Abstract
In the present study, tramadol HCl microspheres were designed in order to accomplish rapid delivery of drug to the brain. For this purpose, lower viscosity grade HPMC (E15) was chosen as mucoadhesive polymer and used at different drug/polymer ratios in the microspheres formulations. The spray-dried microspheres were evaluated with respect to the production yield, incorporation efficiency, particle size, mucoadhesive property, in vitro drug release, histopathological study, and radio imaging study in rabbits. DSC and XRD study showed molecular dispersion and conversion of the drug into amorphous form. Size and surface morphology of microspheres was analyzed by SEM and found to be spherical in shape with smooth surface. It was found that the particle size, swelling ability, and incorporation efficiency of microspheres increase with increasing drug-to-polymer ratio. Microspheres show adequate mucoadhesion and do not have any destructive effect on nasal mucosa. In vitro drug release of optimized formulation was found to be 94% after 90 min. The radio imaging study indicated localization of drug in the brain. Hence, tramadol HCl microspheres based on a HPMC E15 may be a promising nasal delivery system for CNS targeting.
Introduction
Therapy through intranasal administration has been an accepted form of treatment in the ayurvedic system of Indian medicine. Recently, many drugs have been shown to achieve better systemic bioavailability through the nasal route than by oral administration (CitationChien et al., 1989). There are an ever increasing number of research and review articles addressing various issues related to nasal drug delivery. This interest arises from the different possible advantages presented by the nasal cavity, such as: much vascularized epithelium, large surface area available for drug absorption, lower enzymatic activity compared with the gastrointestinal tract and liver, and the direct drug transport into the systemic circulation, thereby avoiding hepatic first-pass metabolism and irritation of the gastrointestinal membrane. The nasal route has received great attention as an alternative administration route to target drugs directly to the brain via the olfactory neurons, providing more opportunity for the drug to enter the central nervous system (CNS) (CitationWang et al., 2008). A number of hydrophilic and lipophilic therapeutic agents have been shown to enter the brain directly from the nasal cavity (CitationKaur & Kim, 2008). The advantages of the nasal route for systemic delivery can include rapid onset of action and the preferential drug delivery to the brain via the olfactory pathway (CitationIllum, 2000). Moreover, the nasal route is non-invasive; therefore, reduced risk of infection, ease of convenience, and self-medication result in improved patient compliance. However, along with these important advantages, the nasal route has some disadvantages also. One of the most important disadvantages is nasal mucociliary clearance that limits the time allowed for drug absorption to occur. To overcome the rapid clearance mainly two approaches have been utilized previously. Use of penetration enhancers (surfactants, bile salts, cyclodextrins, phospholipids, and fatty acids), which can promote the absorptions of poorly absorbable drugs and use of mucoadhesive systems (bioadhesive liquid formulations, microspheres, powders, and liquid gelling formulations) that decreases the mucociliary clearance of drug formulation thereby increases the contact time between the drug and the site of absorption (CitationJain et al., 2009).
Mucoadhesive drug delivery systems are those systems that utilize bioadhesion of certain polymers that become adhesive on hydration (CitationNagai & Machida, 1985). The mucus membrane lines a number of regions of the body including the gastrointestinal tract (GIT), urogenital tract, airways, ear, nose, and eye. These are strong sites for attachment of any bioadhesive system, and hence the mucoadhesive drug delivery systems include buccal, oral, vaginal, rectal, nasal, and ocular delivery systems. The nasal mucosa has far higher permeability than other mucosal surfaces including the various regions of the GIT, buccal, and vaginal cavities (CitationJain et al., 2004). Possible pathways for a drug to permeate across the nasal mucosa are passive transportation, carrier-mediated transport, transcytosis, and transport through tight junctions. It has been reported that for both liquid and powder formulations that are not mucoadhesive, the half-life of clearance is in the order of 15–20 min (CitationMahajan & Gattani, 2009).
Dry powder delivery systems such as microspheres swell in contact with nasal mucosa and form a gel-like structure which controls the rate of clearance from the nasal cavity. In the presence of microspheres, the nasal mucosa is dehydrated due to moisture uptake by the microspheres. This results in reversible shrinkage of the cells, providing a temporary physical separation of the tight (intercellular) junction which increases absorption of the drug. Hence, a formulation that would increase residence time in the nasal cavity and at the same time improve absorption of the drug would be highly beneficial in all respects (CitationJain et al., 2009).
Tramadol HCl is a synthetic opioid belonging to the amino cyclohexanol group. Chemically it is (±) cis-2-[(dimethylamino)methyl]-1-(3-methoxyphenyl) cyclohexanol hydrochloride. Tramadol HCl is a clinically effective, centrally acting analgesic. It is a weak agonist of μ-opioid receptors and inhibits re-uptake of norepinephrine and serotonin. When given intra-nasally, it may directly transport to the brain through the olfactory region due to its high lipophilicity (log p-value 2.4). It has been used in the treatment of post-surgical pain, obstetric pain, cancer pain, and chronic pain of mechanical and neurogesic origin (CitationJeevana & Sunitha, 2009).
The aim of the present study was to formulate mucoadhesive microspheres of tramadol HCl in order to avoid first pass metabolism, achieve rapid onset of action for the patients with intense pain associated with chronic diseases, and to assess nose-to-brain delivery of the radiolabeled microspheres using a gamma scintigraphic study.
Materials and methods
Materials
Tramadol HCl was received as a kind gift from Flemingo Pharmaceuticals Pvt. Ltd. (Mumbai, India). HPMC E15 was obtained as a gift sample from Colorcon Asia Limited (Goa, India). All other reagents used were of analytical grade.
Methods
Preparation of microspheres
Tramadol HCl-loaded microspheres were prepared using HPMC E15 in five different drug-to-polymer ratios (). HPMC E15 was added and dissolved in double distilled water and tramadol HCl was added to the above polymer solution. Microspheres were obtained by spraying the feed with a spray-drier (LU222 Advanced, Labultima, India) using a standard 0.7 mm nozzle. When the liquid was fed to the nozzle with a peristaltic pump, atomization occurred by the force of the compressed air, disrupting the liquid into small droplets. The droplets together with hot air were blown into a chamber where the solvent in the droplets was evaporated and discharged out through an exhaust tube. The dry product was then collected in a collection bottle. In the standard condition, the process parameters of the spray-drying technique were: Inlet temperature: 120–130°C; Outlet temperature: 80–90°C; Aspirator speed: 35–40%; and Feed pump speed: 8–10 ml/min.
Table 1. Formulation composition of mucoadhesive microspheres.
Characterization of microspheres
Production yield
The production yield of microspheres of various batches were calculated using the weight of final product after drying with respect to the initial total weight of the drug and polymer used for preparation of microspheres and percent production yields were calculated as per the formula (1) mentioned below (CitationKellaway & Ei-Hameed, 1997).
Drug loading and incorporation efficiency
Spectrophotometric methods were used for determination of drug contents. The weighed amount of microspheres were dissolved in distilled water and kept overnight. The drug content was measured spectrophotometrically (UV 1700, Shimadzu, Japan) at 272 nm for tramadol HCl. The drug loading and incorporation efficiency (%) were calculated using equations (2) and (3), respectively.
where Mactual is the actual tramadol HCl content in weighed quantity of powder of microspheres and Mtheoretical is the theoretical amount of tramadol HCl in microspheres calculated from the quantity added in the spray-drying process (CitationFu-De et al., 2003).
Particle size analysis
A microscopical image analysis technique for determination of microspheres size distribution was applied. The particle size was determined using a digital microscope (Motic DMW2-223, Motic Instruments Inc, China) equipped with a 1/399 CCD camera imaging accessory and computer controlled image analysis software (Motic images 2000, 1.3 version, China). The microspheres were dispersed on a microscope slide. A microscopical field is scanned by video camera. The images of the scanned fields are digitalized and analyzed by the software. In all measurements at least 100 particles were examined. The average particle size of the microspheres was expressed as the volume surface diameter; dvs (μm) and standard deviation σ was calculated for each batch of microspheres (CitationMartinac et al., 2005).
Swelling property
The swelling ability of the microspheres was determined by allowing them to swell to their equilibrium in nasal simulated fluid. Accurately weighed amounts of microspheres were placed on a millipore filter (NY 11 0.22 μm) using a Franz diffusion cell (11 ml) with phosphate buffer (pH 6.6) and kept for 3.5 min (CitationJuan et al., 2001).
The following formula was used for calculation of degree of swelling.
where, α = Degree of swelling; Wo = Initial weight of microspheres; and Ws = Weight of microspheres after swelling.
In vitro mucoadhesion study
Falling liquid film technique was used to determine the mucoadhesive property of HPMC E15 microspheres. A freshly cut piece, 5 cm long, of sheep nasal mucosa obtained from a local abattoir within 1 h of killing the animal was cleaned by washing with isotonic saline solution. An accurate weight of microspheres was placed on the mucosal surface, which was attached over a polyethylene plate that fixed in an angle of 45° relative to the horizontal plane and pH 6.6 phosphate buffer warmed at 37°C was peristaltically pumped at a rate of 5 mL/min over the tissue (). After 1 h, the concentration of the drug in the collected perfusate was spectrophotometrically determined. The drug amount corresponding to the microspheres amount in perfusate was calculated. The number of adhered microspheres was estimated from the difference between the applied microspheres and the flowed microspheres. The ratio of the adhered microspheres was computed as percent mucoadhesion (CitationSaraparn et al., 2006).
In vitro drug diffusion studies
An in vitro drug release test of the microspheres was performed using Franz diffusion cells with dialysis membrane in which the donor compartment contained the microspheres while the receptor compartment was filled with phosphate buffer solution of pH 6.6 that was within the pH range in the nasal cavity. The donor chamber was placed in such a way that it just touched the diffusion medium in the receptor chamber. The temperature was maintained constant at 37 ± 1°C with the help of a circulating water bath. Samples were periodically withdrawn from the receptor compartment, replaced with the same amount of fresh pre-warmed buffer solution, and assayed using a UV spectrophotometer (1700, Shimadzu, Japan) at 272 nm.
Ex vivo permeation studies
An ex vivo permeation test of the optimized batch was performed using Franz diffusion cells with sheep nasal mucosa, other process parameters were the same as used for in vitro drug release study.
Histological study
Sheep nasal mucosa obtained from a local abattoir within 1 h of killing the animal was cleaned by washing with isotonic saline solution. After applying the drug-loaded microspheres, the nasal mucosa was fixed in 10% neutral carbonate buffered formalin solution, routinely processed and embedded in paraffin. Paraffin sections (7 μm) were cut on glass slides and stained with hematoxylin and eosin (HE). Sections were examined under a light microscope, to detect any damage to the tissue during in vitro permeation by a pathologist blinded to the study (CitationMahajan & Gattani, 2009).
Scanning electron microscopy (SEM)
The morphology of microspheres was examined by scanning electron microscopy (JSM 6390®, JEOL, Japan). Samples of microspheres were dusted onto double-sided tape on an aluminum stub and coated with gold using a cold sputter coater to a thickness of 400 Å, and then images were captured using a 15 kv electron beam.
Differential scanning calorimetry (DSC)
Differential scanning calorimetry (DSC) studies of pure drug, drug-loaded microspheres, and blank microspheres were conducted using a differential scanning calorimeter (DSC 60, Shimadzu, Japan) at a heating rate of 10°C/min for HPMC E15 microspheres. The measurements were performed at a heating range of 40–300°C under nitrogen atmospheres.
X-ray diffraction study (XRD)
The X-ray diffraction (XRD) patterns of plain drug, blank microspheres, and drug-loaded microspheres were recorded on an X-ray diffractometer (Brucker AXS, D8 Advance, Germany). The samples were irradiated with monochromatized Cu Kα radiation and analyzed between 3–80° (2θ). The voltage and current used were 30 kV and 30 mA, respectively.
Stability study
Optimized batch was sealed with rubber caps and was kept under ambient temperature and moisture conditions (40°C and 75% RH) for a period of 3 months in a stability chamber (CHM-10S®, Remi Instruments, Mumbai, India). After every month, the microspheres of the stability samples were evaluated on the basis of measurement of particle size, drug content and percent mucoadhesion.
Radiolabeling of microspheres
Tramadol HCl microspheres were radiolabeled using 99mTc by direct labeling method. Accurately weighed 10 mg microspheres equivalent to 4.4 mg of tramadol HCl were suspended in the labeling medium comprising 0.5 mL of normal saline and 50 μL of stannous chloride followed by pH adjustment to 6.5 using 0.5 M sodium bicarbonate. To the resultant mixture, required volume of sterile 99mTc-pertechnetate was added with continuous stirring for ~ 10 min and separated by centrifugation. The resultant formulation obtained had 5 mCi of activity. The labeled microspheres were washed with acetone and microspheres were separated by centrifugation and dried at 30°C for 30 min.
The labeling efficiency was determined by ascending instant thin layer chromatography using silica gel coated fiberglass sheets (Gelman Sciences Inc, Ann Arbor, MI) and acetone as the mobile phase. The effects of incubation time, pH, and stannous chloride concentration on labeling were studied to achieve optimum reaction conditions. The optimized, stable radiolabeled-microspheres were used to study brain targeting in rabbits (CitationSaha, 1993; CitationKumar et al., 2008; CitationPatil et al., 2010).
Gamma scintigraphic study
In vivo gamma scintigraphic studies were performed according to the guidelines approved by the committee for the purpose of control and supervision of experiments on animals, Ministry of Social Justice and Empowerment, Government of India. The study protocol was duly approved by the Institutional Animal Ethics Committee of R.C. Patel Institute of Pharmaceutical Education & Research, Shirpur, India (Reg. No. 651/02/C/CPCSEA).
The New Zealand white strain rabbits (2.00–2.50 kg) were selected for the study. The radiolabeled 99mTc-microspheres, 10 mg (equivalent to 4.4 mg of tramadol HCl having 5 mCi activity), were administered intra-nasally using a monodose insufflator. The rabbits were held from the back in a slanted position during nasal administration of the formulation. The animals were conscious during the experiments and were held in rabbit restrainers during the scintigraphic studies and placed on the imaging platform. Imaging of whole body of rabbits was performed using Single Photon Emission Computerized Tomography (SPECT, LC 75-005, Diacam, Siemens AG; Erlanger, Germany) (CitationKumar et al., 2008; CitationPatil et al., 2010).
Results and discussion
The spray-drying method described here appeared to be a suitable and simple technique to prepare HPMC E15 microspheres loaded with tramadol HCl. It is a one-step process, easy and rapid, as it combines drying of the feed and embedding of the drug into a one-step operation. On the basis of results of particle size determination, in vitro drug diffusion study, mucoadhesive strength, and ex vivo permeation studies, formulation batch F1 was considered optimized and used for further studies including in vivo gamma scintigraphic study.
Characterization of microspheres
Production yield
Yield of production was found in the range between 35–49% () for HPMC E15 microspheres. These relatively low values may be due to loss of material in a spray-drying system by the attachment of sprayed droplets and dry powder to the wall of the drying chamber, which can be affected by the nature of the spray-dried material and spray-drying conditions (CitationWang et al., 2009).
Table 2. Characterization of tramadol HCl loaded microspheres.
Drug loading and incorporation efficiency
Incorporation efficiency was found to be very high for the spray-drying technique. It was found that with increasing the ratio of drug-to-polymer, the incorporation efficiency was also increased (), which may be attributed to the higher availability of polymer to encapsulate the drug (CitationJain et al., 2009; CitationMahajan & Gattani, 2009).
Particle size analysis
Average particle size of microspheres ranged from 12–18 μm, which indicates the narrow size distribution. Such particle size and narrow size distribution were considered to be suitable for nasal administration by insufflations (CitationIllum et al., 1987). It was also noted that increasing the drug-to-polymer ratio slightly increased the size of microspheres (CitationSaraparn et al., 2006). The results of particle size analysis are shown in .
Swelling property
All obtained microspheres rapidly swelled in pH 6.6 phosphate buffer. Swelling capacity of the microspheres was mostly determined by polymer content in the preparation. The amount of polymer greatly affects the swelling of microspheres. It has been observed that as the amount of HPMC E15 increases, swelling property also increases ().
In vitro mucoadhesion study
Mucoadhesion study was carried out to ensure the adhesion of formulation to the mucosa for a prolonged period of time at the site of absorption. The results of in vitro mucoadhesion study () showed that all the batches of microspheres had satisfactory mucoadhesive properties ranging from 70.56–91.43% and could adequately adhere on sheep nasal mucosa. The results also showed that, with increasing polymer ratio, higher mucoadhesion percentages were obtained. This could be attributed to the availability of a higher amount of polymer for interaction with mucus.
In vitro drug diffusion studies
The in vitro release study of tramadol HCl-loaded microspheres was carried out using nasal diffusion cell. The release profile of tramadol HCl from various batches of HPMC E15 microspheres at pH 6.6 phosphate buffer is shown in . The rate and extent of tramadol HCl release from microspheres significantly retarded with an increase in polymer concentration.
On the basis of results of in vitro drug diffusion studies batch F1 showed faster release of drug and thus considered it as an optimized formulation. The release pattern of the optimized formulation (F1) revealed that drug was immediately released from microspheres showing rapid onset of action. Immediate release of drug from microspheres may be attributed to the lower viscosity of HPMC E15.
Ex-vivo permeation studies
The optimized formulation (F1) was further subjected to ex vivo permeation studies using the sheep nasal mucosa. The percent drug permeated after 90 min was found to be 71.18%.
Histological study
It is necessary to examine histological changes in nasal mucosa caused by formulations, if it is to be considered for practical use. Histological studies showed control mucosa that is normal nasal mucosa stained with hematoxylin-eosin () and the effect of formulation on sheep nasal mucosa, after applying the drug-loaded microspheres (). No change in mucosal structure was seen when treated with formulation as compared to the control. Ciliated respiratory epithelium and normal goblet cell were observed. This confirms that formulation does not cause any deleterious response and adverse effect on nasal mucosa.
Scanning electron microscopy (SEM)
The optimized formulation was analyzed by SEM for studying particle shape and surface structure (). Microspheres are spherical in shape and possess a smooth surface. These obtained microspheres had no hole or rupture on the surface, which may result in slow clearance and good deposition pattern in the nasal cavity (CitationTing et al., 1992).
Differential scanning calorimetry (DSC)
DSC is a very useful technique in the investigation of the thermal properties of the microspheres, providing both qualitative and quantitative information about the physicochemical state of drug inside the microspheres. Drug may have been dispersed in crystalline or amorphous form or dissolved in the polymer matrix during formation of the microspheres. There is no detectable endotherm if the drug is present in a molecular dispersion or solid solution state in the polymeric microspheres loaded with drug (CitationVaghani et al., 2010). represents the DSC thermogram of pure drug, blank microspheres, and drug-loaded microspheres. The thermogram of tramadol HCl exhibited a sharp endothermic peak at 181.37°C corresponding to its reported melting point in literature (CitationMoffat, 1986). In the DSC thermogram of the drug-loaded microspheres peak for pure drug did not appear. This revealed that tramadol HCl was molecularly dispersed in HPMC E15.
X-ray diffraction study
X-ray diffraction patterns of pure crystalline drug, blank microspheres, and drug-loaded microspheres are presented in . Tramadol HCl X-ray diffractogram illustrates the crystalline nature of drug. Numerous distinctive sharp peaks were observed for tramadol HCl at ~ 2θ angles of 25.7, 27.5, 32.5, and 52.5°. In contrast, the blank microspheres produce a broader peak with low intensity at 10–25° that indicates the amorphous state of the polymer. The absence of crystalline peaks of tramadol HCl in drug-loaded microspheres indicates that the drug was molecularly dispersed in the polymer and conversion of crystalline form of drug into the amorphous form was achieved. These results are in agreement with results of the DSC study.
Figure 6. X-ray diffractogram of tramadol HCl (a), HPMC E15 blank microspheres (b), and tramadol HCl-loaded microspheres (c).
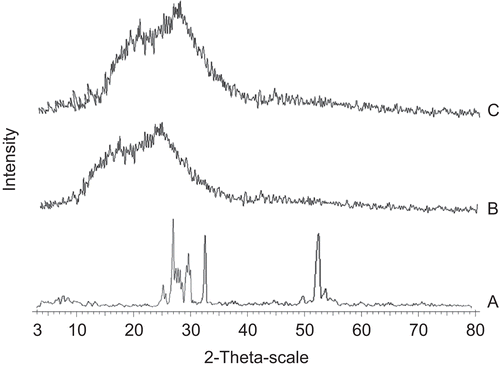
Stability study
Formulations showing lowest particle size and higher drug release were selected for stability studies. The effect on particle size and incorporation efficiency during stability study is shown in . The particle size was increased slightly from 12.42 μm to 14.6 μm during stability studies. The difference in particle size was negligible, which indicates the stability of the system for a period of 3 months under accelerated conditions. The incorporation efficiency of the optimized batch after 3 months (87.1%) indicated that the drug was retained within the microspheres throughout the stability period. The authors observed no change in mucoadhesive strength. From the stability studies of the optimized batch it was found that the microspheres remained stable, even after exposing to stress conditions of temperature and moisture.
Radiolabeling efficiency of microspheres
The formulation was effectively radiolabeled with Technetium-99m (99mTc), optimized for maximum labeling efficiency and stability. Radiochemical purity achieved was 96.23% when evaluated for reduced/hydrolyzed (R/H) 99mTc and free 99mTc. The optimal SnCl2.2H2O concentration was found to be 50 μg/mL at pH 6.5, with an incubation time of 30 min.
Gamma scintigraphic study
The scintigraphy images in rabbits following intranasal administrations are shown in . This clearly demonstrates major radioactivity accumulation in the brain following intranasal administration for an extended period. These results are in good agreement with the previous findings (CitationPatil et al., 2010).
Conclusion
In conclusion, the formulated tramadol HCl nasal mucoadhesive microspheres process is a promising approach for targeting drug to the central nervous system through the olfactory region in order to get rapid onset of action which is desired to relieve intense pain associated with chronic diseases like osteo and rheumatoid arthritis.
Acknowledgements
The authors would like to thank Mr Avins Kumar, Shri. Siddhivinayak Ganapati Cancer Hospital, Miraj, India, for assistance in carrying out scintigraphic study. The authors express their gratitude to Colorcon Asia Ltd, Goa, India and Flemingo Pharmaceuticals, Mumbai, India, for providing gift samples of HPMC E15 and Tramadol HCl, respectively. The authors also thank Dr Nilofer Naikwade, Professor & Head (Pharmacology), Appa Saheb Birnale College of Pharmacy, Sangli, India for providing animals for radio imaging study.
Declaration of Interest
The authors report no conflicts of interest. The authors alone are responsible for the content and writing of the paper.
References
- Chien, Y.W., Su, K.S.E., Chang, S.F. (1989). Nasal systemic drug delivery. New York: Dekker.
- Fu-De, C., Ming-Shi, Y., Ben-Gang, Y., Yu-Ling, F., Liang, W., Peng, Y., He, Y. (2003). Preparation of sustained-release nitrendipine microspheres with eudragit RS and aerosil using quasi- emulsion solvent diffusion method. Int J Pharm. 259:103–13.
- Illum, L. (2000). Transport of drugs from the nasal cavity to the central nervous system. Eur J Pharm Sci. 11:1–18.
- Illum, L., Jorgensen, H., Bisgard, H., Rossing, N. (1987). Bioadhesive microspheres as a potential nasal drug delivery system. Int J Pharm Sci. 39:189–99.
- Jain, S.A., Chauk, D.S., Mahajan, H.S., Tekade, A.R., Gattani, S.G. (2009). Formulation and evaluation of nasal mucoadhesive microspheres of Sumatriptan succinate. J Microencapsul. 26:711–21.
- Jain, S.K., Chourasia, M.K., Jain, A.K., Jain, R.K. (2004). Development and characterization of mucoadhesive microspheres bearing salbutamol for nasal delivery. Drug Delivery. 11:113–22.
- Jeevana, J.B., Sunitha, G. (2009). Development and evaluation of gelatin microspheres of tramadol hydrochloride. J Young Pharm. 1:24–7.
- Juan, J.T., Alfredo, G.A., Santiago, T.S., Luis, G. (2001). Spray-dried powders as nasal absorption enhancers of cyanocobalamin. Bio Pharma Bull. 24:1411–1416.
- Kaur, P., Kim, K. (2008). Pharmacokinetics and brain uptake of diazepam after intra venous and intra nasal administration in rats and rabbits. Int J Pharm. 364:27–35.
- Kellaway, I.W., Ei-Hameed, M.D. (1997). Preparation and in vitro characterization of mucoadhesive polymeric microspheres as intra-nasal delivery systems. Eur J Pharm Biopharm. 44:53–60.
- Kumar, M., Misra, A., Babbar, A.K., Mishra, A.K., Mishra, P., Pathak, K. (2008). Intranasal nanoemulsion based brain targeting drug delivery system of risperidone. Int J Pharm. 358:285–91.
- Mahajan, H.S., Gattani, S.G. (2009). Nasal administration of ondansetron using a novel microspheres delivery system. Pharm Dev Tech. 14:226–32.
- Martinac, A., Filipovic-Grcic, J., Voinovich, D., Perissutti, B., Franceschinis, E. (2005). Development and bioadhesive properties of chitosan-ethylcellulose microspheres for nasal delivery. Int J Pharm. 291:69–77.
- Moffat, A.C. (1986). Clarke’s isolation and identification of drugs: in pharmaceuticals, body fluids, and post-mortem material. 2nd ed. London: Pharmaceutical Press.
- Nagai, T., Machida, Y. (1985). Advances in drug delivery: mucosal adhesive dosage forms. Pharm Int. 6:196–200.
- Patil, S., Babbar, A., Mathur, R., Mishra, A., Sawant, K. (2010). Mucoadhesive chitosan microspheres of carvedilol for nasal administration. J Drug Targeting. 18:321–31.
- Saha, G.B. (1993). Methods of radiolabeling. In: Saha, G.B. (ed) Physics and radiobiology of nuclear medicine. New York: Springer-Verlag:100–106.
- Saraparn, H., Vimolmas, L., Narueporn, S., Garnpimol, C.R. (2006). Spray-dried mucoadhesive microspheres: preparation and transport through nasal cell monolayer. AAPS Pharm Sci Tech. 7:E79–E88
- Ting, T.Y., Gonda, I., Gipps, E.M. (1992). Microspheres of polyvinyl alcohol for nasal delivery. I. Generation by spray-drying and spray-desolvation. Pharm Res. 9:1330–5.
- Vaghani, S., Vasanti, S., Chaturvedi, K., Satish, C.S., Jivani, N.P. (2010). Stomach-specific drug delivery of 5-Fluorouracil using ethylcellulose floating microspheres. Pharm Dev Tech. 15:154–61.
- Wang, A.J., Lu, Y.P., Zhu, R.F., Li, S.T., Ma, X.L. (2009). Effect of process parameters on the performance of spray dried hydroxyapatite microspheres. Powder Tech. 191:1–6.
- Wang, X., Chi, N., Tang, X. (2008). Preparation of estradiol chitosan nanoparticles for improving nasal absorption and brain targeting. Eur J Pharm Biopharm. 70:735–40.