Abstract
The selectivity of monoclonal antibodies against the E2 extracellular fragment of connexin 43 (Cx43) for a glioma focus was studied in in vivo experiments on animals with intracranial C6 glioma. Antibodies labeled with two alternative labels, the radioisotope 125I and the fluorophore Alexa 660, were intravenously injected to rats with 18-day gliomas. Seventy-two hours after injection, 125I-labeled antibodies accumulated in the hemisphere where the glioma was located to a concentration of 0.27 ± 0.01% of the injected dose per gram of wet weight, which exceeded their accumulation in the liver, spleen, and other organs. Fluorescent-labeled antibodies against the Cx43 fragment E2 specifically visualized cells in the peritumoral astroglial bank (a zone of active invasion of glioma cells). Double immunofluorescent visualization using antibodies against the Cx43 fragment E2 and glial fibrillar acidic protein (GFAP) showed that only a small proportion of the cells that bound the antibodies injected into the blood circulation were reactive astrocytes, whereas most of these cells were GFAP-negative and morphologically corresponded to astroblasts. These results suggest that antibodies against the extracellular Cx43 fragment E2 can be used for targeted transport of diagnostic and therapeutic drugs to the peritumoral invasion zone of high-grade gliomas.
Introduction
Connexin 43 (Cx43) is an integral membrane protein forming gap junctions between astroglial cells in the central nervous system (CitationProchnow & Dermietzel, 2008). Cx43 has four transmembrane domains and is oligomerized into hexamers (connexons) forming a pore in the cell membrane. Phosphorylation and dephosphorylation of C-terminal regions allow the transmembrane channel to be opened and closed (CitationBates et al., 2007). Hemichannels of neighboring cells are linked via two extracellular Cx43 fragments (E1 and E2) to form a functioning gap junction connecting the cytoplasms of these cells. The channels are involved in maintaining ion homeostasis and cell volume and transmitting various intercellular signals, including those that control the proliferation, differentiation, apoptosis, and migration of embryonic cells in the course of ontogeny (CitationOliveira et al., 2005; CitationClair et al., 2008; CitationDecrock et al., 2009).
The role of Cx43 in the development of invasive tumors of glial origin is being intensely studied. There is experimental evidence that Cx43 activates invasion of human glioblastoma multiforme and its rat analog, experimental C6 glioma (CitationErrede et al., 2002; CitationLin et al., 2002; CitationOliveira et al., 2005). In particular, Cx43-positive C6 glioma cells have been demonstrated to have a higher migration capacity than Cx43-negative cells and to be more resistant to oxidative stress and a number of other damaging factors (CitationBates et al., 2007; CitationGiardina et al., 2007).
Earlier, we obtained monoclonal antibodies against a recombinant E2 extracellular domain of Cx43 (MAbE2Cx43) interacting with Cx43 in the native conformation (CitationBaklaushev et al., 2009). This study also showed that reactive astrocytes positive for glial fibrillar acidic protein (GFAP) in the peritumoral zone of experimental C6 glioma produced large amounts of Cx43.
Thus, Cx43-positive cells of high-grade gliomas are promising targets in terms of both diagnosis, where they may be used to visualize actively migrating cells and determine the actual boundaries of a glioma, and the search for new therapeutic approaches to suppressing glioma cell invasion.
MAbE2Cx43 was earlier used for targeted transport of liposomal nanocontainers to cells of high-grade glioma cell culture (CitationChekhonin et al., 2010). The purpose of this study was to estimate the possibility of in vivo visualization of Cx43-positive cells in the glioma and peritumoral astroglial bank by intravenously injecting MAbE2Cx43 to animals with model C6 intracranial glioma.
Materials and methods
Glioblastoma modeling was performed by means of intracerebral stereotactic implantation of C6 glioma cells to 20 adult female Wistar rats weighing 200–220 g at the beginning of the experiment. Glioma cells (5 × 105 cells per animal) were implanted to the striatum region of ketamine-anesthetized (100 mg/kg) rats with a Narishige stereotactic device (Japan) at coordinates Ap −1, L 3.0, V 4.5, TBS −2.4 mm according to Swanson’s rat brain atlas. A cell suspension (5–7 μl) was injected at a rate of 3 μl/min using a Hamilton syringe connected with a microinjector.
Raising and preparing monoclonal antibodies
Monoclonal antibodies against the second extracellular fragment of Cx43 were obtained as described earlier (CitationBaklaushev et al., 2009). Hybridoma cells were retrieved from a cryobank and intraperitoneally injected to BALB/c mice. Ten days later, the animals were anesthetized, and ascites containing 3–4 mg/ml of monoclonal antibodies was taken. MAbE2Cx43 was purified from the ascites using affine chromatography on agarose-linked protein G (Invitrogen) as recommended by the manufacturer; then the antibody was concentrated to 3–5 mg/ml, aliquoted, and stored at −70°C.
Experiments with radioactive labels were performed in the Radioisotope Unit of the Institute of Bioorganic chemistry of the Russian Academy of Sciences (head, Yu. S. Skoblov, Dr Sci (Chem)). Purified preparations of monoclonal antibodies against the Cx43 fragment E2, as well as non-specific mouse immunoglobulins (IgGm), were conjugated with 125I (37 Bq per 200 μg of protein) using Iodogen (1,3,4,6-tetrachloro-3α,6α-diphenyl glycoluril, Sigma) buffered with 50 mM phosphate (pH 7.2) at room temperature for 20 min, and then separated from unbound iodine by gel filtration using Sefadex G50. The average effectiveness of radiolabeling by Iodogen was ~30%. The radiochemical purity of the conjugated antibodies was evaluated by thin-layer chromatography in 50% ethanol with a following measurement in flatbed scintillation gamma-counter (Imager, HP). In all experimental series, the radiochemical purity of MAbs was no less than 99%. The yield of radiolabeled antibodies after purification was more than 90% by total protein concentration. The loss of immunochemical activity of 125I-labeled MAbE2Cx43 evaluated by ELISA was no more than 30%. We took this loss into account when we assigned a dose of Ab.
Fluorescent labeling of antibodies against the Cx43 fragment E2 and non-specific mouse IgG was performed using the Alexa Fluor 660 Protein Labeling kit (Invitrogen) as recommended by the manufacturer. Under these conditions, three-to-four dye molecules were bound to an Ab molecule. The absorption peak of the Alexa Fluor 660 is at a wavelength of 663 nm and the emission peak at 690 nm.
The protocol of in vivo experiments
Radiolabeled antibodies
Preparations of 125I-labeled antibodies were injected to 10 animals with glioma (MAbE2Cx43 and non-specific IgGm being injected to five animals each). The injections were made into the femoral vein of ketamine-anesthetized animals on the 18th day after glioma cells were implanted into the brain. The specific radioactivities of the injected preparations of MAbE2Cx43 and IgGm were 1.80 ± 0.05 and 1.57 ± 0.04 mCi/mg, respectively. The preparations were injected at a dose of 25 μg/kg body weight.
To estimate the elimination of the antibodies from the peripheral blood flow, blood was sampled from experimental animals immediately after and 0.5, 1, 6, 24, 48, and 72 h after the antibody injection. Seventy-two hours after the injection of antibodies, the animals were deeply anesthetized and transcardially perfused with PBS. The left and right brain hemispheres, liver, spleen, lung, heart, and thyroid gland were extracted. A scintillation gamma counter was used to estimate the radioactivity in the preparations of blood and the extracted organs; the antibody accumulation in them was calculated in percent of the injected dose per gram of wet weight.
Fluorescent-labeled antibodies
Antibodies labeled with Alexa Fluor 660 were injected into the femoral veins of 12 rats with an 18-day experimental glioma at three concentrations (500, 100, and 50 μg/kg body weight), each concentration being administered to four rats. The control group consisted of four animals injected with 500 μg/kg of non-specific mouse immunoglobulins labeled with Alexa Fluor 660. Forty-eight hours after the administration of fluorescent-labeled antibodies, the animals were injected with an overdose of ketamine and transcardially perfused with cold 4% paraformaldehyde solution in PBS. The brain was post-fixed overnight in the same solution, after which 60-μm sections were made by means of a freezing microtome.
Immunohistochemistry
Additional immunochemical analysis for GFAP, an astroglial marker of intermediate filaments, was performed in the brain sections of the animals intravenously injected with MAbE2Cx43. The sections were incubated in a solution of polyclonal rabbit antibodies against GFAP (0.5 μg/ml) (Serbsky National Research Center for Social and Forensic Psychiatry) and afterwards in the presence of secondary anti-species antibodies labeled with Alexa Fluor 488 (Invitrogen) (1 μg/ml; green fluorescence). Fluorescence was detected by using the laser scanning confocal microscope Leica TCS SP5 STED. For deconvolution, scanning was performed at an excessive spatial frequency of 100 nm along xy and 125 nm along z. 3D data were deconvolved using the maximum likelihood algorithm (the SVI Hyugens software).
Results
The monoclonal antibodies raised against the E2 extracellular domain visualized Cx43-positive cells in specimens of primary rat astrocytes fixed with paraformaldehyde (), as well as Cx43-positive cells in live cultures of C6 rat glioma and U251 human glioblastoma (). In the latter case, only placoid connexon structures were seen on cell membranes.
Figure 1. Immunofluorescent analysis of Cx43 in fixed and live cell cultures with the use of monoclonal antibodies against the second extracellular fragment of Cx43 (MAbE2Cx43). (a) Red fluorescence reveals polyclonal antibodies against GFAP + Goat Antirabbit Alexa Fluor 594 (Invitrogen); green fluorescence, MAbЕ2Cx43 + Goat Antimouse Alexa Fluor 488 (Invitrogen). Cell nuclei have been stained with DAPI (Invitrogen). (b) Cx43 visualization in a live culture of U251 glioblastoma (MAbЕ2Cx43, Alexa Fluor 488, green fluorescence). Cell nuclei have been additionally stained with DAPI. (c) Cx43 visualization in a live culture of С6 glioma (MAbЕ2Cx43, Alexa Fluor 488, green fluorescence). Cell nuclei have been additionally stained with TOTO 633 (Invitrogen). Magnification, ×1000.
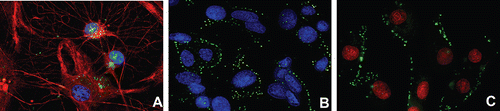
In none of the specimens did substances integrated in gap junctions display the fluorescence characteristic of connexon dimmers (the linear fluorescence in intercellular space of neighboring cells). Therefore, we concluded that the obtained antibodies allowed Cx43 to be visualized only at the stage of connexon presentation on the cell membrane in the form of hemichannels.
In vivo experiments were performed with monoclonal antibodies against the Cx43 fragment E2 conjugated with two different labels: a radioactive isotope with mild gamma-emission (125I) and a fluorescent dye Alexa Fluor 660 (Invitrogen). Labeled antibodies were injected on the 18th day after glioma cells implantation, when, according to earlier morphometric analysis, the glioma size was ~20% of the hemisphere volume (CitationChekhonin et al., 2007) and the astroglial reaction in peritumoral zone was strongly pronounced.
Analysis of blood radioactivity after injection of 125I-labeled antibodies showed that they circulated in the bloodstream for a relatively long time. The half-life of circulating specific antibodies and non-specific IgG was ~6 h (). However, specific antibodies were more rapidly eliminated from the blood: 72 h after the injection, the specific antibody and non-specific IgG concentrations in the blood were 7.3 ± 0.4% and 15.5 ± 1.2% (M ± σ) of their calculated initial values (C0), respectively.
Figure 2. The time course of the blood content of 125I-labeled monoclonal antibodies in rats with experimental glioma.
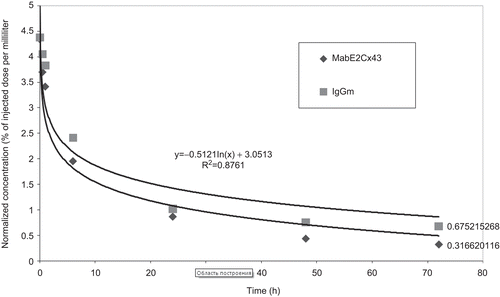
The distributions of 125I-labeled MAbЕ2Cx43 and non-specific mouse IgG among organs did not differ substantially from each other (). MAbЕ2Cx43 somewhat more intensely accumulated in the liver (0.089 ± 0.02%/g vs 0.062 ± 0.01%/g for non-specific IgG). Radioactivity accumulation in other organs, including the spleen, kidney, heart, and lung, was almost the same for both immunoglobulins. In both groups of animals, the radioactivity accumulation was the highest in the thyroid gland (6.95 ± 1.5 and 6.55 ± 0.5%/g for MAbE2Cx43 and non-specific IgG, respectively), which indicated that iodine-labeled antibodies were partly degraded, and the released 125I was taken up by thyrocytes 72 h after injection.
Table 1. The distribution of 125I-labeled monoclonal antibodies against the extracellular fragment of Cx43 among the organs of rats with experimental glioma 72 h after intravenous injection. The radioactivity evaluated in percent of the injected dose per gram of wet weight.
Radioactivity accumulation in the brain was estimated in the right hemisphere, where the glioma was located, and the left hemisphere. Neither antibodies nor non-specific immunoglobulins accumulated in the normal nervous tissue of the left hemisphere (the radioactivity was as low as 0.005% of the injected dose, which may be interpreted as the background accounted for by the traces of blood left in the vessels after perfusion) ().
The glioma bearing hemisphere exhibited accumulation of radioactivity to 0.27 ± 0.01% of the injected dose per gram of wet weight 72 h after the injection of labeled antibodies against the Cx43 fragment E2. This was more than 50-times higher than the radioactivity of the normal brain tissue and almost 5-times higher than accumulation of non-specific mouse IgG in the tumor under the same conditions. In the experiments with injection of 125I-labeled MAbE2Cx43, the specific radioactivity of the glioma bearing hemisphere exceeded the radioactivity measured in our earlier study where rats with glioma were injected with 125I-labeled monoclonal antibodies against GFAP (CitationChekhonin et al., 2009).
Specific fluorescence in cells of the glioma and peritumoral zone was detected in all experimental animals 48 h after the intravenous injection of fluorophore-conjugated antibodies, irrespective of the injected dose (). The fluorescence intensities upon the injection of 500 and 100 μg/kg of the antibodies did not differ substantially from each other; at an antibody dose of 50 μg/kg, the fluorescence intensity was considerably lower, but Cx43-positive cells were still reliably detected (Figure c. Intravenous injection of non-specific immunoglobulins conjugated with Alexa Fluor 660 did not result in visualization of cells in either glioma or the peritumoral zone ().
Figure 3. Visualization of Cx43-positive cells in the glioma upon injection of different concentrations of monoclonal antibodies against the extracellular fragment of Cx43 labeled with Alexa Fluor 660. The doses of the injected MAbE2Cx43: (a) 500 μg/kg, (b) 100 μg/kg, (c) 50 μg/kg. (d) Non-specific mouse IgG conjugated with Alexa Fluor 660 (500 μg/kg). Scale bar, 50 μm.
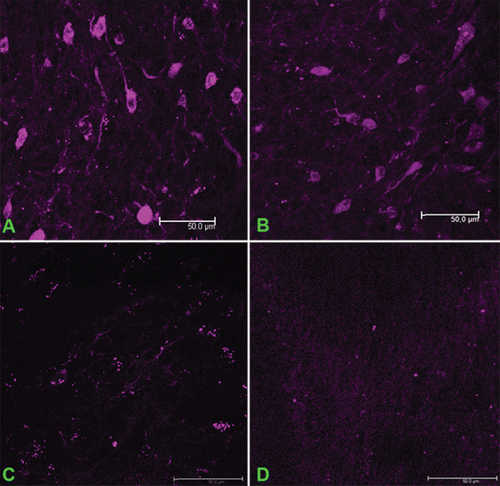
Since Cx43-positive reactive astrocytes are also targets of monoclonal antibodies against Cx43, we performed their immunofluorescent visualization using polyclonal antibodies against the specific astroglia marker GFAP () after preparing the sections of brain of the experimental rats. Fresh cryostat sections of the brain of the rats injected with monoclonal antibodies against the Cx43 fragment E2 that we used for this procedure were made after the stage of brain post-fixation with 4% neutral paraformaldehyde. This study revealed that intravenously injected antibodies against Cx43 visualized both GFAP-positive and GFAP-negative cells in the peritumoral zone.
Figure 4. Double visualization of cells of the peritumoral astroglial bank with the use of MAbE2Сх43 injected in vivo and polyclonal antibodies against GFAP ex vivo. (a) Immunofluorescence of sections obtained using anti-GFAP antibodies (green). (b) Cells intravitally visualized using MAbE2Сх43 conjugated with Alexa Fluor 660 (magenta). (c) Merged images. (d) A negative control (intravenous injection of non-specific immunoglobulins labeled with Alexa Fluor 660). Scale bar, 50 μm.
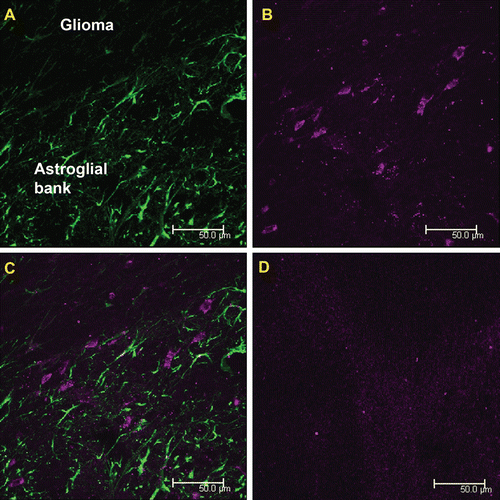
Discussion
We used two experimental approaches to studying the distribution of monoclonal antibodies against the Cx43 fragment E2 after their intravenous injection to rats with experimental glioma. To quantitatively estimate the time course of the MAbE2Cx43 content of the blood and the antibody accumulation in organs, we performed an experiment with a radiolabel (125I); to visualize the cells accumulating these antibodies, they were labeled with Alexa Fluor 660 (Invitrogen) with an emission peak in the deep-red region.
The experiment with 125I-radiolabeled monoclonal antibodies against the extracellular fragment of Cx43 demonstrated that these antibodies are specifically bound by the tumor tissue as a result of interaction with the target antigen presented on glioma cells and reactive astrocytes of the peritumoral zone where the high permeability and retention of blood–brain barrier have been shown (CitationChekhonin et al., 2007). Despite an increase in the BBB permeability for the high-molecular-weight proteins, the accumulation of MAb E2 Cx43 48 h after administration was almost 5-fold higher than the accumulation of non-specific IgGm injected under the same conditions. This fact confirms the binding specificity of anti-Cx43.
Low radioactivity in normal nervous tissue can be explained only by blood–brain barrier integrity which prevents antibodies penetration in spite of the presence of Cx43-positive cells in normal astrocytes. Low radioactivity uptake in the heart which also contains a lot of Cx43 positive cells confirms our hypothesis that our MAbE2Cx43 recognize only Cx43 in hemichannels and do not reach the antigen in functionally active dimeric connexones.
The use of MAbE2Cx43 conjugated with Alexa Fluor 660 made it possible to visualize the cells accumulating these antibodies. The additional staining of reactive astrocytes with anti-GFAP antibodies confirmed that intravenously injected antibodies against Cx43 predominantly accumulated in the peritumoral zone, where glioma cells contact the cells of the astroglial bank surrounding the tumor (). Having superposed two fluorescence channels, we found that most cells binding anti-Cx43 antibodies from the blood were GFAP-negative ().
To analyze the colocalization of intravenously injected antibodies against Cx43 and the astroglial marker GFAP, we scanned stained specimens at an excessive spatial frequency. After deconvolution, 3D data were analyzed for coincidence of the voxels of two spectral channels (Alexa Fluor 660 and Alexa Fluor 488). The thresholds of colocalized intensities were determined using the Costes–Lockett algorithm.
The results of colocalization of anti-Cx43 and anti-GFAP antibodies confirmed that part of these antibodies were bound by reactive astrocytes of the glial peritumoral bank (, yellow arrows); however, most cells visualized upon intravenous injection of MAbE2Cx43 were GFAP-negative (, blue arrows). Since these cells were located in the peritumoral bank and were spindle-shaped, they may be assumed to be Cx43-positive astroblasts that were not differentiated sufficiently for expressing GFAP.
Figure 5. Co-localization of intravenously injected MAbE2Cx43 conjugated with Alexa Fluor 660 (red) and GFAP-reactive astrocytes (green). Yellow arrows show GFAP- and Сх43-positive cells; blue arrows show GFAP-negative Cx43-positive cells. Scanning laser confocal microscopy. Scale bar, 10 μm.
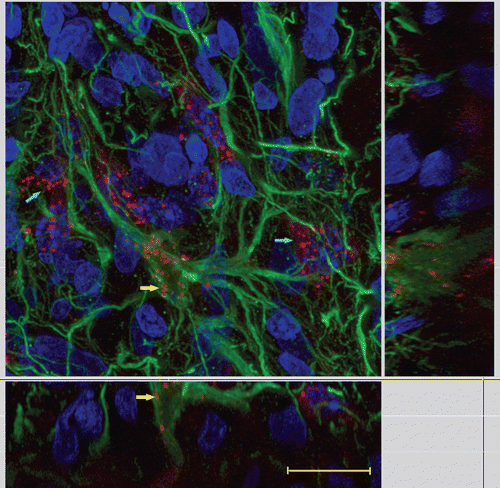
Probably, astroblasts rather than reactive astrocytes predominantly bound antibodies against the Cx43 fragment E2 because most connexons in differentiated astrocytes were in the form of dimeric gap junctions and were not recognized by antibodies against the extracellular fragment.
In vivo visualization of Cx43-positive cells in the peritumoral bank which we have obtained by means of intravenously injected antibodies against E2Cx43 corresponds to our previous data of in vitro experiments (CitationChekhonin et al., 2010). The latter experiments have clearly demonstrated a specific delivery of immunoliposomes into the Cx43-positive cells facilitated by antibodies against E2Cx43 which were used in the present study. This delivery was blocked by a pre-incubation with unmodified E2Cx43 monoclonal antibodies.
Conclusions
We have obtained monoclonal antibodies against the E2 extracellular fragment of Cx43 specifically bound by live Cx43-positive glioma cells, as well as reactive astrocytes and astroblasts of the peritumoral zone in in vivo experiments.
Targeted transport to the peritumoral zone with the use of anti-Cx43 antibodies has several obvious implications. First, it is important to accurately determine the boundaries of a tumor, including all areas of perivasal and perineural invasion, e.g. in the case of surgical resection. Since reactive astrocytes and astroblasts accompany all these areas (CitationChekhonin et al., 2009), their visualization would allow clear differentiation between the glioma invasion area and normal tissue. Second, recent studies have shown that the glioma invasion is the most active in the peritumoral zone. Tumor stem cells maintaining the pool of actively migrating glioma cells resistant to radio- and chemotherapy are located in this area (CitationDietrich et al., 2010).
The results of our in vivo study suggest that antibodies against the E2 fragment of Cx43 can be use2244d for targeted delivery of diagnostic and therapeutic drugs to the peritumoral invasion zone of high-grade gliomas.
Acknowledgements
We are grateful to R. I. Dmitriev, PhD (Group of Membrane Bioenergetic Systems, Shemyakin–Ovchinnikov Institute of Bioorganic Chemistry, Russian Academy of Sciences) for assistance in obtaining the recombinant extracellular fragment of Cx43 and Yu. S. Skoblov, Dr Sci (Chem), S. Yu. Tret’yakova, and V. I. Vakhnina (Radioisotope Block, Shemyakin–Ovchinnikov Institute of Bioorganic Chemistry, Russian Academy of Sciences) for their invaluable help in experiments with radioactive isotopes.
Declaration of interest
The authors report no conflicts of interest. The authors alone are responsible for the content and writing of the paper.
References
- Baklaushev, V.P., Gurina, O.I., Yusubalieva, G.M., Grinenko, N.F., Tsitrin, E.B., et al. (2009). Immunofluorescent analysis of connexin-43 using monoclonal antibodies to its extracellular domain. Bull Exp Biol Med. 148:725–30.
- Bates, D.C., Sin, W.C., Aftab, Q., Naus, C.C. (2007). Connexin43 enhances glioma invasion by a mechanism involving the carboxy terminus. Glia. 55:1554–64.
- Chekhonin, V.P., Baklaushev, V.P., Gurina, O.I., Grinenko, N.F., Yusubalieva, G.M., et al. (2010). A binary immunoliposomal system directed to Cx43-positive glioma cells. Drug Delivery Tech. 10:42–7.
- Chekhonin, V.P., Baklaushev, V.P., Yusubalieva, G.M., Gurina, O.I. (2009). Targeted transport of (125)I-labeled antibody to GFAP and AMVB1 in an experimental rat model of C6 glioma. J Neuroimmune Pharmacol. 4:28–34.
- Chekhonin, V.P., Baklaushev, V.P., Yusubalieva, G.M., Pavlov, K.A., Ukhova, O.V., et al. (2007). Modeling and immunohistochemical analysis of C6 glioma in vivo. Bull Exp Biol Med. 143:501–9.
- Clair, C., Combettes, L., Pierre, F., Sansonetti, P., Tran Van Nhieu, G. (2008). Extracellular-loop peptide antibodies reveal a predominant hemichannel organization of connexins in polarized intestinal cells. Exp Cell Res. 314:1250–65.
- Decrock, E., De Vuyst, E., Vinken, M., Van Moorhem, M., Vranckx, K., et al. (2009). Connexin 43 hemichannels contribute to the propagation of apoptotic cell death in a rat C6 glioma cell model. Cell Death Differ. 16:151–63.
- Dietrich, J., Diamond, E.L., Kesari, S. (2010). Glioma stem cell signaling: therapeutic opportunities and challenges. Expert Rev Anticancer Ther. 10:709–22.
- Errede, M., Benagiano, V., Girolamo, F., Flace, P., Bertossi, M., et al. (2002). Differential expression of connexin43 in foetal, adult and tumour-associated human brain endothelial cells. Histochem J. 34:265–71.
- Giardina, S.F., Mikami, M., Goubaeva, F., Yang, J. (2007). Connexin 43 confers resistance to hydrogen peroxide-mediated apoptosis. Biochem Biophys Res Commun. 362:747–52.
- Lin, J.H., Takano, T., Cotrina, M.L., Arcuino, G., Kang, J., et al. (2002). Connexin 43 enhances the adhesivity and mediates the invasion of malignant glioma cells. J Neurosci. 22:4302–11.
- Oliveira, R., Christov, C., Guillamo, J.S., DeBoüard, S., Palfi, S., et al. (2005). Contribution of gap junctional communication between tumor cells and astroglia to the invasion of the brain parenchyma by human glioblastomas. BMC Cell Biology. 6:7.
- Prochnow, N., Dermietzel, R. (2008). Connexons and cell adhesion: a romantic phase. Histochem Cell Biol. 130:71–7.