Abstract
In the present study an elastic liposomes-based paclitaxel formulation was developed with the objective to remove Cremophor EL. Cremophor EL is currently used for solubilizing paclitaxel in the marketed formulation and is known to produce toxic effects. Elastic liposomal paclitaxel formulation was extensively characterized in vitro, ex-vivo, and in vivo. The results obtained were compared against the marketed paclitaxel formulation. The maximum amount of paclitaxel loaded in the elastic liposomal formulation was found to be 6.0 mg/ml, which is similar to the commercial strength of marketed paclitaxel formulation. In vitro skin permeation and deposition studies showed 10.8-fold enhanced steady state transdermal flux and 15.0-fold enhanced drug deposition in comparison to drug solution. These results further confirmed with the vesicle–skin interaction study using FTIR technique. Results of the hemolytic toxicity assay indicate that elastic liposomal formulation induced only 11.2 ± 0.2% hemolysis in comparison to the commercial formulation which showed 38 ± 3.0%. Further, results of the Draize test showed no skin irritation of paclitaxel elastic liposomal formulation. Findings of the study demonstrate that elastic liposomes as a carrier is an attractive approach for localized delivery of paclitaxel.
Introduction
Cancer is a leading cause of death worldwide. There are many types of cancer, but breast cancer is the most common in women. Breast cancer develops from cells in the breast and the most common sites for the spread of breast cancer are skin and distant lymph nodes. Surgery, radiotherapy, immunotherapy and chemotherapy are the common strategies used to treat breast cancer.
The best available treatment for breast cancer is surgery, but this associates with the risk of recurrence of the disease stemming from residual malignant cells. Other options available for breast cancer therapy are administration of local radiotherapy or systemic chemotherapy. Paclitaxel has been shown to have impressive activity in both first and second line settings for breast cancer (CitationPerez, 1998). The major limitation of paclitaxel chemotherapy is its insolubility in water and several side-effects. In the current clinical parenteral dosage form of paclitaxel, drug is dissolved in a mixture of Cremophor EL (Poly-oxyethylated castor oil):ethanol (1:1) and needs to be diluted right before injection. However, Cremophor itself can be toxic and produces vasodilation, labored breathing, lethargy, hypersensitivity, cardiotoxicity, nephrotoxicity, and neurotoxicity in many patients (CitationWeiss et al., 1990). Although a pre-medication regimen with corticosteroids and anti-histamine reduces the incidence of serious hypersensitivity, milder reactions have still been reported in 5–30% of patients. Therefore, in attempts to develop Cremophor-free paclitaxel formulation, many studies have been directed towards a new formulation, such as use of hydrogels (CitationGariepy et al., 2004), polymeric micelles (CitationZhang et al., 2009), emulsions (CitationLo et al., 2009), microemulsion (CitationNornoo et al., 2008), nanoparticles (CitationDong and Feng., 2004), dendrimers (CitationOoya et al., 2003), and liposomes (CitationKoudelka et al., 2009). These approaches have the following limitations: hydrogels have poor drug loading efficiency, dendrimer has the problem of toxicity, and nanopaticles have the limitation of difficulty in preparation.
Thus, there is a need to explore an effective drug carrier for paclitaxel that not only acts as a formulation aid but also alters the biodistribution of paclitaxel in such a way so that a greater fraction of the dose reaches the target site. One strategy to achieve the high local drug concentration at tumor vicinity and also sustain the release of drug is to encapsulate paclitaxel in the deformable vesicular drug carrier, elastic liposomes. Elastic liposomes are of several orders of magnitude more deformable than the standard liposomes, and thus well suited for skin penetration (CitationCevc et al., 1997; CitationJain et al., 2003). In our previous study, we have found higher skin permeation and deposition potential of elastic liposomes-based formulation of diclofenac (CitationJain et al., 2005), rizatriptan (CitationGarg et al., 2008), colchicine (CitationSingh et al., 2009), and isotretinoin (CitationKaur et al., 2010).
Localized drug delivery is a way to deliver the drug from a dosage form to a particular site in the biological system where its entire pharmacological effect is desired. The localized delivery of chemotherapeutics has been explored to limit the indiscriminate toxicities caused to normal tissues (CitationGariepy et al., 2004). Localized delivery of paclitaxel will give sustained drug exposure to tumor cells and increase the tumor penetration of cytotoxic agents and decrease the rate of replication of tumor cells. Local administration of chemotherapeutics at the tumor site is also thought to enhance the chemo responsiveness by exposing tumors and adjacent metastases to high drug concentrations while reducing its systemic exposures. In the past polymer–lipid implants were reported for localized delivery of paclitaxel (CitationEmmanuel et al., 2005). However, this approach used the polymer as a component that sometimes leads to local toxicity and an unexpected release pattern (CitationEmmanuel et al., 2005). In the present study, an attempt was made to develop an elastic liposomes-based formulation for localized delivery of paclitaxel.
Materials and methods
Materials
Paclitaxel and soya phosphatidylcholine were received as gift samples from Dabur, India Ltd and Life Care Innovative Pvt, India Ltd. (Gurgaon, India), respectively. Chloroform, propylene glycol (PG), sodium lauryl sulfate (SLS), and methanol were purchased from S.D. Fine chemicals Ltd. (Mumbai, India). Acetonitrile was procured from Loba Chemie Pvt. Ltd. (Mumbai, India). Span 80 was procured from Himedia Ltd. (Mumbai, India). Ethanol was procured from Bangal chemicals (India). All other reagents were of AR grade.
Methods
Preparation of elastic liposomal formulations
The elastic liposomes were prepared by conventional rotary evaporation sonication method as described by CitationCevc et al. (1997). Different batches of elastic liposomes were prepared using surfactant and phospholipids in the ratio shown in . An accurately weighed amount of phospholipid, surfactant, and drug were taken in a clean, dry, round-bottom flask, and this lipid mixture was dissolved in a small quantity of chloroform. The organic solvent was removed by rotary evaporation under reduced pressure at 40 ± 1°C (Perfit, India). Final trace of solvent was removed under vacuum overnight. The deposited lipid film was hydrated with the 7% ethanolic solution in water at 60 rev/min for 1 h. The resulting vesicles were swollen for 2 h at room temperature to get large multi lamellar vesicles (LMLVs). To prepare smaller vesicles, LMLVs were probe sonicated for 10 min (Probe sonicator, VCX 505, Sonics, New Town, CT, USA). The final lipid and drug concentration in paclitaxel elastic liposomal formulation were 5% w/v and 0.6% w/v.
Table 1. Composition and characterizations of elastic liposomal formulations.
The same method was used for preparing conventional liposomal formulation (phosphatidylcholine:cholesterol, 7:3) that was used as control for comparison purposes. The final lipid concentration in liposomal formulation was also 5% w/v.
Characterization of elastic liposomal formulation
Vesicle shape was determined by transmission electron microscopy (TEM) using phosphotungstic acid (PTA) as negative stain ( Philips, TEM, Amsterdam, Netherlands). Vesicles without sonication were also visualized using an Optical Microscope (Leica, Bensheim, DMLB, Germany).
The entrapment efficiency was determined after separating unentrapped drug by dialysis for 4 h against the solution of 1% SLS in phosphate buffer saline (PBS 7.4) (CitationMura et al., 2007). Paclitaxel-loaded elastic liposomal dispersion (2 mL) was dropped into a cellulose acetate dialysis bag (Cellophane membrane, Mw cut-off 12,000–14,000, HIMEDIA, Mumbai, India) immersed in 100 mL solution of 1% SLS in phosphate buffer saline (PBS 7.4), and magnetically stirred at 30 rpm. Samples, taken at time intervals from the receiver solution, were replaced with equal volumes of fresh solvent, and drug content was analyzed by HPLC assay. The experiment was stopped when constant drug concentration values were obtained in subsequent withdrawals from the receiver phase (taking into account the progressive dilution of the medium). The dialyzed formulation was lysed using Triton-X 100 (0.1% v/v) and subsequently analyzed for drug content using HPLC assay. The percent of encapsulation efficiency (EE%) was then calculated according to the following equation:
Elastic liposomal formulation (without sonication) was diluted five times with 0.9% NaCl solution and number of vesicles per cubic mm were counted by optical microscopy using a hemocytometer (Marienfeld, Hamburg, Germany). Turbidity of elastic liposomal formulation was determined by UV-visible method as per the reported method (CitationFang et al., 1997).
For measurement of elasticity of vesicular membrane, elastic liposomal formulations were extruded at 2.5 bar through the polycarbonate filter membrane having pore diameter of 60–200 nm using a stainless steel pressure holder for 25 mm diameter filters with 200 ml capacity barrel. The amount of vesicle suspension which was extruded during 5 min was measured and vesicle shape (by TEM) and size (by Dynamic light scattering method [DLS]) were monitored before and after filtration. The elasticity of vesicle membrane was calculated by using the following formula (CitationJain et al., 2005):
where E = elasticity of vesicle membrane; J = amount of suspension, which was extruded during 5 min; rv = vesicles size (after extrusion); and rp = pore size of the barrier.
Incorporation of paclitaxel into vesicles dispersion at saturated concentration
To determine the maximum amount of paclitaxel that can be loaded in vesicles, formulations were prepared with increasing amounts of paclitaxel (1.0, 2.0, 4.0, 6.0, 8.0, and 10 mg/ml). Drug-loaded formulations were examined over a period of 14 days for the appearance of drug crystals, turbidity, and entrapment efficiency.
Skin permeation study
The in vitro skin permeation of paclitaxel from different formulations was studied using Franz glass diffusion cell maintained at 37 ± 1.0°C under non-occlusive conditions. The effective permeation area of the diffusion cell was 2.303 cm2. The receptor compartment contained 22.5 ml of 1% SLS in phosphate buffer saline (PBS 7.4) and was constantly stirred at 100 rpm. Excised albino abdomen rat skin was mounted between the donor and receptor compartment. The elastic liposomal formulation (2 ml) was applied to the epidermal sheet of excised rat skin. The samples (2 ml) were withdrawn through the sampling port of the diffusion cell at 1, 2, 3, 4, 6, 12, 18, and 24 h time intervals and analyzed by the HPLC assay. An equal volume of fresh phosphate buffer maintained at 37°C was replaced into the receptor compartment after each sampling. At the end of the permeation experiment the surface of the skin was washed five times with 50% ethanol. The skin was then cut into small pieces. The tissue was further homogenized with 50% ethanol (10 ml) and left for 24 h at room temperature. After shaking for 5 min and centrifugation for 5 min at 3000 rpm, the paclitaxel content in the supernatant was determined by HPLC assay.
Vesicle–skin interaction study
The vesicle–skin interaction of optimized elastic liposomal formulation (EL-SP3) was evaluated by FTIR (Fourier transform infrared spectroscopy). The albino rat skin was excised, and adhering fat and visceral debris were removed and washed with physiological saline solution. Epidermis was separated and treated by dipping in the drug solution acting as control, optimized elastic liposomes, and conventional liposomal formulation for 24 h. Treated skin samples were washed with water and blotted dry. The FT-IR spectrum of the skin was recorded in the range of 4000–400 cm using a FT-IR spectrophotometer.
Skin irritation potential using Draize test
The irritancy of different formulations was determined in male albino rabbits (1.9–2 kg) based on the method described by CitationDraize et al. (1944). The animals were housed in an air-conditioned room (22 ± 2.0°C) and hair of the back was trimmed short, 24 h before the beginning of the assay. Three squares were drawn on each side of the back of each rabbit. The animals were divided into six groups, each consisting of three animals. The first group did not receive any treatment and acted as a sham control, the second group (control) received topical application of the PBS (7.4), and the third group received 20% SLS solution acting as a positive control. The fourth, fifth, and sixth groups received paclitaxel solution and paclitaxel elastic liposomal and conventional liposomal formulations, respectively. At different time intervals of 0, 1, 24, 48, and 72 h after application the exposed area was scored for the erythema and edema on grade of 0–4 as described by US-FDA guidelines. All investigations were performed after approval of the Institutional Animal Ethics Committee of the Department of Pharmaceutical Sciences and Drug Research, Punjabi University, Patiala, and were in accordance with the disciplinary principles and guidelines of the Committee for the Purpose of Control and Supervision of Experiments on Animals (CPCSEA).
Transepidermal water loss measurement (TEWL)
The TEWL measurement was carried out using the same protocol as discussed above for skin irritation study. The rabbit was anaesthetized during the measurement. TEWL measurement was carried out at 0, 24, and 48 h time intervals. The TEWL was measured using a Tewameter TM 210 (Courage + Khazaka Electronic Gmbh, Koln, Germany). The probe of the Tewameter was kept perpendicular to the surface of the skin, and a stable reading of TEWL was reached in ~ 60 s. The results were expressed in g/hm2. The measurement was performed in an acclimatized room with mean relative humidity of 50.0 ± 5.0 and room temperature of 22 ± 0.5°C.
Hemolytic toxicity assay
Hemolytic toxicity was determined using red blood cell (RBC) lysis assay as per the method reported by CitationSinghai et al. (1997). Tested samples of paclitaxel commercial formulation (Intaxel, Dabur India Ltd, Ghaziabad, India) and elastic liposomal formulation were diluted with 0.9% normal saline solution in the concentration range of 6000–6 µg/ml with a dilution factor of 10×. Own blood was freshly drawn from the antecubital vein and centrifuged at 3000 rpm for 5–10 min and the supernatant was discarded. The red blood cell (RBC) suspension was diluted with saline solution to a concentration of 5% w/v. The RBC suspension (0.5 ml) was mixed with distilled water, which was considered as producing 100% hemolysis, normal saline producing no hemolysis, hence acting as blank, paclitaxel commercial formulation and paclitaxel elastic liposomal formulation in a concentration range of 6000–6 µg/ml. After incubation at 37°C for 1 h, the mixture was centrifuged at 3000 rpm for 10 min to separate non-lysed RBC. The supernatant was taken and diluted with an equal volume of PBS (pH 7.4) and absorbance was taken at 540 nm against supernatant of normal saline. The percent hemolysis was thus determined for each sample by taking absorbance of distilled water as 100% hemolytic sample using the following equation
HPLC assay
Paclitaxel was estimated by the HPLC method as reported by CitationPerdue et al. (2006). Acetonitrile:water (70:30 v/v) was used as a mobile phase and delivered at 1.0 mL/min. The injected fluid (20 µL) was eluted in a C18 column at room temperature and paclitaxel was monitored at 229 nm using a UV detector (Waters, Milford, MA). The calibration curve within a concentration range from 2–20 µg/mL was used to measure the paclitaxel concentration. The relative standard deviation around the calibration line ranged from 1.0–4.0% and the squared correlation coefficient was 0.9927.
Statistical analysis
Data are expressed as mean ± standard deviation (SD) of obtained results. Statistical analysis of the data was performed by analysis of variance (ANOVA) (Statpro, Version 2.01, San Diego, CA). The p-value < 0.05 was considered statistically significant.
Results and discussion
Preparation and in vitro characterization of elastic liposomal formulations
In the present study, paclitaxel elastic liposomal formulation was prepared in order to provide an alternate formulation suitable for its localized delivery. The composition of elastic liposomal formulations are summarized in . The major ingredients of elastic liposomes are phospholipids (vesicle forming component) and surfactant (for providing flexibility to vesicle membrane) in the optimum ratio. In this study, we used Span 80 in different concentrations (5–30% w/w of phospholipid) and optimization was carried out on the basis of encapsulation efficiency, elasticity, number of vesicle per cubic mm, and turbidity measurement study. Span 80 was selected due to its biocompatible and lipophilic nature (HLB value 4.3 and CMC 0.135 g/L). A liposomal formulation consisting of phospholipids and cholesterol (7:3) was used as control for comparison purposes (CitationGarg et al., 2008). The basic difference between liposomes and elastic liposomes is in their composition. Elastic liposome consists of phospholipids along with sublytic concentration of surfactant in comparison to phospholipids along with cholesterol in the case of liposomes.
Paclitaxel is a poorly water soluble drug and currently its commercial formulation uses 50% Cremophor EL and 50% dehydrating ethanol as solubilizing agents for its parenteral administration. Drawbacks associated with the use of Cremophor for formulating paclitaxel give a strong rational for reformulating paclitaxel using a safer and better tolerated vehicle. summarizes the results of the amount of drug loading in paclitaxel elastic liposomal formulation. The maximum amount of drug loaded in the elastic liposomal formulation was found to be 6.0 mg/ml, with drug loading efficiency of 96.2 ± 4.2% that is similar to commercial strength of paclitaxel formulation. In comparison, maximum amount of drug loaded in the conventional liposomal formulation was found to be 2.0 mg/ml. The higher drug loading with the elastic liposomal formulation is due to the lipophilic nature of paclitaxel and use of surfactant. Elastic liposomes consist of phospholipid and sub-lytic concentration of surfactant. Surfactant causes the increase in fluidity of the vesicle bilayer and is probably responsible for better retention of paclitaxel. Similarly, CitationKoudelka et al. (2009) reported the use of lysophospholipid to increase the membrane fluidity and create the bilayer pockets for increasing the encapsulation of paclitaxel.
Table 2. Optimization of the maximum amount of paclitaxel loaded in the elastic liposomal formulation.
In vitro characterization
show the transmission electron microscopy photomicrographs of elastic liposomal formulations after sonication. Elastic liposomal formulations were found to have a spherical shape and vesicular structure. shows the optical microscopy photomicrographs of elastic liposomal formulation with different concentrations of Span 80. Both visualization techniques showed that there was no change in vesicle structure for increasing the concentration from 5 to 15% w/w and further increase in concentration from 20 to 30% w/w leads to a decrease in vesicle population.
Figure 1. Transmission electron microscopy photomicrographs of elastic liposomal formulation (after sonication) at different surfactant concentration EL-SP3 (a), EL-SP4 (b), EL-SP5 (c), EL-SP6 (d). Scale bar = 100 nm.
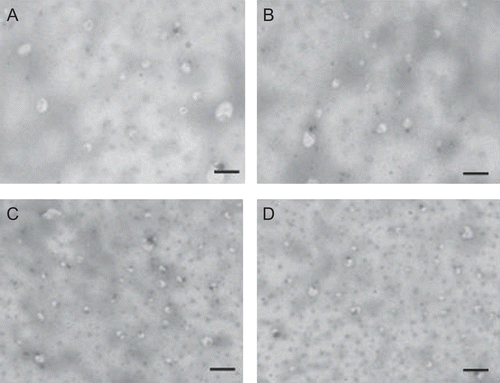
Figure 2. Optical microscopy photomicrographs of elastic liposomal formulation (without sonication) at different surfactant concentrations EL-SP1 (a), EL-SP2 (b), EL-SP3 (c), EL-SP4 (d), EL-SP5 (e), EL-SP6 (f) (Magnification 450×).
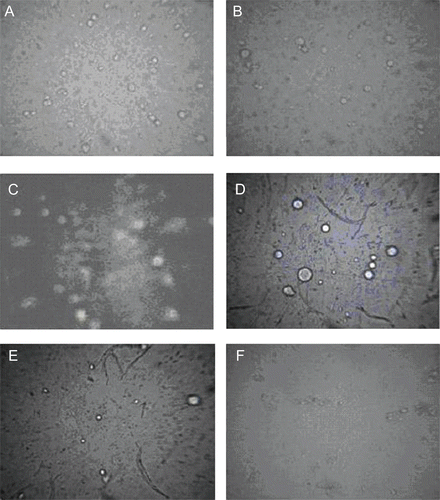
The entrapment efficiency of elastic liposomes was also found to depend on the surfactant concentration (). After a threshold level (above 15% w/w), further increase in the surfactant concentration leads to decrease in entrapment efficiency. This is possibly because at a lower concentration (up to 15% w/w) surfactant molecules get associated with the phospholipid bilayer, resulting in better partitioning of drug. Above the concentration of 15% w/w, surfactant molecules start aggregating and forming micelles in a bilayer and this will lead to depletion in the lipoidal cavity of the vesicle membrane (CitationJain et al., 2006). These mixed micelles are reported to possess the lower drug carrying capacity and poor skin permeation due to its structural features, small particle size, and absence of lipid bilayer cavity for drug encapsulation (CitationCevc et al., 1996). TEM photomicrographs at the higher concentration of surfactant showed the depletion of vesicle population and presence of dot-like mixed micelles structure ( and ). The above hypothesis was further supported by results of number of vesicles per cubic mm measurement, turbidity, and elasticity measurement study (). These studies showed the maximum value of these characteristic parameters at 15% w/w concentration of Span 80 (EL-SP3) ().
Table 3. Characterization of optimized paclitaxel elastic liposomal formulation EL-SP3 (after sonication).
Skin permeation and deposition study
Skin permeation study with the elastic liposomal formulations, control liposomes and drug solution was carried out using the abdomen rat epidermal sheet of skin. In vitro permeation studies give us valuable information about the formulation behavior in vivo. Drug release profiles were studied in triplicate and standard deviation, % cumulative release, steady state transdermal flux and enhancement ratio were calculated and summarized in .
Table 4. Skin permeation parameters for transport of paclitaxel from rat skin.
shows the results of the cumulative amount of drug permeated from different paclitaxel formulations. Elastic liposomal formulations revealed flux of paclitaxel to range between 3.5 ± 0.2–37.87 ± 2.9 (µg/cm2/h). The enhancement ratio (ER) of steady state transdermal flux for optimized elastic liposomal formulation was found to be 10.8 in comparison to drug solution prepared in Propylene glycol (PG):Ethanol (7:3) and 5-fold higher in comparison to conventional liposomal formulations. Steady state transdermal flux value was 2.6-times higher than reported by CitationPanchagnula et al. (2005). They studied the effects of chemical penetration enhancer ethanol and isopropyl myristate on skin permeation of paclitaxel. Chemical penetration enhancers have the limitation of causing the skin irritation on long-term use (CitationLashmar et al., 1989).
Figure 3. Cumulative amount of paclitaxel permeated across rat skin from different formulations. Values represented as mean ± SD (n = 3).
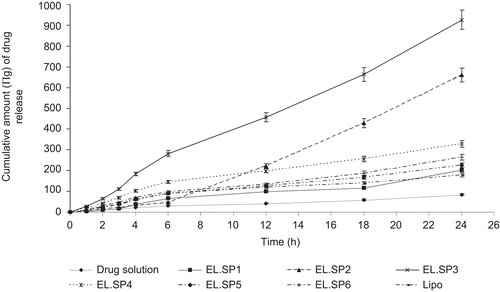
The elastic liposomes were found to have high skin permeation and deposition potential in comparison to other formulations. According to the obtained results, transdermal flux value first increases with increasing surfactant concentration to 15% w/w of lipid and then starts to decrease with the further increase in surfactant concentration. These results convinced that very low or high concentration of surfactant is not beneficial in the elastic liposomal formulation. A possible reason for lower transdermal flux value at high surfactant concentration is poor entrapment efficiency and disruption of lipid membrane, as evidenced by microscopy study ( and ).
Skin deposition study was carried out with the object to determine the depot forming ability of elastic liposomes in skin. compares the amount of drug deposited after topical application of elastic liposomal formulations, liposomes and drug solution of strength 6 mg/mL in a similar amount (2 mL). The amount of drug deposited in the skin was found to be 15-fold higher after 24 h administration of elastic liposomes as compared to drug solution. Results of skin deposition study further showed the depot forming ability of elastic liposomal formulation for localized delivery of paclitaxel. The observed better skin accumulation of elastic liposomes could be attributed to sustained release of drug from vesicular formulation. Localized delivery to tumors is a primary clinical objective in breast cancer chemotherapy. It is usually achieved by surgery or radiation. However, in most of the patients local recurrence of tumors generally occurs near the previous surgical excision site of the primary tumor. In order to minimize this, loco regional chemotherapy has been proposed by several investigators to minimize the systemic side-effects of paclitaxel (CitationNsereko & Amiji., 2002).
Vesicle skin interaction study
Results of skin permeation and deposition study indicated higher skin permeation and deposition potential of elastic liposomes in comparison to drug solution. The mechanism for better skin permeation and deposition of elastic liposomes is less known to the scientific world. To determine the effect of vesicle treatment on biophysics of skin lipids arrangement, FTIR spectroscopy of vesicle-treated skin was carried out. –d) show the FTIR spectra of skin treated with phosphate buffer saline (pH 7.4) as a control, drug solution, optimized elastic liposomes and conventional liposomal formulation. FTIR study reveals the molecular conformations of fatty acyl chains of lipids and proteins in the skin and, thus, provides an insight into the biophysical status of skin. FTIR spectra of rat skin showed characteristic peaks of asymmetric and symmetric C-H vibration obtained at 2950 and 2850 cm−1, respectively, ascribed to hydrocarbon chains of SC. The height, area, and displacement of these two peaks have been found to show the lipid perturbation and extraction of skin lipids. FTIR spectra of control skin shows the presence of two characteristic peaks at 2850 and 2950 cm−1 due to asymmetric and symmetric C-H vibration of SC lipids. The treated skin with the elastic liposomal formulation significantly broadens the area of this peak, indicating its lipid perturbation effect. It was reported that reduction in area of this peak showed the lipid extraction effect, broadening and displacement of this peak showed the lipid perturbation effect of the vehicle applied to the skin surface. Broadening of peak with the elastic liposomal formulation-treated skin is mainly due to the presence of Span 80 and phospholipids reported to have lipid perturbation effects on skin lipids (CitationGolden et al., 1986).
Hemolytic toxicity assay
Commercial paclitaxel formulation is known to cause hemolysis due to the use of a high percentage of ethanol (50% v/v) for dissolving the paclitaxel (CitationPark et al., 2008). In the present study, hemolytic toxicity of paclitaxel elastic liposomal formulation was evaluated using in vitro hemolysis assay and results were compared with the commercial formulation. Hemolytic activity has been suggested as a toxicity screen in vitro, serving as a simple and reliable measure for estimating the membrane damaged caused by formulation in vivo (CitationReed & Yalkowsky, 1985). This assay gives a quantitative measurement of hemoglobin (Hb) release caused by formulation RBC interaction. The data obtained in such assays also give a qualitative indication of damage to RBC due to cell vesicle interaction.
Results of the hemolytic toxicity assay of commercial and elastic liposomal formulations at the concentration range of 6000, 600, 60, and 6 µg/ml are summarized in . The hemolytic toxicity assay was performed in a clinically recommended concentration range. The results indicate that both formulations showed the concentration-dependent hemolysis. The paclitaxel elastic liposomes formulation induced 11.2 ± 0.2% hemolysis at the concentration of 6.0 mg/ml. In comparison, commercial formulation showed 38 ± 3% hemolysis at the same concentration. This could be related to the use of a high percentage of ethanol (50%) to dissolve paclitaxel in the commercial formulation. A high percentage of ethanol is known to cause the membrane toxicity and hemolysis (CitationChi & Wu., 1991). Similarly, CitationPark et al. (2008) found the significantly lower toxicity of paclitaxel solid dispersion in comparison to commercial Taxol® formulation. Significantly lower (p < 0.05) hemolytic activity of elastic liposome is due to its deformable nature and composition. These vesicles are made from biocompatible phospholipids as a major constituent.
Skin irritation potential using Draize test
Skin irritation is defined as a non-immunological local inflammatory reaction, and is characterized by erythema and edema, following a single or repeated application of a chemical or drug to the same cutaneous site (CitationCorsini & Galli., 1986). The local inflammatory reaction following perturbation of the epidermal barrier (either by exposure to a physical or chemical irritant) can appear within minutes to hours after the insult, and is believed to be initiated by the release of primary cytokines from keratinocytes (CitationKupper, 1990). Many chemotherapeutic agents are reported to cause cutaneous toxicity secondary to localized extravasation. Paclitaxel marketed formulations also show many toxic effects to the skin like erythema, tenderness, skin discoloration, or swelling at the injection site. More severe events such as phlebitis, cellulitis, induration, necrosis, and fibrosis were also reported (CitationBanerjee et al., 1987). Draize test is the recommended method for assessing the skin irritation caused by topically applied substances in rabbits. summarized the results of Draize test of paclitaxel formulations. SLS solution was used as a positive control due to its skin irritant nature. Paclitaxel elastic liposomal formulation was found to be non-irritant, with a mean score of 0.6 for erythema and 0 for edema. Less skin toxicity of paclitaxel elastic liposomal formulation is due to encapsulation and sustained release of drug.
Table 5. Skin irritation score as per Draize method after application of different formulations.
TEWL measurement
TEWL measurement, which is considered a good indicator of the functional status of the stratum corneum where the barrier of the skin is thought to reside, is a measure of the diffusion rate of water or water vapor across the skin. Water loss through the skin is the normal process, but whenever this barrier is altered or damage will allow the greater loss of the water vapor through the skin (CitationHaq et al., 2009). TEWL measurement is a good technique to determine the alteration in barrier nature of the skin. In the present study, it is used for describing the mechanism of skin permeation of vesicular formulation and also for determining the reversible damage to the skin. The results of TEWL measurement after 0, 24, and 48 h time intervals of different formulations are summarized in . A solution of sodium lauryl sulfate (SLS) was used as positive control and values are represented as the ratio of TEWL value pre- and post-treatment. The elastic liposomal formulation showed a 2.1-fold increase in TEWL value after 24 h of application and after 48 h the value was decreased to 1.2-fold, showing the reversible nature of SC barrier alteration. In comparison, SLS treatment showed the irreversible damage to skin barrier and TEWL loss was observed 2.1-fold after a 48 h period of time. The reason for higher TEWL value of the vesicle-treated group is skin permeation effects due to lipid perturbation and extraction of skin lipids. However, the value of TEWL is almost normal after 48 h, with the elastic liposomal formulation showing the irreversible nature of lipid perturbation.
Conclusion
The results of present study demonstrated paclitaxel elastic liposomal formulation as an attractive approach for localized delivery for treatment of breast cancer. Amount of drug deposited was found to be 15-fold higher with elastic liposomal formulation in comparison to the drug solution. Maximum amount of drug loading was also found to be 6 mg/ml, similar to the strength of commercial formulation without using Cremophor EL. Hemolytic toxicity measurement showed significantly lower hemolysis in comparison to the commercial formulation that demonstrated the protective effect of elastic liposomal formulation. Finally, it can be concluded that the vesicular carrier approach is a good alternative for localized delivery of paclitaxel.
Acknowledgements
We are grateful for President, Bio plus Life Sciences Pvt. Ltd., Hosur, India for providing facilities for FTIR study and Director, Electron Microscopy Section, All India Institute of Medical Sciences (AIIMS), New Delhi, India for providing the facilities for transmission electron microscopy.
Declaration of Interest
We are thankful to the Council of Scientific and Industrial Research (CSIR), New Delhi, India for providing the financial assistance [San no. 01(2247)/08/EMR-II].The authors report no conflicts of interest.
References
- Banerjee, A., Brotherston, T.M., Lamberty, B.G.H., Campbell, R.C. (1987). Cancer chemotherapy agent-induced perivenousextravasation injuries. Postgrad Med. 63:5–9.
- Cevc, G., Blume, G., Schatzlein, A. (1997). Transfersomes mediated Tran epidermal delivery improves the regiospecificity and biological activity of corticosteroids in vivo. J Contr Rel. 45:211–26.
- Cevc, G., Blume, G., Schatzlein, A., Gebauer, D., Paul, A. (1996). The skin: a pathway for systematic treatment with patches and lipid based agent carriers. Adv Drug Delivery Rev 18:349–78.
- Chi, L.M., Wu, W.G. (1991). Mechanism of hemolysis of red blood cell mediated by ethanol. Biochim Biophys Acta. 1062:46–50.
- Corsini, E., Galli, C.L. (1986). Cytokines and irritant contact dermatitis. Toxicol Lett. 102–103:277–82.
- Dong, Y., Feng, S.S. (2004). Methoxy poly(ethylene glycol)-poly(lactide) (MPEG-PLA) nanoparticles for controlled delivery of anticancer drugs. Biomaterials. 25:2843–9.
- Draize, J., Woodard, G., Calvery, H. (1944). Methods for the study of irritation and toxicity of substance applied to the skin and mucous membranes. J Pharmacol Exp Ther. 82:377–90.
- Emmanuel, A.H., Vessela, V., Christine, A., Micheline, P.M. (2005). In vitro and in vivo characterization of a novel biocompatible polymer-lipid implant system for the sustained delivery of paclitaxel. J Contr Rel. 104:181–91.
- Fang, J.Y., Lin, H.H., Hsu, L.R., Tsai, Y.H. (1997). Characterization and stability of various liposome-encapsulated enoxacin formulations. Chem Pharm Bull. 45:1504–9.
- Garg, T., Jain, S., Singh, H.P., Sharma, A., Tiwary, A.K. (2008). Elastic liposomal formulation for sustained delivery of anti-migraine drug: in vitro characterization and biological evaluation. Drug Dev Ind Pharm. 34:1100–10.
- Gariepy, E.R., Matthew, S., Ali, B., Mohammed, B., Garrec, D.L., Abdellatif, C., Leroux, J.C. (2004). A thermosensitive chitosan− based hydrogel for the local delivery of paclitaxel. Eur J Pharm Biopharm. 57:53–63.
- Golden, G.M., Guzek, D.B., Harris, R.R., McKie, J.E., Potts, R.O. (1986). Fourier transform raman spectroscopy. J Invest Dermatol. 86:255–9.
- Haq, M.I., Smith, E., John, D.N., Kalavala, M., Edwards, C., Anstey, A., Morrissey, A., Birchall, J.C. (2009). Clinical administration of microneedles: skin puncture, pain and sensation. Biomed Microdevices. 11:35–47.
- Jain, S., Jain, N., Bhadra, D., Jain, N.K. (2005). Transdermal delivery of an analgesic agent using elastic liposomes: preparation, characterization and performance evaluation. Curr Drug Deliv. 2:1–11.
- Jain, S., Jain, P., Umamaheshawari, R.B., Jain, N.K. (2003). Transferosomes - A novel vesicular carrier for enhanced delivery: development, characterization and performance evaluation. Drug Dev Ind Pharm. 29:1013–26.
- Jain, S., Tiwary, A.K., Jain, N.K. (2006). Sustained and targeted delivery of and anti-HIV agent using elastic liposomal formulation: mechanism of action. Curr Drug Deliv. 3:157–66.
- Kaur, N., Puri, R., Jain, S. (2010). Drug cyclodextrin-Vesicles dual carrier approach for skin targeting of anti-acne agent. APPS PharmSci Tech. 11:528–37.
- Koudelka, S., Knotigova, P.T., Masek, J., Korvasova, Z., Skrabalova, M., Plockova, J., Barthedyova, E., Turanek, J. (2009). Liposomes with high encapsulation capacity for paclitaxel: preparation, characterization and in vivo anti cancer effect. J Pharm Sci. 99:2309–19.
- Kupper, T.S. (1990). Role of epidermal cytokines. In: Oppenheim, J.J., Shevach, E.M., eds. Immunophysiology: the role of cells and cytokines in immunity and inflammation. New York: Oxford University Press, 285–305.
- Lashmar, U.T., Hadgraft, J., Thomas, N. (1989). Topical application of penetration of chemical enhancers to the skin of nude mice; a histopathological study. J Pharm Pharmacol. 41:118–22.
- Lo, J.T., Chen, B.H., Lee, T.M., Han, J., Li, J.L. (2009). Self emulsifying O/W formulations of paclitaxel prepared from mixed non ionic surfactants. J Pharm Sci. 99:2320–32.
- Mura, P., Maestrelli, F., Gonza´lez-Rodrýguez, M.L., Michelacci, I., Ghelardini, C., Rabasco, A.M. (2007). Development, characterization and in vivo evaluation of benzocaine-loaded liposomes. Eur J Pharm BioPharm. 67:86–95.
- Nornoo, A.O., Osborne, D.W., Chow, S.L. (2008). Cremophor- free intravenous microemulsions for paclitaxel I: formulation, cytotoxicity and hemolysis. Int J Pharm. 349:108–16.
- Nsereko, S., Amiji, M. (2002). Localized delivery of paclitaxel in solid tumors from biodegradable chitin microparticle formulations. Biomaterials. 23:2723–31.
- Ooya, T., Lee, J., Park, K. (2003). Effects of ethylene glycol-based graft, star-shaped, and dendritic polymers on solubilization and controlled release of paclitaxel. J Contr Rel. 93:121–7.
- Panchagnula, R., Desu, H., Jain, A., Khandavilli, S. (2005). Feasibility studies of dermal delivery of paclitaxel with binary combinations of ethanol and isopropyl myristate: role of solubility, partitioning and lipid bilayer perturbation. Farmaco. 60:894–9.
- Park, J.H., Chi, S.C., Woo, J.S. (2008). Preparation and evaluation of paclitaxel solid dispersion by supercritical anti-solvent process. J Korean Pharm Sci. 38:241–7.
- Perdue, J.D., Seaton, P.J., Tyrell, J.A., DeVido, D.R. (2006). The removal of Cremophor® EL from paclitaxel for quantitative analysis by HPLC-UV. J Pharm Biomed Anal. 41:117–23.
- Perez, E.A. (1998). Paclitaxel in breast cancer. Oncologist. 3:375–89.
- Reed, K.W., Yalkowsky, S.H. (1985). Lysis of human red blood cells in the presence of various cosolvents. J Parenter Sci Tech. 39:64–9.
- Singh, H., Utreja, P., Jain, S., Tiwary, A.K. (2009). Elastic liposomal formulation for sustained delivery of anti-gout agent: in vitro characterization and biological evaluation of anti gout activity. AAPS J. 11:54–64.
- Singhai, A.K., Jain, S., Jain, N.K. (1997). Evaluation of an aqueous injection of ketoprofen. Pharmazie. 52:2149–51.
- Weiss, R.B., Donehower, R.C., Wiernik, P.H., Ohnuma, T., Gralla, R.L., Trump, D., Baker, J.R., VanEcho, D.A., VonHoff, D.D., Leyland-jones, B. (1990). Hypersenstivity reactions from taxol. J Clin Oncol. 8:1263–8.
- Zhang, W., Junguo, H., Yuan, S., Li, Y., Juan, W., Sha, X., Fang, X. (2009). Paclitaxel-loaded pluronic P123/F127 mixed polymeric micelles: formulation, optimization and in vitro characterization. Int J Pharm. 376:176–85.