Abstract
Ricin was encapsulated in various charged liposomes having 5mol% PEG of different chain length on the surface. The cytotoxicity of ricin entrapped in these liposomal formulations was examined in human epidermoid carcinoma (KB) cells with a view to develop an optimum delivery system for ricin in vivo. It was observed that the cytotoxicity of ricin entrapped in various charged liposomes was significantly dependent on the surface charge as well as chain length of PEG. The maximum cytotoxicity of ricin was observed when it was delivered through negatively charged liposomes having 5 mol% PEG-2000 on the surface. Monensin enhances the cytotoxicity of ricin entrapped in various charged liposomes depending on the surface charge. Maximum potentiation of cytotoxicity of ricin was observed when it was delivered through negatively charged liposomes having 5 mol% PEG-2000 on the surface. Studies on the kinetics of inhibition of protein synthesis by ricin revealed that the lag period of inhibition of protein synthesis is significantly lengthened following its delivery through various charged liposomes. Monensin significantly reduced the lag period of action of ricin. It was also observed that the efficacies of monensin on the enhancement of cytotoxicity of ricin entrapped in various charged PEG-liposomes were highly related to their amount of cell association. The current study has demonstrated that by suitable adjustment of charge, density, and chain length of PEG on the surface of liposomes it would be possible to direct liposomal ricin to human tumor cells for their selective elimination in combination with monensin.
Introduction
Ricin, a heterodimeric toxic protein (consisting of A- and B-chain), is known to inhibit the synthesis of proteins in eukaryotic cells by cleaving a specific adenine residue from 28S rRNA, presumably mediated by the N-glycosidase activity of the A-chain (CitationEndo & Tsurugi, 1987). However, the potential use of ricin as an anti-cancer agent arising from its ability to arrest protein synthesis in eukaryotic cells is limited due to the non-selectivity of the B-chain. Attempts have been made to replace the binding moiety of the toxin (B-chain) by monoclonal antibodies directed against neoplastic cells (CitationThrush et al., 1996; CitationPastan & Kreitman, 1998) or by hormones eliciting specific interaction with the target cells (CitationSingh et al., 1989). While such conjugates exhibit high selectivity towards different types of cells, the toxic effect of the conjugates is considerably reduced due to sub-optimal entry of the A-chain in the cytoplasm in the absence of facilitation afforded by the B-chain (CitationOlsnes & Sandvig, 1985). The development of an alternative delivery system for ricin is, therefore, essential for exploiting its role as an anti-cancer agent.
Recently, we have shown for the first time that monensin and NH4Cl significantly enhanced the cytotoxicity of ricin entrapped in negatively charged liposomes composed of soya phosphatidylcholine, cholesterol, and phosphatidic acid (CitationBharadwaj et al., 2006). We have also reported that the enhancing potency of monensin on the cytotoxicity of liposome-entrapped ricin is highly dependent on the charge as well as the density of DSPE-mPEG-2000 on the surface of liposomes (CitationRathore & Ghosh, 2008). All these works have been carried out in Chinese Hamster Ovary (CHO) pro-cells. However, the CHO pro-cell is not a good predictive in vivo tumor model to test the efficacy of liposomal ricin. In order to determine whether liposomal ricin in combination with monensin can be used as an anti-cancer agent, in the present investigation the effect of ricin entrapped in various charged conventional and sterically stabilized liposomes in combination with monensin on the sensitivity of a human epidermoid carcinoma cell line (KB) was examined. The kinetics of inhibition of protein synthesis, the effect of incorporation of different chain length of PEG on the surface of various charged liposomes containing ricin in the aqueous compartment, in combination with monensin on the growth of this tumor cells was examined. The exocytosis, degradation, and intracellular level of ricin was also investigated.
Materials and methods
Materials
Cholesterol, L-α-phosphatidic acid (PA), Stearylamine (SA), lactoperoxidase, Monensin, 2,5-diphenyl oxazole (PPO), 1,4 bis [2-(5 phenyl oxazole)] benzene (POPOP), Guar gum, Penicillin–G (potassium salt), streptomycin sulfate, 2.5% trypsin in HBSS, and HEPES were purchased from Sigma Chemical Co. (St. Louis, MO). Soya phosphatidylcholine (SPC) was obtained as gift from Lifecare Innovations Pvt. Ltd (Haryana, India). DSPE-mPEG (2000 and 5000), (Distearoyl phosphatidylethanolamine Polyethylene glycol) was purchased from Avanti Polar Lipids, Inc. (Alabaster, AL). Fetal calf serum (FCS) was obtained from Biological Industries Ltd. (Beit-Haemek, Israel). Sepharose CL6B, Sephacryl S-300, and Sephadex G-25 were obtained from Pharmacia Fine Chemicals (Uppsala, Sweden). Powdered RPMI-1640 (deficient) medium was obtained from Sigma Chemical Co. Dulbecco’s Modified Eagle’s Medium (DMEM) purchased from Invitrogen Corporation (New York, NY). [3H] Leucine and Na 125I were bought from American Radiochemicals Inc. (St. Louis, MO).
Human epidermoid carcinoma cells
Human epidermoid carcinoma (KB) cells were procured from the National Center for Cell Science, Pune, India, and these cells were maintained in DMEM. The culture media were supplemented with 10% FCS, HEPES, NaHCO3, penicillin (100 units/ml), and streptomycin (100 µg/ml). Cells were then grown in a humidified incubator at 37°C, 5% CO2, and 95% air atmosphere.
Purification and radioiodination of ricin
Ricin was purified from the seeds of Ricinus communis by affinity chromatography on a cross-linked guar gum column following the procedure published by CitationAppukuttan et al. (1977), followed by gel permeation chromatography on Sephacryl S-300. Purified ricin was radiolabeled with Na125I by lactoperoxidase according to the method reported earlier (CitationMadan & Ghosh, 1992).
Liposome preparation
Liposomes with different compositions were prepared as described previously (CitationRathore & Ghosh, 2008). Briefly, the lipids (40 µmol total lipids) were dissolved in chloroform and evaporated to dryness at 37°C, under reduced pressure by a rotary evaporator (Millville, NJ). The thin film so formed was desiccated for 1 h, followed by hydration with 1 ml PBS (20 mM, pH 7.4), containing ricin (3 mg/ml) and trace amounts of 125I-ricin as an aqueous phase marker overnight (under N2 atmosphere to avoid lipid oxidation) at 4°C. The following day, liposomes were sonicated in a bath type sonicator (Branson, Danbury, CT) at 25°C for 5 min. The liposomal ricin was separated from free ricin by affinity chromatography on a Sepharose CL 6B column pre-equilibrated with PBS (20 mM, pH 7.4) at 25°C. Traces of free ricin were separated by ultra centrifugation at 60,000 rpm (340,000 × g) for 1 h, three times. Negatively and positively charged liposomes containing ricin were prepared exactly as described above by adding 10 mol% of either phosphatidic acid (PA) or stearylamine (SA) and sterically stabilized liposomes by adding 5 mol% of DSPE-mPEG of different chain lengths (2000 and 5000) during the preparation of lipid film.
Characterization of liposomes
The phosphatidylcholine content of the vesicles was determined using the method described by CitationStewart (1980), and the phosphatidylcholine content showed a yield of more than 60%. Liposome size and size distribution together with zeta potential were determined at room temperature in PBS pH 7.4 with a Malvern Zeatasizer Nano ZS (Malvern Instruments, Worcestershire, UK).
Assessment of cytotoxicity and cellular uptake of ricin in free and liposomal forms in KB cells in vitro
In vitro cytotoxicity was assessed by [3H] leucine incorporation in cellular protein. The cellular uptake experiments were performed at both 4°C and 37°C to study the concentration-dependent binding and internalization of 125I-Ricin in free and various liposome-entrapped forms as previously described in detail (CitationRathore & Ghosh, 2008). Briefly, the 8 × 105 cells/well/ml plated 24 h prior to the experiment were washed and pre-incubated with or without 50 nM monensin for 1 h at 37°C. For cytotoxicity study, cells were incubated with different concentrations of ricin in free and different charged liposomal form for 4 h at 37°C. For cellular uptake study, cells were incubated with varying concentrations of 125I-ricin in free and different liposomal forms at 4°C for binding and at 37°C for internalization. Following incubation, inhibition of protein synthesis was measured as a percentage of incorporation of 3H leucine into proteins by untreated cells, i.e. control, and the cell associated radioactivity was expressed as cell-associated liposomal ricin as described earlier (CitationRathore & Ghosh, 2008).
Assay of degradation and exocytosis of intracellular ricin endocytosed in free or encapsulated in different charged liposomal forms in KB cells
The 8 × 105 cells/well/ml plated 24 h prior to the experiment were washed twice with DBSS and pre-incubated with or without 50 nM monensin for 1 h at 37°C. After pre-incubation, 125I-ricin in free (0.2 μg/ml) and different charged liposomal form (4 μg/ml) were added to each well and incubated at 37°C for 2 h. After 2 h, the surface bound 125I-ricin was removed by washing the cells with 0.1 M lactose three times. A set of cells in duplicate wells was dissolved with 0.1 N NaOH to determine the amount of internalized 125I-ricin at 2 h. The cells in the remaining wells were further incubated with 1 ml fresh DMEM without toxin but containing 1 mM lactose for 2 h at 37°C. After incubation, the culture medium of each well was collected separately and the cells were solubilized with 0.5 ml of 0.1 N NaOH. The amount of 10% TCA soluble and precipitable radioactivity released in culture medium and that associated with cells was determined with respect to the control.
Kinetics of inhibition of protein synthesis by ricin in free and various charged liposomal form: Effect of monensin
The cells at the density of 8 × 105 cells/well in 1 ml DMEM were plated in 24-well plates, 24 h prior to the experiment. The monolayer cultures were washed with DBSS and incubated with FCS free medium containing ricin in free form (0.2 μg/ml) or liposomal (4 μg/ml) form with or without monensin, for various time intervals at 37°C. After different time intervals, the cells were washed twice with DBSS and incubated with leucine free medium containing [3H] leucine with or without monensin for 30 min. The inhibition of protein synthesis was measured as described earlier in the ‘Assessment of cytotoxicity and cellular uptake of ricin in free and liposomal forms in KB cells in vitro’ section.
Results
Cytotoxicity of ricin entrapped in various charged liposomes in KB cells: Effect of free monensin
shows that the cytotoxicity of ricin entrapped in all the formulations examined, i.e. positively charged, negatively charged, and neutral liposomes, significantly reduced as compared to free ricin. The extent of reduction of cytotoxicity of ricin was found to be dependent on the surface charge of the liposomes. The cytotoxicity of ricin entrapped in neutral and negatively charged liposomes was reduced to 58.45- and 48.16-fold, respectively. The reduction of the cytotoxicity of ricin entrapped in positively charged liposomes (29.48-fold) was found to be minimal as compared to the neutral and negatively charged liposomes. shows the effect of monensin on the cytotoxicity of ricin in free and entrapped in different liposomal forms in KB cells. At 50 nM concentration, monensin enhances the cytotoxicity of free ricin by 22-fold. On the other hand, maximum enhancement (58.8-fold) of cytotoxicity of ricin was observed when ricin was entrapped in negatively-charged liposomes as compared to neutral (42-fold) and positively-charged liposomes (47.6-fold).
Figure 1. Cytotoxicity of various charged liposomal ricin in KB cells: Effect of free monensin. The monolayer cultures were pre-incubated without or with (50 nM) monensin for 1 h at 37°C followed by treatment with varying concentrations of free and different charged liposomal ricin for 4 h at 37°C. The inhibition of protein synthesis was then measured by 3H-leucine incorporation into proteins, as described in ‘Materials and methods’. The ID50 of liposomal ricin are calculated as percentage of incorporation of 3H leucine into proteins by untreated cells, i.e. the control. (a) The fold reduction of the cytotoxicity of liposomal ricin was calculated by dividing ID50 of ricin in various charged liposomal form with the ID50 of ricin in free form. (b) The fold potentiation of the cytotoxicity of ricin in free and liposomal form was calculated by dividing ID50 of ricin in the absence of monensin with ID50 in the presence of monensin (50 nM). Each bar represents mean ± SD (n = 3).
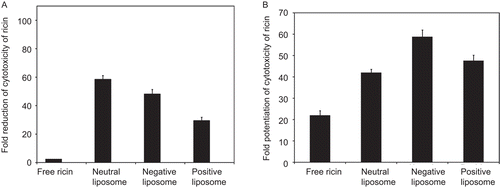
Binding and internalization of 125I-ricin in free and entrapped in various charged liposomes in KB cells
It is known that the first step in the expression of cytotoxicity of ricin involves binding of ricin to the cells surface galactose residues followed by internalization (Citationvan Deurs et al., 1985). In order to determine whether the reduction of cytotoxicity of ricin entrapped in different charged liposomes as compared to free ricin is due to the difference in binding and internalization, we examined the binding and internalization of free and different charged liposomal ricin in KB cells at 4°C as well as 37°C. shows that the binding of 125I-ricin in free form is significantly higher as compared to ricin entrapped in different charged liposomes at 4°C. At 4 h, 6.0% of free ricin is found to be associated with KB cells. About 64% of this bound ricin could be released by washing with 0.1 M lactose, indicating galactose-specific binding. On the other hand, 0.66%, 0.70%, and 1.65% of neutral, negatively-charged, and positively-charged liposomal ricin was associated with KB cells.
Table 1. Binding and internalization of 125I-ricin in free form and entrapped in different charged liposomal formulations at 4°C and 37°C in KB cells.
The specific uptake of 125I-ricin, determined by subtracting the binding at 4°C from the total internalization at 37°C, from negatively charged liposomes was found to be slightly higher than that from positively charged and neutral liposomes (). When 8 × 105 cells were incubated with 4 μg/ml 125I-ricin encapsulated in differently charged liposomes, 80.76 ng specific uptake of 125I-ricin was observed when delivered through negatively charged liposomes followed by positively charged (73.48 ng) and neutral liposomes (60.28 ng). On the other hand, when cells were incubated with 0. 2 μg/ml 125I-ricin in free form, 24.82 ng of ricin was internalized.
Degradation and exocytosis of intracellular 125I-ricin in KB cells
The present study has demonstrated that the degree of enhancement of the cytotoxicity of ricin entrapped in various charged liposomes by monensin is significantly higher as compared to free ricin and highly dependent on the charge on the surface of the liposomes. In order to ascertain whether the differential enhancing potency of monensin on the cytotoxicity of ricin in various charged liposomes as compared to free ricin is due to the variation in degradation and exocytosis of intracellular ricin endocytosed in free and various charged liposomal form, the kinetics of release of intact ricin into extracellular medium and the extent of degradation of intracellular ricin endocytosed in free form and in various charged liposomal form was examined. The effect of monensin, at a concentration that enhances the cytotoxicity of ricin in free and various liposomal forms, on the release, intracellular level, and degradation of 125I-ricin was also studied.
shows the intracellular level and the rate of release of intracellular 125I-ricin as well as the amount of released labeled materials, which is TCA soluble at 37°C after 2 h of internalization of ricin in free and entrapped in various charged liposomes. It was observed that within 2 h, 64.7% of intracellular ricin was released into the extracellular medium. On the other hand, the release of intracellular ricin delivered through various charged liposomes is significantly reduced. Only 26.4, 20.4, and 25.8% of intracellular ricin delivered through neutral, negatively, and positively charged liposomes are released in the extracellular medium. Interestingly, the minimum degradation (7.26% of total internalized ricin) and the maximum intracellular level of intact ricin (90.72% of total internalized ricin) after 2 h of internalization was observed when ricin was delivered through negatively charged liposomes as compared to neutral and positively charged liposomes.
Figure 2. Degradation and exocytosis of intracellular 125I-ricin: The monolayer cultures were pre-incubated without or with 50 nM monensin for 1 h at 37°C. Following the pre-incubation monolayers were treated with ricin in free (0.2 μg/ml) or differently charged liposomal ricin (4 μg 125I-ricin/0.25–0.50 μmol of phospholipids/ml per well) for 2 h at 37°C. After incubation cells were washed twice with lactose (0.1 M). The total cell-associated radioactivity was determined in duplicate wells, while the cells in remaining wells were further incubated with medium containing 1 mM lactose for 2 h. The total (▪), 10% TCA insoluble (□), and 10% TCA soluble (▪) radioactivity released in the medium (a) or associated with the cells (b) was determined as described in ‘Materials and methods’. The values are expressed as a percentage of total cell-associated radioactivity after 2 h pre-incubation of cell cultures with the toxin. Each bar represents mean ± SD (n = 3).
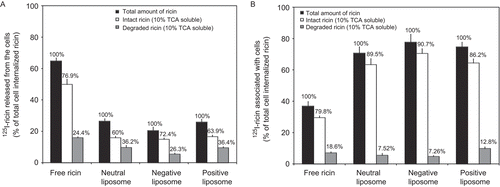
Effect of monensin on the kinetics of inhibition of protein synthesis by ricin in free and different charged liposomal form in KB cells
To examine whether monensin reduces the lag period of cell intoxication by ricin in free and liposomal form in KB cells, the kinetics of protein synthesis inhibition by ricin in free and different charged liposomal ricin in the presence and absence of monensin in KB cells was studied. shows that when the cells were treated with 0.2 μg/ml free ricin a lag period of 30 min was observed with T50 (time required to achieve 50% reduction in protein synthesis) of 90 min. It was observed that the lag period of inhibition of protein synthesis by ricin is significantly lengthened following delivery through different charged liposomes. At 4 μg/ml of various charged liposomal ricin, a lag phase of 2 h for neutral and negatively charged liposomes, on the other hand, a lag phase of 1 h for the positively charged liposomes was observed. Monensin (50 nM) reduced the lag period of free ricin from 30 min to 15 min (i.e. 2-fold reduction of lag period). However, in the presence of monensin, the lag period of neutral and negatively charged liposomal ricin was reduced from 2 h to 1 h (2-fold reduction of lag period) and 2 h to 30 min (4-fold reduction of lag period), respectively. The lag period of positive charge liposomal ricin was reduced from 1 h to 30 min (2-fold reduction of lag period).
Figure 3. Kinetics of inhibition of protein synthesis by ricin in free form and different charged liposomes in the presence and absence of monensin in KB cells: The monolayer cultures pre-incubated in serum-free DMEM medium with (-•-) or without 50 nM monensin (-▪-) for 1 h, followed by incubation with either free ricin (0.2 μg/ml) or differently charged liposomal ricin (4 μg 125I-ricin/0.25–0.50 μmol of phospholipids/ml per well), for various times. After indicated times, the cells were washed twice with DBSS and labeled with (3H) leucine for 1 h.The inhibition of protein synthesis was then measured by 3H-leucine incorporation into proteins as described in ‘Materials and methods’. All the points represent the mean ± SD (n = 3).
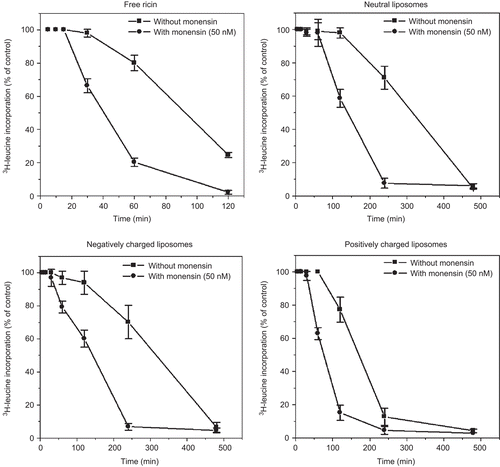
Effect of 5 mol% of DSPE-mPEG-2000 on the surface of various charged liposomes on cellular uptake and the cytotoxicity of entrapped ricin in KB cells: Effect of monensin
It has been reported that incorporation of 5 mol% PEG-PE on the surface of liposomes inhibits adsorption of liposomes to the surface of cells and subsequent endocytosis (CitationAllen et al., 1991; CitationDu et al., 1997; CitationMiller et al., 1998). Similarly we have observed that there was a reduction in the cytotoxicity and cellular uptake of liposomes when ricin was delivered through various charged liposomes following incorporation of 5 mol% of DSPE-mPEG-2000 on the surface of liposomes in KB cells (). It was observed that ID50 of liposomal ricin significantly increased from 1.83 μg/ml to 4.16 μg/ml when 5 mol% DSPE-mPEG-2000 was incorporated in positively-charged liposomes as compared to neutral (3.26 μg/ml to 3.48 μg/ml) and negatively-charged liposomes (3 μg/ml to 3.28 μg/ml). On the other hand, when the cells were treated with 8 μg/ml liposomal 125I-ricin, the binding of ricin encapsulated in conventional neutral, negatively-charged, and positively-charged liposomal forms was found to be 52.21 ng, 72.61 ng, and 126.42 ng/8 × 105 cells, respectively. These values decreased to 43.26 ng, 50.82 ng, and 40.83 ng following incorporation of 5 mol% DSPE-mPEG 2000 on the surface.
shows that monensin exhibits the enhancing potency on the cytotoxicity of ricin entrapped in various charged liposomes following incorporation of 5 mol% DSPE-mPEG-2000 on the surface in KB cells. The fold potentiation of cytotoxicity of ricin entrapped in negatively charged liposome was increased significantly from 58.8 to 88.6 following incorporation of DSPE-mPEG-2000. In contrast, the increase in fold potentiation was found to be marginal from 42 to 44.2 and from 47.6 to 50.4 when ricin was delivered through neutral and positively charged liposomes, respectively ().
Effect of incorporation of different chain lengths of DSPE-mPEG on the surface of various charged liposomes on cellular uptake and the cytotoxicity of entrapped ricin in KB cells: Effect of free monensin
It is well established that PEG can prolong the circulatory life of liposomes by avoiding the uptake by liver and spleen and the circulatory life of these liposomes is significantly dependent on the density as well as chain length of PEG on the surface of liposomes (CitationAllen et al., 1991; CitationZalipsky et al., 1994; CitationLevchenko et al., 2002). It has been reported by a number of investigators that the interaction of these liposomes with macrophages (CitationAllen et al., 1991) and cancer cells (CitationSadzuka et al., 2003; CitationBorowik et al., 2005) significantly influenced by the chain length of PEG. The influence of chain length on the biodistribution of liposomes to liver, spleen, and drainage into lymphatics from the injection site has also been demonstrated (CitationLitzinger & Huang, 1992; CitationMoghimi, 2006). Litzinger and Huang showed that liposomes with either PEG-1000-PE or PEG-2000-PE accumulated primarily in the liver, whereas those containing PEG-5000-PE were mainly taken up by the spleen with decreased liver uptake (CitationLitzinger & Huang, 1992). We have examined the effect of chain length of PEG on the cytotoxicity of ricin entrapped into various charged PEG-liposomes and the effect of monensin on the enhancement of the cytotoxicity of ricin entrapped in these liposomes. The cytotoxicity of ricin entrapped into various charged liposomes is reduced when chain length of PEG increased from 2000 to 5000. shows that the maximum increase in ID50 was observed in positively-charged liposomes (from 1.83 μg/ml to 4.16 and 6.0 μg/ml) followed by negatively-charged (from 3.0 μg/ml to 3.28 and 4.88 μg/ml) and neutral (from 3.26 μg/ml to 3.46 and 4.67 μg/ml liposomes when DSPE-mPEG of chain length 2000 and 5000 incorporated on the surface, respectively. Binding studies revealed that the amount of cell-associated various charged liposomes was attenuated following increase in the chain length of PEG from 2000 to 5000 (). The maximum reduction in binding was observed in positively-charged (from 126.4 ng to 40.8 and 25.3 ng) liposomes followed by negatively-charged (from 72.6 ng to 50.8 and 34.5 ng) and neutral (from 52.2 ng to 43.3 and 36.5 ng) liposomes when DSPE-mPEG2000 and DSPE-mPEG-5000 were incorporated on the surface of liposomes.
Figure 4. Effect of 5 mol% DSPE-mPEG-2000 on the surface of various charged liposomes on cellular uptake and the cytotoxicity of entrapped ricin in KB cells: Effect of monensin. The monolayer cultures were pre-incubated with or without 50 nM monensin for 1 h at 37°C. (a) For assessment of the cytotoxicity of liposomal ricin, the cells were incubated with different concentrations of various charged conventional (▪) and PEGylated (□) liposomal ricin for 4 h at 37°C. The inhibition of protein synthesis was then measured by 3H-leucine incorporation into proteins, as described in ‘Materials and methods’. The ID50 of liposomal ricin are calculated as a percentage of incorporation of 3H leucine into proteins by untreated cells, i.e. the control. (b) For binding study, cells were incubated with various charged conventional (▪) and PEGylated (□) liposomal ricin (8 μg 125I-ricin/0.50–0.75 μmol of phospholipids/ml/well), for 4 h at 4°C. After incubation, cells were washed three times with ice-cold DBSS. The binding of liposomal ricin is expressed as cell-associated liposomal ricin after 4 h incubation. (c) The fold potentiation of the cytotoxicity of liposomal ricin was calculated by dividing ID50 of ricin in the absence of monensin with ID50 in the presence of monensin (50 nM). Each bar represents mean ± SD (n = 3). (ζ = Zeta potential on the surface of liposomes.).
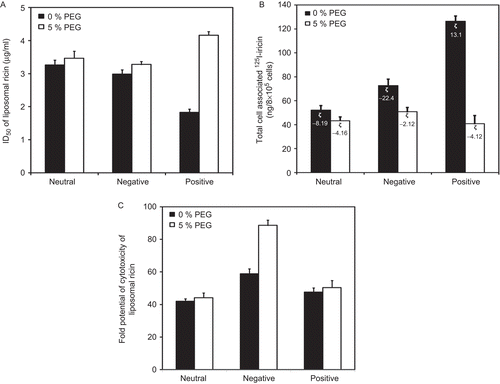
Figure 5. Effect of variation of chain lengths of PEG (5 mol%) on the surface of variously charged liposomes on cellular uptake and the cytotoxicity of entrapped ricin in KB cells: Effect of monensin. The monolayer cultures were pre-incubated with or without 50 nM monensin for 1 h at 37°C. (a) For assessment of the cytotoxicity of liposomal ricin, the cells were incubated with different concentrations of various charged conventional (▪) and PEGylated liposomal ricin having PEG-2000 (□) and PEG-5000 (▪) on the surface, for 4 h at 37°C. The inhibition of protein synthesis was then measured by 3H-leucine incorporation into proteins as described in ‘Materials and methods’. The ID50 of liposomal ricin are calculated as percentage of incorporation of 3H leucine into proteins by untreated cells, i.e. the control. (b) For binding study, cells were incubated with various charged conventional (▪) and PEGylated liposomal ricin (8 μg 125I-ricin/0.50–0.75 μmol of phospholipids/ml/well) having PEG-2000 (□) and PEG-5000 (▪) on the surface, for 4 h at 4°C. After incubation, cells were washed three times with ice-cold DBSS. The binding of liposomal ricin is expressed as cell-associated liposomal ricin after 4 h incubation. (c) The fold potentiation of the cytotoxicity of liposomal ricin was calculated by dividing ID50 of ricin in the absence of monensin with ID50 in the presence of monensin (50 nM). Each bar represents mean ± SD (n = 3). (ζ = Zeta potential on the surface of liposomes.).
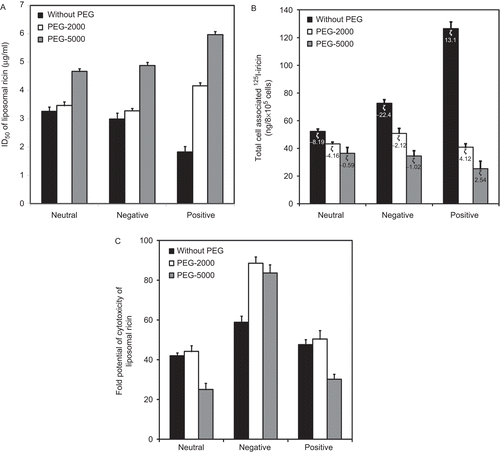
It was also found that modulation of the cytotoxicity of liposomal ricin by monensin was significantly dependent on the charge as well as chain length of DSPE-mPEG on the surface (). The maximum enhancement (from 58.8 to 88.6-fold) on the cytotoxicity of liposomal ricin was observed when DSPE-mPEG-2000 was incorporated on the surface of negatively-charged liposomes. On the other hand, the enhancement of the cytotoxicity of liposomal ricin was found to be marginal following incorporation of DSPE-mPEG 2000 on the surface of neutral (from 42.0 to 44.2-fold) and positively-charged (47.6 to 50.4-fold) liposomes. The potentiation of the cytotoxicity of liposomal ricin by monensin was significantly reduced when DSPE-mPEG-5000 was incorporated on the surface of neutral (from 44.2 to 25.0-fold) and positively-charged (50.4 to 30.2-fold) liposomes, while a very marginal reduction (88.6 to 83.7-fold) in the potentiation was observed in negatively-charged liposomes.
Discussion
The results presented in the current studies clearly showed that cytotoxicity of ricin entrapped in all the formulations, i.e. neutral, negatively and positively-charged liposomes, are significantly reduced depending on the charge on the surface in KB cells. These results support our earlier observations (CitationBharadwaj et al., 2006; CitationRathore & Ghosh, 2008) as well as by others (CitationDimitriadis & Butters, 1979) that there is a marked reduction in the cytotoxicity of ricin following its encapsulation in liposomes.
It was observed that there is a significant reduction in binding and uptake of ricin following its encapsulation into various charged liposomes in KB cells. Inhibition studies with lactose revealed that free ricin enter into KB cells by galactose-mediated endocytic pathways, while liposomal ricin is taken up by an alternative route non-galactose mediated endocytic pathway. This route may be inefficient in transporting ricin across the KB cell membrane as well as release of ricin into cytosol from endocytic vesicles. This reduced binding of liposomal ricin as compared to free ricin may be a possible reason for the reduction of cytotoxicity of ricin following encapsulation into various charged liposomes.
It was also noted that ricin exhibits maximum cytotoxicity in KB cells when delivered through positively-charged liposomes as compared to neutral and negatively-charged liposomes. On the other hand, binding and internalization studies revealed that the maximum uptake of ricin into these cells was observed when it was delivered through negatively-charged liposomes. The reason for this contradictory result is not very clear. However, these results are in agreement with earlier report by CitationLee et al. (1992) that CV 1 cells preferentially endocytosed negatively-charged liposomes. Similarly, we have shown that CHO pro-cells endocytosed positively-charged liposomes to a greater extent than neutral and negatively-charged liposomes; on the contrary, ricin exhibits maximum cytotoxicity in these cells when delivered through negatively-charged liposomes (CitationRathore & Ghosh, 2008). It has been reported that negatively- and positively-charged liposomes are endocytosed into the cytosol of mammalian cells, binding through different cell surface receptors (CitationFraley et al., 1981). It has also been reported that the intracellular fate of different receptor-ligands while in the endosome is highly dependent on the receptor-ligand system present in the endosome (CitationSteinman et al., 1983). Therefore, it is reasonable to speculate that the intracellular routing of different charged liposomes is different and that the release of ricin encapsulated in liposomes from the endosome into cytosol is not only dependent on the charge on the surface of liposomes, but also on the cell types.
It was observed that monensin at 50 nM concentration significantly enhanced the cytotoxicity of ricin entrapped in various charged liposomes in KB cells, suggesting that transport of ricin entrapped in all the formulations of liposomes from endosome to Golgi apparatus is essential for its release into the cytosol and optimal expression of its cytotoxicity. This result is in agreement with our earlier report (CitationRathore & Ghosh, 2008). However, the extent of enhancement of cytotoxicity of ricin entrapped in various charged liposomes by monensin is found to be highly dependent on the charge on the surface of liposomes and significantly higher as compared to free ricin. It appears, therefore, that the rate of transport of ricin entrapped in various charged liposomes to Golgi apparatus in the presence of monensin is different. Consequently, there is a significant variation in the enhancement of the cytotoxicity of ricin entrapped in various charged liposomes by monensin. Further, CitationStraubinger et al. (1983) have reported the presence of negatively-charged liposomes in vesicles in the trans-Golgi region after endocytosis.
The reduced rate of exocytosis, degradation, and higher cell-associated ricin can account for higher intracellular level and greater extent of enhancement of cytotoxicity of ricin by monensin when delivered through negatively-charged liposomes. The observed higher intracellular level of ricin when delivered through various charged liposomes for a longer period of time as compared to free ricin may allow more efficient ricin-A-chain release from liposomal ricin located in an intracellular compartment into the cytosol under the influence of monensin. This may explain why monensin brings about a higher degree of enhancement of cytotoxicity of ricin when delivered through various charged liposomes as compared to free ricin. The mechanism of differential release of intracellular 125I-ricin into extracellular medium and into cytosol internalized through various charged liposomes as compared to free ricin is not obvious. However, it is known that different types of molecules are endocytosed by cells and enter through a coated pit/coated vesicle pathway, the fate of endocytosed molecules can vary from rapid recycling to the cell surface to progression through a variety of intracellular compartments depending on the receptor-ligand system present in the endosome (CitationBrown & Goldstein, 1979; CitationSteinman et al., 1983; CitationMellman et al., 1986). Further, Citationvan Deurs et al. (1986) have reported that routing and sorting events at the level of the endosomal system are influenced by the valency of the ligands or ligand conjugates. They have shown that native ricin and ricin-HRP monoconjugates after endocytosis reached vesicular and tubular vesicular elements as well as Golgi apparatus; on the other hand, ricin colloidal gold conjugates which is polyvalent (2–4 ricin per gold particle) reached vesicular and tubular elements only but did not reach the Golgi elements. However, they have not studied the effect of monensin on the cytotoxicity of polyvalent ricin-colloidal gold conjugates.
However, no work has been done to determine the amount of time spent by various charged liposomes in these vesicles and amount of liposomal markers transported to TGN depending on the charge of the liposomes. Therefore, it is reasonable to speculate that the time spent by various charged liposomes in these vesicles may be different. Consequently, the extent of intracellular 125I-ricin present in endocytic vesicles encapsulated in various charged liposomes, transported to TGN, and released into extracellular medium may be different. As a result, the intracellular level of ricin delivered through various liposomes will vary. It appears, therefore, that rate of variation in the transport of ricin into TGN from various charged liposomal forms present in the intracellular compartment is the limiting step in the toxicity difference between ricin encapsulated in various charged liposomes and free ricin.
The results based on the kinetics of inhibition of protein synthesis suggest that the rate of release of ricin-A chain from ricin encapsulated in various charged liposomes located in an intracellular compartment into cytosol are different. Monensin (50 nM) induced significant but differential reduction (2-4 fold) of the lag period of action of ricin when delivered through various charged liposomes in these cells. The mechanism of differential reduction of the lag phase of inhibition of protein synthesis by ricin encapsulated in various charged liposomes by monensin is not very clear. However, it is known that the monensin-induced enhancement of cytotoxicity of ricin is due to enhanced transport of ricin from the cell surface to Golgi apparatus (CitationWesche et al., 1999) and decreased transfer of ricin to the lysosomes (CitationCarriere et al., 1985; CitationManske et al., 1989). Therefore, it is reasonable to speculate that monensin reduces the lag period of various liposomal ricin actions by enhancing the transport of ricin from the intracellular compartment to TGN and decreasing transfer of liposomal ricin to the lysosomes. This rate of transport of ricin encapsulated in various charged liposomes to TGN and lysosomes is dependent on the surface charge of the liposomes, leading to a variation in the reduction in lag phase of ricin action.
A significant reduction in the lag period of various charged liposomal ricin action by monensin suggests a more rapid and efficient release of ricin from the intracellular compartment into the cytosol. This result is consistent with our earlier observation (CitationGhosh et al., 1985; CitationGhosh & Wu, 1988; CitationMadan & Ghosh, 1992), and those reported by other investigators (CitationCasellas et al., 1984; CitationRaso & Lawrence, 1984; CitationCarriere et al., 1985; CitationRamakrishnan et al., 1989) that pre-treatment of cells with carboxylic ionophore results in a reduction of lag period in the inhibition of protein synthesis by ricin and immunotoxins.
We observed that there was a reduction in the cytotoxicity of ricin entrapped into various charged liposomes following incorporation of 5 mol% of DSPE-mPEG-2000 on the surface of liposomes in KB cells. However, monensin exhibits differential enhancing potency on the cytotoxicity of ricin entrapped into various charged PEG liposomes depending on the charge on the surface in KB cells (). Binding studies revealed that the overall amount of cell-associated various charged PEG-liposomes examined was attenuated relative to the conventional liposomes. However, the extent of attenuation of cell-associated liposomes was greatest for positively (67.7%) and negatively (30.0%) charged liposomes; on the other hand, only 17.14% neutral PEG-liposomes binding was attenuated. The mechanism of differential action of monensin is not very clear. However, it is known that different charged liposomes endocytosed by binding to different sites on the cell surface (CitationFraley et al., 1981). It is also known that monensin act at various steps of intracellular pathways and some steps are promoted by monensin while other pathways are inhibited (CitationMarnell et al., 1982; CitationSandvig et al., 1984; CitationMadan & Ghosh, 1992). Therefore, it is reasonable to speculate that the intracellular routing of different charged PEG-liposomes is different and monensin exhibit differential activity on the various intracellular routes, consequently the mechanism of release of ricin encapsulated in various charged PEG-liposomes from the intracellular vesicles into cytosol is different. In the literature we found that very few investigators have carried out the interaction of PEG-liposomes with human cancer cells in vitro. For example, CitationMiller et al. (1998) have reported that the positively-charged conventional as well as PEG-liposomes interact with and endocytosed more in HeLa cells, a human ovarian carcinoma cell as compared to neutral and negatively-charged liposomes (CitationMiller et al., 1998). On the other hand, CitationSadzuka et al. (2003) have reported that modification of liposome (neutral) surface with PEG-lipid did not prevent liposome uptake into the Ehrlich ascites carcinoma cells, rather PEGylation promoted the uptake of liposome in these cells. However, this group has not examined the effect of various charged liposomes on the uptake of PEG-liposomes in the carcinoma cells. CitationBorowik et al. (2005) have reported that the interaction of liposomes with colon cancer cells is highly dependent on the surface charge as well as the density and chain length of the PEG (CitationBorowik et al., 2005). They have suggested that the association of liposomes with target cells can be tuned by proper adjustment of surface electrostatics and the density and chain length of PEG. These investigators have not examined the efficacy of any biologically active molecules delivered through these liposomes in cancer cells. Our results clearly showed that biological efficacy of a liposomes entrapped molecule are not only dependent on the amount of cell association of the liposomes but also on the intracellular route followed by these liposomes. All these studies together showed that there is a lot of controversy regarding the interaction of various PEG-liposomes with carcinoma cells and need further work to resolve the issues.
We also found that the length of the PEG chain have influence on the cytotoxicity of entrapped ricin as well as the efficacy of monensin on the enhancement of the cytotoxicity of ricin. These results clearly indicate that there is a direct correlation between the cytotoxicity of ricin entrapped into various charged liposomes having PEG chain length 2000 and 5000 on their surface with their amount of cell association. However, there is a significant variation in the enhancing potency of monensin on the cytotoxicity of ricin entrapped in various charged liposomes when chain length of PEG increased from 2000 to 5000. These results clearly showed that the enhancement of the cytotoxicity of ricin entrapped into various charged PEG-liposomes by monensin is not only dependent on the chain length of PEG alone but also depensd on the charge on the surface of the liposomes. This may be attributed to their differential intracellular routing following entry into mammalian cells by endocytosis and differential effect of monensin in various intracellular pathways. These results are in agreement with the report by CitationSadzuka et al. (2003) that the cell uptake of PEG-liposomes not only depends on the chain length of the PEG but also on the lipid anchor of PEG-lipid. Similarly, CitationLevchenko et al. (2002) have also reported that blood clearance of PEG-liposomes in mice is not only dependent on the chain length of PEG but also depends on the chemical nature of the charged lipids incorporated on the surface of liposomes.
This report is the first of its kind to show the effect of surface charge and chain length of PEG on the interaction of liposomes carrying biologically active molecules with tumor cells. These results clearly showed that biological efficacy of a liposomes entrapped molecule are not only dependent on the amount of cell association of the liposomes but also on the intracellular route followed by these liposomes. The present study clearly showed that the delivery of drug can be optimized by manipulating the surface charge, PEG density, and chain length of PEG on the surface of liposome to deliver the biologically active molecule to tumor cells.
Declaration of Interest
This study was supported in part by a grant from the Indian Council of Medical Research (ICMR), and Special Assistance Programme (SAP) from University Grant Commission, Government of India. Nikhil Tyagi was supported by Indian Council of Medical Research (ICMR), Government of India. The authors report no conflict of interest.
References
- Allen, T.M., Austin, G.A., Chonn, A., Lin, L., Lee, K.C. (1991). Uptake of liposomes by cultured mouse bone marrow macrophages: influence of liposome composition and size. Biochim Biophys Acta. 1061:56–64.
- Appukuttan, P.S., Surolia, A., Bachawat, B.K. (1977). Isolation of two galactose-binding proteins from Ricinus communis by affinity chromatography. Indian J Biochem Biophys. 14:382–4.
- Bharadwaj, S., Rathore, S.S., Ghosh, P.C. (2006). Enhancement of the cytotoxicity of liposomal ricin by the carboxylic ionophore monensin and the lysosomotropic amine NH4Cl in Chinese hamster ovary cells. Int J Toxicol. 25:349–59.
- Borowik, T., Widerak, K., Ugorski, M., Langner, M. (2005). Combined effect of surface electrostatic charge and poly(ethyl glycol) on the association of liposomes with colon carcinoma cells. J Liposome Res. 15:199–213.
- Brown, M.S., Goldstein, J.L. (1979). Receptor-mediated endocytosis: insights from the lipoprotein receptor system. Proc Natl Acad Sci USA. 76:3330–7.
- Carriere, D., Casellas, P., Richer, G., Gros, P., Jansen, F.K. (1985). Endocytosis of an antibody ricin A-chain conjugate (immuno-A-toxin) adsorbed on colloidal gold. Effects of ammonium chloride and monensin. Exp Cell Res. 156:327–40.
- Casellas, P., Bourrie, B.J., Gros, P., Jansen, F.K. (1984). Kinetics of cytotoxicity induced by immunotoxins. Enhancement by lysosomotropic amines and carboxylic ionophores. J Biol Chem. 259:9359–64.
- Dimitriadis, G.J., Butters, T.D. (1979). Liposome-mediated ricin toxicity in ricin-resistant cells. FEBS Lett. 98:33–6.
- Du, H., Chandaroy, P., Hui, S.W. (1997). Grafted poly-(ethylene glycol) on lipid surfaces inhibits protein adsorption and cell adhesion. Biochim Biophys Acta. 1326:236–48.
- Endo, Y., Tsurugi, K. (1987). RNA N-glycosidase activity of ricin A-chain. Mechanism of action of the toxic lectin ricin on eukaryotic ribosomes. J Biol Chem. 262:8128–30.
- Fraley, R., Straubinger, R.M., Rule, G., Springer, E.L., Papahadjopoulos, D. (1981). Liposome-mediated delivery of deoxyribonucleic acid to cells: enhanced efficiency of delivery related to lipid composition and incubation conditions. Biochemistry. 20:6978–87.
- Ghosh, P.C., Wellner, R.B., Cragoe, E.J., Wu, H.C. (1985). Enhancement of ricin cytotoxicity in Chinese hamster ovary cells by depletion of intracellular K+: evidence for an Na+/H+ exchange system in Chinese hamster ovary cells. J Cell Biol. 101:350–7.
- Ghosh, P.C., Wu, H.C. (1988). Enhancement of cytotoxicity of modeccin by nigericin in modeccin-resistant mutant cell lines. Exp Cell Res. 174:397–410.
- Lee, K.D., Hong, K., Papahadjopoulos, D. (1992). Recognition of liposomes by cells: in vitro binding and endocytosis mediated by specific lipid headgroups and surface charge density. Biochim Biophys Acta. 1103:185–97.
- Levchenko, T.S., Rammohan, R., Lukyanov, A.N., Whiteman, K.R., Torchilin, V.P. (2002). Liposome clearance in mice: the effect of a separate and combined presence of surface charge and polymer coating. Int J Pharm. 240:95–102.
- Litzinger, D.C., Huang, L. (1992). Amphipathic poly(ethylene glycol) 5000-stabilized dioleoylphosphatidylethanolamine liposomes accumulate in spleen. Biochim Biophys Acta. 1127:249–54.
- Madan, S., Ghosh, P.C. (1992). Monensin intercalation in liposomes: effect on cytotoxicities of ricin, Pseudomonas exotoxin A and diphtheria toxin in CHO cells. Biochim Biophys Acta.1110:37–44.
- Manske, J.M., Buchsbaum, D.J., Vallera, D.A. (1989). The role of ricin B chain in the intracellular trafficking of anti-CD5 immunotoxins. J Immunol. 142:1755–66.
- Marnell, M.H., Stookey, M., Draper, R.K. (1982). Monensin blocks the transport of diphtheria toxin to the cell cytoplasm. J Cell Biol. 93:57–62.
- Mellman, I., Fuchs, R., Helenius, A. (1986). Acidification of the endocytic and exocytic pathways. Annu Rev Biochem. 55:663–700.
- Miller, C.R., Bondurant, B., McLean, S.D., McGovern, K.A., O’Brien, D.F. (1998). Liposome-cell interactions in vitro: effect of liposome surface charge on the binding and endocytosis of conventional and sterically stabilized liposomes. Biochemistry. 37:12875–83.
- Moghimi, S.M. (2006). The effect of methoxy-PEG chain length and molecular architecture on lymph node targeting of immuno-PEG liposomes. Biomaterials. 27:136–44.
- Olsne, S., Sandvig, K. (1985). Entry of polypeptide toxins into animal cells. In: Endocytosis, ed. I. Pastan and M. C. Willingham, Plenum press, New York, pp. 195–234.
- Pastan, I.I., Kreitman, R.J. (1998). Immunotoxins for targeted cancer therapy. Adv Drug Deliv Rev. 31:53–88.
- Ramakrishnan, S., Bjorn, M.J., Houston, L.L. (1989). Recombinant ricin A chain conjugated to monoclonal antibodies: improved tumor cell inhibition in the presence of lysosomotropic compounds. Cancer Res. 49:613–7.
- Raso, V., Lawrence, J. (1984). Carboxylic ionophores enhance the cytotoxic potency of ligand- and antibody-delivered ricin A chain. J Exp Med. 160:1234–40.
- Rathore, S.S., Ghosh, P.C. (2008). Effect of surface charge and density of distearylphosphatidylethanolamine-mPEG-2000 (DSPE-mPEG-2000) on the cytotoxicity of liposome-entrapped ricin: effect of lysosomotropic agents. Int J Pharm. 350:79–94.
- Sadzuka, Y., Kishi, K., Hirota, S., Sonobe, T. (2003). Effect of polyethyleneglycol (PEG) chain on cell uptake of PEG-modified liposomes. J Liposome Res. 13:157–72.
- Sandvig, K., Sundan, A., Olsnes, S. (1984). Evidence that diphtheria toxin and modeccin enter the cytosol from different vesicular compartments. J Cell Biol. 98:963–70.
- Singh, V., Sairam, M.R., Bhargavi, G.N., Akhras, R.G. (1989). Hormonotoxins. Preparation and characterization of ovine luteinizing hormone-gelonin conjugate. J Biol Chem. 264:3089–95.
- Steinman, R.M., Mellman, I.S., Muller, W.A., Cohn, Z.A. (1983). Endocytosis and the recycling of plasma membrane. J Cell Biol. 96:1–27.
- Stewart, J.C. (1980). Colorimetric determination of phospholipids with ammonium ferrothiocyanate. Anal Biochem. 104:10–14.
- Straubinger, R.M., Hong, K., Friend, D.S., Papahadjopoulos, D. (1983). Endocytosis of liposomes and intracellular fate of encapsulated molecules: encounter with a low pH compartment after internalization in coated vesicles. Cell. 32:1069–79.
- Thrush, G.R., Lark, L.R., Clinchy, B.C., Vitetta, E.S. (1996). Immunotoxins: an update. Annu Rev Immunol. 14:49–71.
- van Deurs, B., Pedersen, L.R., Sundan, A., Olsnes, S., Sandvig, K. (1985). Receptor-mediated endocytosis of a ricin-colloidal gold conjugate in vero cells. Intracellular routing to vacuolar and tubulo-vesicular portions of the endosomal system. Exp Cell Res. 159:287–304.
- van Deurs, B., Tonnessen, T.I., Petersen, O.W., Sandvig, K., Olsnes, S. (1986). Routing of internalized ricin and ricin conjugates to the Golgi complex. J Cell Biol. 102:37–47.
- Wesche, J., Rapak, A., Olsnes, S. (1999). Dependence of ricin toxicity on translocation of the toxin A-chain from the endoplasmic reticulum to the cytosol. J Biol Chem. 274:34443–9.
- Zalipsky, S., Brandeis, E., Newman, M.S., Woodle, M.C. (1994). Long circulating, cationic liposomes containing amino-PEG-phosphatidylethanolamine. FEBS Lett. 353:71–4.