Abstract
In this study, carboxymethyl derivative of locust bean gum was prepared, characterized, and its gelling ability with different concentrations (1–5% w/v) of aluminum chloride (AlCl3) was utilized for the development of glipizide-loaded beads in a completely aqueous environment. The beads were spherical when observed under a scanning electron microscope. Increase in gelling ion concentration decreased the drug entrapment efficiency from 97.68% to 95.14%. The beads swelled more slowly in pH 1.2 KCl-HCl buffer and exhibited a slower drug release pattern than that observed in pH 7.4 phosphate buffer. Irrespective of the dissolution media, the drug release became slower at higher AlCl3 concentration. The drug release in alkaline medium was found to be controlled by a combination of diffusion as well as polymer relaxation phenomena. Comparing the release profiles, it was observed that the beads treated with 5% AlCl3 provided slower drug release up to 10 h in alkaline medium without any sign of disintegration and, thus, this formulation was selected for further studies. Fourier transform infrared (FTIR) spectroscopy indicated the stable nature of the drug in the beads. Differential scanning calorimetry and X-ray diffraction analysis showed that most of the drug remained in amorphous state in the beads. Stability study indicated no statistical significant difference in drug entrapment efficiency of the beads. In vivo activity of the beads was tested and a prolonged hypoglycemic effect was achieved. Hence, carboxymethyl locust bean beads could be a potential carrier for controlled oral delivery of glipizide.
Introduction
Various synthetic polymers have been used to develop multi-unit microparticulate dosage forms using solvent evaporation techniques. These techniques, although popular, are based on organic solvents. Possible toxicity in chronic dosing due to the presence of even traces of organic solvents in the dosage forms, the flammability, the environmental associated with the use of large volume of organic solvents coupled with stringent governmental regulation that restricts their use, have put in question its long-term viability (CitationHalder et al., 2005b). Natural gums are promising biodegradable materials and these can be chemically modified to have tailor-made materials for drug delivery systems. In addition, they are non-toxic, freely available, and less expensive compared to their synthetic counterparts (CitationBhardwaj et al., 2000). Additionally, they do not require organic solvents for their processing (CitationVyas & Khar, 2002). Therefore, in the years to come, there is going to be continued interest in the natural polysaccharides and their modifications, with the aim to have a microparticulate carrier system for controlled oral drug delivery. Among the natural polysaccharides, sodium alginate has been studied extensively for the preparation of drug-loaded calcium alginate beads for controlled oral delivery (CitationKikuchi et al., 1999; Arica et al., 2002; Citation2005; CitationYegin et al., 2007; CitationDas & Senapati, 2008). Although alginate beads do not swell appreciably in acidic fluid, the beads swell and erode/disintegrate rapidly in simulated intestinal fluids, leading to quick release of the loaded drugs (CitationTomida et al., 1993; CitationAcarturk & Takka, 1999; CitationAnal et al., 2003) and, hence, calcium alginate beads alone do not seem suitable as an oral controlled release system. Further, coating of alginate beads with polyelectrolytes such as poly-l-lysine (CitationQuong et al., 1999; CitationUeng et al., 2000; Citation2006), polyethyleneimine (CitationHalder et al., 2005a), and chitosan (CitationCoppi et al., 2001; CitationAnal & Stevens, 2005) has been investigated for the controlled delivery of drugs. However, these had a limited degree of success. Chitosan beads have been fabricated by ionic cross-linking with sodium tripolyphosphate (CitationAydin & Akbuğa, 1996; CitationSrinatha et al., 2008); however, higher porosity of the beads led to rapid release of drug in acidic dissolution medium (CitationGupta & Ravi, 2000). CitationWin et al. (2003) developed phosphorylated chitosan beads of ibuprofen by ionotropic gelation with counter polyanion, tripolyphosphate at pH 4.0. The drug release in alkaline dissolution medium (pH 7.4) was noticeably higher than the release in acidic medium (pH 1.4) due to the ionization of phosphate groups and high solubility of drug in alkaline medium. Furthermore, water-soluble derivatives of chitosan including N-carboxymethyl chitosan (CitationJayakumar et al., 2006) and thiol-containing chitosan (CitationJayakumar et al., 2007) was synthesized for the development of indomethacin-loaded gelled beads. However, they reported similar findings to that observed with phosphorylated chitosan beads. Even the use of pectin beads has some drawbacks due to their rapid in vitro drug release (CitationBodmeier et al., 1989; CitationSriamornsak et al., 1997).
Native guar gum and xanthan gum could not form gelled beads in the presence of divalent or trivalent metal ions. However, chemical modification of these gums via carboxymethylation led to the formation of drug-loaded gelled beads. It was reported that barium chloride cross-linked carboxymethyl guar gum beads had a maximum bovine serum albumin (BSA) loading efficiency of 53% depending upon the concentration of barium (Ba+2) ions and released only 5% of the encapsulated BSA in pH 1.2 NaCl-HCl buffer in 6 h; however, the beads released almost 81% of its content within 4 h in pH 7.4 TRIS-HCl buffer (CitationThimma & Tammishetti, 2001). Recently, it has been reported that diltiazem-resin complex-loaded carboxymethyl xanthan beads released ∼ 75–82% drug in simulated gastric fluid within 2 h and 75–98% drug in simulated intestinal fluid within 5 h (CitationRay et al., 2008). It appears that each and every polymeric bead system has its merits and demerits; and none of these is a suitable carrier system for oral controlled drug delivery. Hence, there is a need to screen other natural polysaccharides for the development of polymeric bead systems which could overcome the problems associated with such existing systems.
Locust bean gum is a high molecular weight branch polysaccharide and is extracted from the seeds of carob tree Ceratonia siliqua. It consists of a (1, 4)-linked β-D-mannopyranose backbone with branch points from their 6-positions linked to α-D-galactose (1, 6-linked α-D-galactopyranose) (CitationDea & Morrison, 1975). This galactomannan occurs as a white to yellow-white powder, odorless and tasteless, but acquires a leguminous taste when boiled in water. It is less soluble in water and needs heating to dissolve. Being non-ionic, it is not affected by pH or ionic strength. It is dispersible in either hot or cold water, forming a sol having a pH between 5.4–7.0, which may be converted to a gel by the addition of small amounts of sodium borate. Its film-forming property is used in the textile industry, making it ideal as a sizing and finishing agent, as well as in the pharmaceutical and cosmetics industries for the production of lotions and creams (CitationNisperos-Carriedo, 1994). It also finds its application as excipients for tablets (CitationVenkataraju et al., 2007). Moreover, this ingredient is approved for food uses by the US Food and Drug Administration (FDA). In the present investigation, locust bean gum was chemically modified to alter its physicochemical properties, e.g. primary structures, hydrophilicity, polyelectrolytic nature, solution rheology, and gel forming behavior, such that specific functional properties can be improved (CitationSierakowaski et al., 2000; CitationParvathy et al., 2005). Carboxymethylation is a well established chemical treatment of polysaccharides to convert the hydroxyl groups into carboxymethylate. Thus, the objective of our present investigation was to synthesize carboxymethyl derivative of locust bean gum and develop metal ion cross-linked carboxymethyl locust bean hydrogel beads as a possible carrier system for oral controlled drug delivery.
Glipizide is an oral hypoglycemic agent, which is a commonly prescribed drug for the treatment of patients with type 2 diabetes mellitus (CitationVerma & Garg, 2004). Chemically, it is 1-cyclohexyl-3-[[p-[2-(5-methylpyrazine-carboxamido) ethyl] phenyl] sulfonyl] urea. It is a weak acid (pKa = 5.9), practically insoluble in water and acidic environment, and highly permeable (Class II drugs in accordance to Biopharmaceutics Classification System, BCS) (CitationJamzad & Fassihi, 2006). The oral absorption of glipizide is uniform, rapid, and complete with a bioavailability of nearly 100% (CitationJamzad & Fassihi, 2006), and is reported to have a short biological half-life (3.4 ± 0.7 h) requiring it to be administered in two-to-three doses of 2.5–10 mg per day (CitationFoster & Plosker, 2000). The World Health Organization (WHO) has recommended glipizide as a first-line treatment in type 2 diabetes patients who are not very obese. Many researchers have formulated oral controlled-release products of glipizide by various techniques. Alginate beads/microcapsules with other mucoadhesive polymers like methylcellulose, sodium carboxymethyl cellulose, or carbopol exhibited sustain drug release properties over a period of 10–12 h (Chowdari & Rao, 2003; CitationSanap, 2009). Glipizide has been selected in the development of other controlled release formulations such as glutaraldehyde-treated chitosan (CitationPatel et al., 2005) and galactomannan gum microspheres (CitationGaba et al., 2010), mucoadhesive matrix tablets (CitationChowdary & Sundari, 2003), and buccal films (CitationSemalty et al., 2008). Therefore, glipizide is an ideal candidate for incorporation into the present controlled release polymeric bead system.
Materials and methods
Materials
Glipizide was a gift from Micro Labs Limited (Hyderabad, India). Locust bean gum was purchased from HiMedia Laboratories Pvt. Ltd. (Mumbai, India). Aluminium chloride, sodium hydroxide, acetic acid, D-glucose, and potassium dihydrogen phosphate were obtained form Qualigens Fine Chemicals (Mumbai, India). Monochloroacetic acid, Tween 80, and alloxan were supplied by Loba Chemie Pvt. Ltd. (Mumbai, India). Barium chloride dihydrate and calcium chloride dihydrate were procured form Merck Specialties Pvt. Ltd. (Mumbai, India), and Merck Ltd. (Mumbai, India), respectively. All other regents were of analytical grade and used without further purification.
Synthesis of sodium carboxymethyl locust bean gum
Locust bean gum was derivatized to sodium carboxymethyl locust bean gum with slight modification of the method reported earlier (CitationSetty, 2005). Accurately weighed, 2.2 g of locust bean gum was dispersed in 50 ml methanol and stirred magnetically for 2 h to remove the organic impurities. Then it was filtered through Whatmann filter paper with pore diameter 11 µm and dried. One gram of this dried powder was mixed with 4 ml water heated to 80°C for 15 min and cooled. Then, 2.72 ml 55.59% (w/v) solution of NaOH in ice-cold water was added dropwise over a period of 45 min. Monochloroacetic acid (750 mg) dissolved in 1.66 ml of water was added slowly for a period of 1 h to the above mixture maintained at 15°C. The temperature of the mixture was raised slowly to 65°C and stirred at least for another 1 h. The wetted mass was washed with three successive amounts of 20 ml 80% (v/v) methanol/water mixture for 15 min. The pH of the suspension was adjusted to neutrality with glacial acetic acid. Finally it was washed with methanol and kept for drying at 50–60°C until three consecutive weights were the same.
Determination of degree of O-carboxymethyl substitution
Five hundred milligrams of carboxymethylated locust bean gum was dispersed in 5 ml 80% (v/v) methanol/water mixture. Concentrated hydrochloric acid was added in excess amount and stirred for 2–3 h. The mixture was filtered through Whatmann filter paper with pore diameter 11 µm and the residue was washed with successive 5 ml of methanol until washing shows neutrality. The residue was dried to constant weight.
Accurately weighed, 200 mg of dried sample was taken in a beaker; 1.5 ml of 70% (v/v) methanol/water mixture was added and allowed to stand for a few minutes. Then 20 ml of water and 5 ml of 0.5N NaOH were added. The mixture was shaken for 3–4 h until the sample dissolved completely. The solution was then back titrated with 0.4 N HCl to a phenolphthalein end-point. The degree of substitution (DS) of O-carboxymethyl group was calculated from the equation (CitationMaiti et al., 2007): DS = 0.162A/(1 − 0.058A), where ‘A’ is miliequivalents of NaOH required per gram of sample.
Fourier transform infrared (FTIR) spectroscopy
The introduction of O-carboxymethyl functionalities into native locust bean gum was further identified by Fourier transform infrared spectroscopy. The FTIR spectra of native locust bean gum and derivatized gum were recorded in a Perkin Elmer FTIR Spectrometer (Spectrum RX1, UK) from 4000–500 cm−1 using KBr pellets. The pellets were made by applying a pressure of 1000 kg/cm2 to a mixture of gum and KBr (1:20) for 10 min in a hydraulic press (KP, Kimaya Engineers, India).
Toxicity study
The toxicity study of carboxymethylated locust bean gum (if any) was conducted on 12 Swiss Albino Mice with an average weight of 20 g. The necessary permission was obtained from the Institutional Animal Ethics Committee (Registration no. 955/A/06/CPCSEA). The animals were fasted for 12 h before oral administration of the derivatized gum. The animals received the gum (1 g/kg body wt) in viscous liquid condition by gavages and were monitored for 24 h. No death or abnormal behavior was observed. The animals then had free access to basal diet mixed with the gum (1:1) and water and they were observed for 1 month. No death or abnormal behavior was noticed (CitationMukherjee et al., 2006).
Measurement of viscosity
The viscosity of locust bean gum and sodium carboxymethyl locust bean gum (0.1% w/v) was determined by a programmable Brookfield viscometer (Model DV-II+ Pro, Brookfield Engineering Labs., Inc. Middleboro, MA) at 32.7°C. The locust bean gum was dissolved in hot water and conditioned at 32.7°C. The spindle (Spindle no. CPE 41) was rotated at 0.5 rpm.
Preparation of blank carboxymethyl locust bean hydrogel beads
Four hundred milligrams of sodium carboxymethyl locust bean gum was dissolved in 10 ml of double distilled water and the gum solution (pH 7.0) was added dropwise through a 21-gauge flat-tipped needle into 100 ml of aqueous metallic salt solutions containing 0.08% (w/v) Tween 80. Different metallic salts were used in different concentrations (w/v): calcium chloride dihydrate (5%), barium chloride dihydrate (5%), and aluminium chloride hexahydrate (1%, 3%, and 5%). Following an incubation period of 15 min, the ability of different salt solutions to form isolatable, self-standing gelled beads were examined. The hydrogel beads were formed instantaneously in the presence of trivalent Al+3 ions at all concentrations. The beads were filtered off, washed with distilled water (3 × 50 ml), and air-dried.
Preparation of glipizide-loaded carboxymethyl locust bean hydrogel beads
Glipizide (30%w/w) was dispersed uniformly into 10 ml 4% (w/v) solution of sodium carboxymethyl locust bean gum solution (pH 7.0). The dispersion was added dropwise through a 21-gauze flat-tipped needle into slightly agitated 100 ml of aqueous aluminum chloride (AlCl3) solution: 1, 3, and 5% (w/v), containing 0.08% (w/v) Tween 80. Following an incubation period of 15 min, the beads were isolated by filtration, washed with double distilled water (3 × 50 ml), and air-dried to constant weight.
Estimation of percentage yield
The percentage yield of the beads was calculated using the formula (CitationPatil et al., 2006):
Scanning electron microscopy (SEM)
The dried blank beads and drug-loaded beads were examined under a scanning electron microscope (JEOL-JSM-6360, serial No: G5/IL/42/08, JEOL Datum Ltd, Japan). SEM photographs of uncoated samples were taken at an acceleration voltage of 20 KV and at a chamber pressure of 0.6 mm Hg. The samples were just placed on NEM TAPE adhesive paper and photographs were taken.
Particle size analysis
The size of dried glipizide-loaded locust bean beads was measured using an optical microscope (Olympus Model HB, India). A standard stage micrometer was used to calibrate the eye-piece micrometer. Dried beads were placed in a glass slide and the number of divisions of the calibrated eye piece was counted. A hundred beads were randomly selected from each formulation and the individual particle diameter was calculated based on this formula: 1 eyepiece division = [(no of stage micrometer divisions/no of eyepiece micrometer division) × 10 μm]. In this analysis, an optical combination of 10× eye-piece and 10× objective was used. The arithmetic mean particle diameter and standard deviation were calculated using Microsoft Excel 2002 software (CitationCapan et al., 2003; CitationMaiti et al., 2009; CitationManjanna et al., 2009).
Estimation of drug entrapment efficiency
Accurately weighed, 20 mg of dried beads were crushed with the help of a mortar and pestle and were transferred into 100 ml of pH 7.4 phosphate buffer solution. After 12 h, the suspension was filtered and the samples were analyzed with a spectrophotometer (UV1, Thermo Spectronic, UK) at λmax = 276 nm. Higher time was allowed to affect the maximum drug release. All samples were analyzed in triplicate. The drug entrapment efficiency (%) was calculated following the equation (CitationKulkarni & Sa, 2009): Entrapment efficiency (%) = (actual drug content/theoretical drug content) × 100.
In vitro drug release study
In vitro release of glipizide from CMLBG beads was studied using a paddle-type dissolution tester (VDA-6D, Veego Instruments Corporation, Mumbai, India). Accurately weighed, 10 mg of dried beads was placed in 900 ml pH 7.4 phosphate buffer solution and maintained at 37 ± 0.5°C. The paddle was rotated at 50 rpm. The beads were lying at the bottom of the vessel. In order to provide hydration and swelling of the beads in all directions, a single ring-mesh device was placed within the vessel (CitationDurig & Fassihi, 2000). Five milliliters of aliquot was withdrawn from dissolution medium at specified time intervals and the same volume of fresh medium was replenished immediately. The samples were analyzed spectrophotometrically (UV1, Thermo Spectronic, UK) at 276 nm, without filtration and further dilution. The in vitro drug release study of the samples (50 mg) in pH 1.2 KCl/HCl buffer solution was carried out under the same experimental condition for 2 h. Cumulative percentage of glipizide released in the respective dissolution medium was plotted as a function of time. One sample was tested and analyzed in triplicate.
Drug release mechanism
The mechanism of in vitro glipizide release from the beads was determined from the values of diffusional exponent (n) obtained by modeling the first 60% of the drug release into Korsmeyer-Peppas equation (CitationKorsmeyer et al., 1983): Mt/M∞ = ktn, where Mt/M∞ is the fractional solute release at time t, and k is a constant which incorporates the structural and geometric characteristics of the device. The mechanism of drug release from spherical polymeric devices may be Fickian diffusion when the value of n = 0.43 or less, anomalous (non-Fickian) transport when the value of n lies between 0.43–0.85, and case II transport when n = 0.85. An exponent value of n greater than 0.85 signifies a super case II transport mechanism (CitationRitger & Peppas, 1987).
Swelling study
The swelling behaviors of blank beads cross-linked with different concentrations of AlCl3 (1–5% w/v) were studied in pH 1.2 KCl/HCl buffer and pH 7.4 phosphate buffer solution for 1.5 h. Samples of known weight (10 mg) were exposed to 100 ml of the swelling medium and allowed to swell. The swollen beads were periodically removed, blotted with tissue paper, and weighed (Metler Toledo, AB 204-S, Switzerland). The swelling ratios were calculated following the equation (CitationRoy et al., 2009): Swelling ratio = (Final weight − Initial weight)/Initial weight.
Drug–polymer interaction study through FTIR spectroscopy
FTIR spectra of pure glipizide, physical mixture of glipizide and CMLBG, glipizide-loaded CMLBG beads, and blank CMLBG beads were recorded in a Perkin Elmer IR Spectrophotometer using KBr pellets. The changes in characteristic peaks of pure glipizide were noted in the physical mixture as well as in the beads.
Differential scanning calorimetry (DSC)
DSC thermograms of pure glipizide, physical mixture of glipizide and excipients, glipizide-loaded and blank CMLBG beads were recorded using Perkin-Elmer instrument (Pyris-Diamond TG/DTA, Osaka, Japan). Each sample (3–7 mg) was accurately weighed into a 50 µl aluminium pan in a hermetically sealed condition. The measurements were performed in an atmosphere of nitrogen (20 ml/min) between 30–500°C at a heating rate of 10°C/min.
X-ray diffraction study
Ground samples of pure glipizide, physical mixture of glipizide and excipients, and glipizide-loaded CMLBG beads were scanned from 10–60° 2θ, using a wide angle X-Ray Diffractometer (Ultima III, model: D/Max 2200, Rigaku Corporation, Japan), under the following measurement conditions: source: Cu-Kα radiation; voltage: 40 kV; current: 30 mA; scan speed: 5° per min.
Stability study
The optimized formulation was kept in a stability chamber at a temperature of 40 ± 2°C and a relative humidity of 75 ± 5% for 3 months. The drug content of the beads was determined at the end and compared with the data before stability testing.
In vivo anti-diabetic activity
In vivo anti-diabetic activity of glipizide-loaded beads was performed on normal healthy male Wistar rats weighing 250–300 g each. The approval of the Institutional Animal Ethics Committee (Registration no. 955/A/06/CPCSEA) was obtained before starting the study. The animals were divided into two groups; each consisting of five animals. The animals were fasted (with water) at least 12 h before the conduction of the study. On the day of experiment, normal blood glucose levels of the animals were measured. Experimental diabetes was induced by a single intraperitoneal injection of alloxan 120 mg/kg BW dissolved in normal saline. Rats were maintained on 5% glucose solution to prevent hypoglycemia for 5 days. Blood samples were taken from tail veins 5 days after the injection of alloxan to confirm the induction of diabetes. Group I served as control and received only distilled water; Group II received glipizide-loaded beads. The beads (800 µg/kg BW) were administered orally using stomach intubations. Blood samples were collected at 1 h intervals up to 12 h and the blood glucose levels were measured using Accu-Chek Sensor comfort (Roche Diagnostics, Germany) test strips. The percentage reduction in blood glucose level was measured.
Statistical analysis
The differences in drug entrapment efficiency and release rate from the beads prepared at different concentrations of AlCl3 were evaluated by one way analysis of variance (ANOVA): single factor, using Microsoft Excel 2002 software. Differences were considered significant when p < 0.05. The difference between drug entrapment efficiencies, before and after stability testing of the optimized bead formulation, was analyzed by paired t-test using Microsoft Excel 2002 software. The effect of temperature and humidity on drug stability was considered significant when the t stat value was greater than t critical two-tail value at alpha level of 0.05 (p two-tail < 0.05).
Results and discussion
In the present investigation, a natural polysaccharide, locust bean gum (LBG) was chemically modified to sodium carboxymethyl locust bean gum (CMLBG) and characterized with respect to its degree of O-carboxymethyl substitution, toxicity, aqueous solubility, solution rheology, and gel-forming behaviors in the presence of divalent or trivalent metal ions. Finally, its gel-forming ability with trivalent aluminium ions (Al+3) was utilized for the development of a novel polymeric bead system for controlled oral delivery of glipizide.
The primary and secondary hydroxyl groups of LBG are amenable to etherification with carboxymethyl groups. In carbohydrate chemistry, the level of modification is expressed in terms of degree of substitution, which is the average number of newly substituted O-carboxymethyl groups per anhydro sugar unit. The average degree of O-carboxymethyl substitution in LBG was found to be 0.45 ± 0.05. It has been stated that the relative reactivity of three hydroxyls, one primary on the C-6, and two secondary on the C-2 and C-3 of each sugar, are not the same due to hindrance from involving in hydrogen bonding (CitationYalpani, 1999). The use of strong sodium hydroxide (> 18%) solution promoted decrystallization, allowing penetration of the modifying agent into the interlocking polymer chain and subsequent etherification of the polysaccharide (CitationYalpani, 1999). However, at higher concentration of alkali, probably O-6 was the most reactive species as the trend was towards the formation of relatively strong adducts of vicinal hydroxyl groups, rather than the generation of oxyanions (CitationChen et al., 2003) and probably led to such low O-carboxymethyl substitution in LBG.
Further to confirm the substitution of O-carboxymethyl groups in the native LBG, FTIR analysis was performed. represents the FTIR spectrum of LBG and CMLBG. A broad, very strong O-H stretching of hydroxyl group was identified in both LBG and CMLBG at 3433.86 cm−1 and 3462.00 cm−1, respectively (CitationParvathy et al., 2005). The vibrations related to –CH2 bending/wagging was detected in the spectrum of both LBG and CMLBG at frequencies of 2926.96 cm−1 and 2957.54 cm−1, respectively. The band at ∼ 1000–1100 cm−1 was mainly attributed to C-O-H stretching/bending, and was also identified in the derivative without marked changes. The presence of carboxymethylated groups in CMLBG was demonstrated at 909.82 cm−1, representing symmetric C-O-C stretching (CitationParvathy et al., 2005). In CMLBG, –CH2 stretching vibration was also increased (1325.13 cm−1) compared to LBG (1306.87 cm−1). In addition, while the C-O stretching of ether and C = O stretching of acid were observed in derivatized gum, respectively, at 1607.43 cm−1 and 1451.77 cm−1 (CitationVogt et al., 1995; CitationZhbankov et al., 1997; Thygesen et al., Citation2003), the same were absent in LBG. Thus, it can be interpreted that O-carboxymethyl groups have been introduced into LBG. The chemical structure of CMLBG has been proposed in . Toxicological study on animal model revealed that CMLBG was non-toxic in nature. It is well known that LBG is only slightly soluble in water at room temperature. However, CMLBG became soluble in distilled water at room temperature and a clear solution was achieved. The improvement in aqueous solubility could be attributed to the hydrophilic nature of carboxylate functional groups of CMLBG. The viscosity of 0.1% (w/v) CMLBG solution was lower (89.8 cP) than that of LBG (119.7 cP). Improved physical properties coupled with non-toxic nature of this particular derivative stimulated our interest to develop a controlled drug delivery device. In an attempt to develop a microparticulate device of CMLBG, its gelling ability in the presence of various divalent or trivalent metal ions was assessed. When 4% (w/v) solution of CMLBG was added dropwise into calcium chloride and barium chloride solutions, no gelled beads were formed even at a salt concentration of 5% (w/v). However, in the presence of trivalent Al+3 ions (supplied in form of aluminium chloride: 1, 3, and 5 % w/v), it formed water-insoluble, self-standing gelled beads. Scanning electron microscopy revealed that the blank beads were spherical in shape, having smooth surfaces at all concentrations of aluminium chloride. A representative photograph has been displayed in . The drug-loaded beads were prepared using different concentrations of AlCl3 and were found to be more or less spherical, having wavy surfaces (). The percentage yield of drug-loaded beads was good and ranged from 93.35–94.80% (). The drug entrapment efficiency of the beads decreased with increasing concentration of AlCl3 in the gelation medium (), and such change was statistically significant (p < 0.05). A similar decrease in drug entrapment efficiency of Al+3 ion cross-linked carboxymethyl guar gum beads was reported (CitationReddy & Tammishetti, 2002). Another report indicated that higher concentration of Ca+2 ions decreased the drug encapsulation efficiency of gellan gum beads in a nearly proportional manner (CitationSingh & Kim, 2005). However, the arithmetic mean diameter of the beads increased from 1.24 × 103 µm to 1.27 × 103 µm with the increase in concentration of AlCl3 in the gelation medium ().
Table 1. Effect of AlCl3 concentration of percentage yield, particle size, and drug entrapment efficiency of CMLBG beads loaded with 30% (w/w) glipizide.
Figure 3. Scanning electron micrographs of blank and glipizide-loaded CMLBG bead. Key: Concentration of aluminium chloride: (a) 5% (blank bead); (b) 1%; (c) 3%; (d) 5%.
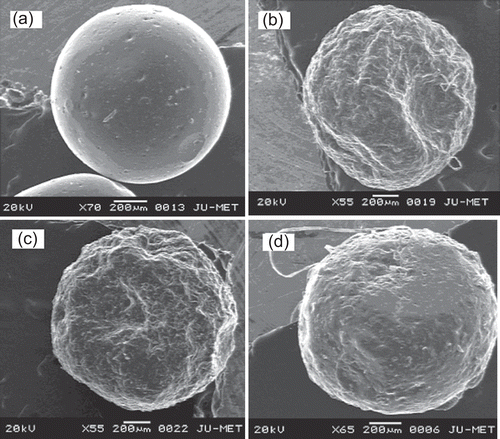
depicts the drug release profiles of the CMLBG beads in pH 1.2 KCl-HCl buffer and pH 7.4 phosphate buffer solutions. The beads incubated in 1% AlCl3 released its content in acidic dissolution medium at a faster rate than those incubated in 3% and 5% AlCl3 solution. However, the beads incubated in 3% and 5% AlCl3 solution showed a marginal difference in their release profiles in the same medium. For brevity, while the drug release from the beads incubated in 1% AlCl3 solution was 17.25 ± 0.27 (%) in 2 h, the same were 9.89 ± 1.36 (%) and 7.35 ± 0.24 (%), respectively, for the beads incubated in 3% and 5% AlCl3 solution. The time point approach was adopted to compare drug release rate of the beads in pH 7.4 phosphate buffer solution as a function of AlCl3 concentration. The t50% and t80% values (i.e. time required for the release of 50% and 80% drug, respectively) have been cited in . It was clear that the drug release in alkaline dissolution medium became slower at higher concentrations of AlCl3. The differences in their t50% and t80% values were statistically significant (p < 0.05), and thus indicated that the concentration of AlCl3 had an impact in retarding the drug release from the beads in alkaline dissolution medium. Although higher concentration of AlCl3 provided slower drug release rate in alkaline dissolution medium, the drug release in this medium could be extended up to 10 h, irrespective of the concentration of AlCl3. Again, the drug release rate from the beads was much faster in alkaline dissolution medium than in acidic medium, when compared up to 2 h. Within the same time frame of 2 h, the drug release in alkaline dissolution medium was found to be 45.09 ± 4.92 (%), 31.98 ± 4.21 (%), and 28.94 ± 1.59 (%) for the beads incubated in 1%, 3%, and 5% (w/v) AlCl3 solution, respectively. To explain such differences in drug release behaviors in different dissolution media, swelling studies were performed with the blank beads (). The beads swelled more rapidly in alkaline dissolution medium than in acidic medium, and this led to higher drug release in alkaline medium. In alkaline medium, probably a large swelling force was created by the electrostatic repulsion between the ionized acid groups (CitationDu et al., 2006). An inverse relationship was observed between AlCl3 concentration and the swelling ratio of the beads. The drug release rate of CMLBG beads treated with higher concentrations of AlCl3 was always less than those prepared with lower concentrations, irrespective of the dissolution media. DSC thermograms of the blank beads prepared with different concentrations of AlCl3 showed shift in enthalpy (δ) due to endothermic transitions (). The δH values of endothermic transitions were 149.52 J/g at 173.28°C, 155.91 J/g at 176.02°C, and 191.98 J/g at 146.0°C, respectively, for the beads cross-linked with 1%, 3%, and 5% (w/v) AlCl3. TGA curve stated that ∼ 15–20% loss in weight was observed in the early stages of heating up to 180°C and was due to water content of the polymeric beads. These endothermic transformations could not be thought of as the melting of the polymers. If it were, this would be an increase in the energy of the system and not a loss in weight due to the phase transition in the TGA curve. A loss in weight occurring at this temperature range proves the reduction in the water content of the polymer (CitationAydinli et al., 2004). As regards to enthalpy data, it was apparent that with increase in AlCl3 concentrations, the cross-linking density was also increased and, thus, caused lower swelling of the beads and made diffusion of the drug to the dissolution media more difficult. CitationAydinli et al. (2004) reported that LBG polymer is resistant to heat up to 300°C and that above that temperature it decomposes exothermically. It is interesting to note that no exothermic transition occurred in DSC thermograms of the cross-linked CMLBG polymers, which is therefore thermally more stable than native LBG.
Table 2. Release characteristics and mathematical modeling of the release data of glipizide-loaded CMLBG beads in pH 7.4 phosphate buffer solution.
Figure 4. Release profiles of glipizide-loaded beads in different dissolution media. Drug release in pH 7.4 phosphate buffer solution; Key: Concentration of aluminium chloride: (Δ) 1%; (□) 3%; (○) 5%. Drug release in pH 1.2 KCl-HCl buffer solution; Key: Concentration of aluminium chloride: (▴) 1%; (▪) 3%; (•) 5%.
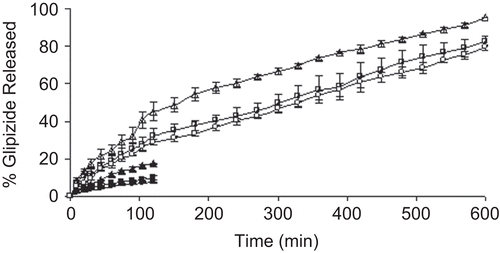
Figure 5. Swelling study of blank beads in different dissolution media. Swelling study in pH 1.2 KCl-HCl buffer solution; Key: Concentration of aluminium chloride: (Δ) 1%; (□) 3%; (○) 5%. Swelling study in pH 7.4 phosphate buffer solution; Key: Concentration of aluminium chloride: (▴) 1%; (▪) 3%; (•) 5%.
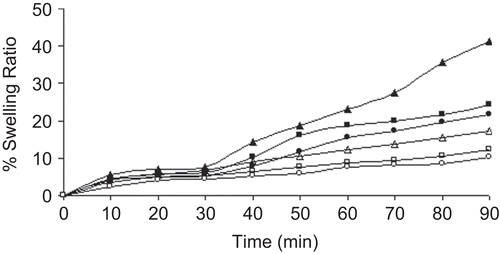
Figure 6. DSC thermograms and TGA curves of blank beads prepared using different concentrations of aluminium chloride. Key: Concentration of aluminium chloride: (a) 1%; (b) 3%; (c) 5%.
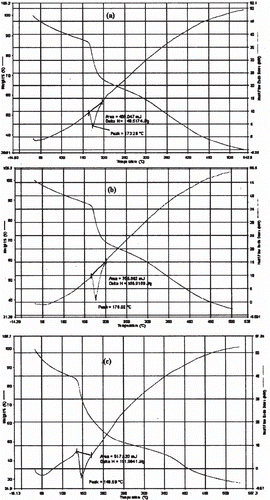
The release data obtained in pH 7.4 phosphate buffer solution were fitted into Korsmeyer-Peppas equation to evaluate the drug release mechanism. The drug release from the beads followed non-Fickian or anomalous transport mechanism because the values of diffusion exponent were between 0.43–0.85 (). That is to say, the drug release was controlled by a combination of diffusion as well as polymer relaxation phenomena. Hence, the concentration of AlCl3 had no effect in modifying drug release mechanism in alkaline dissolution medium.
Comparing the dissolution profiles of CMLBG beads, it was decided that a slower drug release profile could be achieved at the highest AlCl3 concentration. To simulate gastrointestinal conditions, these beads were transferred into pH 7.4 phosphate buffer solution following dissolution in pH 1.2 KCl-HCl buffer solution for 2 h. The drug release was suddenly increased from 7.35 to 22.53% in 10 min; however, the beads released its content gradually up to 10 h without any sign of disintegration (). For this reason, these beads were considered most suitable for the controlled release of glipizde, and further studies were conducted with this optimized formulation. IR stretching bands of glipizide were observed at 1689.0 cm−1 (C = O), 1650.0 cm−1 (-CONH-), 1444.40 cm−1 (substituted cyclohexane), 1159.41cm−1 (sulfate group), and 606.21 cm−1 (disubstituted benzene) (CitationTiwari et al., 2008). These bands were also identified in both the physical mixture and drug-loaded beads with minor differences in frequencies (). This suggested that there was no interaction between drug and polymers.
Figure 7. Release profile of glipizide-loaded beads (5% aluminium chloride) in pH 7.4 phosphate buffer solution following dissolution in pH 1.2 KCl/HCl buffer solution for 2 h.
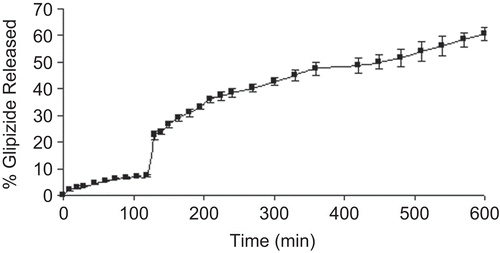
Figure 8. FTIR spectra of (a) blank beads; (b) pure glipizide; (c) glipizide-loaded beads; (d) physical mixture of glipizide and CMLBG.
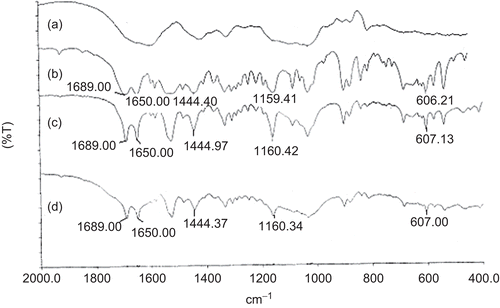
The physical state of the drug was examined through DSC and XRD analysis. Glipizide exhibited a sharp endothermic peak at 214.08°C, corresponding to its melting transition point (). The physical mixture showed two endothermic transitions peaking at 207.48 and 252.6°C. The polymer might have inhibited melting of some drug crystals close to its reported melting point and, hence, a weaker melting endotherm appeared at 252.6°C. On the other hand, the drug-loaded formulation exhibited a strong endotherm at 151.32°C. The endothermic activity at 151.32°C probably resulted from the evaporation of the water. However, a very weak melting endotherm of drug appeared at 236.3°C. XRD patterns of pure glipizide, physical mixture, and drug-loaded beads have been given in . XRD pattern of pure glipizide showed characteristics intense signals at diffraction angles (2θ) of 10.980, 15.620, 16.900, 18.640, 19.200, 21.780, 22.140, 23.540, 25.260, and 28.740 with their relative intensities (cps) of 1922, 7221, 4027, 5591, 1341, 2715, 2864, 1648, 2537, and 2208, respectively. The relative intensities of the signals related to pure glipizide were either reduced or absent at these diffraction angles in physical mixture as well as in drug-loaded CMLBG beads. In particular, drug-loaded beads showed very poor signals of pure crystalline glipizide. Thus, the DSC data coupled with XRD data suggested that most of the glipizide remained in amorphous form in the CMLBG beads and was thoroughly dispersed in the beads. To evaluate the drug stability in this optimized formulation, the samples stored at elevated conditions were analyzed for drug content. The drug entrapment efficiency of this formulation decreased from 95.14 ± 1.01 (%) to 94.37 ± 1.21 (%) after 3 months. Although the drug entrapment efficiency slightly decreased, statistically no significant difference was observed (p two-tail > 0.05). It is noteworthy that there was no change in physical appearance of the optimized bead formulation.
Figure 9. DSC thermograms of pure glipizide (bold dotted line), physical mixture of drug and polymer (faint dotted line), and glipizide-loaded beads (bold continuous line).
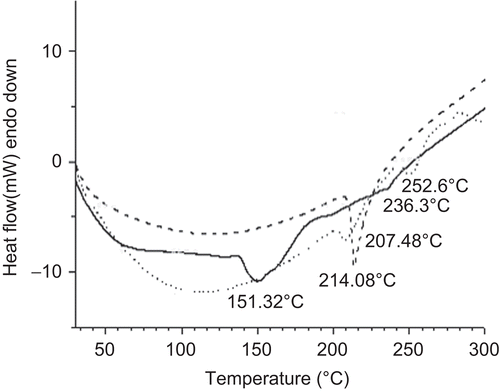
Figure 10. X-Ray diffraction pattern of (a) pure glipizide; (b) physical mixture; (c) glipizide-loaded CMLBG beads.
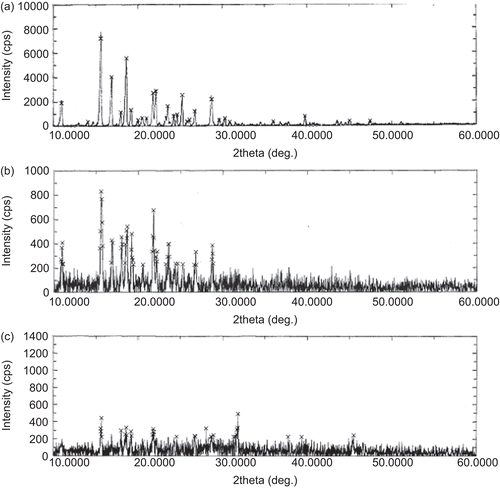
In vivo efficiency of the optimized formulation was tested in healthy normal male Wistar rats by measuring the hypoglycemic effect produced after oral administration. The basal blood glucose level (0 days) for the control group was 78.56 ± 5.25 mg/dl and the glucose level was shifted to 190.00 ± 5.53 mg/dl after 5 days following administration of alloxan to this group. Experimental diabetes of 207.00 ± 4.20 mg/dl was induced in Group II animals (basal blood glucose level was 89.53 ± 5.72 mg/ dl) after 5 days of alloxan administration. The reduction in blood glucose levels was observed for 12 h and it reached a maximum of 54.58% at the 7th hour after oral administration of the glipizide-loaded beads (). The glucose level of the animals belonging to the control group was 182.00 ± 4.43 after 12 h. CitationKahn and Shechter (1991) have suggested that a 25% reduction in blood glucose level can be considered a significant hypoglycemic effect. The hypoglycemic effect was significant in between 1–9 h. After that the blood glucose level was recovered to normal levels. The sustained hypoglycemic effect of the beads, observed over a longer period of time, was due to the slow release and absorption of glipizide over longer periods of time.
Conclusion
This study revealed that carboxymethyl derivative of LBG could form spherical gelled beads through ionotropic gelation with Al+3 ions in a completely aqueous environment. The arithmetic mean diameter of the beads increased from 1.24 × 103 µm to 1.27 × 103 µm, with the increase in AlCl3 concentration from 1–5% (w/v). As high as 97.68% drug entrapment efficiency could be achieved depending upon the concentration of AlCl3 in the gelation medium. The drug release was comparatively slower in acidic dissolution medium than in alkaline medium, and this could be attributed to different degrees of swelling in different dissolution media. The beads prolonged the drug release up to 10 h in alkaline dissolution medium, irrespective of the concentration of gelling agent. Increase in concentration of gelling ion in the gelation medium led to more dense beads and provided slower drug release profiles. Transfer of the beads (cross-linked with 5% w/v AlCl3) into alkaline dissolution medium following dissolution in acidic medium for 2 h also extended the drug release up to 10 h without any sign of disintegration. XRD and DSC analysis demonstrated that the drug was homogeneously distributed in the beads and most of the drug existed in amorphous state. FTIR analysis indicated that the drug was relatively stable in the beads. Moreover, in vivo anti-diabetic activity of these beads on animal models showed encouraging results. Hence, it can be concluded that CMLBG beads could be a useful carrier for controlled oral drug delivery.
Acknowledgements
The authors wish to thank all the management members of Gupta College of Technological Sciences, Asansol, West Bengal, India, and the authority of Jadavpur University, Department of Pharmaceutical Technology, Kolkata, India, for their kind cooperation and facilities provided to carry out the present research work.
Declaration of Interest
The authors report no conflicts of interest. The authors alone are responsible for the content and writing of the paper.
References
- Acarturk, F., Takka, S. (1999). Calcium alginate microparticles for oral administration: II effect of formulation factors on drug release and drug entrapment efficiency. J Microencapsul. 16:291–301.
- Anal, A.K., Bhopatkar, D., Tokura, S., Tamura, H., Stevens, W.F. (2003). Chitosan-alginate multilayer beads for gastric passage and controlled intestinal release of protein. Drug Dev Ind Pharm. 29:713–24.
- Anal, A.K., Stevens, W.F. (2005). Chitosan-alginate multilayer beads for controlled release of ampicillin. Int J Pharm. 290:45–54.
- Arica, B., Çaliş S, Atilla, P., Durlu, N.T., Çakar, N., Kaş, H.S., Hincal, A.A. (2005). In vitro and in vivo studies of ibuprofen-loaded biodegradable alginate beads. J Microencapsul. 22:153–65.
- Arıca, B., Çalış, S., Kaş, H.S., Sargon, M.F., Hıncal, A.A. (2002). 5-Fluorouracil encapsulated alginate beads for the treatment of breast cancer. Int J Pharm. 242:267–9.
- Aydin, Z., Akbuğa, J. (1996). Chitosan beads for the delivery of salmon calcitonin: preparation and release characteristics. Int J Pharm. 131:101–3.
- Aydinli, M., Tutas, M., Bozdemir, O.A. (2004). Mechanical and light transmittance properties of locust bean gum based edible films. Turk J Chem. 28:163–71.
- Bhardwaj, T.R., Kanwar, M., Lal, R., Gupta, A. (2000). Natural gums and modified natural gums as sustained release carriers. Drug Dev Ind Pharm. 26:1025–38.
- Bodmeier, R., Oh, K.-H., Pramar, Y. (1989). Preparation and evaluation of drug-containing chitosan beads. Drug Dev Ind Pharm. 15:1475–94.
- Capan, Y., Jiang, G., Giovangali, S., Na, K.H., Deluca, P.P. (2003). Preparation and characterization of poly (D,L-lactide-co-glycolide) microspheres for controlled release of human growth hormone. AAPS PharmSciTech. 4:1–10.
- Chen, L.Y., Du, Y., Zeng, X. (2003). Relationships between the molecular structure and moisture-absoption and moisture-retention abilities of carboxymethyl chitosan. II. Effect of degree of deacetylation and carboxymethylation. Carbohydr Res. 338:333–40.
- Chowdary, K.P.R., Rao, Y.S. (2003). Design and in vitro and in vivo evaluation of mucoadhesive microcapsules of glipizide for oral controlled release: a technical note. AAPS PharmSciTech. 4:1–6.
- Chowdary, K.P.R., Sundari, G.B. (2003). Design and evaluation of mucoadhesive controlled release oral tablets of glipizide. Ind J Pharm Sci. 65:591–4.
- Coppi, G., Iannuccelli, V., Leo, E., Bernabei, M.T., Cameroni, R. (2001). Chitosan-alginate microparticles as a protein carrier. Drug Dev Ind Pharm. 27:393–400.
- Das, M.K., Senapati, P.C. (2008). Furosemide-loaded alginate microspheres prepared by ionic cross-linking technique: Morphology and release characteristics. Ind J Pharm Sci. 70:77–84.
- Dea, I.C.M., Morrison, A. (1975). Chemistry and interactions of seed galactomannans. Adv Carbohydr Chem Biochem. 31:242–312.
- Du, J., Dai, J., Liu, J.-L., Dankovich, T. (2006). Novel pH-sensitive polyelectrolyte carboxymethyl Konjac glucomannan-chitosan beads as drug carriers. React Func Polym. 66:1055–61.
- Durig, T., Fassihi, R. (2000). Evaluation of floating and sticking extended release delivery systems: an unconventional dissolution test. J Contr Rel. 67:37–44.
- Foster, R.H., Plosker, G.L. (2000). Glipizide: a review of the pharmacoeconomic implications of the extended-release formulation in type 2 diabetes mellitus. Pharmacoeconomics. 18:289–306.
- Gaba, P., Singh, S., Gaba, M., Gupta, G.D. (2010). Galactomannan gum coated mucoadhesive microspheres of glipizide for treatment of type-2 diabetes: designing, in-vitro & in-vivo evaluation. Int J ChemTech Res. 2:312–24.
- Gupta, K.C., Ravi, K.M.N.V. (2000). Drug release behavior of beads and microgranules of chitosan. Biomaterials. 21:1115–9.
- Halder, A., Maiti, S., Sa, B. (2005a). Entrapment efficiency and release characteristics of polyethyleneimine-treated or-untreated calcium alginate beads loaded with propranolol–resin complex. Int J Pharm. 302:84–94.
- Halder, A., Mukherjee, S., Sa, B. (2005b). Development and evaluation of polyethyleneimine-treated calcium alginate beads for sustained release of diltiazem. J Microencapsul. 22:67–80.
- Jamzad, S., Fassihi, R. (2006). Development of controlled release low dose class II drug-glipizide. Int J Pharm. 312:24–32.
- Jayakumar, R., Reis, R.L., Mano, J.F. (2006). Synthesis of N-carboxymethyl chitosan beads for controlled drug delivery applications. Mater Sci Forum. 514–6:1015–9.
- Jayakumar, R., Reis, R.L., Mano, J.F. (2007). Synthesis and characterization of pH-sensitive thiol-containing chitosan beads for controlled drug delivery applications. Drug Deliv. 14:9–17.
- Kahn, C.R., Shechter, Y (1991). Oral hypoglycemic agents and the pharmacology of the endocrinepancreas. >In: Theodore, WR, Alan, SN, Taylor, P, Gilman, AG, ed. Goodman and Gilman’s The Pharmacological Basis of Therapeutics. New York: McGraw-Hill, 1461–95.
- Kikuchi, K., Kawabuchi, M., Watanabe, A., Sugihara, M., Sakuri, Y., Okano, T. (1999). Effect of Ca2+-alginate gel dissolution on release of dextran with different molecular weights. J Contr Rel. 58:21–8.
- Korsmeyer, R.W., Gurny, R., Doelker, E., Buri, P., Peppas, N.A. (1983). Mechanisms of solute release from porous hydrophilic polymers. Int J Pharm. 15:25–35.
- Kulkarni, R.V., Sa, B. (2009). Polyacrylamide-grafted-alginate-based pH-sensitive hydrogel beads for delivery of ketoprofen to the intestine: in vitro and in vivo evaluation. J Biomater Sci Polym Edn. 20:235–51.
- Maiti, S., Ray, S., Mandal, B., Sarkar, S., Sa, B. (2007). Carboxymethyl xanthan microparticles as a carrier for protein delivery. J Microencapsul. 24:743–56.
- Maiti, S., Singha, K., Ray, S., Dey, P., Sa, B. (2009). Adipic acid dihydrazide treated partially oxidized alginate beads for sustained oral delivery of flurbiprofen. Pharm Dev Technol. 14:461–70.
- Manjanna, K.M., Kumar, T.M.P., Shivakumar, B. (2009). Effect of manufacturing conditions on physico-chemical characteristics and drug release profiles of aceclofenac sodium microbeads. Drug Invent Today. 1:98–107.
- Mukherjee, B., Samanta, A., Dinda, S.C. (2006). Gum odina-a new tablet binder. Trends Appl Sci Res. 1:309–16.
- Nisperos-Carriedo, M.O. (1994). Edible coatings and films based on polysaccharides. In: Krochta, J.M., Baldwin, E.A., Nisperos-Carriedo, M.O., eds. Edible films and coatings to improve food quality. Lancaster: Technomic, 305–36.
- Parvathy, K.S., Susheelamma, N.S., Tharanathan, R.N., Gaonkar, A.K. (2005). A simple non-aqueous method for carboxymethylation of galactomannans. Carbohydr Polym. 62:137–41.
- Patel, J.K., Patel, R.P., Amin, A.F., Patel, M.M. (2005). Formulation and evaluation of mucoadhesive glipizide microspheres. AAPS PharmSciTech. 6:E49–55.
- Patil, S., Sharma, S., Nimbalkar, A., Pawar, A. (2006). Study of formulation variables on properties of drug-gellan beads by factorial design. Drug Dev Ind Pharm. 32:315–26.
- Quong, D., Yeo, J.-N., Neufeld, R.J. (1999). Stability of chitosan and poly-L-lysine membranes coating DNA-alginate beads when exposed to hydrolytic enzymes. J Microencapsul. 16:73–82.
- Ray, S., Maiti, S., Sa, B. (2008). Preliminary investigation on the development of diltiazem resin complex loaded carboxymethyl xanthan beads. AAPS PharmSciTech. 9:295–301.
- Reddy, T., Tammishetti, S. (2002). Gastric resistant microbeads of metal ion cross-linked carboxymethyl guar gum for oral drug delivery. J Microencapsul. 19:311–8.
- Ritger, P.I., Peppas, N.A. (1987). A simple equation for description of solute release II. Fickian and anomalous release from swellable devices. J Contr Rel. 5:37–42.
- Roy, A., Bajpai, J., Bajpai, A.K. (2009). Development of calcium alginate-gelatin based microspheres for controlled release of endosulfan as a model pesticide. Ind J Chem Tech. 16:388–95.
- Sanap, G.S. (2009). Formulation and evaluation of mucoadhesive beads of glipizide using 23 factorial design. J Pharm Res. 2:934–8.
- Semalty, M., Semalty, A., Kumar, G., Juyal, V. (2008). Development of mucoadhesive buccal films of glipizide. Int J Pharm Sci Nanotech. 1:184–90.
- Setty, C.M. (2005). Development and evaluation of novel microparticulate drug delivery system using natural polymer. PhD thesis. Kolkata: Jadavpur University, India.
- Sierakowaski, M.R., Milas, M., Desbrieres, J., Rinacedo, M. (2000). Specific modifications of galactomannans. Carbohydr Polym. 42:51–7.
- Singh, B.N., Kim, K.H. (2005). Effects of divalent cations on drug encapsulation efficiency of deacylated gellan gum. J Microencapsul. 22:761–71.
- Sriamornsak, P., Puttipipatkhachorn, S., Prakongpan, S. (1997). Calcium pectinate gel coated pellets as an alternative carrier to calcium pectinate beads. Int J Pharm. 156:189–94.
- Srinatha, A., Pandit, J.K., Singh, S. (2008). Ionic cross-linked chitosan beads for extended release of ciprofloxacin: in vitro characterization. Ind J Pharm Sci. 70:16–21.
- Thimma, R.T., Tammishetti, S. (2001). Barium chloride crosslinked carboxymethyl guar gum beads for gastrointestinal drug delivery. J Appl Polym Sci. 82:3084–90.
- Thygesen, L.G., Løkke, M.M., Micklander, E., Engelsen, S.B. 2003. Vibrational microspectroscopy of food. Raman vs FT-IR. Trends Food Sci Technol. 14:50–7.
- Tiwari, G., Tiwari, R., Srivastava, B., Rai, A.K. (2008). Development and optimization of multi-unit solid dispersion systems of poorly water soluble drug. Res J Pharm Tech. 1:444–9.
- Tomida, H., Mizuo, C., Nakamura, C., Kiryu, S. (1993). Imipramine release from Ca-alginate gel beads. Chem Pharm Bull. 41:1475–7.
- Ueng, S.W.N., Lee, S.-S., Lin, S.-S., Chan, E.-C., Hsu, B.R.-S., Chen, K.-T. (2000). Biodegradable alginate antibiotic beads. Clin Orthopaed Relat Res. 380:250–9.
- Ueng, S.W.N., Yuan, L.-J., Lee, N., Lin, S.-S., Chan, E.-C., Weng, J.-H. (2006). In vivo study of biodegradable alginate antibiotic beads in rabbits. J Orthopaed Res. 22:592–9.
- Venkataraju, M.P., Gowdaa, D.V., Rajeshb, K.S., Shivakumar, H.G. (2007). Xanthan and locust bean gum (from Ceratonia siliqua) matrix tablets for oral controlled delivery of propranolol hydrochloride. Asian J Pharm Sci. 2:239–48.
- Verma, R.K., Garg, S. (2004). Development and evaluation of osmotically controlled oral drug delivery system of glipizide. Eur J Pharm Biopharm. 57:513–25.
- Vogt, S., Heinze, T., Rottig, K., Klemm, D. (1995). Preparation of carboxymethylcellulose sulfate of high degree of substitution. Carbohydr Res. 266:315–20.
- Vyas, S.P., Khar, R.P. (2002). Controlled drug delivery: concepts and advances. New Delhi, India: Vallabh Prakashn.
- Win, P.P., Shin-ya, Y., Hong, K.-J., Kajiuchi, T. (2003). Formulation and characterization of pH sensitive drug carrier based on phosphorylated chitosan (PCS). Carbohydr Polym. 53:305–10.
- Yalpani, M. (1999). Chemistry of polysaccharide modification and degradation. In: Finch P, ed. Carbohydrates: structures, syntheses and dynamics. Germany: Kluwer Academic Publishers, 294–318.
- Yegin, B.A., Moulari, B., Durlu-Kandilci, N.T., Korkusuz, P., Pellequer, Y., Lamprecht, A. (2007). Sulindac loaded alginate beads for a mucoprotective and controlled drug release. J Microencapsul. 24:371–82.
- Zhbankov, R.G., Andrianov, V.M., Marchewka, M.K. (1997). Fourier transform IR and Raman spectroscopy and structure of carbohydrates. J Molec Str. 436–7:637–54.