Abstract
The use of glucocorticoids in the treatment of rheumatoid arthritis has been widely employed, but, owing to their systemic side-effects and also their susceptibility to the first pass metabolism, their use is being discouraged. To circumvent this, triamcinolone (TA) were encapsulated in chitosan microspheres with glutaraldehyde as the cross-linking agent to achieve a prolonged drug release. The percentage of drug loading, encapsulation efficiency, and surface morphology by Scanning electron microscopy (SEM), Phase transition by Differential scanning colorimetry (DSC), as well as Fourier transform infrared spectroscopy (FTIR) studies was carried out to characterize the chitosan microspheres. In-vitro and in-vivo release studies revealed that microspheres were able to control the release of TA with a uniform release pattern up to a period of 36 days and thereafter an extended release up to 63 days. The clinical parameters were investigated for changes in paw volume, hematological parameters like Erythrocyte sedimentation rate (ESR), Paced cell volume (PCV), Total leucocyte count (TLC), Hb, and Differential cell count (DCC) in Fruend’s complete adjuvant induced arthritic rats. Histopathological findings as well as radiology (X-ray) further confirmed the effectiveness of TA encapsulated microspheres in mitigating the rat arthritic model.
Introduction
Rheumatoid arthritis (RA) is a chronic auto immune disease, characterized by inflammation of the synovial lining of the joints and destruction of cartilage and bone (CitationJoosten et al., 2006). Glucocorticoids have been used as effective anti-inflammatory agents (CitationBarnes, 1998) with possible disease modifying activity (CitationChaudhuri & Paul, 2008). The mechanism of action of glucocorticoids starts at the molecular level by binding to specific receptors in the cytoplasm and then migrating to the nucleus, where they bind to selective regulatory sites on DNA, which results in the increased or decreased expression of genes important for the inflammatory process (CitationSteiner et al., 2009). Currently, there are several reports regarding the use of disease-modifying anti-rheumatic drugs (DMARDs), which are believed to modify the fundamental pathological process, but the treatment by DMARDs has to be individualized (CitationSilva et al., 2005). Moreover, methotrexate is the mostly prescribed DMARD, combination therapy of methotrexate, sulfasalazine, and hydroxyl chloroquine or methotrexate and cyclosporine are also preferred. Although methotrexate is well tolerated, potential side-effects include stomatitis, reversible bone marrow suppression, rarely liver fibrosis, cirrhosis, etc. DMARDs work more quickly, but, considering the cost factor, DMARDs are not advisable for long-term therapy. For that reason, doctors frequently prescribe an additional drug such as Glucocorticoids to help control pain and inflammation. However, it was reported (CitationShin et al., 2000) that triamcinolone was susceptible to the first pass metabolism and the systemic side-effects followed by long-term administration of steroidal anti-inflammatory drug (CitationPang et al., 2002). In order to circumvent the problems associated with systemic side-effects and to broaden the spectrum of therapeutic application, obviously there is a need for drug delivery systems that can provide site-specific and continuous therapeutic drug levels for extended periods of time. The use of polymeric carriers in formulations of therapeutic drug delivery systems has gained widespread application, due to their advantage of being biodegradable and biocompatible (CitationMu & Feng, 2002; CitationDhanaraju et al., 2003). The use of naturally occurring proteins as drug carriers has attracted considerable attention over the past years (CitationDhanaraju et al., 2003). Chitosan [Poly (ß-1→4)-2 amino-2-deoxy-p-glucose)], a natural cationic polysaccharide derived from chitin (CitationRavikumar, 2001) combines the properties of bio-compatibility, biodegradability (CitationSinha et al., 2004; CitationKang et al., 2009), non-toxicity, and bioabsorbability (CitationGupta & Jabrail, 2007).
The purpose of this study was to prepare triamcinolone-encapsulated chitosan microspheres by chemical cross-linking using a varying amount of glutaraldehyde saturated toluene. The percentage of drug loading, entrapment, encapsulation efficiency, and surface morphology (SEM), Phase transition (DSC) as well as FTIR studies was carried out to characterize the chitosan microspheres. The triamcinolone-loaded chitosan microspheres were kept for in vitro drug release experiments as well as in vivo drug release studies. The clinical parameters were investigated for changes in paw volume, hematalogical parameters like erythrocyte sedimentation rate (ESR), packed cell volume (PCV), total leucocyte count (TLC), haemoglobin, and differential cell count (DCC). Moreover, histopathological and radiological observations were also studied to determine the effectiveness of the microsphere formulation.
Materials and methods
Materials
Chitosan having a viscosity average molecular weight of 3.15 × 105 and a degree of deacetylation of 74% was obtained from Central Institute of Fisheries and Technology (Cochin, India) and used as received. Glutaraldehyde (25% aqueous solution, biological grade), sorbitan sesquioleate, liquid paraffin of viscosity 18cP at 30°C, petroleum ether, toluene, sodium metabisulfite, acetone, ethanol, and other chemicals were from SD Fine Chemicals Ltd. (Mumbai, India). Triamcinolone was a gift sample from Themis Medicare Ltd. (Gujarat, India).
Microsphere preparation
The manufacture of microspheres was done according to a method described in the literature (CitationJameela et al., 1998). A weighed amount of triamcinolone (TA) (20% by weight of chitosan) was mixed with 6 g of 4% chitosan solution. The chitosan solution was made in 5% acetic acid containing 2% sodium chloride. The above drug-chitosan solution was dispersed in a mixture of 35 ml of liquid paraffin and 25 ml of petroleum ether containing ~ 0.85 g of sorbitan sesquioleate in a 250 ml beaker. This dispersion was stirred using a stainless steel half-moon paddle stirrer at 2000 rpm for 15 min; 10 ml of glutaraldehyde saturated toluene was added and the stirring was continued for another 30 min. At the end of 30 min, 1 ml of aqueous glutaraldehyde was added and stirring was continued further for 3 h. The amount of glutaraldehyde-saturated toluene was varied in order to produce four different batches of microsphere formulation. The hardened microsphere were then separated by centrifugation, washed with petroleum ether, acetone, sodium metabisulfite, and finally with water, centrifuged, air-dried, and then stored in a desiccator.
Determination of drug loading and encapsulation efficiency
Drug loading in microspheres can be estimated by the equation
where L = percentage loading of microspheres, Wm = weight of microspheres in grams, Qm = quantity of drug present in Wm grams of microspheres.
The amount of drug encapsulated was determined by taking a known weight of microspheres into screw-capped vials with 0.1 N HCl and digested for 24 h on a magnetic stirrer in order to extract the entrapped drug completely. After suitable dilution absorbance was measured at 240 nm by using a UV-Spectrophotometer (ELICO-SL151). Blank microspheres treated in a similar manner were used as the blank (CitationRamachandran et al., 2010). Amount of drug encapsulated in the microspheres was determined by
where E = the percentage of encapsulated microspheres, QP = Quantity of drug encapsulated in microspheres (gm), Qt = Quantity of drug added for encapsulation (gm).
Scanning electron microscopy (SEM)
The surface morphology of the microspheres was examined using a Scanning Electron Microscope (JOEL-JFC-5300). The microspheres containing drugs were sprinkled onto one side of the double-sided adhesive stub. The stub was then coated with conductive gold with a JOEL-JFC 1100E sputter coater, and examined under a scanning electron microscope.
Differential scanning colorimetry (DSC)
The DSC scans of pure triamcinolone, Chitosan polymer, and TA-loaded chitosan microspheres were performed using a Perkin-Elmer DSC-7. About 4 mg of sample was placed in hermetically sealed aluminium pans and were heated at a scan speed of 20°C/min over a temperature range of 50–300°C. The heat of fusion was calibrated with indium.
Fourier transform infrared spectroscopy (FTIR) studies
The infrared spectra of TA, chitosan, and TA-loaded chitosan microsphere were recorded on a ABB Bomen MB series (Quebec, QC, Canada). The samples for IR measurements were prepared by a conventional KBr pelleting method. About 5 mg of sample was mixed with 50 mg of spectroscopic grade KBr. The measurements were made at 25°C.
In-vitro drug release studies
The in-vitro release of drug-loaded chitosan microspheres was carried out at 37°C using phosphate buffer pH 7.4. Each batch of the prepared microspheres equivalent to 25 mg of TA were taken in a 100 ml Erlenmeyer flask and made up to volume with phosphate buffer pH 7.4. The standard flasks were subjected to agitation, aliquots of 5 ml were withdrawn at equal intervals of time, and drug concentration was assayed at 240 nm by using a UV-Spectrophotometer (ELICO-SL151). Fresh solution of phosphate buffer pH 7.4 equivalent to the volume withdrawn was replaced in order to maintain sink conditions.
In-vivo release studies
Experiments were carried out on male Wistar rats weighing ~ 200–250 g, which were divided into six groups. The groups were injected with a dose of 3 mg/kg body weight of TA powder and an equivalent dose in microsphere formulation both intraperitoneally (IP) and intramuscularly (IM). Blood samples, 1.5 ml each, were collected at equivalent intervals of time, centrifuged at 3000 rpm for 10 min, and plasma was separated. Plasma TA concentration was analysed using High Performance Liquid Chromatography (HPLC).
HPLC conditions
The determination of TA in plasma was carried out by a modified method reported earlier (CitationDerendorf et al., 1986). The mobile phases consisted of acetonitrile:water:phosphoric acid (85%) in the ratio of 28:72:0.15 at a flow rate of 1.0 ml/min. The detector wavelength was set at 254 nm and the column was used at a temperature of 25°C. The stationary phase used was a Hypersil C-18 (5 μm) column, 250 × 4.6 mm.
Animal treatment and induction of arthritis
Experiments were performed on male Wistar rats (average weight 225–250 g; n = 10/group). They were housed in individual cages and maintained under conditions (24 ± 1°C; 12 h light/dark cycle; food and water available ad libitum) (CitationKumar et al., 2005). This study had the approval of the Institutional Animal Ethical Committee (IAEC) (Vels College of Pharmacy, Pallavaram, Chennai-600 117, India). Group I-CFA+ saline, group II-CFA+TA microspheres, and group III-CFA + pure TA solution. On day 0, arthritis was induced by intradermal injection of 0.1 ml of Freunds’ complete adjuvant at the base of the tail. For 15 days the rats were housed without treatment to maintain the full development of inflammatory arthritic condition. On day 15 treatments was started and microspheres equivalent to 3 mg/kg of rat body weight were administered intraperitoneally as per schedule. The arthritis control group received only saline instead of the drug or the drug-encapsulated microspheres.
Assessment of clinical parameters
Measurement of hind paws volume
Arthritis was quantitatively determined daily by measurement of ankle volume using a plethysmometer. For a consistent measurement, both hind limbs were shaved and a line was marked just above the ankle joint. On day 15 the paw volume was recorded as the initial value, and again the paw volume was recorded on day 30 as well as on day 60 of the study period. The percentage inhibition of increase in thickness of the injected foot was obtained by the formula, as given by CitationFernández et al. (1997).
where Vc is the mean increase of paw volume of control animals, and Vt is the ean increase of paw volume of treated animals.
Hematological parameters
Erythrocyte sedimentation rate (ESR) was carried out by Wintrobe’s method; ~ 1 ml of blood is mixed with the anti-coagulant, EDTA. The blood is loaded in the tube up to the ‘0’ mark and the tube placed on the wintrobe’s stand. The reading was taken after 1 h. The packed cell volume (PCV) was determined by centrifuging heparinized blood in a capillary tube (microhematocrit tube) at 10,000 rpm for 5 min. The routine laboratory experiments, total leucocyte count (TLC), heemoglobin (Hb), and differential cell count (DCC) were also conducted.
Radiological observation
The formalin fixed ankle joints of the rats under study were subjected to radiography on Seimens Tridors (800 mA at 45 cm focal distance, 36 KV, and 2 mA exposure). The exposures were recorded on 4.75 × 6.5 inch x-ray films.
Histological evaluation
Hock joints of the rats were collected in 10% formalin saline. Paraffin-embedded tissues were sectioned to 5 μm thickness and stained by hematoxylin and eosin stain for histopathological examination.
The results were expressed as mean values ± SEM. One way ANOVA with Dunnet’s t-test was applied to evaluate the differences between the control and the treatment groups.
Results and discussion
Preparation of TA-loaded chitosan microspheres was carried out by a chemical cross-linking method using glutaraldehyde saturated toluene as a cross-linking agent. Microspheres were prepared from the natural polysaccharide, chitosan, which suggests its use in humans because of its unique biocompatibility, biodegradability, and non-toxicity characteristics. The prepared microspheres were characterized for drug loading and encapsulation efficiency (). The percentage of drug loading of prepared microspheres was found to be 9.14–11.16, and encapsulation efficiency was 48–55.2%. The surface morphology of the prepared microsphere was investigated by scanning electron micrography (SEM). illustrates surface morphology of microspheres, which reveals small and distributed uniformly. The surface of the microspheres was spherical and smooth without hollows or deformations. TA-loaded microspheres possessed yellow-brown color with good sphericity and compact structure. Increase in color strength represents an increased rate of glutaraldehyde cross-linking (CitationÇelik & Akbuğa, 2007), and good sphericity may also be due to higher glutaraldehyde cross-linking that occurs through a Schiff’s base reaction between aldehyde ends of the cross-linking agent and the amine moieties of chitosan to form aldimine functions (CitationVieira & Beppu, 2006). The particle size of TA-loaded microspheres ranged between 2–18 µm. The DSC analysis was performed in order to assess the physical state of drug inside the chitosan microspheres and check for the possibility of any interaction between the drug and the polymer (CitationRamachandran et al., 2010). DSC thermograms of chitosan (pure), TA (pure), and TA-loaded chitosan microspheres are shown in , respectively. Under the experimental conditions no characteristic endothermy was obtained for pure chitosan, as reported earlier (CitationHe et al., 1999; CitationEl-Gibaly, 2002). However, thermograms of pure TA ) revealed endothermic peaks at 278°C, which clearly resembles the reported value of the melting point of triamcinolone. It should be noted that in the drug-loaded microspheres no such endothermic peak was observed, which indicates molecular dispersion of these drugs inside the matrix of chitosan as a solid solution (CitationHe et al., 1999).
Table 1. Percentage of drug loading, encapsulation efficiency of chitosan microspheres.
Figure 1. Scanning electron micrograph (SEM) of TA-loaded chitosan microspheres. (a) TA microspheres (10:1), (b) TA microspheres (5:1).
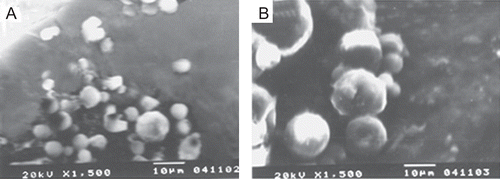
Figure 2. Differential scanning calorimetry (DSC) results of pure chitosan (a), pure TA (b), and TA-loaded chitosan microspheres (c).
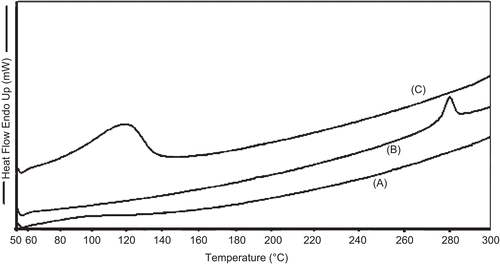
FTIR analysis measures the selective absorption of light by the vibrational modes of specific chemical bonds in the sample (CitationMu & Feng, 2002). represent the FTIR spectra of pure chitosan, pure TA, and TA-loaded chitosan microspheres, respectively. In , of pure chitosan, the characteristic broad absorption band at 3412.82 cm−1 represents the presence of a hydrogen bonded O-H group. The amino group has a characteristic absorption band in the region 3400–3500 cm−1 which must have been masked by the absorption band due to the O-H group (CitationOrienti et al., 2002). The C-H stretching vibration of the polymer backbone is manifested at 2931.73 cm−1. The ether linkage shows characteristic absorption at 1154.28 cm−1. For drug-loaded chitosan microspheres an additional peak at 1707.91 cm−1 was observed which corresponds to the C=0 stretching vibration of pure drugs.
The in vitro drug release profile illustrated in represents the percentage of TA release with respect to the amount of drugs encapsulated. The release from the microspheres having high cross-linking density was slower compared to the release from the microspheres having low cross-linking density (CitationIwanaga et al., 2003). Within 14 days ~ 66.25% of the incorporated TA was released from TA-microspheres of low cross-linking density, whereas only 23.53% of the incorporated TA was released from TA-microspheres of high cross-linking density. This shows that cross-linking effectively controls the drug diffusion from the chitosan microspheres as reported earlier (CitationMitra et al., 2001). A similar result was also reported by CitationKo et al. (2002), where the release profile of drug from TPP-Chitosan microspheres decreased with increased cross-linking agent concentration. An initial burst release was depicted in all the batches following a sustained release between the 17th and the 42nd day, and thereafter an extended release up to 63 days. An initial burst effect was observed in all the batches of microsphere formulations, which may be due to the drug being adsorbed (or) located near the surface of the microspheres (CitationRavi et al., 2008). This might probably be due to the existence of drug crystals in the droplets, owing to its slight water solubility during the formation of (w/o) emulsion (CitationWang et al., 1996). When a water-soluble (or even slightly water-soluble) drug is encapsulated into microspheres, the release rate is often rapid and accompanied by a burst effect (CitationRavi et al., 2008). This was not the case as reported in previous studies (CitationJameela et al., 1998), where a noteworthy low burst of ~ 5% was released from microspheres cross-linked with aqueous glutaraldehyde. The initial phase was followed by a plateau region of gradual release between the 17th day to 42nd day, the 1:1 formulation of TA-loaded chitosan microspheres being an exception where the release lasted only up to the 14th day. A similar extended release was reported by CitationJameela et al. (1994) about mitoxantrone, an anti-neoplastic agent for over 4 weeks at 27°C. A similar biphasic release was observed by CitationWang et al. (1996), where the release of CDDP from the chitosan microspheres in saline was sustained for more than 6 h with an initial rapid release phase (CitationBodmeier & Chen, 1998).
The plasma concentration of triamcinolone in male Wistar rats administered with TA powder and the TA chitosan microsphere in the ratio of 10:1 and 5:1 both intra-peritoneal (I.P) and intra-muscularly (I.M) are shown in . Animals which received 10:1 TA microspheres via an intra-peritoneal route showed an extended steroid concentration of 3–4 μg/ml1, on the other hand the 10:1 TA microspheres injected intramuscularly showed a prolonged release of steroid at a concentration of 3 μg/ml, whereas pure drug shows 18.23 μg/ml at 6 h and 0.102 μg/ml at 24 h when injected intra-peritoneal and intra-muscularly, respectively.
shows that there is an elevated plasma level initially followed by a slow decline in the release rate as the biodegradation of matrix reaches a substantial level and then a second elevated drug level is observed. As demonstrated in the in-vitro data the mechanism of drug release from the chitosan microspheres appears to be predominantly by diffusion as cross-linked chitosan degrades very slowly in in-vivo (CitationOfokansi & Adikwu, 2007). The peak serum concentration of ~ 16.84 μg/ml at 6 h was dropped to ~ 3.1 μg/ml on the 7th day. A similar decrease was observed for all the batches. A similar extended release was reported by CitationJameela et al. (1998). Each of the batches showed an elevated serum level in the initial stage followed by a consistent release pattern up to a period of 36 days and thereafter an extended release up to 63 days. The second elevated drug level was observed in TA microspheres (10:1) injected I.M (CitationJameela et al., 1998).
To evaluate the therapeutic efficacy of the TA-loaded microspheres, clinical parameters such as paw volume, hematological parameters, radiology, and histopathological studies were performed. All hematological parameters were done by blood collected exsanguination in Heller and Paul anti-coagulant mixture. Erythrocyte sedimentation rate was determined by Wintrobe method, and packed cell volume was determined by Microhematocrit method. Hemoglobin was determined by cyanometheglobin method, and total leucocyte count and differencial cell count were performed using Thomas fluid (diluting fluid) and Leishman–Giemsa stain, respectively. illustrates results of paw volume with percentage inhibition and hemoglobin content. Group II differed from a group I, the arthritic control and group III, treatment group with pure TA solution. The results confirm the effectiveness of drug loaded microspheres as the groups injected with pure drug solution showed a strong relapse as seen in data on the 60th day. Since no significant results were obtained for packed cell volume, data is not shown. and depict results of total leucocyte count and differential cell count, respectively. Although the treatment groups which were injected with pure TA solutions depicted a marked change on the 30th day, they failed to produce therapeutic activity on the 60th day, depicting a similar manifestation of inflammation as observed in the control groups. On the 60th day the groups treated with drug-encapsulated chitosan microspheres revealed the efficacy of the carrier systems.
Table 2. Paw volume with percentage inhibition and hemoglobin content.
Table 3. Total leucocytes count.
Table 4. Differential cell count.
Therapeutic efficacy was further confirmed by histopathology and radiology. The formalin-fixed ankle joints of the rats under study were subjected to radiography on Seimens Tridors (800 mA at 45 cm focal distance, 36 KV and 2 mA exposure). The exposures were recorded on 4.75 × 6.5 inch x-ray films, No evidence of bone damage and erosion was observed in Wistar rats treated with TA-loaded chitosan microspheres. A representative X-ray of normal ankle joint is shown in , an X-ray of joint from a rat treated with vehicle (control) is shown in , and TA-loaded chitosan microspheres treaded ankle joint is shown in . Radiographic evidence of disintegration and erosion of surface in the vicinity of the inflamed joints is clearly apparent in the arthritic rats treated with vehicle (control) alone, whereas bone is preserved, or bone destruction and erosion retarded, in rats treated with TA-loaded microspheres. Notably, the joint deformity observed in control rats was eliminated, leaving the appearance of damage indistinguishable from that of naive animals.
Figure 6. Radiographs of joint were obtained at the conclusion of the study. Representative radiographs from Wistar rats with normal (a), Arthritic control (b), and TA-loaded chitosan microsphere treated (c).
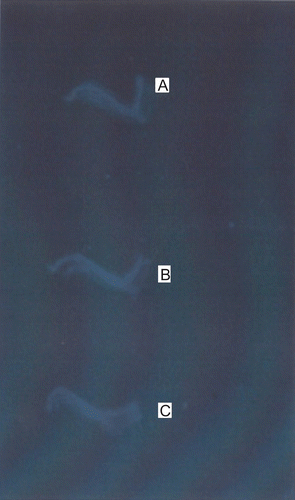
Hock joints of the rats were collected in 10% formalin saline. Paraffin embedded tissues were sectioned to 5 μm thickness and stained by hematoxylin and eosin stain. Hock joints of the rats were evaluated for histopathological changes in cartilage structure, cell layer, infiltration of mononuclear cells, and synovial inflammation indicated that arthritic rats exhibited extensive inflammatory infiltrate within the synovium and synovial hyperplasia. The histology of the inflamed tissue (arthritic control) depicted denudation of the lining cell layer and infiltration of mononuclear cells ), and depicted sub-epithelial edema and scattered leucocyte infiltration. show the normal architecture of synovial membrane. Following treatment on the 30th day, the histology revealed mild sub-epithelial fibrosis, surface synocytes showing hypertrophy, hyperplasia, and mild edema, congestion were also noted ). However, in the case of TA-loaded chitosan-treated groups on the 60th day mild hyperplasic changes in the lining epithelium, but gradual restoration of normal architecture was observed ).
Summary
The use of Glucocorticoids in inflammatory conditions has been illustrated in several publications (CitationHan et al., 2001; CitationPang et al., 2002) and also triamcinolone finds its use in treatment of osteoarthritis (CitationReynauld et al., 2003), thus triamcinolone was chosen as a model drug to treat the rat arthritic model. Fruend’s complete adjuvant was used to induce arthritis in rats, since the literature revealed that it produced the best animal model for the study of secondary inflammation (CitationChillingworth & Donaldson, 2003) and that it shares numerous behavioral and biochemical characteristics with rheumatoid arthritis. The use of CFA in inducing arthritis has enabled successful study of anti-inflammatory and analgesic activity for over a period of 30–60 days (CitationLichtenburger et al., 1999). In our study we illustrated the effectiveness of TA-loaded chitosan microspheres in a rat arthritic model over a period of 60 days since a drug release profile was obtained up to a period 63 days. Chitosan was found to serve as a matrix for controlled release systems and also easily degrades into non-toxic products (CitationKang et al., 2009). Cross-linking was mainly achieved to prolong the drug release which was accomplished up to a period of 63 days, as shown by the in-vitro release profiles. Literature sites a similar slow degradation of drug from cross-linked chitosan microspheres in-vivo over a period of 6 months (CitationJameela et al., 1994). Laboratory findings were supported in our study as RA is usually manifested by anemia, thrombocytopenia, neutropenia, thrombocytotosis, eosinophilia, and hematological malignancies. However, no marked changes were found in ESR rates and packed cell volume between the various test groups as well as the control. The synovial hyperplasia and bone destruction is a predominant feature of RA associated with pain and morbidity. Disintegration and erosion of bone is common, leading to the characteristic erosions seen on radiography. Notably, our studies demonstrated that no radiographic damage whatsoever was observed in histology of cut sections of rat hock joints and similar to those reported in previous studies where arthritis was induced by squalene (CitationCarlson et al., 2000). Histological findings as well as radiology further confirmed the effectiveness of drug-encapsulated microspheres in mitigating the rat arthritic model. The groups which received pure solutions of these drugs showed a relapse of arthritic condition as compared to the control rats, which were not observed in groups administered with drug-loaded microspheres, these support our development of drug carrier systems over a prolonged period of time.
Conclusion
Microsphere carrier systems formulated from the naturally occurring, biodegradable, and biocompatible chitosan polymers are gaining prominence in the field of novel drug delivery systems. Its property to achieve a predictable and reproducible release in specific environment over a sustained period of time with minimum side-effects is of great significance. This provides a wide opportunity for encapsulating drugs such as glucocorticoids, which are always regarded for their adverse effects making their utility a mere theory. Our study provides development of delivery systems for targeting active constituents to inflamed conditions such as those found in RA where the treatment period is usually longer.
Declaration of interest
The authors report no declaration of interest.
References
- Barnes, P.J. (1998). Anti-inflammatory actions of glucocorticoids: molecular mechanisms. Clin Sci. 94:557–72.
- Bodmeier, R., Chen, H. (1998). Preparation of biodegradable poly (±) - lactide microsphers using a spray - drying technique. J Pharm Pharmacal. 40:754–7.
- Carlson, B.C., Jansson, A.M., Larsson, A., Bucht, A., Lorentzen, J.C. (2000). The endogenous adjuvant squalene can induce a chronic T-cell-mediated arthritis in rats. Am J Pathol. 156:2057–65.
- Çelik, Ö, Akbuğ, J. (2007). Preparation of superoxide dismutase loaded chitosan microspheres: characterization and release studies. E J Pharm Biopharm. 66:1:42–7.
- Chaudhuri, K., Paul, A. (2008). Glucocorticoids and rheumatoid arthritis—a reappraisal. Ind J Rheumatology. 3:21–8.
- Chillingworth, N.L., Donaldson, L.F. (2003). Characterisation of a Freund’s complete adjuvant-induced model of chronic arthritis in mice. J Neurosci Meth. 128:1–2;45–52.
- Derendorf, H., Rohdewald, P., Hochhaus, G., Mollmann, H. (1986). HPLC determination of glucocorticoid alcohols, their phosphates and hydrocortisone in aqueous solutions and biological fluids. J Pharm Biomed Anal. 4:197–206.
- Dhanaraju, M.D., Kiran, V., Jayakumar, R., Vamsadhara, C. (2003). Preparation and characterization of injectable microspheres of contraceptive hormones. Int J Pharm. 268:23–9.
- El-Gibaly, I. (2002). Development and in vitro evaluation of novel floating chitosan microcapsules for oral use: comparison with non-floating chitosan microspheres. Int J Pharm. 249:7–21.
- Fernández, P.B., Peinado, I.I., Fresno, A.M.V. (1997). Anti-inflammatory and antiulcer activity of Teucrium buxifolium. J Ethnopharm. 55:93–8.
- Gupta, K.C., Jabrail, F.H. (2007). Glutaraldehyde cross-linked chitosan microspheres for controlled release of centchroman. Carbohydrate Res. 342:2244–52.
- Han, C.W., Choi, J.H., Kim, J.M., Kim, W.Y., Lee, K.Y., Oh, G.T. (2001). Glucocorticoid mediated repression of inflammatory cytokine production in fibroblast like rheumatoid synoviocytes is independent of nuclear factor-κ B activation induced by tumor necrosis factor. Rheumatology. 40:267–73.
- He, P., Davis, S.S., Illum, L. (1999). Chitosan microspheres prepared by spray drying. Int J Pharm. 187:53–65.
- Iwanaga, K., Yabuta, T., Kakemi, M., Morimoto, K., Tabata, Y., Ikada, Y. (2003). Usefulness of microspheres composed of gelatin with various cross-linking density. J Microencapsul. 20:767–76.
- Jameela, S.R., Kumary, J.V., Lal, A.U., Jayakrishnan, H. (1998). Progesterone - loaded chitosan microspheres: a long acting biodegradable controlled delivery system. J Contr Rel. 52:17–24.
- Jameela, S.R., Misra, A., Jayakrishnan, A. (1994). Crosslinked chitosan microspheres as carriers for prolonged delivery of macromolecular drugs. J Biomat Sci Polym. 6:621–32.
- Joosten, L.A.B., Netea, M.G.N., Kim, S.H., Yoon, D.Y., Walgreen, B.O., Radstake, T.R.D., Barrera, P., vandeLoo, F.A.J., Dinarello, C.A., vandenBerg, W.B. (2006). IL-32, a pro inflammatory cytokine in rheumatoid arthritis. PNAS. 103:3298–303.
- Kang, M.L., Cho, C.S., Yoo, H.S. (2009). Application of chitosan microspheres for nasal delivery of vaccines. Biotechnol Adv. 27:857–65.
- Ko, J.A., Park, H.J., Hwang, S.J., Park, J.B., Lee, J.S. (2002). Preparation and characterization of chitosan microparticles intended for controlled drug delivery. Int J Pharm. 249:165–74.
- Kumar, D.A., Kalidindi, V.S., Raju, N., Settu, K., Kumanan, K., Puvanakrishnan, R. (2005). Effect of a derivatized tetrapeptide from lactoferrin on nitric oxide mediated matrix metalloproteinase-2 production by synovial fibroblasts in collagen-induced arthritis in rats. Peptides. 27:1434–42.
- Lichtenburger, L.M., Romero, J.J., Ruijter W.M.J., de Behbod, F., Darling, R., Ashraf, A.Q., Sanduja, S.K. (1999). Phosphatidyl choline association increases the anti-inflammatory and analgesic activity of ibuprofen in acute and chronic models of joint inflammation: relationships to alterations in bioavailability and cyclooxygenase inhibitory potency. J Clin Endocrinol Metab. 84:2788–94.
- Mitra, S., Gaur, V., Ghosh, P.C., Maitra, A.N. (2001). Tumor targeted delivery of encapsulated dextran doxorubicin conjugate using chitosan nanoparticles as carrier. J Contr Rel. 74:317–23.
- Mu, L., Feng, S.S. (2002). Vitamin E TPGS used as emulsifier in the solvent evaporation/extraction technique for fabrication of polymeric nanospheres for controlled release of paclitaxel (taxol). J Contr Rel. 80:129–44.
- Ofokansi, K.C., Adikwu, M.U. (2007). Formulation and evaluation of microspheres based on gelatin-mucin admixtures for the rectal delivery of cefuroxime sodium. Trop J Pharm Res. 6:825–32.
- Orienti, I., Cerchiara, T., Luppi, B., Bigucci, F., Zuccari, G., Zecchi, V. (2002). Influence of different chitosan salts on the release of sodium diclofenac in colon specific delivery. Int J Pharm. 238:51–9.
- Pang, Y.N., Zhang, Y., Zhang, Z.R. (2002). Synthesis of an enzyme-dependent prodrug and evaluation of its potential for colon targeting. World J Gastroenterol. 8:913–17.
- Ramachandran, S., Shaheedha, S.M., Thirumurugan, G., Dhanaraju, M.D. (2010). Floating controlled drug delivery system of famotidine loaded hollow microspheres (microballoons) in the stomach. Curr Drug Deliv. 1, 7:93–7.
- Ravi, S., Peh, K.K., Darwis, Y., Krishna Murthy, B., Raghu Raj Singh, T., Mallikarjun, C. (2008). Development and characterization of polymeric microspheres for controlled release protein loaded drug delivery system. Ind J Pharm Sci. 70:303–9.
- Ravikumar, M.N.V. (2001). A review of chitin and chitosan applications. Reactive Funct Polymers. 46:1–27.
- Reynauld, J.P., Buckland-Wright, C., Ward, R., Choquette, D., Haraoui, B., Martel-Pelletier, J., Uthman, I., Khy, V., Tremblay, J.L., Bertrand, C., Pelletier, J.P. (2003). Safety and efficacy of long term intra articular steroid injections in osteoarthritis of the knee: a randomized, double-blind, placebo-controlled trial. Arthritis Rheum. 48:370–7.
- Shin, S.C., Bum, J.P., Choi, J.S. (2000). Enhanced bioavailability by buccal administration of triamcinolone acetonide from bioadhesive gels in rabbits. Int J Pharm. 209:37–43.
- Silva, M.A., Ishii-Iwamoto, E.L., Bracht, A., Caparroz-Assef, S.M., Kimura, E., Cuman, R.K., Bersani-Amado, C.A. (2005). Efficiency of combined methotrexate/chloroquine therapy in adjuvant-induced arthritis. Fundam Clin Pharmacol. 19:479–89.
- Sinha, V.R., Singla, A.K., Wadhawan, S., Kaushik, R., Kumria, R., Bansal, K., Dhawan, S. (2004). Chitosan microspheres as a potential carrier for drugs. Int J Pharm. 274:1–33.
- Steiner, G., Urowitz M.B. (2009). Lipid profiles in patients with rheumatoid arthritis: mechanisms and the impact of treatment. Semin Arthritis Rheum. 38:372–81.
- Vieira, R.S., Beppu, M.M. (2006). Dynamic and static adsorption and desorption of Hg (II) ions on chitosan membranes and spheres. Water Res. 40:1726–34.
- Wang, Y.M., Sato, H., Adachi, I., Horiboshi, I. (1996). Optimization of the formulation design of chitosan micropheres containing cisplatin. J Pharm Sci. 85:1204–10.