Abstract
Ocular drug delivery remains challenging because of the complex nature and structure of the eye. Conventional systems, such as eye drops and ointments, are inefficient, whereas systemic administration requires high doses resulting in significant toxicity. There is a need to develop novel drug delivery carriers capable of increasing ocular bioavailability and decreasing both local and systemic cytotoxicity. Nanotechnology is expected to revolutionize ocular drug delivery. Many nano-structured systems have been employed for ocular drug delivery and yielded some promising results. Solid lipid nanoparticles (SLNs) have been looked at as a potential drug carrier system since the 1990s. SLNs do not show biotoxicity as they are prepared from physiological lipids. SLNs are especially useful in ocular drug delivery as they can enhance the corneal absorption of drugs and improve the ocular bioavailability of both hydrophilic and lipophilic drugs. SLNs have another advantage of allowing autoclave sterilization, a necessary step towards formulation of ocular preparations. This review outlines in detail the various production, characterization, sterilization, and stabilization techniques for SLNs. In-vitro and in-vivo methods to study the drug release profile of SLNs have been explained. Special attention has been given to the nature of lipids and surfactants commonly used for SLN production. A summary of previous studies involving the use of SLNs in ocular drug delivery is provided, along with a critical evaluation of SLNs as a potential ocular delivery system.
Ocular drug delivery
Challenges and obstacles
Drugs have to encounter several obstacles before reaching their target site where they exert their pharmacological effect. The main obstacle is the ability to cross the tissue epithelium while maintaining stability. Novel drug delivery carriers such as solid lipid nanoparticles (SLNs) can help to alleviate these problems. SLNs are important because it was found that one of the criteria for a particle to enter ocular mucosa, apart from its lipophilicity, is that it should be of submicron size (CitationCalvo et al., 1994; CitationAlonso & Alonso, 2004). For an ocular drug delivery system to be successful it should have small particle size (less than 10 μm) (CitationZimmer & Kreuter, 1995) with a narrow size range, should be non-irritant, adequately bioavailable, be compatible with ocular tissue, and cause no blurred vision (CitationBourlais et al., 1998; CitationSahoo et al., 2008).
Ocular drug delivery is a challenge for pharmaceutical scientists because of the complex nature and structure of the eye. Barriers such as the epithelial, aqueous–vitreous, blood–aqueous barrier, and blood–retinal barrier limit the entry of drugs via different routes to the eye. Usually deep drug penetration into the posterior chamber is necessary to treat glaucoma or uveitis and fight viral infections proliferated within the eye (CitationSeal et al., 1998).
Most drugs are formulated as simple eye drops and ointments for ophthalmic use. Eye drops count for more than 90% of ocular preparations (CitationBourlais et al., 1998). Although eye drops are cost effective, patient compatible, and simple in formulation, a major fraction of the drug applied topically is washed away with tears or removed by other mechanisms. Due to this removal, several applications in a day are required to achieve therapeutic effect. Ocular defence mechanisms limit the drug residence time over the cornea and reduce its absorption. It was estimated that using a conventional ophthalmic dosage form only ∼ 5% of the drug enters the eye intact (CitationGaudana et al., 2009).
In many cases, posterior eye conditions are treated by intravenous or intravitreal administration of high doses of a drug, which has to promise a high therapeutic index as in the case of antibiotics (CitationUrtti, 2006). The patient’s exposure to high concentrations of drugs is risky because certain disease processes require chronic medication or a continuous supply of the drug over a period of time to achieve therapeutic objectives (CitationMoshfeghi & Peyman, 2005). Drugs administered orally, intravenously, or through extravascular junctions barely reach the retina and the posterior chamber, thus delivering very low concentrations to the sites of action (CitationMoshfeghi & Peyman, 2005). Systemic administration of drugs to treat eye conditions such as macular disease can only be optimized if the medicament is lipophilic, because the hydrophilic agents remain behind the blood–retina barrier unless it is interrupted (CitationMoshfeghi & Peyman, 2005). Periocular and intravitreal ocular administration routes have provided advantages over conventional eye drops and ointments but there are still many associated drawbacks. A drug delivered via these routes is cleared rapidly from the site of action and repeated administration of high doses is often necessary (CitationMoshfeghi & Peyman, 2005). Moreover, the intra-vitreal route of administration is considered invasive, which may cause endophthalmitis, cataracts, vitreous hemorrhages, and retinal detachment, especially if repeated exposure is necessary. Due to these reasons this technique is used less frequently unless the therapeutic outcomes are extraordinary (CitationYasukawa et al., 2001; CitationUrtti, 2006). Sustained drug delivery devices including Ocusert®, Vitraser®, and Retisert® offer several therapeutic improvements, but their use is somewhat limited due to a surgical procedure required to implant them. Therefore, it is increasingly desirable to develop novel delivery systems to achieve optimized treatment.
Barriers of the eye
Surface removal
Lachrymal secretions wash away topically applied drugs continuously and the excess of the lachrymal fluid flows down the nasolachrymal duct swiftly (CitationUrtti & Salminen, 1993; CitationUrtti, 2006). Due to the presence of an extensive network of capillaries in the conjunctival sac and the nasal cavity, most of the drugs applied topically are absorbed into the systemic circulation, thereby reducing the ocular bioavailability to 5–10% only (CitationUrtti & Salminen, 1993; CitationGaudana et al., 2009). Systemic absorption from the ocular surface can cause side-effects, especially if the patient has various medication needs. For instance, timolol and other intra-ocular pressure reducing agents can cause cardiac and vascular complications, especially if the patient is susceptible (CitationAttama et al., 2009). Other pre-corneal factors limiting ocular drug absorption are drainage of the instilled solution, tear production (induced lachrymation), drug metabolism, and normal tear turn-over (CitationMainardes et al., 2005). The human cul-de-sac can usually accommodate ∼ 30 μL of fluids, whereas the instilled volume from eye drops is ∼ 50 μL (CitationMainardes et al., 2005). Therefore, large volumes of the medicament are lost through spillage from the cul-de-sac. Nasolachrymal drainage further limits the ocular absorption. These limiting factors undermine therapeutic efficiency and reduce the pre-corneal half-life of drugs to ∼ 1–3 min (CitationZimmer & Kreuter, 1995).
Epithelial barrier
This is the main route through which topically administered drugs reach the aqueous humor. The cornea is a multilayered tissue consisting of the epithelium, the endothelium, and the stroma. The epithelial layer is lipophilic and consists of tight junctions that limit the entry of hydrophilic drugs and macromolecules into the cornea and the aqueous humor (CitationSeal et al., 1998; CitationMainardes et al., 2005). This barrier can be breached by an epithelial defect or by subconjunctival injection. In some eye conditions such as glaucoma and conjunctivitis, the corneal absorption increases significantly due to the morphological changes. This, along with the use of permeation enhancers and muco-adhesives, can be explored further to achieve better corneal penetrations. Beneath the epithelium is a hydrophilic layer called the stroma constituting a large portion of the cornea below which resides a single layer of flat epithelium-like cells called the endothelium. Endothelium is a potential barrier to both hydrophilic and lipophilic drugs and molecules, and therefore complicates the ocular barrier structure to a greater extent. Hence, the epithelium is the main barrier for hydrophilic drugs while the stroma and endothelium limit the entry of lipophilic drugs.
Blood–aqueous barrier
This barrier is situated in the anterior segment of the eye and is composed of endothelial cells in the uvea (CitationUrtti, 2006). It limits the entry of hydrophilic drugs from the systemic circulation into the aqueous humor. This barrier gets disrupted sometimes due to inflammation and results in an enhanced temporary drug permeation (CitationUrtti, 2006). Together with the blood–retinal barrier they make up the blood–ocular barrier. The epithelium of the iris and the cilliary bodies pump anionic drugs out from the aqueous humor to the systemic circulation (CitationSeal et al., 1998).
Blood–retinal barrier
Situated in the posterior chamber, this barrier limits the entry of drugs from the systemic circulation to the retina. It is composed of retinal pigment epithelium (RPE) and the tight walls of the retinal capillaries (CitationHornof et al., 2005; CitationUrtti, 2006). Although drugs can reach the choridal extravascular space easily through the leaky and extensive vasculature of the choroid, their retinal access is denied by RPE and retinal endothelia (CitationUrtti, 2006). The blood–retinal barrier, along with the blood–aqueous barrier, protects the eyes from the entry of xenobiotics and harmful substances (CitationUrtti, 2006). This physiological defence mechanism limits drug delivery to the retina and vitreous humor via the choroid through the systemic circulation.
As well as systemic and topical administration, intravitreal injections, local injections, lipophilic prodrugs, and ocular implants have all been used in retinal drug delivery (CitationDuvvuri et al., 2003). These methods have their own disadvantages and limitations, hence there is a need to develop novel drug carriers which are safe, convenient, and efficient in crossing potential ocular barriers.
Application of nanotechnology in ocular drug delivery and the concept of modified eye drops
Nanotechnology is changing the perception of drug administration using conventional dosage forms. Nanotechnology has the potential to revolutionize the way we develop new therapies, as well as optimize existing ones. In the pharmaceutical sciences, the term nanoparticle refers to a particulate drug delivery system where particle size is in the nanometre range (1–1000 nm). Nanoparticles are being investigated extensively in order to develop drug delivery systems capable of allowing penetration through physiological barriers. Nanoparticles are either in the form of matrix-dispersion (nanospheres) or a membrane-reservoir type (nanocapsules), where drugs can be dissolved, entrapped, encapsulated, and dispersed within the particles or adsorbed on the surface of these particles (CitationBourlais et al., 1998).
A wide range of chemical and physiological materials have been used to prepare SLNs including polymers, lipids, phospholipids, and metals. These multifunctional drug carriers are expected to accommodate large drug loads, help target them to the site of action, and promote sustained/controlled drug delivery while maintaining a minimum size of 30–300 nm (CitationDebbage, 2009). Submicron sized particles have a very high surface-to-volume ratio. Therefore, according to the Noyes Whitney and Kelvin equations, they have increased dissolution rates enabling them to enhance the absorption of poorly soluble drugs such as cyclosporine, paclitaxel, or amphotericin B (CitationWissing et al., 2004). On the contrary, there are a few disadvantages associated with nanoparticles, such as difficulty of production, stability during their storage, aggregation, and complexity of administration (CitationWissing et al., 2004). Another drawback is the faster release rates associated with nanoparticles when compared to microspheres (CitationJanoria et al., 2007).
Physicochemical properties such as particle size, surface net charge, shape, solubility, degree of ionization, and lipophilicity influence drug ocular absorption and determine the route of administration (CitationMainardes et al., 2005). These factors can be tailored using novel nanoparticulate drug delivery systems that enhance the ocular bioavailability of drugs.
In recent years, scientists have been interested in incorporating drugs and other therapeutics into nanoparticulate carriers, administered as modified eye drops which are cost effective and therapeutically efficient (CitationNagarwal et al., 2009). The modified eye drops provide better penetration, extended ocular surface residence time, minimized drainage owing to mucoadhesive properties, simple administration, and patient compatibility (CitationNagarwal et al., 2009). Moreover, colloidal and particulate drug delivery systems can also be utilized for sub-conjunctival, periocular, and intraocular injections (CitationJanoria et al., 2007).
Many nano-structured systems such as SLNs, niosomes, nanocapsules, nanospheres, dendrimers, nanosuspensions, liposomes, and nanoemulsions have been employed in ocular drug delivery. These have alleviated problems associated with poorly soluble drugs, increasing their bioavailability while decreasing their administered dose and toxicity (CitationWissing et al., 2004). For many serious eye conditions (e.g. chronic cytomegalovirus rhinitis (CMV)) a constant and prolonged controlled drug release is necessary to achieve therapeutic goals. Nanotechnology-based delivery systems including SLNs can ensure sustained and controlled release of drugs and medicaments avoiding frequent administrations associated with conventional delivery systems. Further, nanomedicines have several advantages, such as the possibility of formulation as modified eye drops which are easily self-administered by the patient, elimination of the need for repeated administration, and offering protection against metabolic enzymes present on the ocular surfaces by constructing a protective barrier (CitationKaur et al., 2004; CitationAraújo et al., 2009; CitationHironaka et al., 2009). Due to their submicron size range they have the added advantage of not impairing vision (CitationAraújo et al., 2009). Nanoparticles such as positively charged liposomes and SLNs are expected to increase corneal absorption of drugs by increasing drug residence time by ionic interactions. CitationCortesi et al. (2006) developed cationic liposomes to administer certain peptides with anti-herpetic activity to the eye (CitationCortesi et al., 2006). It is widely believed that nanoparticles owe their therapeutic efficiency to their bioadhesive nature (CitationBourlais et al., 1998; CitationShen et al., 2009).
Liposomes are membrane-like lipid bilayer vesicles surrounding aqueous compartments (CitationMeisner & Mezei, 1995). The common problems associated with liposomes are that they are in liquid form, which limits their pharmaceutical formulation feasibility. Moreover, most methods of sterilization are considered unsuitable for liposomes (CitationMeisner & Mezei, 1995). The heating involved in autoclaving can irreversibly damage their vesicular structure and filtration reduces the vehicle to an average of 200 nm which limits their application (CitationMeisner & Mezei, 1995).
CitationHironaka et al. (2009) designed a sub-micron sized liposomal drug delivery system capable of delivering hydrophilic drugs to the posterior segment of the eye (CitationHironaka et al., 2009). The liposomes were formulated in the form of modified eye drops and the authors hope that this system can replace currently used invasive delivery routes in the treatment of major ocular conditions such as age-related macular degeneration, diabetic macular edema, and endophthalmitis. Others have reported that acyclovir containing liposomes are more effective than simple eye drop preparations, due to better penetration and absorption through the cornea (CitationLaw et al., 2000).
Niosomes have been used to deliver drugs like gentamicin to the eye (CitationAbdelbary & El-gendy, 2008). Niosomes are formed from the self-assembling of non-ionic amphiphiles in aqueous medium which are able to accommodate hydrophilic and hydrophobic drugs (CitationCarafa et al., 1998; CitationUchegbu & Vyas, 1998; CitationAbdelbary & El-gendy, 2008). Niosomal ocular drug delivery concept aims to enhance periocular retention, corneal penetration, and provide controlled release in a similae manner to SLNs and liposomes. However, it is believed that SLNs have better stability, acceptable formulation feasibility, no toxicity, and superior release profile.
SLNs
Lipids have been used as an excipient or drug delivery vehicle for accommodating lipophilic drugs and elevating their poor physiological water solubility (CitationChen, 2008). In the case of hydrophilic drugs, water-in-oil emulsions and microemulsions were extensively investigated to solubilize them for potential ocular drug delivery. Although microemulsions have been known to scientists since 1928, the research for potential use in ocular delivery has only begun in the past decade (CitationVandamme, 2002). Despite their simple production, easy sterilization, and modest stability the use of microemulasion in ocular drug delivery is limited by the choice of ingredients possessing good ocular tolerability (CitationVandamme, 2002). Oil-in-water emulsions and microemulsions require a high surfactant concentration to ensure formulation stability. This limits their ocular drug delivery applicability as the surfactants are usually not well tolerated.
SLNs were first patented by CitationMüller and Lucks (1996). Since then they have attracted scientists as a widely used, stable, non-toxic, and reliable particulate drug delivery vehicle. SLNs are structured as a solid lipid core in nanometer ranges accommodating the drug stabilized by a layer of surfactants (CitationSawant & Dodiya, 2008). SLNs have several advantages over other colloidal carriers, such as the possibility of controlling drug release, drug targeting, long-term stability, good drug loading (whether hydrophilic or lipophilic), absence of biotoxicity due to the use of physiological lipids, possibility of sterilization by autoclaving, and easy large scale production (CitationMehnert & Mäder, 2001). In addition, due to their nano size range, SLNs can be an effective ocular drug delivery system by enhancing corneal absorption, improving ocular bioavailability, prolonging the ocular retention time, and providing a sustained drug release profile (CitationKaur et al., 2002). All types of drugs can be loaded into SLNs, the only disadvantage being the burst effect associated with hydrophilic drugs (CitationKaur et al., 2008). The burst effect is often associated with drug adsorption on the surface of the nanoparticles, which results in the release of a major fraction of the dose in a short period of time. SLNs control and stop the degradation process of sensitive lipophilic materials and drugs due to the fact that the mobility of the reactive agents is hindered in solid state when compared to liquid state (CitationHelgason et al., 2009). Also, the mechanism of drug loading and location of microphase surfaces can affect the position of the active substances. The presence of drugs on the surface of particles promotes their chemical degradation, whereas drug orientation towards the centre inhibits the degradation process (CitationHelgason et al., 2009). These types of physiochemical phenomena can be well controlled and can be used to design SLNs to achieve optimum results.
They offer other advantages such as biodegradability, safety, low cost, simple production, and, most importantly, free dispersibility in aqueous media, enabling them to be formulated as modified eye drops (CitationSawant & Dodiya, 2008).
SLNs are mainly built from triglycerides in a specific orientation consisting of a polar core with polar heads facing towards the aqueous phase (CitationWadhwa et al., 2009). Many different lipids (triglycerides, hard fat types, partial glycerides, steroids, and waxes) and all classes of emulsifiers (ionic and non-ionic) have been used to prepare SLNs (CitationMehnert & Mäder, 2001).
In comparison to an aqueous eye drop, an ocular SLN system can have extended residence time on the ocular surface and conjunctival sac (CitationGaudana et al., 2009) leading to sustained release of the drug revealed by in vivo studies (CitationCavalli et al., 2002). However, their efficiency for ocular drug delivery has not been studied extensively (CitationVelpandian & Velpandian, 2009) and further investigation is necessary.
Drug particles engulfed in SLNs should effectively cross the epithelium due to the SLNs lipophilic properties. Moreover, the epithelium is slightly negatively charged, hence cationic SLNs can increase the corneal residence time of the drug and increase its absorption levels.
Modifications of SLNs and emergence of nanostructred lipid carriers (NLCs) and lipid drug conjugates (LDCs)
Apart from their initial success, SLNs are associated with a few drawbacks. Limited drug loading capacity, possibility of drug expulsion during phase modifications, and high water content of SLNs aqueous dispersions (70–90%) have led to the introduction of NLCs (CitationMehnert & Mäder, 2001; CitationMüller et al., 2002; CitationSawant & Dodiya, 2008). NLCs are produced by controlled addition of a spatially incompatible liquid lipid to the solid lipid component in order to accommodate a larger quantity of the drug and achieve a better release profile (CitationMüller et al., 2002; CitationSawant & Dodiya, 2008). This can be achieved by increasing the space between the fatty acid chains of the glycerides, allowing more drugs to be accommodated, and formation of imperfect lipid crystals avoiding drug expulsion during storage (CitationMüller et al., 2002). Although NLCs contain up to 30% liquid lipids, the final product is in the solid state with no crystalline structure.
Imperfect NLCs
Those special systems in which a liquid lipid (e.g. glycerides) is added to the solid lipid can be regarded as imperfect NLCs. They induce a larger distance between the fatty acid chains of the main lipid core, which causes imperfections in the crystalline structure of the lipid where a very large amount of the drug can be incorporated (CitationSawant & Dodiya, 2008). For example, CitationJenning et al. (2000) incorporated a medium length chain triglyceride oil in a matrix of a solid long chain glyceride, achieving higher payloads and controlled release properties (CitationJenning et al., 2000).
Multiple type NLCs
In another mechanism, liquid lipids are mixed in excess with solid lipids, resulting in nanocompartments of liquid lipid formed within the solid core which is already dispersed in the aqueous medium (CitationMüller et al., 2002). The nanocompartments of liquid lipids are dispersed uniformly in the matrix of solid lipids, which are protected from degradation and potentiate prolonged release behavior of the system (CitationMüller et al., 2002). This multiple system is beneficial for drugs that have higher solubility in liquid oils (CitationSawant & Dodiya, 2008). Excess of liquid lipids used in such cases inhibits drug expulsion, especially if a high concentration of drug is used in the preparation step (CitationMüller et al., 2002). For example, NLCs of Compritol 888 ATO can be prepared with incorporation of incompatible liquid lipids such as α-tocopherol or Miglyol 812 (CitationJenning et al., 2000; CitationSouto et al., 2006). These NLCs present a better drug protection and entrapment efficiency when compared to normal SLNs. Moreover, the result of DSC and X-ray diffraction studies have revealed that NLCs are in a less crystalline state than SLNs of compritol (CitationJenning et al., 2000; CitationSouto et al., 2006).
Structureless NLCs
Another approach is to mix and melt together carefully selected liquid and solid lipids which upon cooling solidify but do not crystallize. These are regarded as structureless NLCs and can avoid drug expulsion caused by crystallization (CitationSawant & Dodiya, 2008).
Lipid drug conjugates (LDCs)
Alternatively, lipid drug conjugates are developed by converting a hydrophilic drug to a lipophilic drug conjugate or a prodrug by addition of an ester or amide group (CitationSawant & Dodiya, 2008). These are either used alone or combined with other solid lipids and are expected to enhance biological transport and targeting of hydrophilic drugs (CitationSawant & Dodiya, 2008).
Fabrication of SLNs
The preparation of SLNs is based on solidified emulsion technologies (CitationAlmeida & Souto, 2007). It is comparatively easy to incorporate lipophilic drugs into SLNs, however hydrophilic drugs, especially proteins and peptides, tend to partition in the aqueous phase during the preparation process (CitationAlmeida & Souto, 2007). This problem can be solved by using surfactants as emulsion stabilizers (CitationAlmeida & Souto, 2007).
SLNs can be prepared by several methods. CitationMehnert and Mäder (2001) classified these methods into four categories; namely high shear homogenization and ultrasound (CitationHou et al., 2003), high pressure (hot and cold homogenization) (Citationzur Mühlen et al., 1998; CitationMehnert & Mäder, 2001; CitationOlbrich et al., 2001; CitationRadomska-Soukharev, 2007; CitationHelgason et al., 2009), solvent emulsification/evaporation, and microemulsion-based SLNs preparation (CitationMehnert & Mäder, 2001; CitationHeydenreich et al., 2003; CitationVighi et al., 2007; CitationKuo & Chen, 2009). In addition to the previous methods, SLNs composed of glyceryl monostearate (GMS) can be successfully prepared by a solvent emulsification-diffusion technique (CitationTrotta et al., 2003) or by a double emulsion technique (CitationLv et al., 2009). More recently, CitationHu et al. (2002) used a novel solvent diffusion method in aqueous system to prepare SLNs with clobetasol propionate as the loading drug (CitationHu et al., 2002). CitationYun et al. (2009) presented a continuous method of SLNs preparation by liquid flow focusing and gas displacement method in microchannels (CitationYun et al., 2009), whereas CitationZhang et al. (2008) prepared SLNs in a microchannel system with cross shaped junctions (CitationZhang et al., 2008).
Here are summarized a few important and widely used methods for the production of SLNs:
High pressure homogenization
High pressure homogenization has been used extensively to produce SLNs and nanoemulsions for parenteral use (CitationMehnert & Mäder, 2001). High pressure homogenizers are widely available in pharmaceutical production plants and laboratories, and scaling up the method represents few problems. During the process of hot homogenization the dispersion is passed through a narrow gap by applying a high pressure (CitationMehnert & Mäder, 2001). Passage through a short distance combined with the applied pressure produces high shear stress, breaking down the accelerated particles to submicron size. Lipid concentrations between 5–10% are easily handled in this technique, although higher concentrations reflect no limitation (CitationMehnert & Mäder, 2001). A preparatory step is involved in both hot and cold homogenization where the drug has to be dissolved or dispersed in the lipid melt. This step eliminates the need to use an organic solvent to dissolve the lipids which might cause toxicity concerns if residues remain.
Hot homogenization
In hot homogenization the mixture of drug inside the lipid melt is first emulsified with a solution of proposed surfactant(s) using a high speed stirrer. The primary emulsion affects the quality of the final particles and should ideally produce particles in the micrometer range or lower (CitationMehnert & Mäder, 2001). The primary emulsion is then subjected to high pressure homogenization in temperatures above the melting point of the lipid. The homogenization can be repeated a few times to achieve the desired sized particles, taking into account that extra cycles can actually yield bigger sized particles due to increased kinetic energy causing agglomeration (CitationMehnert & Mäder, 2001). Finally, the hot oil-in-water emulsion is cooled to room temperature to produce a super-cooled melt to yield SLNs (CitationMehnert & Mäder, 2001). One of the drawbacks of this method is the elevated temperatures required to melt the lipid which might further increase while applying high shear stress. This can affect the chemical stability of thermo-labile drugs and therapeutics such as antibiotics, peptides, and proteins which are widely used in ophthalmic procedures. However, if the drug is thermo-stable, the high shear and temperature employed in this technique can help in producing smaller sized particles.
Cold homogenization
In cold homogenization excessive heating is avoided by solidifying the lipid-drug mixture in liquid nitrogen and grinding it to a powder with particle sizes below 100 nm before dispersing it in a cold aqueous solution containing the surfactant(s). However, the preparatory step which involves melting of the lipid and dispersing/dissolving the drug is still necessary. Therefore, in cold homogenization, a suspension is subjected to high shear homogenization rather than an emulsion in the case of hot homogenization (CitationMehnert & Mäder, 2001). Although cold homogenization can control the complex crystallization behavior of lipids during super-cooling and drug distribution through the aqueous phase during homogenization, it usually yields particles with higher average particle sizes (CitationMehnert & Mäder, 2001).
W/O/W double emulsion
Double w/o/w emulsion is a relatively new method which has been utilized in recent years to prepare nanoemulsions and SLNs (CitationLv et al., 2009). Heating is not involved during the process, which makes it suitable for thermo-labile substances. SLNs of good quality can be prepared by adjusting the sonication intensity. The only disadvantages associated with this method are the use of organic solvent and the possibility of the presence of metal impurities from the sonicator (CitationMehnert & Mäder, 2001).
The method involves dissolving a drug in an aqueous solvent (CitationLv et al., 2009). The aqueous solution is then emulsified in an oil phase containing the lipid(s) dissolved in an organic solvent to form a primary w/o emulsion. The primary w/o emulsion is then dispersed into an aqueous solution containing the surfactant. The w/o/w double emulsion system is mechanically agitated to allow complete evaporation of the organic solvent until SLNs are formed.
Solvent emulsification-evaporation
CitationSjöström and Bergenståhl (1992) developed a method for preparing lecithin stabilized nanoparticles containing cholestryl acetate. In this method the lipid component is dissolved in a water immiscible organic solvent and the drug is either dissolved or dispersed in the lipid solution. For preparation of cationic SLNs the main lipid core (e.g. Precirol® ATO 5) is dissolved in an organic solvent (e.g. dichloromethane) and the cationic lipid (e.g. DODAB) can be dispersed in the aqueous phase containing the surfactant (Citationdel Pozo-Rodríguez et al., 2008). This organic phase is then emulsified in an aqueous solution containing a biocompatible cosurfactant such as bile salts (e.g. sodium glycocholate and phosphatidylcholine). If used in combination, the ratio of different cosurfactants is a critical factor which determines final particle size. Emulsification can be achieved using a high speed stirrer Ultra-Turrax followed by high pressure homogenization. The system is mechanically stirred at room temperature until the solvent completely evaporates.
The quality of the primary emulsion directly affects the final SLN average size. The smaller the droplets in the primary emulsions, the smaller will be the final SLN (CitationSjöström & Bergenståhl, 1992). Hence, it is important to optimize all parameters which influence the emulsification process such as the lipid and emulsifier’s concentration and type. If optimized, this method can produce particles with an average size below 100 nm by precipitation into an o/w emulsion (CitationSjöström & Bergenståhl, 1992).
Solvent emulsification-diffusion
This method is based on the water miscibility property of certain organic solvents such as butyl lactate or benzyl alcohol as the oil phase (CitationHu et al., 2004; CitationTrotta et al., 2005). A primary oil-in-water emulsion is prepared containing the drug and the lipid phase solution in a water-miscible solvent. This emulsion is then transferred into water. The hypothesis is that upon introduction to an aqueous phase, the water-miscible solvent will diffuse. Hence, the lipophilic material dissolved in the solvent will solidify due to the diffusion of solvent from droplets to the continuous phase (CitationTrotta et al., 2005).
CitationTrotta et al. (2005) used this method to encapsulate insulin into glyceride monostearate solid lipid micro- and nanoparticles using isobutyric acid as a water-miscible solvent. The entrapment efficiency was significantly satisfactory (80%), however the burst effect which released 20% of the drug suggested the presence of insulin on the surface of particles rather than within. The method is reliable for the entrapment of lipophilic and hydrophilic drugs if optimized.
Oil-in-water microemulsion
Oil-in-water microemulsion technique is an easy and suitable method for the preparation of SLNs. It does not require organic solvents; however, a pre-heating step is required which may be disadvantageous to thermolabile substances. Briefly, the oil components are melted at ∼ 10°C above their melting point and the drug to be loaded inside SLNs is mixed with the lipid melt. The lipid melt is then dispersed and homogenized in a hot aqueous phase containing the surfactant heated at the same temperature of the lipid melt simultaneously. The hot o/w microemulsion is then cooled rapidly while maintaining the mechanical stirring until SLNs are formed. CitationVighi et al. (2007) used this method to prepare SLNs suitable for gene delivery.
The theory is that oil droplets are present in the hot o/w microemulsion. Upon sudden decrease of temperature, the nanoparticles are expected to crystallize rapidly, forming SLNs (CitationMarengo et al., 2000). CitationMarengo et al (2000) developed an apparatus for SLNs preparation which works by quenching of the warm o/w microemulsion into cold water. The warm o/w emulsion can be either cooled using an ice bath (CitationVighi et al., 2007) or it can be placed into cold water directly (CitationCavalli et al., 1996; CitationMarengo et al., 2000).
In another study, CitationCavalli et al. (1996) developed an instrument with the capacity to handle 1 ml of oil-in-water microemulsion at a time in which a glass syringe thermostated with a bronze jacket injects the hot emulsion into cold water. Factors such as the temperature and the delivery rate of the hot emulsion into cold water are very important in deciding final nanoparticle size and composition (CitationMarengo et al., 2000).
Preparation of SLNs in co-flowing microchannels
Preparation of SLNs using microchannels is under investigation so that critical procedures associated with earlier production methods such as high speeds, high pressures, high temperatures, and the use of toxic organic solvents might be avoided (CitationYun et al., 2009). Microchannels offer several advantages such as efficient mass transfer, stable flow field, and uniform concentration distribution, which results in continuous production of SLNs with a narrow size range (CitationYun et al., 2009).
The basic principle behind this method is solvent displacement. As described by CitationZhang et al. (2008), the apparatus consists of a co-flowing microchannel system with inner and outer capillaries. The lipid is dissolved in a water-miscible solvent which is injected into the inner capillary. The aqueous phase containing the surfactant is injected into the outer capillary simultaneously. Displacement of the solvent from the lipid phase to the aqueous phase causes local supersaturation and solidification of lipids resulting in the formation of SLNs. This instrument also employs a digital inversion microscope equipped with a video camera for capturing images of flow patterns in the microchannel (CitationZhang et al., 2008).
Particles with different size and morphology can be prepared by altering parameters such as the velocities of liquids flow to adjust focused flow patterns (CitationYun et al., 2009). Due to the small diameter of microchannels it is often blocked by SLNs hindering continuous production (CitationYun et al., 2009). Gas–liquid slug flow was used by CitationYun et al. (2009) to overcome microchannel blockage.
Post-preparation procedures
Purification and separation of SLNs
Purification is an important step in SLN preparation to avoid toxicity associated with extra surfactants. Therefore, methods like ultrafiltration (CitationTrotta et al., 2005), ultracentrifugation, and dialysis have been employed (CitationHeydenreich et al., 2003).
Sterilization
Sterilization is a required step for all ocular preparations. Ophthalmic products usually have a short shelf-life and they must be used within 1 month after opening due to contamination risks. In many cases a sterilization technique has to be carefully chosen to ensure formulation sterility without degradation and aggregation of the solid lipids to avoid toxicity and instability (CitationMehnert & Mäder, 2001). Commonly used techniques for sterilization are autoclaving, filtration, aseptic production, γ-radiation.
It is almost certain that free radicals are formed after γ-radiation of any sample due to the high energy of these rays (CitationMehnert & Mäder, 2001). Depending on the reactivity of the sample component the free radicals can be either returned to original molecules without chemical modification or cause chemical instability and degradation.
One of the advantages of SLNs over other colloidal systems is that they can be sterilized by autoclaving, a commonly used, straightforward, and reliable technique. A study on trilaurine SLNs loaded with azidothymidine palmitate showed that SLN dispersions maintained good stability after autoclaving at 121°C for 20 min (CitationHeiati et al., 1998). However, it should be noted that heating can induce physical instability and particle aggregation. An increase in the average particle size is usually observed after sterilization by heating. During autoclaving SLNs melt and recrystallize in a controlled manner. Therefore, certain structural features assigned to SLNs by controlling the production parameters will be lost by autoclaving, the severity of which is defined by the composition of the SLNs (CitationMüller et al., 2000).
It is important to know that heat- or radiation-induced degradation is not always associated with increased particles size. In some cases breakdown of lipids results in decreased particle size (CitationMehnert & Mäder, 2001). Degradation of lipids during sterilization is an important issue which can cause potential toxicity; however, this is often not given its due importance by most research articles.
Figure 5. Solid lipid production by liquid flow-focusing and gas displacement method (adopted from CitationYun et al., 2009).
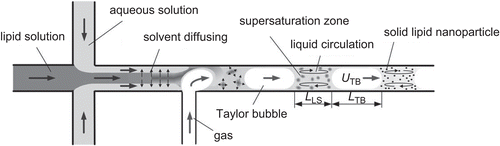
A filtration technique as well as aseptic preparation strategy can be also employed for SLNs sterilization, similar to the sterilization of parenteral emulsions and nutrition (CitationMüller et al., 2002).
Freeze drying of SLNs
Freeze-drying, also known as lyophilization, is a process that allows the stabilization of biomaterials so that they can be stored. Lyophilization involves the removal of water by employing the use of a process known as sublimation, wherein a solid is converted to the vapor state without first passing through the liquid phase. Lyophilization is utilized as a critical technique to convert the lipid dispersion to a solid state to extend the stability and to avoid particle aggregation (CitationMehnert & Mäder, 2001).
This is the chief method used for drying vaccines, pharmaceuticals, and other delicate, heat sensitive materials. With respect to SLNs, the freeze-drying process improves their long-term chemical and physical stability. This means that degradation reactions such as hydrolysis are prevented and initial particle size is preserved (CitationVighi et al., 2007). The freezing procedure also affects the crystal structure and properties of the lyophilizate (CitationVighi et al., 2007). Cooling may be done either rapidly or slowly. Rapid cooling can be performed by dipping the vial containing the preparation into liquid nitrogen or by adding the SLN dispersion dropwise to liquid nitrogen (CitationMehnert & Mäder, 2001). This results in the formation of small, heterogeneous crystals, whereas slow cooling causes large crystals to form as is done by placing the vials in a freeze drier having a shelf temperature of −25°C for 24 h (CitationMehnert & Mäder, 2001). Each type is associated with certain advantages and disadvantages. While rapid cooling decreases freezing out effects, it causes slower sublimation. Therefore, freeze-drying must be done in a sample-specific manner by optimizing the lyophilization process (CitationMehnert & Mäder, 2001).
The process of freeze-drying may have some unfavorable effects on the SLNs themselves, therefore it may be sometimes necessary to use certain protective materials known as cryoprotectors. These additives help to decrease particle aggregation and improve re-dispersion of the dry product (CitationVighi et al., 2007). Commonly used cryoprotective agents are sorbitol, mannose, trehalose, glucose, and polyvinylpyrrolidone (PVP). In a study by CitationZimmermann et al. (2000), the protective effect of several cryoprotectors was investigated. Trehalose was found to be the most effective, while PVP was found to be least effective (CitationZimmermann et al., 2000). As with the type of freezing process used, cryoprotectors need to be chosen specifically for a SLN based on the material it is made of. However, the concentrations of the cryoprotectants need to be optimized. As an example, a study by CitationSchwarz and Mehnert (1997) showed that Trehalose proved to be the most effective for Compritol-SLN and Dynasan-SLN when used at high concentrations. Although the solid state of the SLNs are chemically and physically more stable, attention must be given to the effects of the lyophilization process and the process of redispersion on the overall stability of the SLN once it is reconstituted. The negative effects of these processes can be lessened by employing the optimum freezing velocity and by selecting the best redispersion method, i.e. either sonication or manual shaking (CitationSchwarz & Mehnert, 1997).
Spray-drying of SLNs
Spray-drying is used as an alternative method to increase SLN stability (CitationFreitas & Müller, 1998). It involves the conversion of a solution or a suspension into a dry product. Although spray-drying is a widely used process in the pharmaceutical industry and is cheaper than lyophilization, it has rarely been used for SLN formulations (CitationMehnert & Mäder, 2001). CitationFreitas and Müller (1998) described a process for carrying out spray-drying of SLN wherein a product suitable for i.v. administration was obtained. They briefly summarized the steps into the following: (1) atomization of the feed into the spray, (2) spray–air contact, (3) drying of the spray, and (4) separation of the dried product from the drying gas. Several factors such as spray-drying parameters, the chemical nature of the lipid, and type of redispersion medium need to be taken into consideration in order to obtain a product which has optimum particle size and redispersion (CitationFreitas & Müller, 1998).
Problems associated with SLNs
Drug expulsion during storage
Crystalline behavior of lipids in the nanoscale is very complex. In SLNs, the solidification temperature is usually lower than that of bulk lipids (CitationBunjes & Koch, 2005) and less ordered lipid modifications are often formed (CitationFreitas & Müller, 1999; CitationMüller et al., 2002). However, drug expulsion during storage is one of the most common issues associated with the lipid crystal transformation to a more stable β-modification with more a perfect crystalline lattice, leaving behind less space for drug accommodation (CitationMüller et al., 2002).
Drug enriched shell and the burst release
The burst effect is a common problem associated with SLNs release profiles. The burst effect is explained by the room temperature phase separation effect (CitationMüller et al., 2002). It is proposed that when the hot o/w emulsion containing the drug is cooled to solidify, lipids crystallize to form SLNs with a drug-free core and a drug enriched shell (CitationMüller et al., 2002). This soft deformable drug enriched surface shell was detected in a study by atomic force microscopy (AFM) and small angle X-ray scattering (SAXs) measured as a 15–18 ηm thick layer (Citationzur Mühlen et al., 1998). To achieve a prolonged release profile of SLNs, lower temperatures and surfactant concentrations during production are advised as optimal conditions (CitationMehnert & Mäder, 2001; CitationMüller et al., 2002).
Biotoxicity
SLNs are made up of long or medium chain triglycerides usually mixed with mono- and di-glycerides and combined with individual or mixed ionic and/or non-ionic surfactants. They encompass a wide range of compositions while having complicated characters and functionality (CitationChen, 2008). These complexities are a challenge to pharmaceutical as well as regulatory scientists. The toxicity of lipids as excipients, as well as in formulations, needs further investigation (CitationChen, 2008).
The most important problem associated with most nanoparticulate drug delivery carriers is their lack of biodegradability, which makes them biotoxic (CitationPardeike et al., 2009). Nanoparticles can induce cell toxicity by either one or a combination of the following mechanisms: adherence to the cell membrane due to their nanometer scale or ionic charge, degradation, the release of cytotoxic degradation products, cellular internalization followed by degradation, and toxic effects inside the cells (CitationLherm et al., 1992; CitationPardeike et al., 2009). SLNs are usually made up of physiological lipids which are biodegradable with simple natural processes like enzyme digestion (CitationPardeike et al., 2009). Biodegradability of SLNs is one of the most important advantages in making them an outstanding drug delivery vehicle. These lipids and their degradation products are considered non-toxic to human body cells. However, due to their nano size range their biotoxicity is an important issue as the human body reacts very differently to nanoparticles as compared to larger particles of the same material (CitationPardeike et al., 2009). Certain tests (e.g. EPISKIN test and HET-CAM test) can be performed to evaluate topical and ocular toxicity and irritation caused by SLNs (CitationKüchler et al., 2008; CitationPardeike et al., 2009). In one study, CitationKüchler et al. (2008) found no ocular irritation potential associated with SLNs from the results of the HET-CAM test based on the EU classification system R38 (CitationKüchler et al., 2008). Considering the results obtained by many studies, SLNs are actually very well tolerated at the cellular level (CitationPardeike et al., 2009). All surfactants are potential irritants and are poorly tolerated, hence their biotoxicity remains an independent issue (CitationPouton & Porter, 2008). The choice of surfactant used is also an important consideration as water-insoluble surfactants penetrate and fluidize biological membranes, whereas water-soluble surfactants can solubilize the membrane components (CitationPouton & Porter, 2008). It should be noted that cationic surfactants are more toxic than anionic and non-ionic surfactants due to their ionic interaction with negatively charged cellular membranes (CitationPouton & Porter, 2008).
Characterization and quality assessment of SLNs
Many factors apart from the preparation methods influence the final quality of SLNs. The quality, composition, properties, and quantities of lipids and surfactants used can greatly influence the quality of the final product (CitationMehnert & Mäder, 2001).
Figure 6. Drug expulsion during storage (modified from CitationMüller et al., 2002).
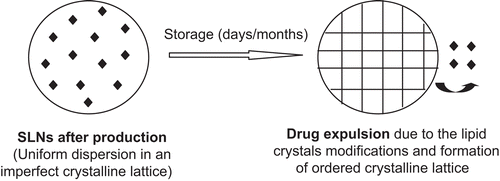
Formulation stability can be evaluated via particle size, zeta potential, and entrapment efficiency studies (CitationLv et al., 2009). Characterization of structure and quality is a very important step in determining stability and release kinetics of SLNs. A number of methods have been employed to measure SLN particle size and morphology, amongst them photon correlation spectroscopy (PCS) (CitationMehnert & Mäder, 2001; CitationOlbrich et al., 2001; CitationHu et al., 2002; CitationKuo & Chen, 2009; CitationLv et al., 2009), atomic force microscopy (AFM) (CitationOlbrich et al., 2001; CitationVighi et al., 2007), transmission electron microscopy (TEM) (CitationHou et al., 2003; CitationTrotta et al., 2003; CitationLv et al., 2009), and field-flow-fractionation (FFF) being the most popular. Zeta potential measurement is equally important in order to predict stability of colloidal dispersions. It is known that particles of the same charges tend to repel each other and hence avoid aggregation (CitationMehnert & Mäder, 2001). Measurement of crystallinity and lipid modification is important because these parameters influence drug incorporation, stability, and release rates. Methods like differential scanning calorimetry (DSC) and X-ray scattering are often used to examine the status of lipids. In addition, nuclear magnetic resonance (NMR), and electron spin resonance (ESR) methods are used to assess the presence of other colloidal structures such as microparticles in the formulation (CitationMehnert & Mäder, 2001).
Figure 7. Explanation of burst release associated with solid lipid nanoparticles release profile (adopted from CitationMüller et al., 2002).
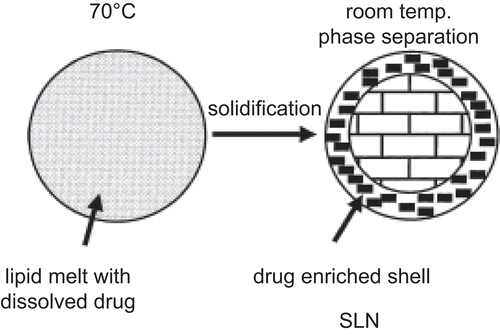
Particle characterization
Particle size
One of the most important characteristic features of SLNs is their nanometer scale. Particle size measurement can be regarded as the easiest way to estimate SLNs stability in dispersion over a period of time. Methods widely used to measure particle size are photon correlation microscopy (PCS) and laser diffraction (CitationRonald & Hong-Shian, 1983).
PCS does not measure the size directly but it measures the fluctuation of the intensity of the scattered light as a function of particle movement (CitationMüller et al., 2000). It is a sensitive method for the particles in the nanometre range, but is inefficient for particles larger than few micrometers. LD, on the other hand, can measure particles ranging in size from few nanometers to sub-millimeters, and the measurement is based on the dependency of the diffraction angle on the particle radius, where smaller particles cause more scattering at high angles compared to larger particles (CitationMüller et al., 2000).
The data obtained from PCS and LD are not always absolute as some SLN preparations contain particles of irregular shapes (not necessarily spherical) which, although they are in the nanometer range, are wrongly measured as microparticles. In such a case, other methods which provide direct information on particle shape such as TEM, SEM, and AFM are useful (CitationMüller et al., 2000).
Many factors such as the nature of the lipid matrix, concentration of lipids in the formulation, type of surfactant, surfactant concentration, and the viscosity of lipid and aqueous phase have an influential role in deciding SLN particle size.
Zeta potential
Electrokinetic potential of colloidal systems, referred to as zeta potential, determines the stability of colloidal dispersions. Zeta potential indicates the extent of particle–particle repulsion forces necessary to avoid agglomeration and aggregation. Higher zeta potential values, whether positive or negative, indicate higher dispersion stability. The zeta potential of an emulsion is determined by the chemical nature of the surfactant. Zeta potential of 30 mV is considered to be sufficient to ensure physical stability of emulsion (CitationLi et al., 2008a). Apart from the stability of the dispersion, surface charges are important in molecular mechanisms of drug absorption. In ocular drug delivery, this is explained by the fact that the corneal epithelial cells are negatively charged. Therefore, to increase drug residence time and ultimately its penetration, cationic SLNs which are proposed to modify corneal morphology through ionic interactions can be used.
Morphology of the particles
The following methods are used to determine particle size, size distribution, and morphology of SLNs.
Transmission electron microscopy (TEM)
TEM evaluates particle morphology by examining the electrons that are transmitted through the specimen (CitationSawant & Dodiya, 2008). An image is produced by interpreting the interaction of the electrons passed through the specimen, which is visualized by an imaging device or detected by a special sensor. Nanoparticles can be visualized using TEM after freeze fracturing and freeze substitution (CitationSawant & Dodiya, 2008).
Scanning electron microscopy (SEM)
This method offers excellent resolution and an easier sample preparation procedure for morphological examination of SLNs (CitationSawant & Dodiya, 2008). SEM measures electrons transmitted from the particle surfaces to evaluate their morphology (CitationSawant & Dodiya, 2008).
Atomic force microscopy (AFM)
AFM produces a three-dimensional image of nanoparticles (CitationSawant & Dodiya, 2008). It is a very sensitive device and spatial resolution of up to 0.01 nm can be achieved by measuring the force acting between the probing tip and the particle surface (CitationSawant & Dodiya, 2008).
Crystalline properties of lipids
Differential scanning calorimetry (DSC)
Differential scanning calorimetry can be described as a thermal analysis technique used in the investigation of melting, crystallization, solid-to-solid transition temperatures of lipids, and determination of the solid fat content of the excipient (CitationJannin et al., 2008). It enables the measurement of temperature change and heat flow which occurs when a material undergoes phase transition. Basically it involves the comparison of the heat required to raise the temperature of a sample and a reference, wherein both the sample and the reference are maintained at the same temperature throughout a given experiment (CitationColeman & Craig, 1996). Such data allows the quantitative and qualitative assessment of physical and chemical properties of the material by measuring changes in either enthalpy or heat capacity of a sample (CitationColeman & Craig, 1996). The thermal events that can be detected by this method may be endothermic phenomena such as melting or exothermic phenomena such as crystallization (CitationColeman & Craig, 1996).
DSC has various applications and is very successfully used in the pharmaceutical industry as a method of drug analysis and characterization of new delivery systems (CitationGill et al., 1993). With specific reference to SLNs, DSC allows the study of their melting and recrystallisation behavior. This is important as the crystallinity of a lipid matrix has an effect on the functional properties such as drug incorporation and release rates of the SLN derived from it (CitationGill et al., 1993).
The paper by CitationAttama et al. (2007) describes the characterization of SLNs prepared with a mixture of theobroma oil and goat fat as the main lipids, Phospholipon 90G as the heterolipid, and polysorbate 80 as the mobile surfactant. Methods of characterization used were time-resolved particle size analysis, zeta potential and osmotic pressure measurements, differential scanning calorimetry (DSC), transmission electron microscopy (TEM), and isothermal heat conduction microcalorimetry (IMC) (CitationAttama et al., 2007).
It is known that a lipid can crystallize in three basic polymorphic forms wherein the α-modification is the least stable form and β-modification the most stable form. If in storage the lipid forms a more stable and highly ordered crystalline matrix it can result in the drug that is incorporated to be expelled. Such a transition would correspond to a higher melting peak, whereas a lower melting peak would correspond to an unstable modification. In the study, it was shown that SLNs prepared with a mixture of two lipids had a lower enthalpy, compared to SLNs prepared with only one lipid. This revealed that SLNs prepared from a mixture of the two lipids did not result in the formation of a stable modification having highly ordered lipid matrices, and thus would be more suitable for use as nanostructures lipid carriers (CitationAttama & Müller-Goymann, 2007).
Wide angle X-ray diffraction (WAXD)
This is a well established method used to investigate the crystalline nature of the formulated SLNs (CitationAttama et al., 2007; Citation2008; CitationBunjes & Unruh, 2007). It is used to qualitatively determine the crystalline ingredients of a dispersion according to their individual diffraction patterns (CitationBunjes & Unruh, 2007). If a crystalline structure is identified, information about polymorphic modifications can be obtained subsequently (CitationBunjes & Unruh, 2007). For example CitationJenning et al. (2000) confirmed the presence of β´-modification of compritol using X-ray diffraction (CitationJenning et al., 2000). This is based on the fact that the X-rays reflected due to the crystalline lipids appear well above the amorphous background of non-crystalline lipids (CitationAttama & Müller-Goymann, 2007).
Drug encapsulation and loading efficiency
Determination of encapsulation efficiency is based on the separation of lipids from the aqueous phase of the dispersion achieved by either ultrafiltration, ultracentrifugation, gel filtration using a sephadex column, or dialysis (CitationSawant & Dodiya, 2008).
In ultrafiltration, the amount of drug loading can be calculated indirectly after centrifugation in a membrane concentrator (CitationAttama et al., 2008). Sometimes ultracentrifugation for a period of time is solely sufficient to separate SLNs from the aqueous phase (CitationKuo & Chen, 2009). The amount of drug present in the aqueous continuous phase can be determined by a sensitive method like HPLC or UV spectroscopy. The loading capacity can then be calculated considering the initial concentration of drug used. CitationAttama et al. (2008) repeated these measurements thrice at 2-week intervals to allow the complete crystallization of the lipids in order to determine if the crystalline lattice modifications cause drug expulsion (CitationAttama et al., 2008). The entrapment efficiency and drug loading can be calculated using equations (1) an (2) (CitationLv et al., 2009):
Ultrafilitration and microdialysis are known to be the most accurate method to measure entrapment efficiency of encapsulated systems (CitationLiu et al., 2009). Although ultracentrifugation is the fastest and easiest method to use, the results obtained are not reliable (CitationLiu et al., 2009).
CitationBasaran et al. (2009) used a different method to determine the drug contents in SLNs. They melted an accurately weighed amount of SLNs at ∼ 80°C in tightly closed glass tubes containing ethanol. Cooling of the obtained hot dispersion to −18°C in a deep freezer resulted in the precipitation of the solid matrix which left behind a transparent liquid mass containing the drug after ultracentrifugation. The amount of drug present was then determined.
Release studies
In vitro release studies
Drugs incorporated into SLNs are usually released by diffusion through the lipid matrix and/or biodegradation and surface erosion of the lipid matrix (CitationSawant & Dodiya, 2008). This should allow a sustained and controlled release of drug from the colloidal system. The extent of the drug incorporation within the SLNs can be revealed by its release profile. An immediate burst effect can release a major portion of the drug in a short period of time. This can be due to surface adsorption rather than encapsulation or dispersion. Many factors such as concentration of lipids, drug solubility inside the lipid core, drug interaction with the lipids, production temperatures, surfactant used, and particle size can influence and promote surface absorption (CitationSawant & Dodiya, 2008). The aqueous solubility of most drugs is enhanced at higher temperatures. This causes a change in the partition coefficient of the drug and its position in the SLNs. Therefore, higher production temperatures promote drug localization on the surface of SLNs, causing a burst effect (CitationSawant & Dodiya, 2008).
In vitro release studies using a modified Franz diffusion cell
In vitro release studies can be performed in a modified Franz diffusion cell (CitationFranz, 1975) over a period of time (CitationAttama et al., 2008). A diffusion barrier like siliconized Spectrapore® MWCO 6000–8000 can be used to mimic physiological conditions (CitationAttama et al., 2008). Alternatively, fresh cornea obtained from white male New Zealand rabbits can be used as the diffusion barrier mounted on a modified Franz-type cell (CitationLi et al., 2008b). Specific amounts of SLNs were placed on the donor compartment and apparatus openings were sealed to prevent evaporation (CitationAttama et al., 2008). A suitable buffer, such as phosphate buffer pH-7.4, is used as the acceptor medium which is magnetically stirred continuously. At specific time intervals, aliquots of samples containing the released drug are taken from the acceptor compartment and are quantified using a suitable method of determination such as HPLC or spectroscopy. The sink condition is usually maintained by replacing the volume of aliquots taken by similar volumes of the buffer to resemble constant clearance of drugs from their physiological site of action.
In vivo release studies and toxicological tests
Animal studies are usually necessary to investigate the ocular bioavailability and release profile of drugs incorporated into different drug delivery vehicles. One animal used extensively for ocular studies is the rabbit (CitationHornof et al., 2005), although larger animals are also employed in some studies (CitationBasaran et al., 2009). The rabbit’s eye has obvious morphological and physiological differences with the human eye. The most important difference is the infrequent blinking rates, which can potentially affect pre-ocular retention of the medicament (CitationHornof et al., 2005). Moreover, ethical and economical issues associated with the use of animals in clinical studies have further limited this approach. Alternatively, release studies can be performed on a cell culture developed to mimic the eye physiology and its barriers. Many different well defined cell culture models of the ocular barriers are available in which parameters and conditions can be easily tailored according to the needs of a particular study (CitationHornof et al., 2005). Ocular cell cultures avoid inter-species variability, and they can be used to study the release profile, mechanisms of cellular transport, metabolism, protein expression, and toxicity tests of SLNs and other ocular preparations (CitationHornof et al., 2005).
In vivo release
Bio-engineering of human cornea constructs (HCC) and diffusion studies
CitationAttama et al. (2008) studied in vivo drug release of diclofenac sodium from SLNs by preparing a human cornea construct using a method described in previous literature (CitationReichl et al., 2004; Citation2005).
The use of HCC can resolve the problems associated with the use of rabbit’s cornea such as differences in physiological structure, enzymes presents, transporters, efflux proteins, surface proteins, and mucins (CitationAttama et al., 2008).
The cornea of the eye is a multilayer barrier consisting of three layers, namely: the epithelium, the stroma, and the endothelium. This multilayered tissue can be engineered and created step-by-step in a Transwell cell culture insert using SV-40 immortalized human endothelial and epithelial cells and native fibroblasts (stromal cells) (CitationReichl et al., 2004). As a result, the human cornea construct prepared by this method will have a cellular structure resembling the real cornea with seven-to-nine layers of flattened epithelial cells, microvilli, and microplicae present (CitationReichl et al., 2004). The method is promising since the permeability characteristics of HCC also closely resemble the permeation behavior of excised porcine cornea (CitationReichl et al., 2004).
The HCC can be then mounted on a modified Franz diffusion cell and the amount of drug released to the acceptor medium can be obtained following a similar procedure as described before.
Periocular retention of SLNs
In a method described in the literature two portions of 25 µl of fluorescent labeled SLNs were inserted into the lower conjunctival sac of a white male New Zealand rabbit at 90 s intervals (CitationCavalli et al., 2002). A slit-lamp fitted with a blue filter was then used to examine the cornea. This test was performed on one eye of at least six rabbits, measuring the time course of fluorescence over the cornea (CitationGiunchedi et al., 1999; CitationCavalli et al., 2002).
Ocular tolerability and hydration levels
Irritation tendencies of SLNs preparations were tested on at least three rabbits by instilling 50 µl of SLNs in one eye while instilling a similar volume of physiological saline in the other eye as reference (CitationCavalli et al., 2002). The eyes were examined using a slit-lamp at 0.5, 1, 3, and 12 h intervals and the irritancy was measured based on a scoring scale described in the literature (CitationBottari et al., 1978; CitationCavalli et al., 2002). Alternatively, the frequency of rabbit blinking in 5 min after SLNs administration to the lower cul-de-sac was measured against the frequency of blinking that occurred with the reference physiological solution or a phosphate buffer of pH 7.4 as a measure of ocular irritation (CitationLi et al., 2008b).
Hydration levels of the cornea can be used as a measure of SLNs/NLCs ocular toxicity (CitationLi et al., 2008b). As described in the literature (CitationRonald & Hong-Shian, 1983) a healthy cornea maintains hydration levels between 76–80%; any value higher than 83% is associated with a certain degree of corneal injury (CitationLi et al., 2008b). Hydration levels can be determined by calculating the difference in weight of the excised cornea before and after drying (CitationLiu et al., 2005; CitationLi et al., 2008b).
Materials used in the preparation of SLNs
In general, the types of lipids, surfactants, and drugs used in the preparation of SLNs have their own influence on the final quality of the product. For instance, release of a lipophilic compound from a lipid carrier depends upon partition between the aqueous medium surrounding the carrier, surfactant, and the lipid exploited (CitationVyas et al., 2008). Many factors contribute to drug disposition in either the lipid or the surfactant phase which need thorough investigation and characterization.
Lipids
With respect to lipids, their crystalline modifications, structure, concentration, and hydrophobicity characteristics are important factors in SLNs formation (CitationVyas et al., 2008). Supercooling of SLNs is also an important feature to be ensured after melt homogenization, otherwise the final product would appear as a nanoemulsion rather than colloidally dispersed SLNs (CitationBunjes et al., 1996). Moreover, the solid matrix is expected to remain in the solid state at body temperatures so that controlled and sustained drug release properties could be achieved (CitationBunjes et al., 1996). It is well known that lipids which form a highly ordered crystalline lattice are unfavorable as drug expulsion is greater. Therefore it is desirable to employ lipids with complex structures containing fatty acid chains of different lengths which are able to accommodate the drug efficiently. However, it has to be noted that lipids with long fatty acid side chains usually form larger particles. The pharmaceutical industry encourages the scientists to use a combination of long chain and short chain fatty acids to achieve better quality, stability, and higher drug loading capacities. One important advantage of SLNs over other nano-carriers apart from their physiological acceptability is their stability and longer shelf life. CitationRadomska-Soukarev (2007) studied the chemical stability of several lipids after SLNs production over a period of 2 years and found that the lipids were sufficiently stable. It was observed that the initial preparation method does not affect the chemical stability of the lipids and that triglycerides produced much more stable SLN formulations than mono- and diglycerides (CitationRadomska-Soukharev, 2007). A comprehensive list of lipids used in the preparation of SLNs for various applications is available in the literature (CitationMehnert & Mäder, 2001).
Table 1. Lipids used in the production of SLNs for ocular drug and gene delivery.
Table 2. Emulsifiers used in the production of SLNs for ocular drug delivery.
Triglycerides
Triglycerides are either short (< 5 carbons), medium (6–12 carbons), or long chain (> 12 carbons) and they might be synthetically hydrogenated to reduce oxidative degeneration (CitationHauss, 2007). The longer chain triglycerides have higher melting points, are believed to be more stable, and expected to produce better SLNs (CitationRadomska-Soukharev, 2007). Many triglycerides such as tricarpin (CitationDomb, 1995), trilaurin (CitationDomb, 1995; CitationWestesen & Bunjes, 1995; CitationBunjes et al., 1996; CitationHeiati et al., 1997; Citation1998; CitationSchwarz & Mehnert, 1997; Citationzur Mühlen et al., 1998), trimyristin (CitationWestesen & Bunjes, 1995; CitationBunjes et al., 1996; CitationWestesen et al., 1997), tripalmitin (CitationWestesen & Bunjes, 1995; CitationBunjes et al., 1996; CitationWestesen & Siekmann, 1997), tristearin (CitationDomb, 1995; CitationWestesen & Bunjes, 1995; CitationBunjes et al., 1996), and hydrated coco-glucerides (CitationAlmeida et al., 1997) have been used in the preparation of SLNs (CitationMehnert & Mäder, 2001). These widely used triglycerides are considered as suitable solid lipid core materials for the production of particles because their melting point is well above body temperature to allow controlled drug release, but sufficiently low to assure solidification after melt homogenization (CitationBunjes et al., 1996). However, it has been shown that melt homogenization with tripalmitin, tristearin, and trimyristin produces a crystalline solid lipid matrix, whereas trilaurin dispersions remained in the form of an emulsion, even after exposure to refrigerated temperatures (CitationBunjes et al., 1996). This problem can be rectified by addition of longer chain triglycerides to speed up nucleation and crystallization of trilaurin or other resembling glycerides (CitationBunjes et al., 1996). During the course of production and storage glycerides may exist under various polymorphic forms such as hexagonal (α), orthorhombic (β´), and triclinic (β), which can be confirmed by X-ray diffraction (CitationJannin et al., 2008). Polymorphic changes depend on the thermal history of the lipid (CitationJannin et al., 2008). Such changes are important and need to be investigated in SLNs as the transition to a more stable crystalline form potentiates drug expulsion. It is suggested that the use of a mixture of triglycerides produces crystals with a poorly ordered matrix (CitationBunjes et al., 1996) which can accommodate more quantities of the drug while avoiding drug expulsion during storage. The properties of colloidally dispersed glycerides, especially the melting, crystallization, and the kinetics of polymorphic transitions, differ greatly from those of their bulk material (CitationBunjes et al., 1996). Therefore, the suitability of these lipids for SLNs formulation should not be evaluated from their bulk properties, and the dispersion properties should be carefully studied to optimize the production parameters which decide the quality of the final product.
Compritol®888 ATO
The solid lipid glycerol behenate (Compritol®888 ATO) is used very often to prepare SLNs. It is composed of a mixture of mono-, di-, and triacylglycerols with a very small amount of α-form which disappears under thermal stress owing to its thermodynamic instability (CitationSouto et al., 2006).
Compritol usually crystallizes in its β´-modification which is very sensible at elevated temperatures (CitationSouto et al., 2006). It can be useful to prepare SLNs with drugs which have solubility problems (e.g. Acyclovir) by using an optimized concentration of surfactants like poloxamer 188 (CitationSouto et al., 2006). It has been shown that Compritol has higher loading capacities compared to stearic acid, monostearin, and tristearin (CitationVyas et al., 2008) and reportedly can be used to avoid re-crystallinity problems associated with other solid lipid used (Citationzur Mühlen et al., 1998).
Gelucire 44/14
Gelucires are semi-solid lipids often used in the preparation of NLCs for ocular drug delivery (CitationLi et al., 2008b). Gelucires are saturated polyglycolized glycerides which consist of a mixture of mono-, di-, and tri-glycerides and di-fatty acid esters of polyethylene glycol (CitationLi et al., 2008b). It is reported in the literature that they can enhance transdermal absorption of certain drugs (CitationNilüfer et al., 2003). This finding has been applied to the corneal permeation issue and some promising results were obtained (CitationLi et al., 2008b).
Cationic lipids
Cationic lipids are often used alone (e.g. stearic acid) or in combination (e.g. octadecylamine and N-[1-(2, 3-Dioleoyloxy) propyl]-N, N, N-trimethylammonium chloride (DOTAP)) to induce a positive charge on SLNs. Cationic SLNs obtained using these cationic lipids have shown better corneal penetration and gene transfection properties (Citationdel Pozo-Rodríguez et al., 2008; CitationBasaran et al., 2009). The enhanced drug penetration through the cornea is probably due to the ionic interaction of cationic particles with negatively charged epithelial cells (CitationCortesi et al., 2006). These ionic interactions promote prolonged drug residence time inside the cornea and possibly induce corneal morphological changes resulting in enhanced drug penetration and absorption. The same ionic interactions are known to be responsible for binding of cationic SLNs used as non-viral gene delivery vectors to the targeted negatively charged cell surfaces promoting enhanced transfection and cellular trafficking (Citationdel Pozo-Rodríguez et al., 2008).
Emulsifiers
Several emulsifiers have been used to prepare SLNs including polysorbates (e.g. Tween-80), polyoxyls (e.g. Cremophor® EL), sodium lauryl sulfate, bile salts (e.g. cholic acid), poloxomer 188, Brij® 78, and saponins with HLB values ranging from 6–18 have been used quite often (CitationMehnert & Mäder, 2001; CitationHauss, 2007). Many of these agents are also used as corneal epithelium penetration enhancers (CitationKaur et al., 2002). Studies have shown that higher concentrations of emulsifiers produce smaller sized particles and low concentrations of emulsifiers produce relatively larger particles (CitationRadomska-Soukharev, 2007; CitationHelgason et al., 2009). During the emulsification process, creation of smaller sized particles produces a large increase in the surface area of the system which requires excessive amounts of surfactants to coat the newly formed surfaces in order to stop the uncovered surfaces from colliding and agglomerating (CitationVyas et al., 2008). Usually, a mixture of surfactants yields smaller sized particles and the system shows a lower polidispersity index and higher lipid stability (CitationRadomska-Soukharev, 2007). It has to be noted that the type and concentration of the surfactant can affect the chemical stability of physiological lipids used to prepare SLNs (CitationRadomska-Soukharev, 2007). In ocular drug delivery the use of cationic surfactants is favored in order to induce a positive charge to the formulated SLNs. This allows a longer residence time in the epithelium and better penetration to the posterior chamber of the eye. Stearylamine is used in the preparation of cationic SLNs for ocular (CitationLi et al., 2008b) and non-ocular drug delivery.
Figure 9. Compritol 888 ATO consists of mixture of monobehenate (a), dibehenate (b), and tribehenate (c) of glycerol (adopted from Brubach et al., 2007).
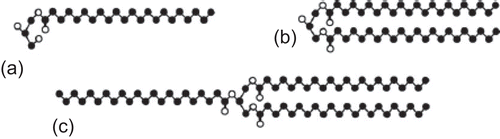
CitationHelgason et al. (2009) used Tween-20 to investigate the effect of surfactant concentration and surface coverage on the stability and crystalline structure of SLNs. They suggested that ‘simple addition of surfactant to the emulsion prior to lipid crystallization helps prevent gel formation’ (CitationHelgason et al., 2009). This means the surfactant molecules cover the newly formed surfaces, inhibiting their agglomeration and increasing the SLN emulsion stability. However, at low excess surfactant concentrations there may not be sufficient surfactant molecules to cover all the surfaces and hence optimal surface coverage may not be achieved (CitationHelgason et al., 2009). It was shown that by adding a higher concentration of a surfactant during the cooling process and crystallization of lipid, one can control the overall stability of the particles as well as particle shape changes avoiding agglomeration and transition from oval or spherical shapes to needle or disc shaped particles.
The drug itself
One of the advantages of SLNs over other particular carrier systems is that both hydrophilic and lipophilic drugs can be incorporated. SLNs can extend drug shelf-life and half-life by protecting them from the external environment. SLNs allow controlled/sustained release of drugs, thereby reducing the required administered dose to achieve therapeutic objectives. This in turn reduces the patient’s risk of exposure to toxic doses of drugs. SLNs are also believed to increase bioavailability of poorly penetrating drugs. Drugs can be incorporated into SLNs through deposition between fatty acid chains, between lipid layers, or in imperfections (CitationVyas et al., 2008).
Case studies
Tobramycin ocular delivery based on SLNs
CitationCavalli et al. (2002) proposed that SLNs can enhance ocular bioavailability of TOB, based on previous evidence that SLNs could enhance TOB duodenal absorption (CitationCavalli et al., 2000). A topical SLN-based eye drop was developed containing a TOB ion-pair complex with hexadecyl phosfate using a warm o/w microemulsion technique, purified by diaultrafiltration and freeze-dried for the determination of drug loading efficiency. The redispersed SLNs were sterilized with saturated steam for 15 min at 121°C. The TOB concentration inside the aqueous humor after topical administration was determined. A HPLC method was developed for quantitative measurements of TOB. Fluorescent marking was employed to determine the residence time of SLN in male New Zealand albino rabbits. The ocular tolerance and degree of irritation of SLNs containing TOB was examined by a previously developed method based on a blinking scoring scale in white New Zealand male rabbits (CitationBottari et al., 1978) and the SLN dispersions were tolerated with no evidence of irritation whatsoever. The average particle size of SLNs loaded with TOB was measured, which was found out to be ∼ 80 nm with a polydispersity index of only 0.12. Periocular retention studies showed the presence of fluorescent SLNs in the rabbit eye extended to over 1 h in comparison to a fluorescent solution which was washed away rapidly from the eye surface.
Ocular bioavailability of TOB incorporated into SLNs was significantly higher than that of the eye drop. TOB loaded SLNs showed Cmax of 36.30 ± 1.09, Tmax of 4 h, and AUC of 155.08 ± 4.31. Cmax was increased by 1.5-fold, Tmax by 8-fold, and AUC by 4-fold. The increased ocular bioavailability could be due to increased ocular residence time and entrapment of SLNs in the mucin layer covering the epithelium owing to their smaller particle size resulting in a sustained release of TOB, and possibly due to enhancement of ocular penetration by the surfactant, in this case epikuron 200, a soya phosphatidyl choline (CitationCavalli et al., 2002).
SLNs for ocular delivery of diclofenac sodium
CitationAttama et al. (2008) developed a SLNs system consisting of a homolipid from Capra hircus (goat fat) templated with phospholipon 90G® to enhance ocular bioavailability of diclofenac sodium. A high pressure hot homogenization technique was adapted to prepare SLNs. A primary homogenization step using Ultra-Turrax at 19,000 rpm for 5 min to form the primary emulsion was included in the preparation method.
The size and zeta potential values after 1 month of storage confirmed the stability of unloaded SLNs. However, increase in drug loading significantly increased average particle size and zeta potential values. Drug loading efficiency was as high as 90% for all SLNs except for those samples which did not contain phospholipids. This reflects that when they are incorporated into SLNs, phospholipids produce specific spaces where drug molecules can be localized. Quality analysis by DSC and WAXD revealed that the phospholipid containing SLNs have a low crystalline state optimizing drug loading efficiency.
In vitro drug release studies showed a burst effect associated with SLNs without phospholipids, whereas SLNs containing phospholipids presented a sustained release of diclofenac sodium which resulted in an increased ocular bioavailability. It is believed that, due to the specific structural effect on SLNs, phospholipids affect drug encapsulation as well as drug release profile. Permeation studies revealed that the drug efficiently crossed the cornea construct, probably due to high loading capacity and sustained release profile of diclofenac sodium. These findings introduced SLNs as excellent ocular drug delivery carriers that might need more research and attention to emerge as a method for delivery of poorly absorbed ocular drugs.
Surface modified SLNs for ocular delivery of timolol
When administered in the form of eye drops, timolol shows low ocular bioavailability and unsatisfactory therapeutic outcomes. Although eye drops are patient compatible, they have many disadvantages as described earlier. A large portion of timolol administered as an eye drop is absorbed into the systemic circulation (CitationAttama et al., 2009). The amount of drug absorbed is sufficient to cause serious respiratory and cardiac side-effects in susceptible patients and may induce adverse drug interactions with co-administered drugs like verapamil (CitationAttama et al., 2009). CitationAttama et al. (2009) developed SLNs based on a lipid matrix consisting of 30% phospholipid in theobroma oil. The SLNs were prepared by melt emulsification method using a high speed stirrer followed by high pressure homogenization. When compared to unmodified SLNs, surface modified SLNs using phospholipids showed better entrapment efficiency and sustained release profile without a burst effect. Presence of no burst effect is ideal as it reflects that the drug is engulfed inside the nanoparticles rather than adsorbed to their surface. This potentiates a sustained release of the drug over an extended period of time following hypothetical mechanisms such as diffusion, leaching, and gradual erosion (CitationAttama et al., 2009). They proved their claims by in vitro release studies using a human cornea construct and a siliconized spectrapore membrane (CitationAttama et al., 2009).
Ocular delivery of cyclosporine-A using SLNs
Cyclosporine-A is a strong immunosuppressant agent used in many ocular conditions. It is a lipophilic agent with limited aqueous solubility (CitationBasaran et al., 2009). It is available in certain topical ocular preparations, but the concentration obtained at the site of action is low and the patient should be tolerant to potential local irritation (CitationBasaran et al., 2009). Hence, CitationBasaran et al. (2009) proposed that cationic SLNs would be suitable to improve cyclosporine-A ocular bioavailability. SLNs were developed based on Dynasan® 116 and Compritol® 888 ATO using a hot homogenization technique with a high speed stirrer (CitationBasaran et al., 2009). Octadecylamine, a positively charged lipid, was added to the lipid melt containing the drug to produce cationic SLNs. The pH of nanoparticle suspension was adjusted to 7.2 using HCl and sterilized by autoclaving at 121°C for 20 min. After only 1 month of storage the pH value fell to ∼ 4. This indicates instability, and the author suggested that fatty acid degradation could be the reason. Adjustment of pH using HCl can be questioned as it is a potential eye irritant besides its degradative nature.
In another study, sterile Cyclosporine A loaded SLNs were prepared by homogenization and ultrasound method using Compritol 888 ATO as the base lipid which was emulsified with Poloxamer 188/Tween 80 mixture (CitationGokce et al., 2008). Preparation of SLNs with this method requires low concentration of surfactant which is an advantage in lowering ocular toxicity (CitationMehnert & Mäder, 2001, CitationGokce et al., 2008). It was observed that sterilization by autoclaving significantly increases the mean particles size of nanoparticles to the micrometer range. It was proposed that sterilizing at a lower temperature for a longer time (110°C for 30 min) and addition of Tween 80 can enhance physical stability of SLNs during autoclaving. Entrapment efficiency values as high as 95% were achieved and TEM results confirmed spherical shaped particles. The release of Cyclosporine A was enzymatic degradation-dependent, hence no release could be detected without the presence of lipase and co-lipase enzyme complex. The cytotoxicity of surfactants depends on whether they are bound to nanoparticle surface or are free in solution. The toxicity studies indicated that autoclaving may release Tween 80 from the nanoparticle surface which can cause cellular toxicity. Lastly, in vivo studies indicated that SLNs can enhance ocular drug delivery by means of a capitation/internalization mechanisms, although in vitro and ex vivo results were not correlating.
Further, they carried out an extensive ocular tolerance and in vivo study in rabbit eyes (CitationGokce et al., 2009). Sterile SLNs dispersion was prepared as previously stated and a specific dose was instilled in rabbit’s eye. At specific time intervals aqueous humor samples were collected and analyzed with HPLC to determine the drug content. Ocular toleration was studied by means of a modified Driaze test (CitationDraize et al., 1944; CitationGokce et al., 2009). The in vivo data showed that the concentration of drug in the aqueous humor reached the therapeutic levels after 3 h. The lag time can be due to internalization and uptake of SLNs into the corneal epithelium. As stated before, release of the drug is enzymatic degradation-dependent and phagocytosis is the possible uptake mechanism. Periocular retention time of the drug was increased to 40 min in comparison to the fluorescent solution which was retained for 10 min. The results of these studies suggest that SLNs can be tailored as a suitable ocular drug delivery device.
Incorporation of liquid lipid in SLNs for ocular drug delivery enhancement
It has been reported that NLCs can enhance ocular retention and corneal penetration of drugs (CitationCavalli et al., 2002; CitationLi et al., 2008b; CitationShen et al., 2009). In comparison to SLNs, NLCs have better drug entrapment efficiency, release profile, and have more imperfections in the crystal structures, which improve their stability and drug retention upon storage (CitationJenning et al., 2000; CitationHu et al., 2005; CitationShen et al., 2010).
In this study, the effect of liquid lipid incorporation on the physiochemical properties of NLCs was investigated using Cyclosporine A as a model drug (CitationShen et al., 2010). NLCs were prepared by melt-emulsification technique, where 2% Tween 80 was used as an emulsifier and 2% polyethylene glycol stearate (PEG-SA) as a surface modifier. The percentage concentration of Miglyol® 840 varied from 20–45%, whereas that of ATO 5 was constant (5%).
It was observed that increasing liquid lipid concentration results in formation of smaller sized particles. This may be due to the inhibition of solid lipid crystallization upon solidification from melted state in the presence of liquid lipids (CitationLin et al., 2007; CitationShen et al., 2010). This was proved by DSC studies where a decrease in melting point and crystallinity index was observed by an increase in the liquid lipid concentration. These observations indicate lower order lattice arrangements and crystal disturbance, respectively. The X-ray diffraction results were in agreement with the DSC observations and confirmed formation of a less ordered structure and pronounced crystal defects in NLCs having a higher concentration of liquid lipid.
The entrapment efficiency of NLCs having increased liquid lipid concentration did not change significantly, but the drug loading was enhanced significantly. This is due to the higher solubility of drug in the liquid lipid and the formation of an imperfect crystalline structure which provides more space to accommodate the drug (CitationJenning et al., 2000; CitationSouto et al., 2004; CitationShen et al., 2010).
The in vitro release studies showed a relatively fast release during the first 12 h followed by a sustained release profile. Further, the release rate was faster in NLCs with higher liquid lipid content. Faster drug diffusion through the liquid lipid phase and involvement of both diffusion and erosion mechanisms of drug release could be possible explanations (CitationSouto et al., 2004). The results of cellular uptake studies revealed that the liquid lipid with lower melting point had the strongest affinity to corneal cell membrane and was more easily taken by cells in comparison with the solid lipid. NLCs having higher concentration of the liquid lipid had a lower melting point and as a result better cellular uptake. Interestingly, NLCs containing 20% liquid lipid had higher cellular uptake in comparison with SLNs having the same entrapment efficiency. This indicates that liquid lipid aids cellular internalization of nanoparticles. Further, the in vivo studies indicated that NLCs prolonged the ocular surface retention and corneal penetration of the drug and that NLCs were non-toxic and exhibited good in vivo tolerance.
Ocular delivery of ibuprofen based on NLCs
In this study, CitationLi et al. (2008b) focused on the preparation of a controlled release NLC ocular drug delivery system using ibuprofen as a highly lipophilic model drug. They prepared four different formulations containing Compritol® 888 ATO as the main lipid core in combination with Gelucire® 44/14, Miglyol® 812, a liquid lipid, stearylamine as charge inducing agent, Transcutol® P as corneal permeability enhancer, and Cremphor® EL as the surfactant. NLCs containing ibuprofen were prepared by the melted-ultrasonic method which is an appropriate method due to aqueous insolubility of ibuprofen. Entrapment efficiency was determined by a gel permeation chromatography method using a Sephadex G-50 column. Entrapment efficiency of more than 80% was obtained for all four formulations and the particle size ranged from 80–160 nm. The cationic NLC formulation containing stearylamine was more stable and had a longer pre-ocular retention time due to its cationic surface charge. Corneal permeation studies revealed significant enhancement compared with the instillation of a simple eye drop. Formulations containing Gelucire® 44/14 and Transcutol® P had significantly enhanced corneal penetration. Glucires can reportedly enhance cornel penetration, mainly due to their lipophilic properties, whereas Transcutol® P enhances permeation by a mechanism involving structural changes in the epithelium as a result of micelle formation (CitationLi et al., 2008b).
SLNs as vectors for retinal gene therapy
Gene therapy can be a promising treatment for ocular disorders, mainly due to the well-defined anatomy of the eye, its accessibility, its transparency, and immunoprivilege (Citationdel Pozo-Rodríguez et al., 2008). Non-viral vectors have certain advantages over viral vectors due to their capability of incorporating DNA of any size and the fact that they are associated with lower risks of oncogenicity or immunogenicity (Citationdel Pozo-Rodríguez et al., 2008). For a vector to be efficient, it has to be able to bind to the cell surface. This is achieved by electrostatic interaction between positively charged vectors and negatively charged ocular epithelium which makes cationic SLNs a successful gene delivery vector (Citationdel Pozo-Rodríguez et al., 2008). The cellular intake can be enhanced due to the lipophilic nature of SLNs, increasing transfection capacity.
CitationDel Pozo-Rodríguez et al. (2008) prepared cationic SLNs for retinal gene delivery by a solvent emulsification/evaporation method described previously. Briefly, the lipid core material precirol® ATO 5 was dissolved in dichloromethane which was emulsified by a probe-type sonicator with the aqueous phase containing the cationic lipid DODAB and the surfactant tween 80. The transfection capacity of SLNs was evaluated in the human retinal pigment epithelial established cell line (ARPE-19) which was revealed to be low (∼ 2.5%). Intercellular trafficking studies suggested that changes in the structure of SLNs and some physiological differences in the cell line might be responsible for delay in cellular uptake. The findings of this study suggest that knowledge of intercellular trafficking is essential to develop non-viral vectors.
Future trends
SLNs have the potential to offer a major contribution to the search for better ocular drug delivery systems. Recently, the FDA and EMEA have taken promising steps in order to lead academic research into more industrial and commercial aspects (CitationBoisseau et al., 2005). What is required to end the long wait for a marketed ocular preparation based on colloidal carriers is more contribution from the scientists, and interest and willingness of the pharmaceutical industry to combine and refine the academic findings into commercially successful products.
Declaration of interest
The authors report no conflicts of interest. The authors alone are responsible for the content and writing of the paper.
References
- Abdelbary, G., El-Gendy, N. (2008). Niosome-encapsulated gentamicin for ophthalmic controlled delivery. AAPS PharmSciTech. 9:740–7.
- Almeida, A.J., Runge, S., Müller, R.H. (1997). Peptide-loaded solid lipid nanoparticles (SLN): influence of production parameters. Int J Pharm. 149:255–65.
- Almeida, A.J., Souto, E. (2007). Solid lipid nanoparticles as a drug delivery system for peptides and proteins. Adv Drug Deliv Rev. 59:478–90.
- Alonso, M.J., Alonso, M.J. (2004). Nanomedicines for overcoming biological barriers. Biomed Pharmacother. 58:168–72.
- Araújo, J., Gonzalez, E., Egea, M.A., Garcia, M.L., Souto, E.B. (2009). Nanomedicines for ocular NSAIDs: safety on drug delivery. Nanomedicine: NBM, 5:394–401.
- Attama, A.A., Müller-Goymann, C.C. (2007). Investigation of surface-modified solid lipid nanocontainers formulated with a heterolipid-templated homolipid. Int J Pharm. 334:179–89.
- Attama, A.A., Reichl, S., Müller-Goymann, C.C. (2008). Diclofenac sodium delivery to the eye: in vitro evaluation of novel solid lipid nanoparticle formulation using human cornea construct. Int J Pharm. 355:307–13.
- Attama, A.A., Reichl, S., Muller-Goymann, C.C. (2009). Sustained release and permeation of timolol from surface-modified solid lipid nanoparticles through bioengineered human cornea. Curr Eye Res. 34:698–705.
- Attama, A.A., Schicke, B.C., Paepenmüller, T., Müller-Goymann, C.C. (2007). Solid lipid nanodispersions containing mixed lipid core and a polar heterolipid: characterization. Eur J Pharm Biopharm. 67:48–57.
- Basaran, Ebru., Demirel, M., Sirmagul, B. and Yazan, Y. (2009). Cyclosporine-A incorporated cationic solid lipid nanoparticles for ocular drug delivery. J Microencapsul. 27:1–11.
- Boisseau, P., Kiparissides, C., Pavesio, A., Saxl, O. (2005). European technology platform on nanoMedicine—nanotechnology for health, vision paper and basis for a strategic research agenda for nanomedicine. European Commission: Office for Official Publications of the European Communities (Luxembourg), 39 pp. ISBN 92-79-02203-2 (keine IP).
- Bottari, F., Giannaccini, B., Cristofori, B., Saettone, M.F., Tellini, N. (1978). Semisolid ophthalmic vehicles. I—A study of eye irritation in albino rabbits of a series of gel-type aqueous bases. Farmaco—Edizione Pratica. 33:434–46.
- Bourlais, C.L., Acar, L., Zia, H., Sado, P.A., Needham, T., Leverge, R. (1998). Ophthalmic drug delivery systems—recent advances. Prog Retin Eye Res. 17:33–58.
- Bunjes, H., Koch, M.H.J. (2005). Saturated phospholipids promote crystallization but slow down polymorphic transitions in triglyceride nanoparticles. J Contr Rel. 107:229–43.
- Bunjes, H., Unruh, T. (2007). Characterization of lipid nanoparticles by differential scanning calorimetry, X-ray and neutron scattering. Adv Drug Deliv Rev. 59:379–402.
- Bunjes, H., Westesen, K., Koch, M.H.J. (1996). Crystallization tendency and polymorphic transitions in triglyceride nanoparticles. Int J Pharm. 129:159–73.
- Calvo, P., Thomas, C., Alonso, M.J., Vila Jato, J.L., Robinson, J. (1994). Study of the mechanism of interaction of poly-E-caprolactone with the cornea by confocal laser scanning microscopy. Int J Pharm. 103:283–91.
- Carafa, M., Santucci, E., Alhaique, F., Coviello, T., Murtas, E., Riccieri, F.M., Lucania, G., Torrisi, M.R. (1998). Preparation and properties of new unilamellar non-ionic/ionic surfactant vesicles. Int J Pharm. 160:51–9.
- Cavalli, R., Gasco, M.R., Chetoni, P., Burgalassi, S., Saettone, M.F. (2002). Solid lipid nanoparticles (SLN) as ocular delivery system for tobramycin. Int J Pharm. 238:241–5.
- Cavalli, R., Zara, G.P., Caputo, O., Bargoni, A., Fundarò, A., Gasco, M.R. (2000). Transmucosal transport of tobramycin incorporated in SLN after duodenal administration to rats. Part I—a pharmacokinetic study. Pharmacol Res. 42:541–5.
- Cavalli, R., Marengo, E., Rodriguez, L., Gascom, R. (1996). Effects of some experimental factors on the production process of solid lipid nanoparticles. Eur J Pharm Biopharm. 43:110–5.
- Chen, M.-L. (2008). Lipid excipients and delivery systems for pharmaceutical development: a regulatory perspective. Adv Drug Deliv Rev. 60:768–77.
- Coleman, N.J., Craig, D.Q.M. (1996). Modulated temperature differential scanning calorimetry: a novel approach to pharmaceutical thermal analysis. Int J Pharm. 135:13–29.
- Cortesi, R., Argnani, R., Esposito, E., Dalpiaz, A., Scatturin, A., Bortolotti, F., Lufino, M., Guerrini, R., Cavicchioni, G., Incorvaia, C., Menegatti, E., Manservigi, R. (2006). Cationic liposomes as potential carriers for ocular administration of peptides with anti-herpetic activity. Int J Pharm. 317:90–100.
- Debbage, P. (2009). Targeted drugs and nanomedicine: present and future. Curr Pharm Des. 15:153–72.
- Del Pozo-Rodríguez, A., Delgado, D., Solinís, M.A., Gascón, A.R., Pedraz, J.L. (2008). Solid lipid nanoparticles for retinal gene therapy: transfection and intracellular trafficking in RPE cells. Int J Pharm. 360:177–83.
- Domb, A.J. (1995). Long acting injectable oxytetracycline-liposphere formulations. Int J Pharm. 124:271–8.
- Draize, J.H., Woodard, G., Calvery, H.O. (1944). Methods for the study of irritation and toxicity of substances applied topically to the skin and mucous membranes. J Pharmacol Exp Therapeut. 82:377–90.
- Duvvuri, S., Majumdar, S., Mitra, A.K. (2003). Drug delivery to the retina: challenges and opportunities. Expert Opin Biol Ther. 3:45–56.
- Franz, T.J. (1975). Percutaneous absorption on the relevance of in vitro data. J Investig Dermatol. 64:190–5.
- Freitas, C., Müller, R.H. (1998). Spray-drying of solid lipid nanoparticles (SLN™). Eur J Pharm Biopharm. 46:145–51.
- Freitas, C., Müller, R.H. (1999). Correlation between long-term stability of solid lipid nanoparticles (SLN™) and crystallinity of the lipid phase. Eur J Pharm Biopharm. 47:125–32.
- Gaudana, R., Jwala, J., Boddu, S.H., Mitra, A.K., Gaudana, R., Boddu, S.H.S., Mitra, A.K. (2009). Recent perspectives in ocular drug delivery. Pharm Res. 26:1197–216.
- Gill, P.S., Sauerbrunn, S.R., Reading, M. (1993). Modulated differential scanning calorimetry. J Therm Anal Calorim. 40:931–9.
- Giunchedi, P., Conte, U., Chetoni, P., Saettone, M.F. (1999). Pectin microspheres as ophthalmic carriers for piroxicam: evaluation in vitro and in vivo in albino rabbits. Eur J Pharm Biopharm. 9:1–7.
- Gokce, E.H., Sandri, G., Bonferoni, M.C., Rossi, S., Ferrari, F., Guneri, T., Caramella, C. (2008). Cyclosporine A loaded SLNs: evaluation of cellular uptake and corneal cytotoxicity. Int J Pharm. 364:76–86.
- Gokce, E.H., Sandri, G., Egrilmez, S., Bonferoni, M.C., Guneri, T., Caramella, C. (2009). Cyclosporine A-loaded solid lipid nanoparticles: ocular tolerance and in vivo drug release in rabbit eyes. Curr Eye Res. 34:996–1003.
- Hauss, D.J. (2007). Oral lipid-based formulations. Adv Drug Deliv Rev. 59:667–76.
- Heiati, H., Tawashi, R., Phillips, N.C. (1998). Drug retention and stability of solid lipid nanoparticles containing azidothymidine palmitate after autoclaving, storage and lyophilization. J Microencapsul. 15:173–84.
- Heiati, H., Tawashi, R., Shivers, R.R., Phillips, N.C. (1997). Solid lipid nanoparticles as drug carriers. I. Incorporation and retention of the lipophilic prodrug 3'-azido-3'-deoxythymidine palmitate. Int J Pharm. 146:123–31.
- Helgason, T., Awad, T.S., Kristbergsson, K., McClements, D.J., Weiss, J. (2009). Effect of surfactant surface coverage on formation of solid lipid nanoparticles (SLN). J Colloid Interface Sci. 334:75–81.
- Heydenreich, A.V., Westmeier, R., Pedersen, N., Poulsen, H.S., Kristensen, H.G. (2003). Preparation and purification of cationic solid lipid nanospheres—effects on particle size, physical stability and cell toxicity. Int J Pharm. 254:83–7.
- Hironaka, K., Inokuchi, Y., Tozuka, Y., Shimazawa, M., Hara, H., Takeuchi, H. (2009). Design and evaluation of a liposomal delivery system targeting the posterior segment of the eye. J Contr Rel. 136:247–53.
- Hornof, M., Toropainen, E., Urtti, A. (2005). Cell culture models of the ocular barriers. Eur J Pharm Biopharm. 60:207–25.
- Hou, D., Xie, C., Huang, K., Zhu, C. (2003). The production and characteristics of solid lipid nanoparticles (SLNs). Biomaterials. 24:1781–5.
- Hu, F.Q., Hong, Y., Yuan, H. (2004). Preparation and characterization of solid lipid nanoparticles containing peptide. Int J Pharm. 273:29–35.
- Hu, F.-Q., Jiang, S.-P., Du, Y.-Z., Yuan, H., Ye, Y.-Q., Zeng, S. (2005). Preparation and characterization of stearic acid nanostructured lipid carriers by solvent diffusion method in an aqueous system. Colloids Surf B Biointerfaces. 45:167–73.
- Hu, F.Q., Yuan, H., Zhang, H.H., Fang, M. (2002). Preparation of solid lipid nanoparticles with clobetasol propionate by a novel solvent diffusion method in aqueous system and physicochemical characterization. Int J Pharm. 239:121–8.
- Jannin, V., Musakhanian, J., Marchaud, D. (2008). Approaches for the development of solid and semi-solid lipid-based formulations. Adv Drug Deliv Rev. 60:734–46.
- Janoria, K.G., Hariharan, S., Dasari, C.R., Mitra, A.K. (2007). Recent patents and advances in ophthalmic drug delivery. Recent Patents Drug Deliv Formul. 1:161–70.
- Jenning, V., Mäder, K., Gohla, S.H. (2000). Solid lipid nanoparticles (SLN(TM)) based on binary mixtures of liquid and solid lipids: a 1H-NMR study. Int J Pharm. 205:15–21.
- Kaur, I.P., Garg, A., Singla, A.K., Aggarwal, D. (2004). Vesicular systems in ocular drug delivery: an overview. Int J Pharm. 269:1–14.
- Kaur, I.P., Kanwar, M., Kaur, I.P., Kanwar, M. (2002). Ocular preparations: the formulation approach. Drug Dev Ind Pharm. 28:473–93.
- Kaur, I.P., Rana, C., Singh, H. (2008). Development of effective ocular preparations of antifungal agents. J Ocul Pharmacol Therapeut. 24:481–93.
- Küchler, S., Radowski, R.M., Haag, R., Schäfer-Korting, M. (2008). Comparison of solid lipid nanoparticles and dendritic core-multishell nanoparticles as drug delivery systems for topical application. European Workshop on Particulate Systems, Berlin.
- Kuo, Y.-C., Chen, H.-H. (2009). Entrapment and release of saquinavir using novel cationic solid lipid nanoparticles. Int J Pharm. 365:206–13.
- Law, S.L., Huang, K.J., Chiang, C.H. (2000). Acyclovir-containing liposomes for potential ocular delivery: corneal penetration and absorption. J Contr Rel. 63:135–40.
- Lherm, C., Müller, R.H., Puisieux, F., Couvreur, P. (1992). Alkylcyanoacrylate drug carriers: II. Cytotoxicity of cyanoacrylate nanoparticles with different alkyl chain length. Int J Pharm. 84:13–22.
- Li, X., Lin, X., Zheng, L., Yu, L., Lv, F., Zhang, Q., Liu, W. (2008a). Effect of poly(ethylene glycol) stearate on the phase behavior of monocaprate/Tween80/water system and characterization of poly(ethylene glycol) stearate-modified solid lipid nanoparticles. Colloid Surf Physicochem Eng Aspect. 317:352–9.
- Li, X., Nie, S.-F., Kong, J., Li, N., Ju, C.-Y., Pan, W.-S. (2008b). A controlled-release ocular delivery system for ibuprofen based on nanostructured lipid carriers. Int J Pharm. 363:177–82.
- Lin, X., Li, X., Zheng, L., Yu, L., Zhang, Q., Liu, W. (2007). Preparation and characterization of monocaprate nanostructured lipid carriers. Colloid Surf A Physicochem Eng Aspects. 311:106–11.
- Liu, X., Zhang, Y., Tang, X., Zhang, H. (2009). Determination of entrapment efficiency and drug phase distribution of submicron emulsions loaded silybin. J Microencapsul. 26:180–6.
- Liu, Z., Pan, W., Nie, S., Zhang, L., Yang, X., Li, J. (2005). Preparation and evaluation of sustained ophthalmic gel of enoxacin. Drug Dev Ind Pharm. 31:969–75.
- Lv, Q., Yu, A., Xi, Y., Li, H., Song, Z., Cui, J., Cao, F., Zhai, G. (2009). Development and evaluation of penciclovir-loaded solid lipid nanoparticles for topical delivery. Int J Pharm. 372:191–8.
- Mainardes, R.M., Urban, M.C.C., Cinto, P.O., Khalil, N.M., Chaud, M.V., Evangelista, R.C., Daflon Gremiao, M.P. (2005). Colloidal carriers for ophthalmic drug delivery. Curr Drug Target. 6:363–71.
- Marengo, E., Cavalli, R., Caputo, O., Rodriguez, L., Gasco, M.R. (2000). Scale-up of the preparation process of solid lipid nanospheres. Part I. Int J Pharm. 205:3–13.
- Mehnert, W., Mäder, K. (2001). Solid lipid nanoparticles: production, characterization and applications. Adv Drug Deliv Rev. 47:165–96.
- Meisner, D., Mezei, M. (1995). Liposome ocular delivery systems. Adv Drug Deliv Rev. 16:75–93.
- Moshfeghi, A.A., Peyman, G.A. (2005). Micro- and nanoparticulates. Adv Drug Deliv Rev. 57:2047–52.
- Müller, R.H., Lucks, J.S. (1996). Arzneistoffträger aus festen Lipidteilchen-Feste Lipid Nanosphären (SLN), European patent EP 0 605 497 B1.
- Müller, R.H., Mäder, K., Gohla, S. (2000). Solid lipid nanoparticles (SLN) for controlled drug delivery—a review of the state of the art. Eur J Pharm Biopharm. 50:161–77.
- Müller, R.H., Radtke, M., Wissing, S.A. (2002). Nanostructured lipid matrices for improved microencapsulation of drugs. Int J Pharm. 242:121–8.
- Nagarwal, R.C., Kant, S., Singh, P.N., Maiti, P., Pandit, J.K. (2009). Polymeric nanoparticulate system: a potential approach for ocular drug delivery. J Contr Rel. 136:2–13.
- Nilüfer, Y., Aysegül, K., Yalcin, O., Ayhan, S., Sibel, A.O., Tamer, B. (2003). Enhanced bioavailability of piroxicam using Gelucire 44/14 and Labrasol in vitro and in vivo evaluation. Eur J Pharm Biopharm. 56:453–9.
- Olbrich, C., Bakowsky, U., Lehr, C.-M., Müller, R.H., Kneuer, C. (2001). Cationic solid-lipid nanoparticles can efficiently bind and transfect plasmid DNA. J Contr Rel. 77:345–55.
- Pardeike, J., Hommoss, A., Müller, R. H. (2009). Lipid nanoparticles (SLN, NLC) in cosmetic and pharmaceutical dermal products. Int J Pharm. 366:170–84.
- Pouton, C.W., Porter, C.J.H. (2008). Formulation of lipid-based delivery systems for oral administration: materials, methods and strategies. Adv Drug Deliv Rev. 60:625–37.
- Radomska-Soukharev, A. (2007). Stability of lipid excipients in solid lipid nanoparticles. Adv Drug Deliv Rev. 59:411–8.
- Reichl, S., Bednarz, J., Muller-Goymann, C.C. (2004). Human corneal equivalent as cell culture model for in vitro drug permeation studies. B J Ophthalmol. 88:560–5.
- Reichl, S., Döhring, S., Bednarz, J., Müller-Goymann, C.C. (2005). Human cornea construct HCC—an alternative for in vitro permeation studies? A comparison with human donor corneas. Eur J Pharm Biopharm. 60:305–8.
- Ronald, D.S., Hong-Shian, H. (1983). Corneal penetration behavior of beta-blocking agents I: physicochemical factors. J Pharm Sci. 72:1266–72.
- Sahoo, S.K., Dilnawaz, F., Krishnakumar, S. (2008). Nanotechnology in ocular drug delivery. Drug Discov Today. 13:144–51.
- Sawant, K.K., Dodiya, S.S. (2008). Recent advances and patents on solid lipid nanoparticles. Recent Patents Drug Deliv Formulation. 2:120–35.
- Schwarz, C., Mehnert, W. (1997). Freeze-drying of drug-free and drug-loaded solid lipid nanoparticles (SLN). Int J Pharm. 157:171–9.
- Seal, D.V., Bron, A.J., Hay, J. (1998). Ocular infection; investigation and treatment in practice., London: Martin Dunitz Ltd.
- Shen, J., Sun, M., Ping, Q., Ying, Z., Liu, W. (2010). Incorporation of liquid lipid in lipid nanoparticles for ocular drug delivery enhancement. Nanotechnology. 21:1–10.
- Shen, J., Wang, Y., Ping, Q., Xiao, Y., Huang, X. (2009). Mucoadhesive effect of thiolated PEG stearate and its modified NLC for ocular drug delivery. J Contr Rel. 137:217–23.
- Sjöström, B., Bergenståhl, B. (1992). Preparation of submicron drug particles in lecithin-stabilized o/w emulsions I. Model studies of the precipitation of cholesteryl acetate. Int J Pharm. 88:53–62.
- Souto, E.B., Mehnert, W., Müller, R.H. (2006). Polymorphic behaviour of Compritol 888® ATO as bulk lipid and as SLN and NLC. J Microencapsul. 23:417–33.
- Souto, E.B., Wissing, S.A., Barbosa, C.M., Müller R.H. (2004). Development of a controlled release formulation based on SLN and NLC for topical clotrimazole delivery. Int J Pharm. 278:71–7.
- Trotta, M., Cavalli, R., Carlotti, M.E., Battaglia, L., Debernardi, F. (2005). Solid lipid micro-particles carrying insulin formed by solvent-in-water emulsion-diffusion technique. Int J Pharm. 288:281–8.
- Trotta, M., Debernardi, F., Caputo, O. (2003). Preparation of solid lipid nanoparticles by a solvent emulsification-diffusion technique. Int J Pharm. 257:153–60.
- Uchegbu, I.F., Vyas, S.P. (1998). Non-ionic surfactant based vesicles (niosomes) in drug delivery. Int J Pharm. 172:33–70.
- Urtti, A. (2006). Challenges and obstacles of ocular pharmacokinetics and drug delivery. Adv Drug Deliv Rev. 58:1131–5.
- Urtti, A., Salminen, L. (1993). Minimizing systemic absorption of topically administered ophthalmic drugs. Surv Ophthalmol. 37:435–56.
- Vandamme, T.F. (2002). Microemulsions as ocular drug delivery systems: recent developments and future challenges. Progr Retin Eye Res. 21:15–34.
- Velpandian, T., Velpandian, T. (2009). Intraocular penetration of antimicrobial agents in ophthalmic infections and drug delivery strategies. Expert Opin Drug Deliv. 6:255–70.
- Vighi, E., Ruozi, B., Montanari, M., Battini, R., Leo, E. (2007). Re-dispersible cationic solid lipid nanoparticles (SLNs) freeze-dried without cryoprotectors: characterization and ability to bind the pEGFP-plasmid. Eur J Pharm Biopharm. 67:320–8.
- Vyas, S.P., Rai, S., Paliwal, R., Gupta, P.N., Khatri, K., Goyal, A.K., Vaidya, B. (2008). Solid lipid nanoparticles (SLNs) as a rising tool in drug delivery science: one step up in nanotechnology. Curr Nanosci. 4:30–44.
- Wadhwa, S., Paliwal, R., Paliwal, S.R., Vyas, S.P. (2009). Nanocarriers in ocular drug delivery: an update review. Curr Pharm Des. 15:2724–50.
- Westesen, K., Bunjes, H. (1995). Do nanoparticles prepared from lipids solid at room temperature always possess a solid lipid matrix? Int J Pharm. 115:129–31.
- Westesen, K., Bunjes, H., Koch, M.H.J. (1997). Physicochemical characterization of lipid nanoparticles and evaluation of their drug loading capacity and sustained release potential. J Contr Rel. 48:223–36.
- Westesen, K., Siekmann, B. (1997). Investigation of the gel formation of phospholipid-stabilized solid lipid nanoparticles. Int J Pharm. 151:35–45.
- Wissing, S.A., Kayser, O., Müller, R.H. (2004). Solid lipid nanoparticles for parenteral drug delivery. Adv Drug Deliv Rev. 56:1257–72.
- Yasukawa, T., Kimura, H., Tabata, Y., Ogura, Y. (2001). Biodegradable scleral plugs for vitreoretinal drug delivery. Adv Drug Deliv Rev. 52:25–36.
- Yun, J., Zhang, S., Shen, S., Chen, Z., Yao, K., Chen, J. (2009). Continuous production of solid lipid nanoparticles by liquid flow-focusing and gas displacing method in microchannels. Chem Eng Sci. 64:4115–22.
- Zhang, S.-H., Shen, S.-C., Chen, Z., Yun, J.-X., Yao, K.-J., Chen, B.-B., Chen, J.-Z. (2008). Preparation of solid lipid nanoparticles in co-flowing microchannels. Chem Eng Sci. 144:324–8.
- Zimmer, A., Kreuter, J. (1995). Microspheres and nanoparticles used in ocular delivery systems. Adv Drug Deliv Rev. 16:61–73.
- Zimmermann, E., Müller, R.H., Mäder, K. (2000). Influence of different parameters on reconstitution of lyophilized SLN. Int J Pharm. 196:211–3.
- Zur Mühlen A., Schwarz, C., Mehnert, W. (1998). Solid lipid nanoparticles (SLN) for controlled drug delivery—drug release and release mechanism. Eur J Pharm Biopharm. 45:149–55.