Abstract
Drug delivery systems based on synthetic polymers are widely employed in the treatment of several pathologies. In particular, the use of implantable devices able to release one or more active principles in a topic site with a controlled delivery kinetic represents an important improvement in this field. However, the release kinetic, that could be affected by different parameters, like polymer composition or chemical nature and initial drug loading, represents one of the problems related to the implantation of delivery systems. In this study, acrylic membranes with different macromolecular composition were prepared and studied analyzing delivery kinetic properties. Drug delivery systems were prepared using as matrix the copolymer poly(methylmethacrylate-co-butylmethacrylate) in three different compositions and folic acid (less hydrophobic) or Paclitaxel (more hydrophobic) as drugs, to evaluate the effect of macromolecular composition and hydrophilicity degree on the release properties. In addition, the effect of the initial drug loading was considered, loading drug delivery systems with four different initial drug percentages. Results showed a direct dependence of kinetics from macromolecular composition, hydrophilicity degree of solutes, and initial drug loading, allowing one to conclude that it is possible to design and to develop drug delivery systems starting from poly(methylmethacrylate-co-butylmethacrylate) matrices with specific properties by varying these three parameters.
Introduction
The employment of polymeric platforms in drug delivery is a significant and promising technique adopted in a wide range of treatments of several pathologies (CitationJanát-Amsbury et al., 2000; CitationNagarwal et al., 2009; CitationSosnik et al., 2009). The practice to incorporate solutes in polymeric matrices with different chemical properties is considered a good approach in the delivery of different active principles. In particular, poorly soluble drugs administration represents a usual problem, difficult to resolve using conventional formulation approaches. For this reason, different polymer/drug systems were developed (CitationGao et al., 2008; CitationMin et al., 2008; CitationZeng & Sun, 2009). Some polymeric platforms are in fact able to release hydrophilic and/or hydrophobic active principles, and the delivery rate is dependent on chemical nature of polymers, interaction between polymer and drug, and interactions between delivery system and surrounding environment.
Regarding hydrophobic drugs, the therapeutic efficiency of several active principles is often limited by the poor aqueous solubility and systemic toxicity (CitationMikhail & Allen, 2009). In addition, side-effects due to the toxicity towards healthy tissues limit the administration doses, compromising the effectiveness of the treatment (CitationVan Zuylen et al., 2001). The common practice to incorporate hydrophobic and poor water-soluble drugs within biocompatible materials has been shown to significantly reduce systemic toxicity and in the meanwhile to increase the drug solubility (CitationTorchilin, 2004; CitationLiu et al., 2006; CitationNishiyama & Kataoka, 2006; Silvestri et al., Citation2009a). Novel drug delivery systems are currently studied and developed to optimize drug release profiles with the aim to be employed in different therapeutic areas. Commonly, biodegradable materials that allow a high cumulative release, a long-term treatment, and the biocompatibility of biodegradation products represent the most employed materials in controlled drug delivery areas. In such applications, where adhesion, surface properties, and mechanical characteristics are particularly important, the use of biocompatible but biostable materials is widely diffused. The coating of implantable devices in cardiovascular fields (coronary stents, vascular grafts, annuloplasty rings, etc.) or orthopedic applications is the most evident example of applicability of these materials.
In the present work, a novel poly(methylmethacrylate-co-butylmethacrylate) copolymer was studied as a drug delivery platform. Transport properties were analyzed considering two solute molecules: folic acid, more soluble in aqueous environment, and Paclitaxel, less soluble in water. The releasing profiles were analyzed using two kinetic models, proposed by Higuchi and Korsmeyer, and kinetic parameters were quantified. Also, the diffusion coefficients were evaluated. Finally, the hydrophilicity degree of the matrices was studied through hydrophilicity tests for a best interpretation of the drug release properties.
Materials and methods
Materials employed as delivery platforms were three poly(methyl methacrylate-co-butyl methacrylate) (P(MMA-co-BMA)) copolymers with different macromolecular compositions. In order to compare the effect of the macromolecular composition on delivery characteristics, also homopolymers polymethylmethacrylate (PMMA) and polybutylmethacrylate (PBMA) were tested. Materials were synthesized from the authors with a monomer-starved semi-batch emulsion polymerization (Silvestri et al., Citation2009b). In the nomenclature and the composition of the copolymers are reported.
Table 1. Poly(methyl methacrylate-co-butyl methacrylate) copolymer nomenclature, percentage molar compositions and percentage weight compositions (Silvestri et al., Citation2009c).
Solutes were selected on the different chemical behavior. Two molecules with different hydrophilicity degree were employed: folic acid (FA, solubility in water in anhydrous form at 25°C: 1.6 μg/ml, Sigma®, (Milan, Italy) molecular weight 441.4 g/mol) and Paclitaxel (PLX, C47H51NO14, Sigma®, solubility in water in anhydrous form at 37°C: 0.7 μg/ml (CitationMathew et al., 1992), molecular weight 854 g/mol). Paclitaxel is a common anti-neoplastic drug used in the treatment of cancer (CitationElstad & Fowers, 2009), extensively used in the preparation of implantable drug delivery systems (CitationLiu et al., 2007; CitationWang et al., 2008; CitationElstad & Fowers, 2009). Also, folic acid is used to prepare polymeric delivery systems (CitationStevanovic et al., 2008) and in cancer treatment, in particular in the functionalization of carriers for targeted delivery of molecules (CitationTaira et al., 2007; CitationHu et al., 2009). Solvent used to prepare drug-loaded systems was tetrahydrofuran (THF, Carlo Erba Reagenti®; Milan, Italy).
Preparation of drug-loaded samples
Drug delivery platforms were prepared starting from a polymeric solution in THF (2% w/v) blended with one of the active principles. Two millilters of polymer/drug solution were deposited on a glass flat support using a specific apparatus equipped by a moveable knife, able to produce membranes with uniform and controlled thickness. The knife was positioned 200 μm above the glass plate. An electronic controller was used to move the knife at a fixed rate (1 m/min). After loading, a casting procedure was carried out in a vented oven at controlled temperature (37°C ± 1°C) for 5 h. Finally, membranes were detached from the glass support and cut into samples with a fixed-dimension area (1 cm × 1 cm). Final thickness after the casting process was evaluated using a calliper. In thicknesses, weights, and amount of drug loaded in the samples tested in this work are summarized.
Table 2. Mean values of thicknesses, weights, and amount of drug loaded in the tested samples; reported data are referred to three samples for each system.
In order to verify the complete evaporation of the organic solvent, membranes were weighted several times during the casting procedure and a FT-IR analysis was carried out on the final products, ensuring the complete absence of the solvent in the membranes.
Solute delivery tests
Polymeric membranes containing four different amounts of FA or PLX were prepared. The initial amounts of solute loaded in the membranes were 0.5%, 1%, 3%, and 5% in weight of solute in respect of the polymer weight. Drug delivery tests were carried out in a phosphate buffered solution (PBS) at pH 7.4 containing 0.05% (w/v) in sodium dodecyl sulfate (SDS, Sigma®). The use of the SDS surfactant was finalized to increase the solubility of the PLX in water. For the FA delivery tests a surfactant was generally not strictly necessary, however it was added to have the same conditions as the PLX delivery tests. In addition, the use of SDS allowed reproducing a behavior more similar to in vivo conditions. Tests were performed immersing samples in 2 ml of delivery solution. Samples were maintained in a lightly stirred bath at a fixed temperature (37°C ± 1°C) through the testing period (24 days). Withdrawals from the delivery medium were carried out at established times, the delivery solution was replaced by fresh solution after each withdrawal, in order to maintain a sink condition. The sink condition occurs when the amount of solute delivered is lower than 10–20% or even 30% of the maximum solubility of the solute in the dissolution medium (CitationCosta & Sousa Lobo, 2001). For this reason, solubility of FA and PLX in the delivery solution at 37°C was evaluated. To obtain this result, two supersaturated solutions of FA and PLX were prepared in PBS solution containing 0.05% of SDS. Then, solutions were filtered to remove the solute surplus and finally analyzed through High Performance Liquid Chromatography (HPLC).
Withdrawals from delivery medium were analyzed using HPLC. Analytical apparatus consisted of a 410 LC pump, a UV detector (Perkin-Elmer®; Waltham, MA, USA), and a chromatographic column. Chromatographic methods used to analyze solutes are reported in . Tests were carried out in triplicate.
Table 3. Chromatographic methods employed to analyze FA and PLX released amounts.
Solute delivery kinetics
Solute delivery kinetics were studied using two different mathematical models, expressed by CitationHiguchi (1963) (equation 1) and CitationKorsmeyer et al. (1983) (equation 2) equations:
where Mt represents the amount of solute released at the generic time t, M∞ is the amount of drug released when the system reaches the plateau condition, k, k’, and n represent the Higuchi kinetic constant, the Korsmeyer kinetic constant, and the release order, respectively. These equations are only valid for the first 60% of the total released drug (Ritger & Peppas, Citation1987a). Release orders allowed establishing the regime governing the release process. In particular, when n ≤ 0.5 a Fickian release occurs (Ritger & Peppas, Citation1987b).
Diffusion coefficients
Solute diffusion coefficients at early (equation 3) and late (equation 4) times were evaluated as (CitationTomic et al., 2007):
In these equations, Mt and M∞ represent the amount of solute eluted by membranes at the generic time t and at the plateau, respectively, Dearly and Dlate are the solute diffusion coefficients across the membrane at early and late times, respectively, and s is the thickness of the sample.
Hydrophilicity tests
Two different tests were performed to evaluate the hydrophilicity of polymeric materials. In particular, water contact angle tests and swelling tests were carried out.
Water contact angle tests
Water contact angle tests were performed by shedding 5 μl of water on a polymeric coating obtained by a spin-coating process on a flat glass support. Polymeric coatings were prepared by depositing 500 μl of polymeric solution in THF (2% w/v) onto the support. The spin-coating process was performed in two steps: during the first step (30 s at 800 rpm) the solution wet the glass plate, in the second step (60 s at 1500 rpm) a uniform thickness was obtained. From the contact angle measurements, the work of adhesion (equation 5) and the work of spreading (equation 6), both associated to the degree of wetting of the surface, were evaluated.
where Wa represents the energy necessary to separate the liquid phase from the solid substrate, and Ws is the free energy associated to the spreading of the liquid phase on the support. In equations (5) and (6), γ represents the surface tension and θ the measured water contact angle. Ten measurements per sample were performed.
Swelling tests
Membranes not containing drug, obtained with the same preparation method previously illustrated, were tested to evaluate the hydrophilicity degree of materials by swelling tests. Swelling tests were performed by immersing polymeric membranes with a known weight in 2 ml of pure bi-distilled water. Samples were maintained at 37°C ± 1°C through the entire period of the test, then withdrawn, blotted,and weighted after established periods, until the reaching of a constant weight. From experimental results, the percentage swelling coefficient (%SW, equation 7) and swelling kinetic parameters (equation 8) were evaluated. Results were used for a better interpretation of solute elution tests results. The percentage swelling coefficient (%SW) was evaluated with the following equation:
where w(t) represents the weight of the sample at the generic time t and w(0) the initial weight of the sample. The swelling kinetic was evaluated with the generic kinetic equation (CitationTomic et al., 2007):
In this equation, Mwater(t) and Mwater(∞) represent the mass of water absorbed by membranes at the generic time t and at the thermodynamic swelling equilibrium, respectively, ksw represents the swelling kinetic constant, nsw is the swelling kinetic order. Water diffusion coefficients at early and late times were evaluated using equations (3) and (4), substituting the amount of drug eluted with the amount of water absorbed by membranes. Five samples for each material were tested.
Results
Following, the results obtained from delivery tests are reported. In addition to experimental results, a mathematical modelization is proposed, in order to evaluate the kinetic parameters, typical for each polymer/drug system, the diffusion coefficients, and the swelling behavior.
Delivery tests
Delivery of the less hydrophobic principle
The authors first examined the release properties of the less hydrophobic solute. Following, the results obtained from FA delivery tests are reported. First of all, the perfect sink condition was verified. Solubility of FA in the PBS solution containing SDS was found to be 0.24 ± 0.04 mg/ml at 37°C. Then, the perfect sink condition can be considered satisfied if the detected concentration of FA in the delivery medium is smaller than 7.2·10−3 mg/ml, which represents the upper limit of 30% in respect to the maximum solubility. This condition was verified for all samples and then it was possible to conclude that delivery kinetic was not affected from this aspect. In the cumulative released fraction of FA from tested materials vs time is reported. In particular, considering the trend of the released fraction of FA from each material, it was noted that this parameter was, in all cases, inversely proportional to the initial load of drug. This could be owing to the different interaction between solute/polymer and solute/solute: increasing the fraction of solute initially loaded, greater interaction occurred and then a smaller percentage amount of drug was eluted from the systems. However, increasing the amount of drug loaded at the beginning of the test, the cumulative amount of solute released after 24 days () increased. This trend was not verified in the analysis of the cumulative fraction: on the contrary, the fraction tended to decrease, increasing the initial load of solute (). In addition, the released fraction was, for all systems, smaller than one, which means that part of the solute could be released after 24 days or could remain in the membrane. Further, these systems were characterized by a short-term release behavior: the most part of the released solute was always registered after 3 days.
FA delivery kinetic
Using equations (1) and (2), Higuchi constants (), Korsmeyer constants (), and release order () were evaluated. Using the Higuchi model, kinetic constants evaluated were similar and did not show a specific trend. In addition, correlations between experimental and theoretical data were comprised between 0.87–0.91. This means that this model could not accurately describe the behavior of the systems. Considering that Higuchi equation is generally used when the initial concentration of solute is much greater than the solubility limit, obtained results are an indirect evidence of a greater solubility of FA in the polymeric matrices. This evidence was strengthened by FT-IR chemical imaging analyses: the correlation maps between the mean characteristic spectrum of the FA-loaded membrane and the spectral map, obtained acquiring several spectra in a specific area of the sample (1 mm2), was evaluated and samples which resulted were homogeneous, because correlation values found were greater than 0.8 for all systems. In the correlation map obtained from analysis of copolymer P(MMA-co-BMA) 75/25 loaded with 10% of FA is reported as an example.
Table 4. Mean values of the Higuchi kinetic constants (s−0.5) evaluated for tested materials by varying the initial drug loading; in parentheses, correlations between experimental and theoretical data, evaluated using the reported kinetic constants, are listed.
Figure 3. Kinetic constants (s−n) (a) and release orders (b) related to the delivery of FA and kinetic constants (s−n) (c) and release orders (d) related to the delivery of PLX, obtained using the Korsmeyer model (equation 2).
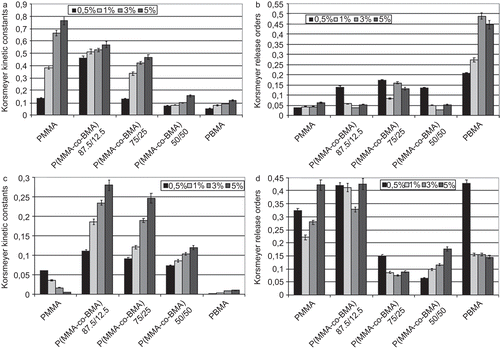
Figure 4. FT-IR chemical imaging correlation maps of poly(methylmethacrylate-co-butylmethacrylate) 75/25 loaded with 10% w/w of FA (a) and PLX (b).
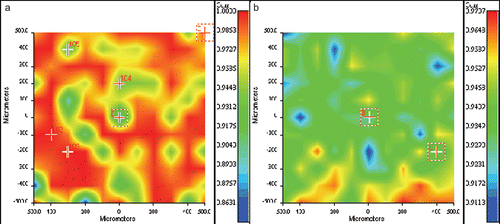
Kinetic constants obtained with the Korsmeyer model were shown to be dependent from the initial drug load. In particular, k’ increased with increasing the initial load of solute. Considering release orders, all values were smaller than 0.5 which means that the transport regime can be reasonably considered as Fickian. In this case, correlation values between theoretical models and experimental results were very high for each analyzed system and were comprised in the range from 0.98–1.
FA diffusion coefficients
In diffusion coefficients of FA from polymeric matrices at early and late times are reported. Both coefficients were found to be dependent on the initial drug load: diffusion coefficients increased with increasing the initial solute load. Also, Dearly was greater than Dlate in all cases except in systems with 0.5% of initial FA load. This could be considered symptomatic of a faster elution of the solute in the first period of the test than at late times. This hypothesis is also confirmed by the important burst effect detected in the FA release during the first 3 days of test. Particular trends were not highlighted in respect of the macromolecular composition.
Delivery of the more hydrophobic principle
In the cumulative released fraction of PLX for tested materials vs time is reported. Also in this case, the perfect sink condition was preliminarily verified. Solubility of PLX in the PBS solution containing SDS was found to be 0.08 ± 0.01 mg/ml at 37°C. Then, the perfect sink condition can be considered satisfied if the detected concentration of PLX in the delivery medium is smaller than 2.4·10−3 mg/ml, which represents the upper limit of 30% in respect of the maximum solubility. This condition was verified for all samples and then it was possible to conclude that delivery kinetic was not affected from this aspect. Also in this test, as already verified in the previous analysis of the FA delivery, the released fraction was in all cases inversely proportional to the initial load of solute. The same considerations could be proposed to explain this behavior: greater interactions between drug molecules occurred when a greater fraction of the solute was loaded. Values tended to decrease, increasing the amount of solute initially loaded (), and a sensible increase in the delivered amount was registered, increasing the amount of BMA in the macromolecules (). However, as described in the study of the FA delivery, for all systems, the released fraction was smaller than 1, which means that part of the solute could be released after 24 days. Compared to the FA releasing profiles, in this case, a small burst release, followed by an important sustained delivery, was verified. At the end of the test period, PLX delivery systems did not reach a plateau and a further massive release of solute could be hypothesized.
PLX delivery kinetic
As occurred in the analysis of the FA release kinetic using the Higuchi model, kinetic constants () evaluated for PLX release were similar and did not show a particular trend in function of the initial solute load or the macromolecular composition. In this case, correlations between experimental and theoretical data were greater than FA (from 0.92–0.99), allowing one to suppose that release order tends to 0.5 in the Korsmeyer model for systems where correlation values resulted higher. Also for PLX, FT-IR chemical imaging correlation maps were constructed with the same technique described earlier, and the high chemical homogeneity of the loaded samples was verified (for example, in the correlation map obtained from a sample composed of copolymer 75/25 loaded with 10% in PLX is reported). The homogeneous distribution of PLX allowed deducing that the initial PLX loading did not exceed the solubility limit. Release kinetic constants obtained using the Korsmeyer equation () for polymeric systems loaded with Paclitaxel were found to be dependent on the chemical nature of the matrix. In particular, a different trend in function of the initial solute load was detected in systems composed of PMMA with respect to systems where BMA was present. Increasing the initial amount of PLX in the matrices, kinetic constants decreased in PMMA-based drug-eluting systems. Because the kinetic constant is proportional to the diffusion rate of the solute outside the matrix (considered in respect of the released amount when this rate tends to zero) a decrease in this parameter is symptomatic of a faster achievement of the plateau. On the contrary, in PBMA-based systems, kinetic constants increased with increasing the initial fraction loaded in the matrices and the same trend was found in the copolymer-based systems. In addition, considering the effect of the macromolecular composition and a fixed initial drug loading, kinetic constants decreased when the BMA fraction in the macromolecule increased. Release orders () were smaller than 0.5, as expected after the analysis carried out using the Higuchi model, which indicated a Fickian release.
PLX diffusion coefficients
In diffusion coefficients of PLX from polymeric matrices at early and late times are reported. As detected in the analysis of FA diffusion coefficients, values at early and late times were detected to be dependent on the initial amount of drug loaded in the polymeric platform: diffusion coefficients increased with increasing the initial solute load. In this case, values of Dearly were greater than Dlate in all cases, except in system with 0.5% of PLX initially loaded, but differences were smaller in comparison with the FA diffusion coefficients. This could mean that an initial burst effect is present in the delivery of PLX, as occurred in the release of FA, but this elution of solute was not massive and it could justify the previous observation related to the presence of an important sustained release during the testing period.
Hydrophilicity tests
Water contact angle tests
Water contact angle tests were performed to evaluate the hydrophilicity of the materials and the wettability of the surfaces. In the water contact angles obtained are reported. All five materials could be considered hydrophobic: water contact angles were greater than 90° in all cases, except for PMMA. Also, water contact angles were shown to be affected from the macromolecular composition of the materials. In particular, the water contact angle increased with increasing the amount of BMA in the macromolecule. This trend could be associated to an increase of the hydrophobicity of the materials increasing the amount of BMA. This trend is reasonable because the BMA unit differs from the MMA in the alkyl group bonded to the carboxylic oxygen. Alkyl group characteristic of MMA is composed of a single -CH3, alkyl group characteristic of BMA is composed of a -(CH2)3CH3. A greater hydrophobicity of the macromolecules increasing the BMA amount is reasonable. Assuming a surface tension value γ = 7.28·10−2 N/m (CitationPallas & Harrison, 1990) for the interface water/air at 20°C, work of adhesion and work of spreading were evaluated. Results are reported in . Both work of adhesion and work of spreading decreased with increasing the amount of BMA in the macromolecules. The work of adhesion is related to the energy necessary to separate liquid and solid phases. The decrease of the energy associated to this separation process, in function of the increase of the fraction of BMA in the materials, can be directly associated to a greater repulsion between water and polymeric substrate. Analogous consideration could be made considering the values of the Ws. Small values of work of spreading can be associated to a greater hydrophobicity of the surface.
Table 5. Work of adhesion and work of spreading evaluated from water contact angle measurements using equations (5) and (6).
Swelling tests
Using equation 7, the percentage swelling coefficient was calculated. Results are reported in . Data showed that the %SW was similar for PMMA and copolymers with a greater MMA amount in the macromolecular chain (copolymers 87.5/12.5 and 75/25). Copolymer P(MMA-co-BMA) 50/50 showed an intermediate swelling behavior between PMMA and PBMA, the amount of water retained and the %SW showed an exponential trend, similar to PBMA. PBMA showed the greatest %SW, and this parameter reached 200%.
Figure 8. Percentage swelling coefficients, evaluated using equation (7), for tested materials.
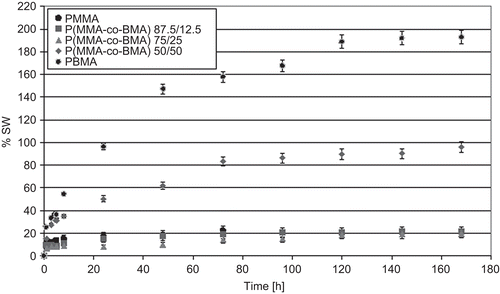
Swelling kinetics
From experimental data, swelling kinetic was studied using equation 8. Two kinetic parameters, ksw and nsw, were quantified. Kinetic parameters are summarized in . When materials were immersed in the water, the fluid diffused into the structures of the membranes and caused a volume phase transition related to an increase of the volume. In the first stage, the diffusion process could be attributed only to the migration of the molecules of water within the voids between the polymeric chains. When all the cavities between molecules were filled, the macromolecules underwent large motions, resulting in an increase of the sample volumes. This increase in volume rose until the thermodynamic equilibrium was reached. The thermodynamic equilibrium in the swelling process occurs when the osmotic pressure, cause of the penetration of the water in the cavities formed between macromolecules, and the elastic force of the chains become equal (CitationTomic et al., 2007). Because PMMA and PBMA present the same principal structure of the macromolecules, it was reasonable to hypothesize that the stiffness of the macromolecules did not significantly vary and when the swelling equilibrium was reached the elastic forces exerted by PMMA, PBMA, and their copolymers were similar. This could mean that the water uptake could be principally related to the cavities present between macromolecules. From this point of view, the greater steric hindrance of the alkyl group of the BMA could justify the presence of a greater vacuum degree between macromolecules and, consequently, a greater amount of water was necessary to fill all these voids. Data reported in , obtained by a fitting of experimental data, showed that the swelling kinetic constant was directly proportional to the amount of MMA present in the macromolecules. This could mean a faster achievement of the swelling equilibrium in materials where a greater amount of MMA is present. Therefore, this faster attainment of the swelling equilibrium was associable not to a greater hydrophobicity of the material that caused a smaller water uptake, but to a smaller presence of voids. In this perspective, results obtained from water contact angle tests reinforced this theory.
Figure 9. Swelling kinetic constants (s−n) (a), release orders (b), water diffusion coefficients at early (c) and late (d) times (mm2/s) for tested materials.
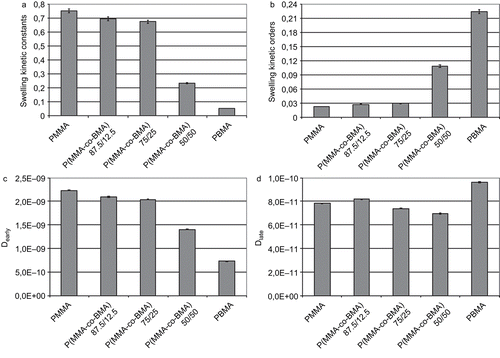
Further, water diffusion coefficients were evaluated. In particular, a difference before and after 48 h in the swelling behavior was highlighted. Dearly was then referred to the period from 0–48 h, while Dlate was evaluated with experimental data collected from 48–168 h.
Water diffusion coefficients are summarized in . In all cases, Dearly was greater than Dlate, which could be explained with the difference in osmotic pressure occurring at the beginning of the water absorption process. As the absorption occurred, the osmotic pressure decreased and then the absorption rate decreased.
Discussion and conclusion
In the present paper, the analysis of the mass transport properties of copolymers synthesized from methylmethacrylate and butylmethacrylate was carried out. In particular, the aim of the work was to study the effects of three different factors on the transport properties: (1) macromolecular composition of the polymeric platform, (2) chemical nature of the solutes, and (3) initial solute load.
Three different copolymers as drug delivery platform, two different solutes with diverse hydrophilicity degree, and four initial loads were considered.
Regarding the macromolecular composition, it was highlighted that this aspect influenced the elution of both molecules. As previously stated, increasing the hydrophobicity degree of the material the drug release increased. This could seem in contrast with results obtained from water contact angle tests that allowed concluding that the hydrophilicity of materials decreased with increasing the amount of BMA in the polymer. On the contrary, swelling tests showed that materials with a greater BMA amount in the macromolecular chain tended to swell more than those with a lower amount of BMA. This behavior is probably related to the different macromolecular organization that causes the formation of a greater number of micro/nano-voids in the materials when the steric hindrance of the alkyl group, characteristic of BMA, is present in greater amount. From this point of view, the increased elution of drug in materials with greater BMA amount could find a justification.
Considering the effect of the different hydrophilicity degree of the solute molecule, the amount of FA eluted is in all cases greater than the PLX amount at the end of the test. However, it is important to underline that the release of FA tended to reach a plateau value after 3 days while a similar behavior was not observed in the PLX delivery tests performed in the present work. From this observation it is possible to hypothesize that PLX could be released from tested matrices in a longer period than 24 days, even if the possibility of an irreversible entrapment of PLX in acrylic matrices cannot be excluded (Silvestri et al., Citation2009c).
Concerning the effect of the initial load of drugs, experimental results showed how this parameter strongly influenced the releasing profiles. In particular, the delivered fraction decreased with increasing the initial amount of the drug, while the opposite behavior was observed in the cumulative released amount. Results could help in the selection of the best polymer/drug system in order to maximize the released amount and minimize costs related to the waste of drugs. As expected, the cumulative amount of PLX eluted was smaller than FA for each system. This was due to the different rate of dissolution of drugs in water. Considering the cumulative released fraction, it can be interesting to highlight that a greater amount of BMA in the macromolecules particularly favored the elution of PLX in respect of FA, as verified in copolymer P(MMA-co-BMA) 50/50 and in homopolymer PBMA.
Analyzing the release kinetics, Higuchi model, based on the assumption that the release fraction is proportional to √t, did not seem to describe accurately the FA release mechanism: correlations between experimental and theoretical data were poor. This observation was stressed also by the evaluation of release orders using the Korsmeyer model, based on the best fitting parameters of a set of experimental data with a power law. The Korsmeyer model allowed evaluating release orders for the FA delivery kinetics much smaller than 0.5 in all cases. This means that the FA transport occurred in a Fick regime and it was not affected by other phenomena, like the macromolecule chain relaxation. The same results were reached in the analysis of PLX delivery kinetics.
Delivery kinetic constants can be compared with water absorption kinetic constants. In particular, from swelling tests, a faster reaching of the thermodynamic plateau of absorption of water in materials with a smaller BMA amount in the macromolecule was highlighted. Water absorption kinetic constants were greater than delivery kinetic constants of both drugs in systems composed of PMMA and copolymers 87.5/12.5 and 75/25 for all initial drug loads. A greater kinetic constant can be considered symptomatic of a faster reaching of the equilibrium. This could mean that for these materials the controlling parameter in the drug delivery process was represented by the diffusion of the solute outward to the polymeric system and not by the diffusion of water into the membrane. On the contrary, in systems composed of PBMA, the water absorption kinetic constant was smaller than the solute delivery kinetic constant, which could mean that the controlling parameter was the water absorption and not the diffusion of the solutes. The same comparison for results obtained for copolymer 50/50 needed to consider the initial drug load. In particular, smaller initial drug loads resulted in controlling the delivery process while for greater drug loads the controlling parameter was the water uptake rate. Also, from the comparison between swelling kinetic and release kinetic it was possible to deduce that systems showed a bi-linear trend, characterized by an ‘early time’ stage, where both water uptake and diffusion tended to increase with time, and a ‘late time’ stage, when water uptake and release kinetic both slowed down. The transition from early times to late times occurred after 3 days for all systems.
Transport properties characterization proposed in the present work showed how to control the delivery kinetic of a less hydrophobic and a more hydrophobic principle from polymeric matrices obtained from a copolymerization of two materials widely used in biomedical applications, polymethylmethacrylate and polybutylmethacrylate. The possibility to foreseeing a trend was verified.
As previously reported, both Paclitaxel and folic acid are used in cancer treatment. The possibility to control the delivery kinetic from a polymeric matrix renders these materials a promising pharmaceutical platform for a topic therapeutic drug administration, for example, in Drug Eluting Stents, where anti-neoplastic agents are generally loaded in a polymeric coating able to furnish a sustained release of the principle. From this point of view, in a possible clinical application as coatings for Drug Eluting Stents, it will be fundamental to consider also the biocompatibility of the materials. In the immediate future, the cytocompatibility and the hemocompatibility will be analyzed. However, at the end of the present study it is possible to deduce that it is possible to design specified drug delivery systems with desired characteristics using poly(methylmethacrylate-co-butylmethacrylate), folic acid, and Paclitaxel.
Acknowledgements
The authors want to acknowledge Dr Niccoletta Barbani (University of Pisa) for the realization of FT-IR chemical imaging maps; and Elena Bellotti, Ilaria Baldoli, and Simone Parrini (undergraduate students at University of Pisa) for the collaboration in part of experimental work.
Declaration of Interest
The authors report no conflicts of interest. The authors alone are responsible for the content and writing of the paper.
References
- Costa, P., Sousa Lobo, M.J. (2001). Influence of dissolution medium agitation on release profiles of dustained-release tablets. Drug Dev Ind Pharm. 27:811–7.
- Elstad, N.L., Fowers, K.D. (2009). OncoGel (ReGel/paclitaxel)—clinical applications for a novel paclitaxel delivery system. Adv Drug Del Rev. 61:785–94.
- Gao, L., Zhang, D., Chen, M. (2008). Drug nanocrystals for the formulation of poorly soluble drugs and its application as a potential drug delivery system. J Nanopart Res. 10:845–62.
- Higuchi, T. (1963). Mechanism of sustained-action medication. Theoretical analysis of rate of release of solid drugs dispersed in solid matrices. J Pharm Sci. 52:1145–9.
- Hu, F., Neoh, K.G., Kang, E.T. (2009). Synthesis of folic acid functionalized PLLA-b-PPEGMA nanoparticles for cancer cell targeting. Macromol Rapid Commun. 30:609–14.
- Janát-Amsbury, M.M., Gupta, K.M., Kablitz, C.D., Peterson, C.M. (2000). Drug delivery for in vitro fertilization: rationale, current strategies and challenges. Adv Drug Del Rev. 61:871–82.
- Korsmeyer, R.W., Gurny, R., Doelker, E.M., Buri, P., Peppas, N.A. (1983). Mechanism of solute release from porous hydrophilic polymers. Int J Pharm. 15:25–35.
- Liu, J., Lee, H., Allen, C. (2006). Formulations of drugs in block copolymer micelles: drug loading and release. Curr Pharm Des. 12:4685–701.
- Liu, J., Meisner, D., Kwong, E., Wu, X.Y., Johnston, M.R. (2007). A novel trans-lymphatic drug delivery system: Implantable gelatin sponge impregnated with PLGA-paclitaxel microspheres. Biomaterials. 28:3236–44.
- Mathew, A.E., Mejillano, M.R., Nath, J.P., Himes, R.H., Stella, V.J. (1992). Synthesis and evaluation of some water-soluble prodrugs and derivatives of taxol with antitumor activity. J Med Chem. 35:145–51.
- Mikhail, A.S., Allen, C. (2009). Block copolymer micelles for delivery of cancer therapy: transport at the whole body, tissue and cellular levels. J Contr Rel. 138:214–33.
- Min, T., Ye, H., Zhang, P., Liu, J., Zhang, C., Shen, W., Wang, W., Shen, L. (2008). Water-soluble poly(ethylene glycol) prodrug of pemetrexed: synthesis, characterization, and preliminary cytotoxicity. J Appl Polym Sci. 111:444–51.
- Nagarwal, R.C., Kant, S., Singh, P.N., Maiti, P., Pandit, J.K. (2009). Polymeric nanoparticulate system: a potential approach for ocular drug delivery. J Contr Rel. 136:2–13.
- Nishiyama, N., Kataoka, K. (2006). Current state, achievements, and future prospects of polymeric micelles as nanocarriers for drug and gene delivery. Pharmacol Ther. 112:630–48.
- Pallas, N.R., Harrison, Y. (1990). An automated drop shape apparatus and the surface tension of pure water. Coll Surf. 43:169–94.
- Ritger, P.L., Peppas, N.A. (1987a). A simple equation for description of solute release I. Fickian and non-fickian release from non-swellable devices in the form of slabs, spheres, cylinders or discs. J Contr Rel. 5:23–36.
- Ritger, P.L., Peppas, N.A. (1987b). A simple equation for description of solute release II. Fickian and anomalous release from swellable devices. J Contr Rel. 5:37–42.
- Silvestri, D., Cristallini, C., Gagliardi, M., Barbani, N., D’Acunto, M., Ciardelli, G., Giusti, P. (2009c). Acrylic copolymers as candidates for drug-eluting coating of vascular stents. J Biomater Appl. 24:353–83.
- Silvestri, D., Gagliardi, M., Barbani, N., Cristallini, C., Giusti, P. (2009a). Synthesis and characterization of copolymers of methylmethacrylate and 2-hydroxyethyl methacrylate for the aqueous solubilization of Paclitaxel. Drug Del. 16:116–24.
- Silvestri, D., Gagliardi, M., Cristallini, C., Barbani, N., Giusti, P. (2009b). Different composition poly(methyl methacrylate-co-butyl methacrylate) copolymers through seeded semi-batch emulsion polymerization. Polym Bull. 63:423–39.
- Sosnik, A., Chiappetta, A.D., Carcaboso, A.M. (2009). Drug delivery systems in HIV pharmacotherapy: what has been done and the challenges standing ahead. J Contr Rel. 138:2–15.
- Stevanovic, M., Radulovic, A., Jordovic, B., Uskokovic, D. (2008). Poly(DL-lactide-co-glycolide) nanospheres for the sustained release of folic acid. J Biomed Nanotechnol. 4:349–58.
- Taira, S., Hatanaka, T., Moritake, S., Kai, Y., Ichiyanagi, Y., Setou, M. (2007). Cellular recognition of functionalized with folic acid nanoparticles. e-J Surf Sci Nanotechnol. 5:23–8.
- Tomic, S.L., Micic, M.M., Filipovic, J.M., Suljovrujic, E.H. (2007). Swelling and drug release behavior of poly(2-hydroxyethyl methacrylate/itaconic acid) copolymeric hydrogels obtained by gamma irradiation. Radiat Phys Chem. 76:801–10.
- Torchilin, V.P. (2004). Targeted polymeric micelles for delivery of poorly soluble drugs. Cell Mol Life Sci. 61:2549–59.
- Van Zuylen, L., Verweij, J., Sparreboom, A. (2001). Role of formulation vehicles in taxane pharmacology. Invest New Drugs. 19:125–41.
- Wang, X., Zhang, X., Castellot, J., Herman, I., Iafrati, M., Kaplan, D.L. (2008). Controlled release from multilayer silk biomaterial coatings to modulate vascular cell responses. Biomaterials. 29:894–903.
- Zeng, Q., Sun, M. (2009). Poly(lactide-co-glycolide) nanoparticles as carriers for norcantharidin. Mat Sci Eng C. 29:708–13.