Abstract
The asialoglycoprotein receptor, which is abundantly and near exclusively expressed on hepatocytes, has received much attention in the design of non-viral hepatotropic DNA delivery systems. Thus, asialoglycoproteins and hexopyranosyl ligands have been coupled to DNA-binding cationic polymers and liposomes in the assembly of complexes intended for uptake by liver parenchymal cells. The aim of the study was to construct a hepatocyte-targeted multimodular liposome-based transfecting complex, in which the biotin-streptavidin interaction provides the cohesive force between the ligand asialorosomucoid and the liposome bilayer, and to evaluate its transfection capabilities in the hepatocyte-derived human transformed cell line HepG2. Dibiotinylated asialoorosomucoid was attached to cationic liposomes constructed from 3β[N-(N’,N’-dimethylaminopropane)-carbamoyl] cholesterol (Chol-T):dioleoylphosphatidylethanolamine:biotinylcholesterylformylhydrazide (MSB1) (48:50:2 mole ratio) through streptavidin interposition. Liposome-pGL3 DNA interactions were studied by gel band shift and ethidium displacement assays. The cytotoxicity of assemblies was evaluated in the HepG2 cell line and transfection capabilities determined by measuring the activity of the transgene luciferase. Binding assays showed that all DNA was liposome associated at a DNA (negative):liposome (positive) charge ratio of 1:1. Accommodation of a streptavidin dibiotinylated asialoorosomucoid assembly was achieved at a DNA:liposome:streptavidin dibiotinylated asialoorosomucoid ratio of 1:4:9 (weight basis). Complexes showed optimal transfection activity at this ratio, which was reduced 10-fold by the presence of the competing ligand asialofetuin. The streptavidin-biotin interaction has been applied for the first time to the assembly of hepatocyte-targeted lipoplexes that display asialoorosomucoid and that are well tolerated by a human hepatoma cell line in which transfection is demonstrably achieved by receptor mediation. Favorable size and charge ratio characteristics suggest that this system may be suitable for in vivo application.
Introduction
The development of non-viral gene delivery systems with the design capacity for application in humans continues to receive close attention as effective alternatives are being sought to virus-based modalities. The capacity to target the gene(s) of interest to specific cell types is a key requirement in this pursuit. In this regard receptor-mediated gene delivery is proving to be a particularly promising avenue. This approach may be studied in detail in liver hepatocytes which express the asialoglycoprotein receptor (ASGP-R) selectively. The receptor, which is found on the sinusoidal face of the parenchymal cells (CitationWu et al., 2002) has a high affinity for the galactose-terminating heteroglycans of asialoglycoproteins (ASGPs) (CitationBilder et al., 1995) and can be studied in vitro in the human hepatoma hepatocyte-derived cell line HepG2, in which it is displayed abundantly (CitationSchwartz et al., 1982). Several DNA delivery vehicles have therefore been designed to incorporate ASGPs as the hepatocyte-targeting component.
This approach was first demonstrated with polyplexes comprising asialoorosomucoid (AOM) cross-linked to the polycation poly-L-lysine, to which the plasmid pSV2CAT was electrostatically bound. Receptor-mediated delivery of the construct was shown initially in HepG2 cells (CitationWu & Wu, 1986) and later in vivo in rat liver (CitationWu & Wu, 1987). It has since emerged that the D-galactopyranosyl moiety alone, if correctly presented, will ensure ASGP-R recognition of poly-L-lysine-based polyplexes (CitationMidoux et al., 1993; CitationPerales et al., 1994; CitationHashida et al., 1998; CitationNishikawa et al., 1998; CitationHan & Yeom, 2002; CitationSakai et al., 2005) and polyethylenimine-based polyplexes (CitationZanta et al., 1997; CitationBettinger et al., 1999; CitationKunath et al., 2003; CitationSakai et al., 2005). It has, however, been demonstrated that these polycations induce apoptosis in a number of human cell lines and efforts are underway to reduce toxicities by chemical elaboration (CitationHunter, 2006). Liposomes, which generally exhibit low cytotoxicity, have also been engineered to deliver DNA via the ASGP-R. Thus, Hashida and co-workers have developed galactosylated liposomes incorporating the Gal-C4-Chol derivative for in vitro and in vivo gene delivery (CitationKawakami et al., 1998; CitationManagit et al., 2005, respectively). In a refinement to the system, novel liposomes have been enabled with a ‘proton sponge’ capability to facilitate endosomal escape of transfecting lipoplexes (CitationShigeta et al., 2007). In a recent study galactosylated cationic liposomes have been prepared from 3β[N-(N’,N’-dimethylaminopropane)-carbamoyl] cholesterol (Chol-T), dioleoylphosphatidylethanolamine (DOPE), and cholesteryl-β-D-galactopyranoside or its α-anomer. Transfection experiments in HepG2 cells reveal that lipoplexes bearing the β-linked D-glycoside are 4-times more effective than lipoplexes displaying the α-D-galactopyranose at the membrane surface (CitationSingh et al., 2007). This strongly suggests that the ASGP-R is still able to discern the anomeric difference, even when the hexose is anchored to the liposome bilayer without a spacer. Accessibility of the galactopyranosyl moieties for receptor recognition, however, may be compromised by the presence of bulky DNA in lipoplexes. Pitard and coworkers have addressed this issue by placing galactose units at the extremities of polyethylene glycol units which form a protective cloud around a core of DNA condensed onto cationic bis(guanidinium)-tren-cholesterol liposomes (CitationLetrou-Bonneval et al., 2008). Thus, cholesterol-anchored cationic cytofectins, which are commonly formulated into liposomes for DNA delivery, when combined with galactose functionalities afford liposomes capable of DNA delivery to cells of hepatic origin.
In attempts to ensure more prominent display of the galacto entities, natural ligands for the ASGP-R have been linked to DNA-bearing liposomes in a multimodular fashion. Hence, asialofetuin-modified lipoplexes have delivered and ensured long-term expression of human α1-antitrypsin (hAAT) gene in mice (CitationDasi et al., 2001), while more recently they have been shown to mediate high transfection activity in HepG2 cells (Citationde Ilarduya, 2010). Cationized AOM has been covalently and non-covalently linked to Chol-T lipoplexes, which have transfected HepG2 cells efficiently by ASGP-R uptake (CitationSingh & Ariatti, 2003; CitationSingh et al., 2001, respectively). Therefore, decoration of lipoplexes with hepatocyte-directing ASGPs merits further attention in attempts to develop efficient gene carriers for delivery to the liver. In recent reports it has been demonstrated that the biotin–streptavidin interaction may be used successfully in the preparation of immunoliposomes directed towards CD38, which is upregulated in many lymphoid tumors (CitationOrciani et al., 2008), and towards degenerating cells in the necrotic regions of H460 tumors in nude mice (CitationPan et al., 2008). In these and earlier studies on transferrin-bearing lipoplexes (CitationSingh et al., 2006), liposomes and the protein ligands were biotinylated and streptavidin provided the cohesive force between the biotinylated species. We report here on the first application of the strong non-covalent biotin–streptavidin interaction to secure AOM to biotinylated cationic liposomes in multimodular lipoplexes (). Moreover, we show transfection of human hepatoma HepG2 cells by ASGP-R-mediated cell entry of the lipoplexes. In addition, electron microsocopy studies carried out on lipoplexes reveal clusters of nano-sized vesicles small enough for entry through epithelial fenestrations of human liver sinusoids.
Figure 1. Schematic representation of the cell-specific modular complex formed between DNA, biotinylated liposomes, streptavidin, and dibiotinylated AOM. Chol-T is the liposome cationic component. B = biotin; S = streptavidin; AOM = asialoorosomucoid; R = asialoglycoprotein receptor. Drawing not to scale.
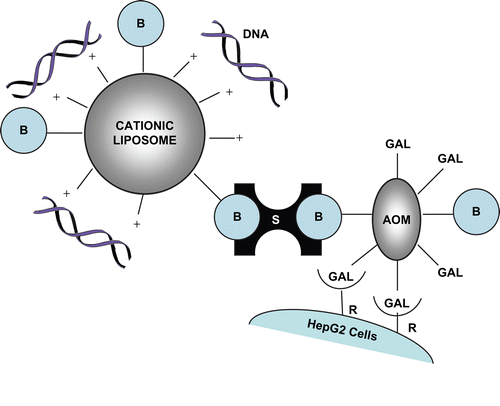
Materials and methods
The bicinchoninic acid (BCA) Protein Assay kit, 1,2-dioleoyl-sn-glycero-3-phosphoethanolamine (DOPE), human orosomucoid, insoluble neuraminidase from Clostridium perfringens type IVA, streptavidin, N-(2-hydroxyethyl)piperazine-N’-(2-ethanesulfonic acid) (HEPES), and biotin were purchased from Sigma Chemicals (St. Louis, MO). Plasmids pBR322 and pGL3 were from Roche Applied Science (South Africa) and 6-aminocaproic acid was from Merck (Darmstadt, Germany). Penicillin–streptomycin mixtures were obtained from Whittaker Bioproducts (Walkerville, MD). Biotinylcholesterylformylhydrazide (MSB1) was prepared from cholesteryl chloroformate, hydrazine, and the N-hydroxysuccinimide ester of biotin, as described elsewhere (CitationSingh et al., 2006). Tritiated aminohexanoylbiotin-N-hydroxysuccinimide (230 µCi/mmole) was synthesized from [3H] biotin-N-hydroxysuccinimide (541 mCi/mmole, Amersham, UK) by the method of CitationWilchek and Bayer (1990). 3β[N-(N’,N’-dimethylaminopropane)-carbamoyl] cholesterol (Chol-T) was prepared from cholesteryl chloroformate and N,N-dimethylaminopropylamine (CitationSingh et al., 2001). All other reagents were of analytical grade and Ultrapure water (Milli-Q50) was used throughout.
Streptavidin aminohexanoyl biotinylated asialoorosomucoid (streptavidin(bio2-AOM))
The desialylation of orosomucoid to afford AOM was achieved using agarose-bead-immobilized neuraminidase by a method adapted from that of CitationKawasaki and Ashwell (1977). Sialic acid release was followed by the thiobarbituric acid assay (CitationWarren, 1959) and the AOM was examined for homogeneity by HPLC on a TSK-GEL-DEAE-5W column eluted with 0.1 M sodium acetate (pH 4.0). To a solution of AOM (2.44 mg, 0.061 µmol) in 0.15 M NaCl (0.5 ml) was added 0.5 M NaHCO3 (0.1 mL) and aminohexanoyl-biotin-N-hydroxysuccinimide (0.083 mg, 0.183 µmol) in DMF (9 µL). The reaction mixture was incubated at 20°C in the dark for 2 h and thereafter dialysed at 4°C against 0.15 M NaCl exhaustively. The biotinylated AOM was stored at −10°C. To determine the extent of biotinylation, a separate preparation of the aminohexanoyl biotinylated AOM was made in the presence of [3H] aminohexanoyl-biotin-N-hydroxysuccinimide and radioactivity present in the product was measured by liquid scintillation. The biotin:AOM mole ratio was found to be 2:1. A solution of streptavidin (1 mg, 0.017 µmol) in 0.9 ml 0.2 M NaCl, 5 mM Tris-HCl (pH 7.6) was mixed with bio2-AOM (0.64 mg, 0.16 µmol) and incubated at 20°C for 1 h in the dark. Sterile glycerol was added to the mixture (1:1 v/v) before storage at −20°C.
Preparation of cationic liposomes containing biotinylcholesterylformylhydrazide (MSB1)
The liposome components were formulated in a 48:2:50 mole ratio of Chol-T:MSB1:DOPE, with the phospholipid fixed at 2 µmol. Liposomes were prepared by the method of CitationGao and Huang (1991). Briefly, liposome components (4 µmol total lipid) were dissolved in CHCl3 and deposited as a thin film on the inner walls of a test tube by rotary evaporation of solvent. Volatile residues were removed overnight in a drying pistol. The film was rehydrated in 1 mL sterile HEPES buffer for 14 h (20 mM HEPES, 150 mM NaCl, pH 7.5). The suspension was vortexed and sonicated for 5 min at 20°C in a Transsonic bath-type sonicator. The resulting liposome suspension was stored at 4°C. No visible aggregation was noted on storage for several months. The preparation was routinely checked in mobility shift assays (results not shown).
Cationic liposome:DNA:streptavidin(bio2-AOM) interactions
Mixtures (25 µl) containing pGL3 plasmid DNA (1 µg), biotinylated cationic liposomes (3 and 4 µg), and streptavidin(bio2-AOM) (9–11 µg) in HEPES-buffered saline (HBS) (20 mM HEPES, 150 mM NaCl, pH 7.5) were incubated for 30 min at 20°C. Liposome–DNA complexes were formed first, after which the streptavidin(bio2-AOM) component was introduced. Samples were then subjected to electrophoresis in 1% agarose gels at 70 V for 60 min in a buffer containing 36 mM Tris-HCl, 30 mM sodium phosphate, and 10 mM EDTA (pH 7.5). Gels were stained with ethidium bromide (1 µg/mL) and viewed under transillumination at 300 nm.
Ethidium displacement assay
The assay was conducted in a Shimadzu RF-551 spectrofluorometer set to excitation and emission wavelengths of 520 and 600 nm, respectively. Relative fluorescence was set at zero in HBS (500 µL) containing ethidium bromide (1 µg). Plasmid pBR322 DNA (6 µg) was added and fluorescence set at 100%. Thereafter, aliquots of biotinylated cationic liposomes were added in a stepwise manner and relative fluorescence was monitored up to 30 µg.
Electron microscopy of liposomes and lipoplexes
Liposome suspension (50 µL) was added to bovine serum albumin (5% w/v) in Tris-HCl (0.1 M, pH 7.5, 100 µL). Glutaraldehyde (2 %, 50 µL) was added to induce the formation of a gel which was then treated with OsO4 (24 h). This was followed by stepwise dehydration (70–100% ethanol). Thereafter, samples were placed in propylene oxide (20 min) followed by propylene oxide: Spurr’s resin (1:1, v/v). Finally, samples were incubated in Spurr’s resin for 45 min at 60°C. Sections were collected on C-200 copper grids, stained with uranyl acetate (10 min) and lead citrate (10 min), then viewed in a Jeol 1010 transmission electron microscope at 60 kV. Lipoplex suspensions (50 µL) on parafilm were treated with uranyl acetate (5%, 50 µL) for 3 min. Formvar-coated grids (matt surface) were brought into contact with the suspension (3 min). Grids were air-dried overnight and viewed in a Jeol 1010 transmission electron microscope at 60 kV.
Cell culture
HepG2 cells (Highveld Biologicals Pty Ltd., Kelvin, South Africa) were propagated at 37°C in 25 cm2 flasks in 5 mL of minimum essential medium (MEM) with Earle’s salts (Gibco-BRL, Life Technologies Ltd., Inchinnan, Scotland) supplemented with 20 mM HEPES (pH 7.5), 10% fetal bovine serum (Delta Bioproducts, Johannesburg, South Africa), penicillin G (100 U/mL), and streptomycin (100 µg/mL). Cultures were routinely trypsinized (0.25% w/v trypsin, 0.1% w/v EDTA) and split 1:3 after 4–5 days.
Growth inhibition assays
The toxicity of lipoplexes containing biotinylated cationic liposomes, pGL3 plasmid DNA, and streptavidin(bio2-AOM) was determined in the human hepatoblastoma cell line HepG2. Cells were trypsinized and seeded into 24-well plates at a density of 2 × 104 cells per well in complete medium. Plates were incubated for 24–36 h to permit cells to achieve semi-confluence. Medium was removed and after washing with PBS (2 × 0.5 mL) serum-free medium was added (0.5 mL/well). Streptavidin(bio2-AOM):liposome:DNA complexes were assembled 30 min prior to introduction to cells by adding streptavidin(bio2-AOM) (9 µg) to a mixture of biotinylated cationic liposomes (2–5 µg) and pGL3 DNA (1 µg) in a final volume of 25 µl HBS. Modular lipoplexes were introduced to cells and after 4 h at 37°C the medium was replaced with complete medium (MEM + 10% FBS + antibiotics) and cells were incubated for a further 48 h. Cell numbers were determined by a method adapted from that of CitationSchellekens and Stitz (1980). Briefly, wells were drained and washed twice with PBS and cells stained with crystal violet solution (0.5% w/v crystal violet, 0.8% w/v sodium chloride, 5% v/v formaldehyde, 50% v/v ethanol) for 20 min. After washing with water and air-drying, stain was extracted into 2-methoxyethanol over 36 h. Absorbances were read at 550 nm.
Transfection of HepG2 cells with streptavidin(bio2-AOM):pGL3 DNA:liposome lipoplexes
Cells were seeded into 24-well plates (2 × 104 cells/well) and grown at 37°C for 24–36 h to semiconfluence. Lipoplexes were assembled from pGL3 plasmid DNA (1 µg), biotinylated cationic liposomes (2–5 µg), and streptavidin(bio2-AOM) (9 µg) in HBS (25 µl) 30 min prior to incubation with cells. Complexes were introduced to cells bathed in serum-free medium (0.5 mL) and incubated for 4 h, whereupon medium was replaced by complete medium. In competition assays, asialofetuin (300 µg) was included in incubations. After a further 48 h, cells were lysed and assayed for luciferase activity using the Promega Luciferase Assay System. The concentration of soluble protein in lysate supernatants was determined by the BCA assay using BSA as the standard.
Results and discussion
Cationic liposomes
Cationic liposomes were formulated to contain Chol-T for plasmid DNA binding and the co-lipid DOPE, which has a small polar head group and large hydrophobic component and which adopts an inverted hexagonal structure. The combination of this phospholipid and many monocationic cholesteryl derivatives in near equimolar ratios is known to promote the formation of stable unilamellar liposomes (CitationGao & Huang, 1991; CitationSingh et al., 2001; CitationPiperno-Neumann et al., 2003; CitationIslam et al., 2009). Furthermore, the biotinylated cholesteryl derivative MSB1 was included at 2% on a mol/mol basis. It was deemed necessary to display the biotin moiety in low abundance on liposomes to reduce the possibility of macro assembly formation by extensive cross-linking on introduction of the streptavidin(bio2-AOM). Liposome preparations were examined by transmission electron microscopy for size and lamellarity. Images () revealed unilamellar vesicles with a size distribution in the 300–1000 nm range. However, these large liposomes have been shown previously to form complexes with DNA that present as clusters (100–200 nm) composed of small unilamellar vesicles in the 30–50 nm size range (CitationSingh et al., 2006). These observations are consistent with the ultrastructure reported for lipoplexes formed between liposomes containing the monocationic cholesteryl cytofectin trimethylaminoethane carbamoyl cholesterol (TMAEC-chol) and the plasmid pCMV-β (CitationCao et al., 2000).
Liposome:pGL3 plasmid DNA interactions
The association between pGL3 DNA and biotinylated cationic liposomes was examined in a fluorometric assay in which the liposome-induced displacement of the DNA-intercalated planar fluorophore ethidium was followed by measuring changes in relative fluorescence (CitationXu et al., 1999). Thus, no further drop in fluorescence was observed above a DNA:liposome ratio of 1:3 (w/w), corresponding to a plasmid DNA (–ve):cationic liposome (+ve) charge ratio of 1:1 (), signifying no additional DNA condensation at higher liposome levels. The maximum reduction in fluorescence was 45%, pointing to a moderately high degree of DNA condensation by cationic liposomes at a charge ratio of 1:1.
Cationic liposome:pGL3 DNA:streptavidin(bio2-AOM) assemblies
Although complete liposome association of plasmid DNA was achieved at a ratio of 1:3 (w/w) in a binary system (), the introduction of streptavidin(bio2-AOM) partially displaced the plasmid DNA at this ratio. This was clearly evident in band shift assays (, lanes 2–4). To overcome this effect, lipoplexes were assembled with proportionately higher levels of biotinylated cationic liposomes (DNA:liposome, 1:4, w/w). Streptavidin(bio2-AOM) was accommodated in a final DNA:liposome:streptavidin(bio2-AOM) ratio of 1:4:9 (w/w/w) without DNA displacement (, lane 6). However, at higher levels of streptavidin(bio2-AOM), unbound plasmid was in evidence (, lanes 7 and 8). The results clearly suggest that the presence of streptavidin(bio2-AOM) on the liposome bilayer partially masks some positively-charged Chol-T dimethylammonium head groups on the outer leaflet of the liposome membrane, therefore reducing the available positive charges for electrostatic interaction with the plasmid DNA. A similar steric crowding effect has been observed with PEGylated liposomes displaying triantennary galactose units at the distal ends of the polyethyleneglycol chains (CitationFrisch et al., 2004). Ternary assemblies that afforded the highest transfection activity viz. pGL3 DNA:liposome:streptavidin(bio2-AOM) 1:4:9 (w/w/w) were sampled for ultrastructural analysis by transmission electron microscopy 30 min after formation and just prior to transfection experiments. Electron micrographs showed 200–300 nm aggregates of 50–80 nm beads (). These assemblies have been constructed with the intended capacity to enter hepatocytes in vivo by receptor mediation. The size of transfecting assemblies is therefore of some importance as they are to pass through the fenestrations of the liver sinusoidal endothelial cells (LSEC) which act as dynamic filters (CitationBraet & Wisse, 2002). Human fenestrae, which have been shown to range from 50–300 nm in diameter (CitationHorn et al., 1987) are therefore theoretically permeable to the unopsinized lipoplexes. Thereafter, receptor mediated entry into the cell is via coated pits. CitationZuhorn et al. (2002) have suggested that clathrin-mediated endocytosis of particles exceeding diameters of 200 nm is possible and that there is considerable flexibility in the size of resultant coated vesicles.
Figure 4. Band shift assay of modular lipoplex assemblies. Plasmid pGL3 (1 µg) in 25 µl HBS was incubated with cationic liposomes (lanes 2–4, 3 µg; lanes 6–8, 4 µg) and streptavidin(bio2-AOM) (lanes 2–4 and 6–8, 9, 10, and 11 µg, respectively). After 30 min samples were subjected to electrophoresis on 1% agarose gels.
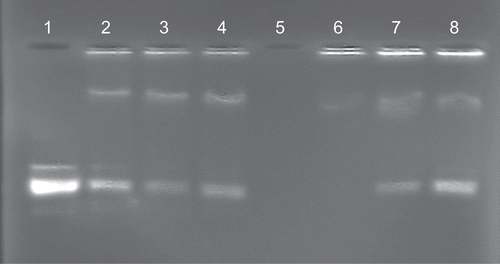
Growth inhibition assays
Cytotoxicity studies were carried out on HepG2 cells with modular lipoplexes in which the pGL3 DNA:streptavidin(bio2-AOM) ratio was maintained at 1:9 (w/w), but biotinylated cationic liposome content was varied. The assay, developed by CitationSchellekens and Stitz (1980) for the measurement of growth inhibition of cells grown in monolayer, revealed that toxicity increased with increasing liposome concentration (). Thus, the maximal growth inhibition (40%) was recorded at a pGL3 DNA:liposome:streptavidin(bio2-AOM) ratio of 1:5:9 and a total of 15 µg of lipoplex per well in 0.5 ml medium. Inhibition at the optimal transfection ratio 1:4:9 was, however, 25%. This is comparable to levels obtained with ternary complexes constructed from cationized AOM, pRSVL DNA, and Chol-T liposomes reported elsewhere (CitationSingh & Ariatti, 2003).
Figure 5. Growth inhibition studies of modular lipoplexes in HepG2 cells. Cells in 24-well plates bathed in medium (0.5 ml) were exposed to preformed complexes containing pGL3 DNA (1 µg), streptavidin(bio2-AOM) (9 µg), and varying amounts of biotinylated liposomes (2–5 µg in 1 µg increments). Data are presented as means ± SD (n = 4).
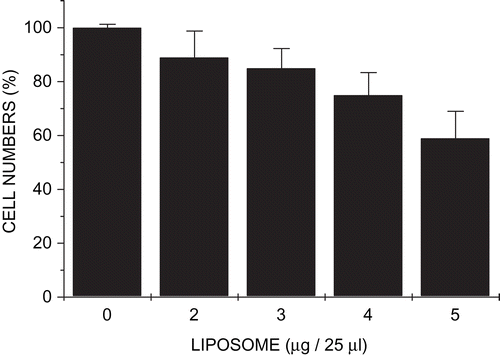
Transfection activity of lipoplexes
Gene transfer experiments were conducted with targeted lipoplexes containing 2–5 µg liposomes, 1 µg pGL3 DNA, and 9 µg streptavidin(bio2-AOM) in 0.5 ml medium, bathing semi-confluent HepG2 cells. It was found that the transfection activity increased with increasing liposome content up to a maximum reached at a pGL3DNA:liposome:streptavidin(bio2-AOM) ratio of 1:4:9 corresponding to DNA (−ve): liposome (+ve) charge ratio of 1:1 The inclusion of competing asialofetuin in incubation mixtures reduced significantly transfection activity of all lipoplexes up to 10-fold (). These findings strongly support the notion that lipoplexes gain entry into hepatocyte-derived cells by ASGP-R mediation and demonstrate that biotinylated AOMs are still recognized by the receptor even when they form part of a multicomponent lipoplex.
Figure 6. Transfection assays. Cells were treated with modular lipoplexes containing pGL3 DNA (1 µg), streptavidin(bio2-AOM) (9 µg), and increasing amounts of biotinylated liposomes (2–5 µg in 1 µg increments). In competition assays incubation mixtures included asialofetuin (300 µg) (light grey bars). Control 1 contained only cells. Control 2 contained cells with naked plasmid DNA. Data are shown as means ± SD (n = 4). ** p < 0.01, Student’s t-test.
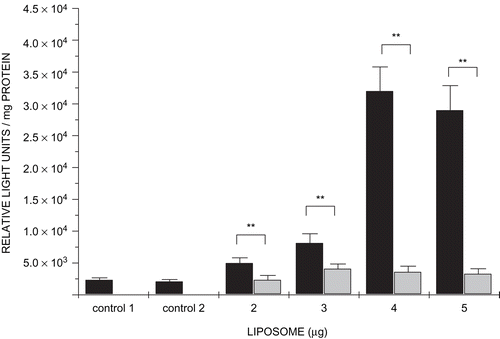
Conclusion
In the strategy adopted here to target lipoplexes to the ASGP-R, which is almost exclusively expressed on hepatocytes in the body and which may be studied in the hepatoblastoma cell line HepG2, streptavidin has served to link biotinylated DNA-binding cationic liposomes to biotinylated AOM. To ensure prominent display of biotin and therefore its streptavidin-accessibility, a 6 carbon spacer element has been included between the biotinyl moieties and the AOM. The lipoplexes are of a non-covalent nature and their assembly relies on an initial electrostatic interaction to anchor the DNA component to the liposomes followed by the streptavidin-biotin interaction to tether the targeting asialoglycoprotein to the lipoplex. Assembly is therefore ordered and controlled. Furthermore, we demonstrate here that these stable pGL3 DNA:liposome:streptavidin(bio2-AOM) multimodular lipoplexes enter HepG2 cells via the ASGP-R route. This was evidenced by markedly reduced levels of luciferase activity when transfections were carried out in the presence of the competing ligand asialofetuin. It has been pointed out that complexes carrying an excess positive charge are generally cytotoxic to cells and do not permit specific transfection (CitationLetrou-Bonneval et al., 2008). Here we have generated lipoplexes in which the DNA (−ve): cationic liposome (+ve) charge ratio is 1:1, and have tagged them with the targeting AOM component in a non-electrostatic manner. Moreover, the sizes of transfecting complexes are generally within the permissible limits of liver sinusoidal fenestrations, suggesting that this system may be profitably assessed in vivo. The modular nature of the lipoplexes discussed here clearly points to a broader application of the approach in which constructs could be targeted to specific cell types by assembling biotinylated lipoplexes from biotinylated liposomes, streptavidin, and biotinylated ligands such as insulin, growth factors, and antibodies.
Declaration of interest
The authors report no conflicts of interest. The authors alone are responsible for the content and writing of the paper.
References
- Bettinger, T., Remy, J.S., Erbacher, P. (1999). Size reduction of galactosylated PEI/DNA complexes improves lectin-mediated gene transfer into hepatocytes. Bioconj Chem. 10:558–61.
- Bilder, M.C., Cescato, R., Neno, P., Spiess, M. (1995). High affinity ligand to subunit H1 of asialoglycoprotein receptor in the absence of subunit H2. Eur J Biochem. 230:207–12.
- Braet, F., Wisse, E. (2002). Structural and functional aspects of liver sinusoidal endothelial cell fenestrae: a review. Comparative Hepatol. 1:1.
- Cao, A., Briane, D., Coudert, R., Vassy, J., Lievre, N., Olsman, E., Tamboise, E., Salzmann, J.L., Rigaut, J.P., Taillandier, E. (2000). Delivery and pathway in MCF7 cells of DNA vectorized by cationic liposomes derived from cholesterol. Antisense Nucleic Acid Drug Devel. 10:369–80.
- Dasi, F., Benet, M., Crespo, J., Crespo, A., Alino, S.F. (2001). Asialofetuin liposome-mediated human alpha1-antitrypsin gene transfer in vivo results in stationary long-term gene expression. J Mol Med. 79:205–12.
- De Ilarduya, C.T. (2010). Serum-resistant lipoplexes in the presence of asialofetuin. Methods Mol Biol. 605:425–34.
- Frisch, B., Carriere, M., Largeau, C., Mathey, F., Masson, C., Schuber, F., Scherman, D., Escriou, V. (2004). A new triantennary galactose-targeted PEGylated gene carrier, characterization of its complex with DNA and transfection of hepatoma cells. Biocon Chem. 15:754–64.
- Gao, X., Huang, L. (1991). A novel cationic liposome reagent for efficient transfection of mammalian cells. Biochem Biophys Res Commun. 179:280–5.
- Han, J., Yeom, Y.I. (2002). Specific gene transfer mediated by galactosylated poly-L-lysine into hepatoma cells. Int J Pharm. 202:151–60.
- Hashida, M., Takemura, S., Nishikawa, M., Takahura, Y. (1998). Target delivery of plasmid DNA complexed with galactosylated poly(L-lysine). J Contr Rel. 54:301–10.
- Horn, T., Christoffersen, P., Henriksen, J.H. (1987). Alcoholic liver injury: defenestration in noncirrhotic livers: a scanning electron microscope study. Hepatol. 7:77–82.
- Hunter, A.C. (2006). Molecular hurdles in polyfectin design and mechanistic background to polycation induced cytotoxicity. Adv Drug Deliv Rev. 58:1523–31.
- Islam, U.R., Hean, J., van Otterlo, W.A.L., de Koning, C.B., Arbuthnot, P. (2009). Efficient nucleic acid transduction with lipoplexes containing novel piperazine- and polyamine-conjugated cholesterol derivatives. Bioorg Med Chem Lett. 19:100–3.
- Kawakami, S., Yamashita, F., Nishikawa, M., Takahura, Y., Hashida, M. (1998). Asialoglycoprotein receptor-mediated gene transfer using novel galactosylated cationic liposomes. Biochem Biophys Res Commun. 252:78–83.
- Kawasaki, T., Ashwell, G. (1977). Isolation and characterization of an avian hepatic binding protein specific for N-acetylglucosamine-terminated glycoproteins. J Biol Chem. 252:6536–43.
- Kunath, K., von Harpe, A., Fischer, D., Kissel, T. (2003). Galactose-PEI-DNA complexes for targeted gene delivery: degree of substitution affects complex size and transfection efficiency. J Contr Rel. 88:159–72.
- Letrou-Bonneval, E., Chèvre, R., Lambert, O., Costel, P., André, C., Tellier, C., Pitard, B. (2008). Galactosylated multimodular lipoplexes for specific gene transfer into primary hepatocytes. J Gene Med. 10:1198–209.
- Managit, C., Kawakami, S., Yamashita, F., Hashida, M. (2005). Effect of galactose density on asialoglycoprotein receptor-mediated uptake of galactosylated liposomes. J Pharm Sci. 94:2266–75.
- Midoux, P., Mendes, C., Legrand, A., Raimond, J., Mayer, R., Monsigny, M., Roche, A.C. (1993). Specific gene transfer mediated by lactosylated poly-L-lysine into hepatoma cells. Nucleic Acids Res. 21:871–8.
- Nishikawa, M., Takemura, S., Takakura, Y., Hashida, M. (1998). Targeted delivery of plasmid DNA to hepatocytes in vivo: optimization of the pharmacokinetics of plasmid DNA/galactosylated poly(L-lysine) complexes by controlling their physicochemical properties. J Pharmacol Exp Ther. 287:408–15.
- Orciani, M., Cavaletti, G., Fino, V., Mattioli-Belmonte, M., Tredici, G., Bruni, P., Di Primio, R. (2008). Exploiting CD38-mediated endocytosis for imunoliposome internalization. Anticancer Drugs. 19:599–605.
- Pan, H., Han, L., Chen, W., Yao, M., Lu, W. (2008). Targeting to tumor necrotic regions with biotinylated antibody and streptavidin modified liposomes. J Contr Rel. 125:228–35.
- Perales, J.C., Ferkol, T., Bergen, H., Ratnoff, O.D., Hanson, R.H. (1994). Gene transfer in vivo: sustained expression and regulation of genes introduced into the liver by receptor-mediated uptake. Proc Natl Acad Sci USA. 91:4086–90.
- Piperno-Neumann, S., Oudar, O., Reynier, P., Briane, D., Cao, A., Jaurand, M.C., Naejus, R., Kraemer, M., Breau, J.L., Taillandier, E. (2003). Transfer into a mesothelioma cell line of tumor suppressor gene p16 by cholesterol-based cationic lipids. Biochim Biophys Acta. 1611:131–9.
- Sakai, M., Nishikawa, M., Thanaketpaisarn, O., Yamashita, F., Hashida, M. (2005). Hepatocyte-targeted gene transfer by combination of vascularly delivered plasmid DNA and in vivo electroporation. Gene Ther. 12:607–16.
- Schellekens, H., Stitz, L.W. (1980). Simple method for measuring growth inhibition by interferon of cells in monolayer. J Virol Meth. 1:197–200.
- Schwartz, A.L., Genze, H.J., Lodish, H.F. (1982). Recycling of the asialoglycoprotein receptor: biochemical and immunocytochemical evidence. Philos Trans R Soc Lond B Biol Sci. 300:229–35.
- Shigeta, K., Kawakami, S., Higuchi, Y., Okuda, T., Yagi, H., Yamashita, F., Hashida, M. (2007). Novel histidine-conjugated galactosylated cationic liposomes for efficient hepatocyte-selective gene transfer in human hepatoma HepG2 cells. J Contr Rel. 118:262–70.
- Singh, M., Ariatti, M. (2003). Targeted gene delivery into HepG2 cells using complexes containing DNA, cationized asialoorosomucoid and activated cationic liposomes. J Contr Rel. 92:383–94.
- Singh, M., Hawtrey, A., Ariatti, M. (2006). Lipoplexes with biotinylated transferrin accessories: novel, targeted, serum tolerant gene carriers. Int J Pharm. 321:124–37.
- Singh, M., Kisoon, N., Ariatti, M. (2001). Receptor-mediated gene delivery to HepG2 cells by ternary assemblies containing cationic liposomes and cationized asialoorosomucoid. Drug Deliv. 8:29–34.
- Singh, M., Rogers, C.B., Ariatti, M. (2007). Targeting of glycosylated lipoplexes in HepG2 cells: anomeric and epimeric preference of asialoorosomucoid receptor. S Afr J Sci. 103:204–10.
- Warren, L. (1959). The thiobarbituric acid assay for sialic acid. J Biol Chem. 234:1971–5.
- Wilchek, M., Bayer, E.A. (1990). Avidin and streptavidin-containing probes. Methods Enzymol. 184:123–38.
- Wu, G., Nantz, M.H., Zern, M.A. (2002). Targeting of hepatocytes for drug and gene delivery: emerging novel approaches and applications. Front Biosci. 7:717–25.
- Wu, G., Wu, C.H. (1986). Receptor-mediated in vitro gene transformation by a soluble DNA carrier system. J Biol Chem. 262:4429–32.
- Wu, G., Wu, C.H. (1987). Receptor-mediated gene delivery and expression in vivo. J Biol Chem. 263:14621–4.
- Xu, Y., Hui, S.-W., Frederik, P., Szoka, F. (1999). Physicochemical characterization and purification of cationic liposomes. Biochem J. 77:341–53.
- Zanta, M.A., Boussif, O., Abid, A., Behr, J.P. (1997). In vitro gene delivery to hepatocytes with galactosylated polyethylenimine. Bioconj Chem. 8:839–44.
- Zuhorn, I.S., Kalicharan, R., Hoekstra, D. (2002). Lipoplex-mediated transfection of mammalian cells occurs through the cholesterol-dependant clathrin-mediated pathway of endocytosis. J Biol Chem. 277:18021–8.