes were prepared by the thin film hydration method using span 60, cholesterol, and dicetyl phosphate in molar ratios of (1:1:0), (4:2:0), (1:1:0.1), and (4:2:1). The prepared niosomes were characterized in vitro for vesicle size, drug entrapment, drug release profiles, and vesicular stability at refrigerator temperature. The results indicated that niosomes of the molar ratio (4:2:1) had a significantly (p < 0.05) higher entrapment percentage of ribavirin than the other molar ratios, moreover, they revealed sustained release characteristics as well as longer release pattern than other niosomal formulations. Accordingly, niosomes of molar ratio (4:2:1) was selected for in vivo liver targeting study. Separately, niosomal ribavirin dispersion and free ribavirin solution were administered as a single dose of 30 mg/kg by intraperitoneal injection into two groups of rats to compare the liver ribavirin concentration. The obtained results show that the niosomal formulation significantly increased ribavirin liver concentration (6-fold) in comparison with ribavirin-free solution. Based on the previous results, the use of niosomes as a drug delivery system for ribavirin has significant liver targeting properties, this is expected to improve the efficacy of low doses of ribavirin and minimize its toxic side-effects at higher doses.
Introduction
Ribavirin is a synthetic water-soluble nucleoside that possesses broad spectrum activity against a variety of DNA and RNA viruses, including those of the flavivirus family, of which hepatitis C virus (HCV) is a member (CitationPatterson & Fernandez-Larsson, 1990). The current treatment for chronic hepatitis C infection is the combination of a pegylated interferon and ribavirin. The recommended daily dose of ribavirin ranges from 800–1200 mg, dependent on hepatitis C virus genotype and bodyweight (CitationStrader et al., 2004). The optimal dose of ribavirin remains uncertain, but studies suggest that higher serum ribavirin concentrations result in higher response rates (CitationJen et al., 2000; CitationLarrat et al., 2003; CitationLindahl et al., 2005). However, achieving the latter with higher doses of ribavirin must be balanced against potential increases in drug-related hemolysis. Encapsulation of the drug in vesicular structures like liposomes and niosomes can be expected to enhance penetration into target tissue, reduce the required therapeutic dose, and decrease the toxicity of the encapsulated drug (CitationBiju et al., 2006).
A drug delivery system using colloidal particulate carrier such as liposomes (CitationIjeoma & Vyas, 1998) or niosomes (CitationSchreier & Bouwstra, 1994) has distinct advantages over conventional dosage forms because the particles can act as drug containing reservoirs. Modification of the particle composition or surface can adjust the affinity for the target site and/or the drug release rate. Niosomes are vesicular delivery systems which result from the self-assembly of hydrated surfactant monomers (CitationArunothayanun et al., 1999). They are similar in physical structure and form to the more widely studied phospholipid vesicles (liposomes), consisting of single or multiple surfactant bilayers. However, they may have some advantages over liposomes with respect to chemical stability, lower costs of the chemicals, and large amount of surfactant classes available for the design of this vesicular system (CitationBaillie et al., 1985).
Niosomes have been used to modify the tissue distribution of entrapped methotrexate (CitationAzmin et al., 1985; CitationChandraprakash et al., 1990), doxorubicin (CitationRogerson et al., 1987; Citation1988), sodium stibogluconate (CitationHunter et al., 1988), and diclofenac sodium (CitationRaja et al., 1993).
In the present study, ribavirin encapsulated niosomes were formulated and evaluated for their in vitro and in vivo characteristics in an attempt to provide a maximum concentration of ribavirin in the liver by niosomal encapsulation of the drug; this is expected to improve the efficacy of low doses of the drug and minimize the risk of hemolytic anemia associated with the higher doses of ribavirin.
Materials
Ribavirin was supplied from October Pharm. Co (Cairo, Egypt). Cholesterol (CH) was from Fluka Biochemika, Sigma Aldrich (Germany). Dicetyl phosphate (DCP) was purchased from Sigma Chemical Co (St. Louis, MO). Sorbitan monostearate (Span 60; SP) was purchased from Merck Schuchardit OHG (Germany). All other chemicals used were of analytical grade.
Methods
Preparation of ribavirin niosomes
Ribavirin niosomes were prepared using the thin film hydration method (CitationArunothayanun et al., 2000). Span 60, cholesterol, and dicetyl phosphate in molar ratios of 1:1:0 and 4:2:0 were used for preparation of neutral niosomes. In addition, 1:1:0.1 and 4:2:1 molar ratios were used for preparation of negatively charged niosomes.
The lipid mixtures (100 mg) used in the different niosomal formulations were dissolved in 10 ml chloroform. The organic solvent was evaporated using a rotary evaporator (BÜCHI, M, HB-140, Switzerland) to produce a thin lipid film. The thin film was then hydrated for 1 h at 60 ± 2°C with 10 ml of acetate buffered saline (pH 5.6) containing 20 mg of ribavirin pre-warmed to 56–58°C. The hydration step took place in the presence of seven glass beads 4 mm in diameter. The resulting niosomes dispersion was used for the determination of the entrapment efficiency, particle size analysis, and morphological studies.
Characterization of ribavirin niosomes
Microscopic examination
The freshly prepared niosomes in acetate buffered saline buffer (pH = 5.6) was imaged using an image analyzer (Leica Q 500 MC, Imaging System, Germany) with the magnification power of ×40.
Particle size and particle size distribution
The vesicle size distribution was determined using a laser diffraction technique on a Mastersizer X Ver. 2.15; Malvern instruments Ltd. (Malvern, UK). The measurements were performed at 25°C, using a 45 mm focus lens and a beam length of 2.4 mm.
Entrapment efficiency
Free ribavirin was separated from niosomes-entrapped ribavirin by centrifugation. Ten milliliters of niosomes dispersion was centrifuged (Union 32R Hanil – Co.) at 7000 rpm at −4°C for 1 h. The supernatant was separated and the niosomal residue was re-suspended in 15 ml of acetate buffered saline (pH 5.6) and centrifuged again. This washing procedure was repeated twice to ensure that the free drug was no longer present in the voids between the niosomes. The collected supernatant fractions were suitably diluted with acetate buffered saline and were used for determination of the free ribavirin spectrophotometerically at λ = 221 nm. The amount of entrapped ribavirin was obtained by subtracting the amount of free drug from the total drug incorporated in 10 ml niosomal dispersion (CitationDeepika & Indu, 2005). The purified niosomal residues were used in determination of the in vitro release of ribavirin from niosomes.
In-vitro release of ribavirin from niosomes
The niosomal residues (separated by centrifugation and washed as described above) of the different niosomal formulations were re-suspended in 10 ml of acetate buffer (pH 5.6). The niosomal suspensions were placed in a shaking water bath at 37°C and rotated at 50 rpm. One milliliter samples were withdrawn at the following time intervals: 3, 6, 9, and 12 h. Samples were centrifuged and niosomal pellets was washed as mentioned before and supernatants were assayed spectrophotometerically for ribavirin content at 221 nm. The percentage of drug release was plotted as a function of time (CitationKaur & Kanwar, 2002; CitationMitra, 2003).
Kinetics studies of the release data
The release data were fitted to mathematical models such as the zero order, first order, and Higuchi’s model. The criterion for selecting the most appropriate model was based on a goodness of fit test.
Stability studies
The stability study was carried out on the niosomes of molar ratio of 4:2:1. The ability of niosomal vesicles to retain the drug was assessed by keeping the niosomal dispersion at refrigeration temperature 4–8°C for a period of 6 months. The niosomal formulation was stored in glass vials sealed with aluminum foil. The samples were withdrawn at the end of the study period. The free ribavirin was separated (as previously described) and was analyzed for drug content spectrophotometerically.
Ribavirin liver targeting study
Approval to carry out the ribavirin in vivo liver targeting study was obtained from the Animal Ethics Committee of Faculty of Pharmacy, Helwan University. Guidelines of the ethics committee were followed for the studies. Healthy female hamster rats (8–10 weeks old and weight 270 ± 50 g, n = 10) were used in this study. All rats were housed and received similar diet. The rats were divided randomly into two groups, each was of five rats. Rats were fasted overnight for 12 h with free access to water. On the day of experiment, each group received a single dose of ribavirin (30 mg/kg) through intra-peritoneal injection (CitationChen-Chung et al., 2003). The first group received free ribavirin solution in normal saline acetate buffer (pH, 5.6) and the second group received the same dose of ribavirin niosomal formulation. Four hours after administration, all rats were sacrified by cervical dislocation. The rats were dissected, and their livers were removed and rinsed with saline solution to remove any adhered debris, blotted dry with filter paper, then stored at −20°C until analysis.
Liver sample preparation
Frozen liver samples were thawed. A portion of the liver (1 g) and 3 ml of perchloric acid (3.5%) were homogenized and centrifuged at 3000 rpm for 15 min. The supernatant was neutralized with 10 N ammonium hydroxide to a pH of 4.8. Enzyme digestion to convert all phosphorylated ribavirin metabolites to ribavirin was accomplished by adding acid phosphatase (0.8 U/ μl) to the sample and incubating the mixture at 37°C. After digestion, the resulting mixture was purified using a NH2 SPE cartridge. The final extract is injected into the HPLC system to determine ribavirin concentration in liver extract (CitationLi-Tain et al., 2007). The amount of the drug in the liver was calculated as µg ribavirin per 1 g liver tissue.
Analysis of ribavirin level in the liver extract
Ribavirin in liver extract was quantified by a reported HPLC method (CitationAntonio et al., 2006) with slight modifications. The HPLC system was a Merck-Hitachi La Chrom (Tokyo, Japan). The system consisted of a pump model L-7100, an L-7200 auto-sampler, and L-7400 UV detector. D-7000 interface and HPLC system manager software (HSM, version 4.1, Merck Hitachi; Tokyo, Japan) were used for management of the HPLC system. Reversed phase column with phomenex-lichrosorb 5 RP-18 (250 mm × 4.6 mm I.D) column as a stationary phase (CA). The wavelength was set at 235 nm. The mobile phase used distilled water:acetonitrile at a proportion of 95:5% v/v. The mobile phase was prepared daily and degassed by a DGU-12A Shimadzu degasser. Analysis was run at a flow rate of 1.5 ml/min.
Calibration curve
A stock solution of ribavirin was prepared at a concentration of 10 µg/ml in HPLC-grade water. For calibration curve preparation, standard samples were prepared by transferring aliquots of standard solution to 1 ml of drug free liver homogenate to yield final ribavirin concentrations of 10, 20, 50, 100, 200, 300, 500, and 1000 ng/ml. The standard samples were subjected to the same extraction procedure as mentioned above. The calibration curve was determined by plotting the peak area of ribavirin against the ribavirin added in the blank liver homogenate sample. The peak areas of ribavirin and concentrations are linear (R2 = 0.997) over the tested concentrations range. The accuracy of the method was characterized by relative recoveries of ribavirin from rat liver extract ranging from 97.17–99.67% and 97.37–102.51% in the case of intra-day and inter-day recoveries, respectively, indicating the suitability of the method for the extraction of ribavirin from liver extract. Within- and between-day precision are within 0.19–4.6% relative standard deviation.
Statistical analysis
The data were reported as mean ± SD. Student’s t-test was performed to test the significance of the difference of the obtained results at a 5% level of significance (p < 0.05) using Microsoft Office 2003, Excel package.
Results and discussion
Image analyzer photographs
shows photographs of freshly prepared niosomal ribavirin dispersions as recorded by the image analyzer system. The prepared niosomes appeared as large unilamellar vesicles with spherical shape.
Size and size distribution
The average particle size of 90% of the particles and polydispersity index of the freshly prepared ribavirin niosomal formulations are shown in . The average particle size showed a narrow size distribution ranging from 0.85–1.01 μm. These results were in good agreement with the particle size of carboxyfluorescein niosomes prepared by using span 60 as a surfactant (CitationYoshioka & Florence, 1994). The average vesicle size of the niosomes prepared by using the molar ratios 1:1:0 and 1:1:0.1 are relatively larger than the molar ratio 4:2:0 and 4:2:1, this is due the relatively higher cholesterol content in the 1:1:0 and 1:1:0.1 molar ratios. CitationMcIntosh (1978) found that cholesterol increases the width of lipid bilayers and consequently increases the vesicle size. The calculated polydispersity index of all niosomal formulations ranged from 0.171–0.280. The polydispersity index was calculated according to CitationBhavana et al. (1998). A polydispersity index of 1 indicates large variations in particle size; a reported value of 0 means that size variation is absent (CitationRaymond et al., 2005). The obtained low values of polydispersity index of the prepared niosomes indicate a limited variation in particle size.
Table 1. Characterization of ribavirin niosomes.
Entrapment efficiency of ribavirin
The mean entrapment percentages of ribavirin by the different niosomes are shown in . It was clear that the mean entrapment percentage of ribavirin by niosomes formed using SP:CH:DCP in a molar ratio of 4:2:1 (14.4 %) is much higher than that of the same molar ratio without DCP (4.89%). This was apparently due only to the effect of surface charge. The same finding was noticed with the molar of SP:CH:DCP 1:1:0.1. The improvement in the drug entrapment of negatively charged niosomes (p < 0.05) may be due to two factors. First of all, the negatively charged lipid DCP might have increased the hydrophilicity of the bilayers of span 60 vesicles, thereby increasing the water intake of the bilayers of these vesicles, which resulted in higher entrapment of ribavirin as a water-soluble drug in comparison with DCP-free niosomes. This effect might increase the particle size and polydispersity of niosomes (CitationCarafa et al., 1998). Secondly, DCP increases the stability of niosome dispersion (CitationGianasi et al., 1997). The lower entrapment percentage of the neutral niosomes may be attributed to the instability of the prepared neutral niosomes. This may be due to the fact that cholesterol in the presence of DCP was more efficiently able to stabilize the structure of the niosomal membrane (CitationIjeoma & Vyas, 1998). The absence of DCP in the neutral niosomes might increase the membrane permeability and leakage of the drug from the niosomes and lead to decrease the entrapment efficiency of the neutral niosomes.
The effect of niosomes molar ratio on the entrapment percentage was investigated statistically by comparing each pair of niosomal formulations of different molar ratio and the same surface charge using t-test. The results indicated that negatively charged niosomes of the molar ratio (4:2:1) had a significantly (p < 0.05) higher entrapment percentage of ribavirin than the other molar ratio (1:1:0.1).
In-vitro release profile of niosomal ribavirin
illustrates the drug release profiles from the neutral and negatively charged niosomes. All niosomal ribavirin formulations had a plateau pattern after 9 h, except negatively charged niosomes of molar ratio (4:2:1). Furthermore, its release pattern was much lower than other niosomal formulations. The release data were analyzed mathematically according to zero-order, first-order, and Higuchi’s equations. The data were best fitted to Higuchi’s equation for both neutral and negatively charged niosomes of the two molar ratios with R2 = 0.968 and 0.975, respectively.
Figure 2. In vitro release profiles of ribavirin from niosomes of different molar ratios 1: 1: 0 (A), 4: 2: 0 (B), 1: 1: 0.1 (C) and 4: 2: 1 (D) of span 60: cholesterol: dicetyl phosphate.
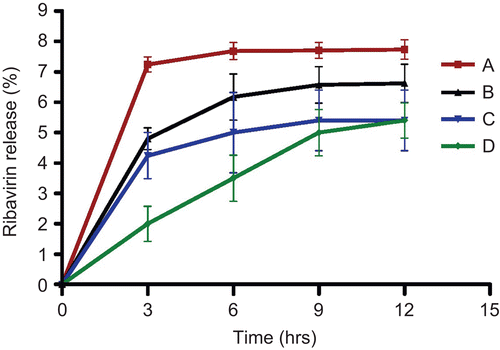
The negatively charged niosomes of both molar ratios released ribavirin to a lower extent than its comparable neutral niosomes. This may be attributed to higher stability of negatively charged niosomes than neutral niosomes (CitationCable, 1989). In addition, the repulsion forces between anionic entrapped ribavirin and the surface negative charge of DCP might hinder or decrease drug release from negatively charged niosomes. However, negatively charged niosomes of molar ratio (4:2:1) revealed a sustained characteristics as well as longer release pattern than other niosomal formulations. Therefore, negatively charged niosomes of molar ratio (4:2:1) were selected for in vivo study.
Stability study
The stability study on niosomes of molar ratio of 4:2:1 stored at refrigeration temperature of 4–8°C. The drug leakage from niosomes was restricted to just 2% of the encapsulated drug at the end of 6 months, indicating the stability of the prepared niosomal formulation at refrigerator.
Ribavirin liver targeting study
reveals that the mean ribavirin concentration in liver 4 h after administration of a single dose for niosomal ribavirin dispersion was much higher than that obtained from free ribavirin solution by ∼ 6-fold, where ribavirin concentration (mean ± SD µg/g of liver weight, n = 5) was 30.34 ± 6.25 µg/g and 4.78 ± 0.65 µg/g in the case of niosomal ribavirin dispersion and free ribavirin solution, respectively. These results were in a good agreement with that reported by CitationMeir et al. (1985); when liposomal ribavirin dispersion was administrated into female mice, the ribavirin concentration in liver after 1 h was increased by 5-fold than those attained with the same doses of free ribavirin solution.
Figure 3. Mean ribavirin concentration in rat liver 4 hrs after intraperitoneal administration of a single dose (30 mg/kg) of free ribavirin solution and niosomal ribavirin dispersion.
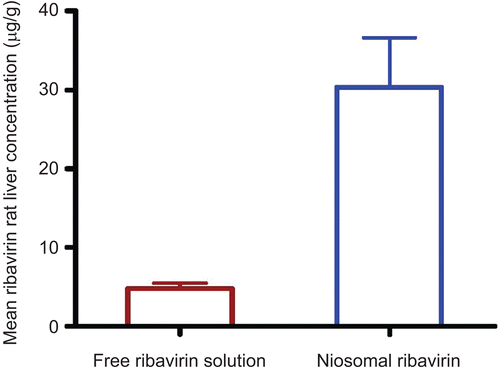
It was worthy to note that higher concentration of ribavirin reached the liver from niosomal ribavirin as compared to free ribavirin solution; this can be explained based on the propensity for niosomes of to be phagocytosed by macrophages of the liver (CitationAlving et al., 1978; CitationAlving, 1983). Since the viral pathogen HCV replicates in phagocytic cells (CitationLaskus et al., 2000; CitationMarek et al., 2004) and the major drug-related toxicity occurs outside the phagocytic cells, it is reasonable to expect that niosomes might increase drug efficacy without inducing toxicity. Therefore, the use of niosomes as a drug delivery system for ribavirin may enhance the efficacy of low doses of ribavirin and minimize the toxic side-effects.
Conclusion
The present study indicates that, the niosomal formulation significantly increased ribavirin liver concentration (6-fold) in comparison with ribavirin free solution. In conclusion, ribavirin niosomes have significant liver targeting properties. Based on the previous results, the use of niosomes as a drug delivery system for ribavirin is expected to improve the efficacy of low doses of ribavirin and minimize the toxic side-effects. However, further studies on the efficacy and safety of niosomal ribavirin formulation are required.
Declaration of Interest
The authors report no conflicts of interest. The authors alone are responsible for the content and writing of the paper.
References
- Alving, C.R. (1983). Delivery of liposome-encapsulated drugs to macrophages. Pharmacol Ther. 22:407–24.
- Alving, C.R., Steck, E.A., Chapman, W.L., Waits, V.B., Hendricks, L.G., Swartz, G.M., Hanson, W.L. (1978). Therapy of leishmaniasis: superior efficacies of liposome-encapsulated drugs. Proc Natl Acad Sci USA. 75:2959–63.
- Antonio, D., Avolio, A.I., Mauro, S., Marco, S., Daniel Gonzalez de Requena, S.B., Giovanni, D.P. (2006). Validation of Liquid/liquid extraction method coupled with HPLC-UV for measurement of ribavirin plasma levels in HCV-positive patients. J Chromatography B. 835:127–30.
- Arunothayanun, P., Bernard, M.S., Craig, D.M., Uchegbu, I.F., Florence, A.T. (2000). The effect of processing variables on the physical characteristics of non-ionic surfactant vesicles (niosomes) formed from hexadecyl diglycerol ether. Int J Pharm. 201:7–14.
- Arunothayanun, P., Turton, J.A., Uchegbu, I.F., Florence, A.T. (1999). Preparation and in vitro/ in vivo evaluation of leutenizing hormone releasing hormone (LHRH)-loaded polyhederal and spherical/tubular niosomes. J Pharm Sci. 88:34–8.
- Azmin, M.N, Florence, A.T., Handjani-Vila, R.M., Stuart, J.B., Van-lerberghe, G., Whittaker, J.S. (1985). The effect of non-ionic surfactant vesicle (niosome) entrapment on the absorption and distribution of methotrexate in mice. J Pharm Pharmacol. 37:237–42.
- Baillie, A.J., Florence, A.T., Hume, L.R., Muirhead, G.T., Rogerson, A. (1985). The preparation and properties of niosomes non-ionic surfactant vesicles. J Pharm Pharmacol. 37:863–8.
- Bhavana, V., Ajay, J.K., Jain, N.K. (1998). Proniosome based transdermal delivery of levonorgestrel for effective contraception. J Contr Rel. 54:149–65.
- Biju, S.S., Talegaonkar, S., Misra, P.R., Khar, R.K. (2006). Vesicular systems: an overview. Indian J Pharm Sci. 68:141–53.
- Cable, C. (1989). An examination of the effects of surface modifications on the physicochemical and biological properties of non-ionic surfactant vesicles. PhD Thesis, University of Strathclyde, Glasgow, UK.
- Carafa, M., Santucci, E., Alhaique, F., Coviello, T., Murtas, E., Riccieri, F.M., Lucania, G., Torrisi, M.R. (1998). Preparation and properties of new unilamellar nonionic/ionic surfactant vesicles. Int J Pharm. 160:51–9.
- Chandraprakash, K.S, Udupa, N., Umadevi, P., Pillai, G.K. (1990). Pharmacokinetic evaluation of surfactant vesicle-entrapped methotrexate in tumor-bearing mice. Int J Pharmacokinet. 61:R1–3.
- Chen-Chung, L., Li-Tain, Y., Trong, L., David, L., Johnson, Y.L. (2003). Pharmacokinetics and metabolism of 14 C ribavirin in rats and cyanomolgus monkeys. Antimicrob Agents Chemother. 47:1395–8.
- Deepika, A., Indu, P.K. (2005). Improved pharmacodynamics of timolol maleate from mucoahesive niosomal ophthalmic drug delivery system. Int J Pharm. 290:155–9.
- Gianasi, E., Cociancich, F., Uchegbu, I.F., Florence, A.T., Duncan, R. (1997). Pharmaceutical and biological characterization of a doxorubicin-polymer conjugate (PK1) entrapped in sorbitan monostearate Span 60 niosomes. Int J Pharm. 148:139–48.
- Hunter, C.A., Dolan, T..F., Coombs, G.H., Baillie, A.J. (1988). Vesicular systems (niosomes and liposomes) for delivery of sodium stibogluconate in experimental murine visceral Leischmaniasis. J Pharm Pharmacol. 40:161–5.
- Ijeoma, F.U., Vyas S.P. (1998). Non-ionic surfactant based vesicles (niosomes) in drug delivery. Int J Pharm. 172:33–70.
- Jen, J.F., Glue, P., Gupta, S., Zambas, D., Hajian, G. (2000). Population pharmacokinetic and pharmacodynamic analysis of ribavirin in patients with chronic hepatitis C. Ther Drug Monit. 22:555–65.
- Kaur, I.P., Kanwar, M. (2002). Ocular preparations: the formulation approach. Drug Dev Ind Pharm. 28:473–93.
- Larrat, S., Stanke-Labesque, F., Plages, A., Zarski, J.P., Bessard, G., Souvignet, C. (2003). Ribavirin quantification in combination treatment of chronic hepatitis C. Antimicrob Agents Chemother. 47:124–9.
- Laskus, T., Radkowski, M., Piasek, A. (2000). Hepatitis C virus in lymphoid cells of patients coinfected with human immunodeficiency virus type 1: evidence of active replication in monocytes/macrophages and lymphocytes. J Infect Dis. 181:442–8.
- Lindahl, K., Stahle, L., Bruchfeld, A., Schvarcz, R. (2005). High-dose ribavirin in combination with standard dose peginterferon for treatment of patients with chronic hepatitis C. Hepatology. 41:275–9.
- Li-Tain, Y., Mai, N., Sohelia, D., Wei, B., Chin-chung, L. (2007). LC-MS/MS method for simultaneous determination of viramidine and ribavirin levels in monkey red blood cells. J Pharm Biomed Anal. 43:1057–64.
- Marek, R., Agnieszka, B., Andrzej, H., Janusz, S., Jeffrey, W., Debra, M., Marek, N.R., Tomasz, L. (2004). Infection of primary human macrophages with hepatitis C virus in vitro: induction of tumour necrosis factor-a and interleukin 8. J Gen Virol. 85:47–59.
- McIntosh, T.J. (1978). The effect of cholesterol content on the structure of phosphatidylcholine bilayers. Biochim Biophys Acta. 51:43–58.
- Meir, K., Carl, R.A., Wayne, L.R., Glenn, M., Swartz, J.R., Peter, G.C. (1985). Enhanced efficacy of liposome-Encapsulated ribavirin against rift valley fever virus infection in mice. Antimicrob Agents Chemother. 27:903–7.
- Mitra, A.K. (2003). Ophthalmic drug delivery systems. 2nd ed. Mitra, A.K., ed. Drugs and the Pharmaceutical Sciences. New York: Marcel Dekker, Vol. 130.
- Patterson, J.L., Fernandez-Larsson, R. (1990). Molecular mechanisms of action of ribavirin. Rev Infect Dis. 12:1139–46.
- Raja, N.A., Singti, U.V., Udupa, N., Pillai, G.K. (1993). Anti-inflammatory activity of niosome encapsulated diclofenac sodium in rats. Indian Drugs. 30:275–8.
- Raymond, M., Josbert, M., Marcel, H., Adrienne, P., Grietje, M., Gert, S. (2005). Liposome-encapsulated prednisolone phosphate inhibits growth of established tumors in mice. Neoplasia. 7:118–27.
- Rogerson, A., Cummings, J., Florence, A.T. (1987). Adriamycin loaded niosomes: drug entrapment, stability and release. J Microencapsulation. 4:321–8.
- Rogerson, A., Cummings, J., Willmott, N., Florence, A.T. (1988). The distribution of doxorubicin in mice following administration in niosomes. J Pharm Pharmacol. 40:337–42.
- Schreier, H., Bouwstra, J. (1994). Liposomes and niosomes as topical drug carrier: dermal and transdermal drug delivery. J Contr Rel. 30:1–15.
- Strader, D.B., Wright, T., Thomas, D.L., Seeff, L.B. (2004). Diagnosis, management and treatment of hepatitis C. Hepatology. 39:1147–71.
- Yoshioka, T., Florence, A.T. (1994). Vesicle (niosome)-in-water-in-oil (v:w:o) emulsions—an in-vitro study. Int J Pharm. 108:117–23.