Abstract
Two model drug eluting stents of poly(lactic acid) (PLA)/everolimus and poly(ethylene vinyl alcohol) copolymer (EVAL)/everolimus have been investigated using complementary surface analysis techniques including AFM, XPS, and ATR-IR to assess their structure and its relation to drug release. Different surface morphologies were observed for these stents, with phase separation evident on the PLA coating and a homogeneous system for the EVAL-based coating. This indicates a potentially different drug distribution for the different stents, although both showed a surface enrichment of the drug compared to the bulk. Dissolution studies for PLA/everolimus stents showed an immediate loss of drug from the surface as well as a longer term polymer matrix erosion. The EVAL/everolimus stent also displayed a loss of drug from its surface, but an intact surface after 28 days in dissolution media. These data are discussed in relation to the different release mechanisms occurring in the stents.
Keywords::
Introduction
The advent of stents has greatly improved the outcome of coronary disease treatment. Stents are proven to reduce restenosis, the re-narrowing of the coronary blood vessel after balloon angioplasty rate by around one half (CitationPanescu, 2004). Restenosis is a three-stage response of the vessel wall to wall injury: acute elastic recoil, negative remodeling, and neointimal proliferation (CitationVan der Hoeven & Pires, 2004). The original bare metal stent could significantly reduce the effects of vessel recoil and remodeling (CitationMudra et al., 2001). However, it could also possibly induce excessive neointimal proliferation and inflammation due to the vessel injury, which are the main reasons of in-stent restenosis (CitationHanke et al., 1990; CitationPeng et al., 1996; CitationLau et al., 2004; CitationToutouzas et al., 2004). Drug eluting stents (DES) are designed to address this issue. Such stents are typically coated with a biocompatible polymer and loaded with an appropriate drug. Both biodegradable and biostable polymers can be applied as carriers for drug delivery depending upon the formulation and process designs (CitationAnderson, 2001). The extensively used biodegradable polymers include poly(lactic acid) (PLA), poly(glycolic acid), and their copolymers. Recently, block copolymers have received attention in the area of drug delivery technology, due to their capability to provide a biomaterial having a broad range of amphiphilic characteristics, as well as targeting the drugs to specific site (CitationYokoyama, 1992; CitationKumar et al., 2001; CitationKavanagh et al., 2004; CitationRanade et al., 2005). The release of drug from a polymer can occur through single or multiple mechanisms, inclusive of dissolution, diffusion, and degradation of the polymer (CitationLanger, 1990). To prolong the drug release from diffusion-based systems, drug-free polymer can be applied to the surface of the drug-loaded polymer to act as a barrier layer (CitationWaksman, 2002). The drugs loaded in the polymer coating should show the ability of either anti-proliferation or anti-inflammation, or both. Currently, sirolimus (CitationChen et al., 2005), everolimus (CitationMarcel et al., 2007), and paclitaxel (CitationVogt et al., 2004) are the main drugs used in stents.
Previously we have shown that local force measurements using atomic force microscopy (AFM) reveal that the drug everolimus does not significantly alter the mechanical properties of a PLA-based coating, and that immersion in dissolution media causes a reduction in the glass transition of the coating (CitationWu et al., 2009). Here we report a study of drug release from two sets of everolimus eluting stents, one coated with PLA and the other with poly(ethylene vinyl alcohol) copolymer (EVAL). PLA has been used widely as a carrier for drug delivery. It is considered biocompatible and biodegradable, and needs no re-treatment of the carrier after drug depletion (CitationChung et al., 2001). EVAL also has good biocompatibility. Compared to PLA it is bio-stable and due to the vinyl alcohol repeat units it is more hydrophilic (CitationKenawy et al., 2003). Here we investigate how these different properties effect everolimus distribution and how this distribution influences drug release. Everolimus is an anti-proliferation drug with a higher polarity which helps overcome formulation problems regarding high tissue distribution of sirolimus and gives a slightly higher lipophilic property leading to a more rapid absorption into the arterial wall (CitationWaksman, 2002; CitationRanade et al., 2004). In this study, AFM has been used to characterize the surface morphology and monitor the in-situ morphology changes due to the drug release. Attenuated total internal reflection infra-red spectroscopy (ATR-IR) and X-ray photoelectron spectroscopy (XPS) have been used to characterize the surface chemistry, identifying the drug concentration at the surface and near-surface. With the combination of these techniques, we have identified the drug distribution in the polymer coating before and during dissolution. This data, complemented by the bulk release profiles of the stents, is used to reveal the drug release mechanisms of each system.
Materials and methods
Materials
Both the PLA/everolimus (50%/50% weight ratio) and EVAL/everolimus (78%/22% weight ratio) coated drug eluting stents were provided by Abbott Vascular (CA). The coatings were deposited using an in-house custom spraying technique by Abbott. The length of stent is 14 mm and the thickness of coating is ∼ 9 µm. The overall drug loading is 200 µg. PLA density is 1.25 g/cm3, everolimus density is 1 g/cm3. The molecular weights of PLA and EVAL are ∼ 50 kD and ∼ 25 kD, respectively. All information was provided by Abbott. Triton X100 was purchased from Sigma (Poole, UK). Tempfix adhesive was purchased from Agar (Stansted, UK). The coating surface morphology was studied with AFM and the coating surface chemistry was analyzed with XPS and ATR-IR.
Atomic force microscopy (AFM)
The stent surface morphology in air and in liquid was observed by D3000 AFM (Veeco, Cambridge, UK). The stents were cut, open, flattened, and mounted onto silicon wafers with Tempfix adhesive. The surface coverage as well as the average diameter of domains was calculated using the AFM images acquired in air using the Nanoscope software (Veeco). Knowing the drug density, the amount of the drug loading at the surface can be calculated. For the dissolution study on the PLA/everolimus stent, the stent sample was kept in release media (1% Triton X100) for 30 min. Subsequently the stent was removed from the liquid, dried under ambient conditions, and analyzed by tapping mode AFM (TM-AFM) in air. Then the stent was put into liquid for further 30 min periods and analyzed by TM-AFM in air again. The same position was analyzed at 0, 30, and 60 min time points. The in-situ study was also performed by keeping the stents continuously in the release media up to 60 min. The volumes of the pits formed were measured around every 10 min in order to reveal the erosion rate of the domain after its initial burst release. For the long-term in situ study the stent samples were kept in release media for up to 28 days. The release media was refreshed regularly. All imaging was undertaken at the ambient conditions of 25°C in 1% Trition X100 release media which was refreshed regularly. The use of Triton as dissolution media aims to facilitate the drug diffusion through the polymer matrix, thereby accelerating the overall drug release rate for this in-vitro study to understand the drug release mechanism in a relatively short time period (CitationKamberi et al., 2009).
Attenuated total internal reflection infra-red spectroscopy (ATR-IR)
An Avatar 360 Inspect IR spectrometer equipped with silicon crystal was employed (Thermo Nicolet, Waltham, MA). The stents samples were pressed into intimate optical contact before and after dissolution with the top surface of the ATR silicon crystal for analysis in the infrared range of 4000–500 cm−1 with 256 times scans at the resolution of 1 cm−1 for each position. ATR-IR was applied to identify the chemical information of the near surface region to ∼ 1 μm in depth to investigate drug distribution. Characterization was performed on the drug powder and polymer only coated stents first to obtain the reference IR spectra before the analysis of the drug-loaded stents. Representative peaks for everolimus were at 1645 cm−1 and 990 cm−1, for PLA at 1268 cm−1, and for EVAL at 835 cm−1.
X-ray photoelectron spectroscopy (XPS)
The stent samples were scanned by X-ray photoelectron spectroscopy (Kratos AXIS ULTRA, Manchester, UK) with a monochromated Al Kα X-ray source (1486.6 eV) operated at 15 mA emission current and 10 kV anode potential before and after dissolution. The take off angle for the photoelectron analyzer was 90°, with an acceptance angle of 30°. A magnetic immersion lens system allowed the area of analysis to be defined by apertures, a ‘slot’ aperture of 300 × 700 μm for wide/survey scans and high resolution scans.
Results and discussions
The PLA/everolimus stent typically showed a two phase separated surface morphology with circular domains surrounded by a continuous matrix (). The bright domains in the topography are higher than the surrounding matrix by several tens of nanometres and correspond to the dark regions in the phase image. The observed AFM phase difference is due to the variation in material properties which are detected through variations in the degree and nature of the AFM probe interactions over these regions. The darker phase indicates more energy lost by the vibrating AFM cantilever, which can be due to, for example, increased probe–sample contact area, a softer surface, or a more adhesive interaction compared to the brighter phase (CitationChen et al., 1998; Citation2002). These data hence clearly imply that the domains consist of a different material or combination of materials compared to the matrix. Previously we have shown these regions to be drug enriched regions which also contain the polymer (CitationWu, 2008; CitationWu et al., 2009). The average surface coverage of the domains was ∼ 70%. After 30 min dissolution pits were found at the previous locations of the domains as highlighted with boxes (). This suggested a rapid loss of domain material from the top surface within 30 min. Similar results have been reported previously on another polymeric system (CitationRanade et al., 2004). The ongoing existence of phase contrast at 30 min implied that, although pits have formed in the previous domain positions, the original domain material has not been completely lost, as indicated by continued contrast. After a further 30 min period of dissolution, no significant change could be seen in the topography image. However, the loss of relative phase contrast was found showing the exhaustion of the domain material (). The matrix material beneath the domains has now been completely exposed, with the AFM phase imaging indicating a homogenous surface. Analysis of the diameter and the volume of such pits as they developed showed a linear increase with time, with a rough cubic relationship between volume and diameter. This suggests that the loss of material from the stent surface within this 60 min period of dissolution was related to the loss of the domain material and not from the surrounding matrix, as a general loss of material from across the stent surface would distort this relationship. In addition, from these observations the domains’ erosion rate could be determined as being on the order of 0.13 × 106 nm3/min ().
Figure 1. Images from a AFM dissolution study of the PLA/everolimus stent (a) before and after (b) 30, (c) 60 min in liquid (1% Triton X100) and (d) pit volume change vs time.
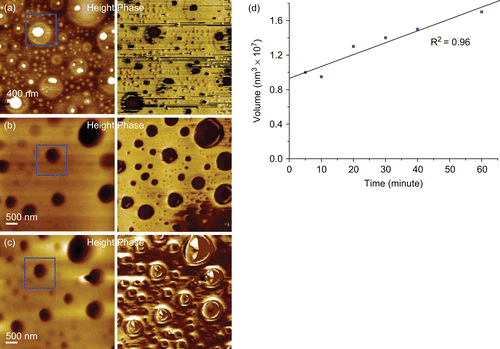
To explore the nature of the loss of the domain material, XPS was carried out on PLA/everolimus stent before and after 30 and 60 min dissolution (). The observed Fluorine peak was likely due to contamination from the stent packaging. Before dissolution, a nitrogen peak was found on the chemical composition spectra (, 0 min), which is a diagnostic peak for the drug (everolimus, C53O14NH86) since it is the only material containing nitrogen in this delivery system. By comparing the N level in the pure drug which is 1.47% with the N level in the drug/polymer system which is 1.25 ± 0.09%, the percentage of drug on the surface could be calculated which is ∼ 85% (1.25% divided by 1.47%) from five different positions. This is clearly higher than the designed bulk ratio of the drug of 50%, strongly suggesting a drug-rich surface of the PLA/everolimus stent. A similar drug-rich surface was also found on a sirolimus-loaded PLGA coated stent analyzed by ToF-SIMS (CitationMahoney & Fahey, 2008). Secondly, taking into account the stent surface area, the 70% surface coverage of the domains, and the drug density, the weight of drug in the domains could be calculated as ∼ 8.5 µg, or 4.3% of the total drug loading. After 30 min dissolution, nitrogen could not be detected from the surface with XPS, which means the drug was released within this period of time and the drug weight percentage on the surface was at least lower than 0.14% (the XPS detection limit). According to the general drug release profile in this media indicated in , ∼ 16% of total loaded drug (32 µg) is expected to be released after 30 min dissolution. Hence, in addition to the ∼ 8.5 µg drug from the surface domains, a further contribution to the initial release must come from the regions below the 10 nm depth of the XPS analysis, indicating significant diffusion of the drug from the bulk of the coating. In the carbon component spectra (, 30 min) only three peaks at 285, 286.9 and 289 eV, diagnostic for PLA (C-C, C-O, C=O) could be observed which further confirmed the loss of the drug from the stent surface after 30 min dissolution. The XPS data after 60 min was similar (data not shown). In making a comparison between the dissolution data in and the ex-situ XPS and ATR-IR and the in situ AFM data it should be noted that dissolution took place under flow for the bulk studies and under static conditions for the surface studies. Hence, the kinetics of the processes being studied may vary, although the fundamental nature of these processes is expected to remain the same.
Figure 2. Example XPS spectra of a PLA/everolimus stent before and after 30 min dissolution in 1% Triton X100. (a) The chemical composition spectra at 0 and 30 min. (b) The corresponded carbon component spectra.
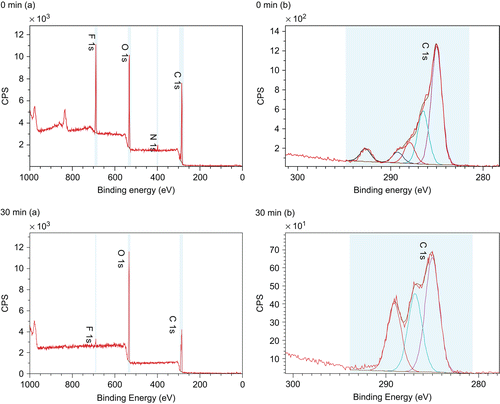
Figure 3. Drug release profile of PLA/everolimus stents and EVAL/everolimus stents in 1% Triton X100 up to 72 h.
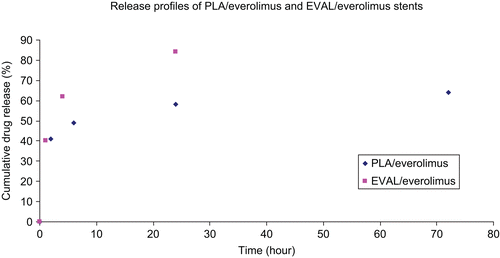
The null XPS results for the dissoluted samples at 30 and 60 min confirm the depletion of the drug in the top 10 nm of the coating. However, at 60 min the bulk dissolution data () indicates that ∼ 80% of the drug still remains in the PLA coating. To investigate the profile of transport of the drug from the bulk to the surface (and hence release), ATR-IR was applied to acquire further chemical information at a greater depth of analysis (∼ 1 μm in depth). shows a series of spectra recorded for the PLA-based stent at time points up to 216 h. A clear weakening of the drug representative peak at 1645 cm−1 with time is evident. The polymer-related peaks remain relatively constant. The ratio of the intensity of the diagnostic peak for the drug to the polymer in each single spectrum was used to indicate the relative loss of drug. shows the relative drug release profile in this near surface region, with the drug being depleted below the detection limit at the last time point. An initial burst release is evident (as seen in the bulk release profile, ) over the same time period covered by the AFM data in . This is followed by a phase where the drug concentration in this region remains roughly constant up to 5 days, which is consistent with the bulk release profile shown in . On keeping the stent in release media beyond 5 days, the drug level gradually reduces, indicating that the reservoir of the drug in the bulk of the coating is no longer able to maintain a constant concentration of the drug in the region of analysis. To aid evaluation of this longer time period of behavior as observed by ATR-IR, a PLA/everolimus stent was observed in dissolution media by AFM for up to 28 days (). In these data an area of matrix only is shown to examine changes in these regions. A clear granular nature of the matrix can be seen with average sizes of ∼ 40 nm. This size did not change throughout. After exposures exceeding 8 days, evidence of significant erosion could be observed (see example in ). At this stage the polymer coating was easily disturbed by the small forces applied by the scanning AFM tip (), suggesting a softening of the polymer surface. This damage is likely to be due to changes in the polymer’s structure after long-term exposure to liquid. Hydration (plasticization) of the polymer film or partial dissolution could possibly lead to this softer structure. A similar surface-like erosion was also found on a PVA-g-PLGA paclitaxel releasing film (CitationWestedt et al., 2006).
Figure 4. (a) ATR-IR spectra of dissoluted PLA/everolimus coated stents (50wt%/50%wt drug polymer ratio) at different time points (from top to bottom) of 0, 0.5, 1, 2, 6, 24, 72, 120, 168, and 216 h. The peak at 1645 cm−1 was contributed from everolimus only. (b) Near surface drug release profiles of PLA/everolimus and EVAL/everolimus-coated stents (50wt%/50wt% drug polymer ratio) derived from ATR-IR spectra at the release in 1% Triton XL-80N up to 216 h.
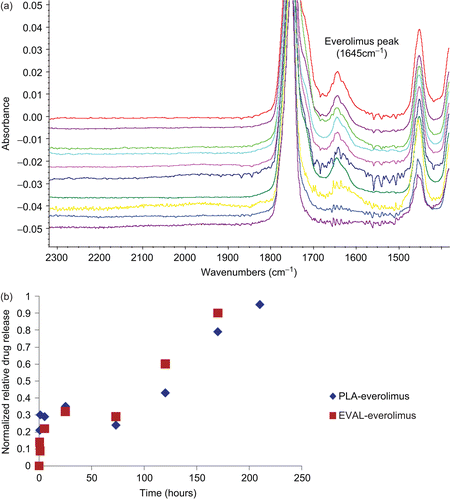
Figure 5. Example data from a long-term AFM in-situ study of PLA/everolimus stent up to 28 days in 1% Triton X100. (a) AFM image at 4 h, z-range 10 nm. (b, d) the same area at 8 and 28 days, z-range 10 nm. (c) An AFM imaging the soft state of the surface at 28 days showing an area within the image previously imaged and displaying significant disruption due to the scanning AFM probe working in tapping mode, z-range 1400 nm.
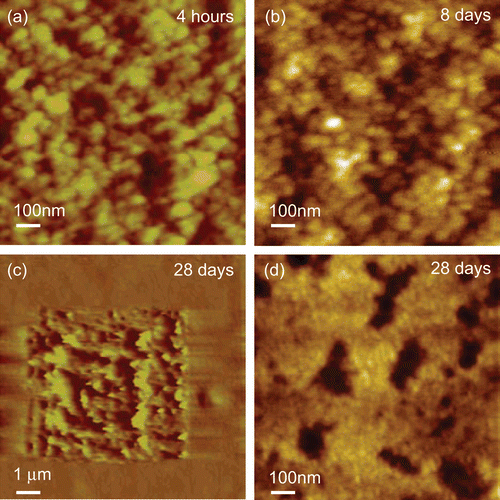
Taken together this study suggests three different stages of drug release from the biodegradable PLA/everolimus stent: a rapid release of the drug from the top surface due to the loss of domain material, which is known to be drug-rich (CitationWu et al., 2009), followed by a much slower erosion of the bulk material over weeks and months. Such erosion would be expected to lead to zero-order release kinetics (CitationWang et al., 2006). However, whilst drug release is occurring due to this erosion process, in a similar manner to that described by CitationAlexis et al. (2004; Citation2005), since we can place a maximum level of dug concentration at the surface of 0.14%, this is perhaps not likely to be the sole source of drug release in this phase. Therefore, the diffusion of the drug from the bulk of the sample is also occurring from buried drug-rich domains, possibly through channels or pits generated after the initial burst release as well as by diffusion through the polymer matrix (CitationEhtezazi & Washington, 2000). Such release mechanisms are associated with a first order process, leading to a degree of time dependence in the release, as evident in the bulk dissolution profile. The noted softening of the polymer matrix in the Triton buffer over a period of days may also contribute to this release process through increasing diffusion rates of the drug within the polymer. Finally, after ∼ 5 days the drug remaining within the coating can no longer maintain the internal diffusion concentration gradient and the amount of drug in the near-surface region reduces to a negligible amount beyond 20 days. The noted softening of the PLA films also suggests that in vivo the film itself may eventually be removed from the stent in a period of months.
shows the morphology of the EVAL/everolimus stent as observed by AFM. No phase separated domains were found in this stent, suggesting an intimate mixture of the drug and polymer. Compared to the drug release profile of PLA/everolimus stent, the drug release rate of EVAL/everolimus stent is faster in the same period of time (). This is possibly because the EVAL matrix has a lower Tg than PLA, facilitating more rapid diffusion of the drug from the polymer (CitationGeulcher & Hollinger, 2005). On keeping the stent in a 1% Triton X100 environment for up to 28 days, the surface showed a consistent granular structure. No voids or significant changes could be seen from the AFM topography images over this time. Clearly the EVAL/everolimus stent has a different drug release mechanism compared to the PLA/everolimus stent. XPS spectra for this stent are shown in . Before dissolution the drug-related nitrogen peak was evident in the chemical composition spectra (, 0 min). The drug weight percentage on the surface could also be calculated, which is 41%, again much higher than the bulk percentage (here 22%). As in the case of the PLA stent after 30 min dissolution, the nitrogen peak had disappeared (, 30 min). The existence of the C-C and the C-O peaks but the lack of a C=O peak in the carbon component spectra (, 30 min) further confirmed the loss of the drug from the surface. This indicates that the drug was lost from the surface, although there were no detectable morphology changes during the long-term in situ AFM study. As for the PLA coating, ATR-IR analysis was carried out to investigate drug concentration in the near surface region over a longer time scale (). This shows a broadly similar profile to the PLA stent in this region, with a fast initial release, a plateau region of drug concentration and a final phase of depletion in this region. This similarity indicates that the drug release profile from ATR-IR is dominated by the diffusion related profile within the coating. Clearly, the lack of any evident erosion suggests that the drug release mechanism for the EVAL/everolimus stent was solely based on diffusion control (CitationAcharya & Park, 2006) and is more rapid in the first instance then the PLA coating, possibly due its lower glass transition temperature (CitationAhn & Jeong, 1998; CitationWu, 2008).
Conclusions
Two different formulations of drug-eluting stents, PLA/everolimus and EVAL/everolimus, have been characterized. A two phase separated structure with circular domains surrounded by matrix were observed on PLA/everolimus stent. XPS data indicated an initial drug enriched surface compared to the bulk (85% vs 50%). AFM dissolution studies on the PLA/everolimus stent showed a rapid loss of the domain material from the surface within 1 h followed by slow erosion and softening of the film over a period of days. A parallel XPS study indicated a similarly rapid loss of drug from the immediate surface after the loss of this drug-rich domain material. XPS could not detect any drug at the surface beyond 60 min (whilst it is known that drug is being released). This indicates that the drug surface concentration at any time point after 1-h is less than 0.14% and that therefore release of drug through a surface erosion-based mechanism from the polymer matrix areas is unlikely to be the only source of release. An ATR-IR based dissolution study over several days showed a rapid reduction in drug concentration from the top ∼ 1 μm region of the stent during the burst release phase and then a constant concentration over a period of ∼ 5 days before the drug began to be exhausted. Taken together this study suggests three different stages of drug release from the biodegradable PLA/everolimus stent: a rapid release of the drug from the top surface due to the loss of domain material followed by a period of both erosion and diffusion from the matrix controlled release of drug from the stent. Finally, after ∼ 5 days the drug remaining within the coating can no longer maintain the internal diffusion concentration gradient and the amount of drug in the near-surface region reduces to a negligible amount beyond 20 days and drug release ceases.
No domains were found on the EVAL/everolimus stent surface, indicating a homogeneous mixture of drug and polymer. No significant changes of the surface could be observed during long-term in situ AFM studies. However, the XPS confirmed that the drug does exist on the surface prior to dissolution studies, but failed to detect drug at the surface at time point at and beyond 30 min. Again the XPS data indicated a drug enriched surface compared to the bulk (41% vs 22%). ATR-IR analysis showed a similar trend in drug concentration in the top one micron region as the PLA stent, despite the lack of surface erosion-related release, indicating that this profile is dominated by the diffusion related profile within the coating. Based upon this data we propose a diffusion controlled drug release mechanism for the EVAL/everolimus stent, where the lower glass transition of EVAL compared to PLA is responsible for its more rapid initial burst release.
Acknowledgements
We would like to thank Abbott Vascular and the University of Nottingham for providing a PhD studentship for Ming Wu.
Declaration of interest
The authors report no conflicts of interest. The authors alone are responsible for the content and writing of the paper.
References
- Acharya, G., Park, K. (2006). Mechanisms of controlled drug release from drug-eluting stents. Adv Drug Deliv Rev. 58:387–401.
- Ahn, S.-B., Jeong, H.M. (1998). Phase behavior and hydrogen bonding in poly(ethylene-co-vinyl alcoho)/poly(N-vinyl-2-pyrrolidone) blends. Kor Polym J. 6:389–95.
- Alexis, F., Rath, S.K., Venkatraman, S.S. (2005). Controlled release from bioerodible polymers: effect of drug type and polymer composition. J Contr Rel. 102:333–44.
- Alexis, F., Venkatraman, S.S., Rath, S.K., Boey, F. (2004). In vitro study of release mechanisms of paclitaxel and rapamycin from drug-incorporated biodegradable stent matrices. J Contr Rel. 98:67–74.
- Anderson, J.M. (2001). Biodegradation of polymers. Encyclopedia of Materials: Science and Technology, London: Pergamon, 560–3.
- Chen, M.C., Liang, H.F., Chui, Y.L., Chang, Y., Wei, H.J., Sung, H.W. (2005). A novel drug-eluting stent spray-coated with multi-layers of collagen and sirolimus. J Contr Rel. 108:178–89.
- Chen, X., Davies, M.C., Roberts, C.J., Tendler, S.J.B., Williams, P.M., Davies, J., Dawkes, A.C., Edwards, J.C. (1998). Interpretation of tapping mode atomic force microscopy data using amplitude-phase-distance measurements. Ultramicroscopy 75:171–81.
- Chen, X., Roberts, C.J., Zhang, J., Davies, M.C., Tendler, S.J.B. (2002). Phase contrast and attraction-repulsion transition in tapping mode atomic force microscopy. Surface Sci. 519:L593–8.
- Chung, T.W., Huang, Y.Y., Liu, Y.Z. (2001). Effects of the rate of solvent evaporation on the characteristics of drug loaded PLLA and PDLLA microspheres. Int J Pharm. 212:161–9.
- Ehtezazi, T., Washington, E. (2000). Controlled release of macromolecules from PLA microspheres: using porous structure topology. J Contr Rel. 68:361–72.
- Geulcher, S.A., Hollinger, J.O. (2005). An introduction to biomaterials. Taylor & Francis, CRC Press; Boca Raton, FL, USA.
- Hanke, H., Strohschneider, T., Oberhoff, M., Betz, E., Karsch, K.R. (1990). Time course of smooth muscle cell proliferation in the intima and media of arteries following experimental angioplasty. Circ Res. 67:651–9.
- Kamberi, M., Nayak, S., Myo-Min, K., Carter, T.P., Hancock, L., Feder, D. (2009). A novel accelerated in vitro release method for biodegradable coating of drug eluting stents: insight to the drug release mechanisms. Eur J Pharm Sci. 37:217–22.
- Kavanagh, C.A., Rochev, Y.A., Gallagher, W.M., Dawson, K.A., Keenan, A.K. (2004). Local drug delivery in restenosis injury: thermoresponsive co-polymers as potential drug delivery systems. Pharmacol Ther. 102:1–15.
- Kenawy, E.R., Layman, J.M., Watkins, J.R., Bowlin, G.L., Matthews, J.A., Simpson, D.G., Wnek, G.E. (2003). Electrospinning of poly(ethylene-co-vinyl alcohol) fibers. Biomaterials. 24:907–13.
- Kumar, N., Ravikumar, M.N.V., Domb, A.J. (2001). Biodegradable block copolymers. Adv Drug Deliv Rev. 53:23–44.
- Langer, R. (1990). New methods of drug delivery. Science. 249:1527–1533.
- Lau, K.W., Mak, K.H., Hung, J.S., Sigwart, U. (2004). Clinical impact of stent construction and design in percutaneous coronary intervention. Am Heart J. 147:764–73.
- Mahoney, C.M., Fahey, A.J. (2008). Three-dimensional compositional analysis of drug eluting stent coatings using cluster secondary ion mass spectrometry. Anal Chem. 80:624–32.
- Marcel, A., Beijk, M., Piek. J.J. (2007). XIENCE V everolimus-eluting coronary stent system: a novel second generation drug-eluting stent. Exp Rev Med Devices. 4:11–21.
- Mudra, H., Di Mario, C., de Jaegere, P., Figulla, H.R., Macaya, C., Zahn, R., Wennerblom, B., Rutsch, W., Voudris, V., Regar, E., Henneke, K.H., Schachinger, V., Zeiher, A. (2001). Randomized comparison of coronary stent implantation under ultrasound or angiographic guidance to reduce stent restenosis. Circulation. 104:1343–9.
- Panescu, D. (2004). Drug eluting stents. Eng Med Biol Mag. 23:21–3.
- Peng, T., Gibula, P., Yao, K., Goosen, M.F.A. (1996). Role of polymers in improving the results of stenting in coronary arteries. Biomaterials. 17:685–94.
- Ranade, S.V., Miller, K.M., Richard, R.E., Chan, A.K., Allen, M.J., Helmus, M.N. (2004). Physical characterization of controlled release of paclitaxel from the TAXUSTM express2TM drug-eluting stent. J Biomed Mater Res A. 71A:625–34.
- Ranade, S.V., Richard, R.E., Helmus, M.N. (2005). Styrenic block copolymers for biomaterial and drug delivery applications. Acta Biomat. 1:137–44.
- Toutouzas, K., Colombo, A., Stefanadis, C. (2004). Clinical impact of stent construction and design in percutaneous coronary intervention. Eur Heart J. 25:1679–87.
- Van der Hoeven, B.L., Pires, N.M.M. (2004). Drug-eluting stents: results, promises and problems. Int J Cardiol. 99:9–17.
- Vogt, F., Stein, A., Rettemeier, G., Krott, N., Hoffmann, R., Dahl, J.V., Bosserhoff, A.K., Michaeli, W., Hanrath, P., Weber, C., Blindt, R. (2004). Long-term assessment of a novel biodegradable paclitaxel-eluting coronary polylactide stent. Eur Heart J. 25:1330–40.
- Waksman, R. (2002). Drug eluting stents from bench to bed. Cardiovasc Radiat Med. 3:226–41.
- Wang, X., Venkatraman, S.S., Boey, F.Y.C., Loo, J.S.C., Tan, L.P. (2006). Controlled release of sirolimus from a multilayered PLGA stent matrix. Biomaterials. 27:5588–95.
- Westedt, U., Wittmar, M., Hellwig, M., Hanefeld, P., Greiner, A., Schaper, A.K., Kissel, T. (2006). Paclitaxel releasing films consisting of poly(vinyl alcohol)-graft-poly(lactide-co-glycolide) and their potential as biodegradable stent coatings. J Contr Rel.111:235–46.
- Wu, M. (2008). The micro and nanoscale characterization of drug eluting stents. Thesis, University of Nottingham.
- Wu, M., Lothar, K., Fuh-Wei, T., Davies, M.C., Roberts, C.J. (2009). Nanoscale mechanical measurement determination of the glass transition temperature of poly(lactic acid)/everolimus coated stents in air and dissolution media. Eur J Pharm Sci. 36:493–501.
- Yokoyama, M. (1992). Block copolymers as drug carriers. Crit Rev Ther Drug Carrier Syst. 9:213–48.