Abstract
The aim of this study was to investigate the potential of thiolated matrix tablets for gastroretentive delivery systems. Poly(acrylic acid)-cysteine (PAA-Cys) and chitosan-4-thiobuthylamidine (chitosan-TBA) were evaluated as anionic and cationic thiolated polymers and riboflavin was used as a model drug. Tablets were prepared by direct compression and each formulation was characterized in terms of disintegration, swelling, mucoadhesion, and drug release properties. Thereafter, the gastric residence times of tablets were determined with in vivo study in rats. The resulting PAA-Cys and chitosan-TBA conjugates displayed 172.80 ± 30.33 and 371.11 ± 72.74 µmol free thiol groups, respectively. Disintegration studies demonstrated the stability of thiolated tablets up to 24 h, whereas tablets prepared with unmodified PAA and chitosan disintegrated within a time period of 1 h. Mucoadhesion studies showed that mucoadhesion work of PAA-Cys and chitosan-TBA tablets were 1.341- and 2.139-times higher than unmodified ones. The mucoadhesion times of PAA, PAA-Cys, chitosan, and chitosan-TBA tablets were 1.5 ± 0.5, 21 ± 1, 1 ± 0.5, 17 ± 1 h, respectively. These results confirm the theory that thiol groups react with mucin glycoproteins and form covalent bonds to the mucus layer. Release studies indicated that a controlled release was provided with thiolated tablets up to 24 h. These promising in vitro results of thiolated tablets were proved with in vivo studies. The thiolated tablets showed a gastroretention time up to 6 h, whereas unmodified tablets completely disintegrated within 1 h in rat stomach. Consequently, the study suggests that thiolated matrix tablets might be promising formulations for gastroretentive delivery systems.
Introduction
Prolonged residence time of a delivery system on the gastric mucosa leads to an extended period of absorption and consequently to an improved bioavailability and a greater therapeutic benefit of the drug substance. For instance, drugs that are absorbed in the proximal part of the gastrointestinal tract and drugs that are less soluble or degraded in the alkaline pH may benefit from prolonged gastric retention. In addition, local and sustained drug delivery to the stomach may offer numerous advantages including improved bioavailability and therapeutic efficacy. Thus, dose reduction may become feasible (CitationTalukder & Fassihi, 2004).
Various strategies have been pursued to develop efficient formulations for gastroretentive drug delivery such as floating, swelling, and high/low density systems, and promising results were obtained (CitationBardonnet et al., 2006). Unfortunately, up-to-date gastric mucoadhesive systems have not reached their full potential despite a few notable exceptions. Until now, first generation mucoadhesive polymers (i.e. polyacrylic acid, sodium carboxymethylcellulose, polycarbophil, chitosan) were utilized for such systems, but their success was limited due to insufficient adhesion to the GI tract. Therefore, second-generation mucoadhesive polymers, called thiomers, are now receiving increased attention for developing novel approaches. Thiomers mimic the natural mechanism of secreted mucus glycoproteins which are covalently anchored in the mucus layer by disulfide bonds (CitationBernkop-Schnürch et al., 1999; CitationLeitner et al., 2003b). This leads to increased residence time and bioavailability. Disulfide bonds are also formed within the thiomers itself, leading to improved cohesive properties (CitationBernkop-Schnürch, 2005). Furthermore, the permeation enhancing effect of thiomers, especially in combination with glutathione, from rat gastric mucosa was demonstrated previously (CitationAy Senyigit et al., 2010).
The aim of this study was to prepare matrix tablets formulated with thiomers and to evaluate their potential for gastroretentive drug delivery both under in vitro and in vivo conditions. For this aim, the PAA-Cys was chosen as an anionic polymer and chitosan-TBA as a cationic polymer for comparison due to their comparatively high mucoadhesive properties and excellent cohesiveness (CitationLeitner et al., 2003a). Mucoadhesive matrix tablets were chosen as a formulation type because they seem to be useful as they are easy to produce and handle. In addition, the production costs of matrix tablets are low. On the other hand a significant problem with these formulations is the poor adherence to mucosal surfaces due to high mass of the dosage form combined with the vigorous movement of the stomach (CitationAndrews et al., 2009). In order to improve their efficacy, mucoadhesive properties were intended to be increased by using thiolated polymers and a longer residence time with a controlled drug release was aimed at.
Riboflavin-5’-monophosphate sodium salt dihydrate (RF5’PNa) was chosen as a model drug because it lacks adverse effects and has no pharmacological effect on gastric motility (CitationKlausner et al., 2002). It has a narrow absorption window in the upper part of the gastrointestinal tract; therefore, gastroretentive dosage forms would be beneficial to improve its bioavailability and therapeutic efficacy (CitationKagan et al., 2006; CitationGröning et al., 2007).
Materials and methods
Materials
Riboflavin-5’-monophosphate sodium salt dihydrate (RF5’PNa) and poly(acrylic acid) (PAA) (average molecular weight 450 kDa) were purchased from Sigma-Aldrich (Germany). Chitosan (medium molecular mass: 400 kDa) was obtained from Fluka Analytical (Japan). All other chemicals were of analytical grade.
Synthesis of PAA-Cys
The poly(acrylic acid)–cysteine conjugates (PAA–Cys) used in this study were synthesized according to a method described previously (CitationBernkop-Schnürch et al., 1999). The covalent attachment of L-cysteine to PAA was achieved by formation of amide bonds between the primary amino groups of cysteine and the carboxylic acid groups of the polymer.
In brief, PAA was hydrated in demineralized water (1% w/v) and pH was adjusted to 6 with 5 M NaOH (WTW Series pH-meter, Inolab, Germany). Then, 4 mL 1-ethyl-3-(3-dimethlyaminopropyl) carbodiimide HCl (EDAC, Sigma, Germany) solution (100 mM) was added to activate the carboxylic acid moieties of the hydrated polymers. After 20 min of incubation under stirring at room temperature, 10 mL L-cysteine solution (10% w/v) (Sigma) was added and pH was readjusted to 6 with 1 M NaOH. Reaction mixtures were incubated for 3 h at room temperature under stirring. The resulting conjugates were isolated by dialyzing (Dialysis tubing cellulose, M.W.C.O.: 12 kDa) twice against 0.2 mM HCl, twice against the same medium but also containing 1% NaCl and then twice against 0.2 mM HCl in the dark at 10°C. Thereafter, samples were immediately frozen at −80°C and frozen polymer solutions were freeze-dried at −30°C and 0.01 mbar (Benchtop 2K, VirTis, NY) and stored at 4°C until further use.
Synthesis of Chitosan-4-thiobuthylamidine (Chitosan-TBA)
Chitosan-TBA was modified according to a method descried previously (CitationRoldo et al., 2004; CitationImam & Bernkop-Schnürch, 2005). 2-Iminothiolane HCl (Traut’s reagent, Sigma) was covalently linked to the primary amino group of chitosan via formation of amide bonds.
Shortly, 500 mg of medium molecular mass chitosan was dissolved in 50 mL acetic acid solution (1%, v/v). After adjusting pH to 6.5 with 1 M NaOH, 200 mg of 2-iminothiolane HCl (Traut’s reagent; Sigma, Germany) was added to the chitosan solution. The reaction mixture was incubated for 24 h at room temperature under stirring. The resulting conjugate was dialyzed (Dialysis tubing cellulose, M.W.C.O.: 12 kDa) to eliminate the remaining uncoupled 2-iminothiolane once against 5 mM HCl and twice against 5 mM HCl containing 1% NaCl, once against 5 mM HCl and finally once against 0.4 mM HCl. Afterwards, the polymer solution was freeze-dried at −30°C and 0.01 mbar (Benchtop 2K, VirTis) and stored at 4°C until further use.
Quantification of the thiol/disulfide content
The amount of free thiol groups immobilized on polymer backbones was determined photometrically with Ellman’s reagent (DTNB, 5,5’-dithiobis(2-nitronbenzoic acid)). In addition, disulfide bonds were quantified after reduction with NaBH4 and subsequent addition of Ellman’s reagent according to a method described previously (CitationMarschütz & Bernkop-Schnürch, 2002).
Preparation of the tablets
For each tablet, 20 mg of unmodified PAA or PAA-Cys was hydrated in demineralized water and 20 mg of chitosan or chitosan-TBA was hydrated in 1% acetic acid solution until homogeneous gels were formed. Unmodified polymers were used in lyophilized state in order to provide comparability. After the addition of 10 mg of RF5’PNa, these gels were homogenized and lyophilized. In the following, 30 mg of dried homogenates were compressed (Hanseaten Type EI, Hamburg, Germany) into 5.0 mm diameter flat faced tablets. The compaction pressure of 3 kN was kept constant during the preparation of all tablets.
After the preparation, the tablets were subjected to different physical characterization studies such as weight variation, hardness, friability and diameter/thickness measurements according to USP 2007. The weight variation was determined on 20 tablets using electronic balance (Sartorius, Genius, Germany). Tablet hardness was determined for a minimum of six tablets (Heberlein hardness tester). Friability was calculated as the percental weight loss of 20 tablets after rotation of 4 min at 25 rpm (Erweka TAR-10 friabilator). The diameter and thickness measurements were conducted on 20 tablets using Mitutoyo Absolute Digimatic Caliper. Diameter/thickness ratio was also calculated.
In vitro disintegration studies
Disintegration behavior of the tablets was analyzed in simulated gastric fluid pH 1.2 (80 mM HCl solution containing 0.2% NaCl) according to USP XXX with an oscillation frequency of 0.5 s−1 at 37°C.
Evaluation of the swelling behavior
The water-absorbing capacity was determined by a gravimetric method as described previously (CitationKast & Bernkop-Schnürch, 2001). In brief, tablets (30 mg) were fixed to a needle and immersed in a beaker containing simulated gastric fluid, pH 1.2 at 37°C. At pre-determined time intervals, the swollen tablets on the needle were taken out of the incubation medium, blotted to remove excess water, and the amount of water uptake was determined gravimetrically.
The swelling ratio was then calculated according to the following equation:
where Wut is the weight of uptaken water at time t and W0 is the initial weight of the dry tablet.
Morphological evaluation of the swollen tablets was carried out using a digital camera (Panasonic, Lumix, FX10). Photo imaging was performed on each tablet formulation after hydrating in simulated gastric fluid pH 1.2 for 6 h. The tablets were taken out of the medium and were imaged by a digital camera.
Evaluation of the mucoadhesive strength
TA-XTplus Texture Analyzer (Stable Micro Systems, England) was employed in order to evaluate the mucoadhesive properties of unmodified and thiolated tablets. The mucoadhesive strength of the formulations was evaluated by measuring the force required to detach the formulation from a mucin disc using a 5 kg load cell in tension mode as described previously (CitationJones at al., 2000; Citation2009; CitationBruschi et al., 2007). Mucin discs were prepared by compression of 300 mg mucin from porcine stomach (Type II, Sigma-Aldrich, Germany) using a tablet machine (Carver Laboratory press, USA) with a 13-mm diameter die. Prior to mucoadhesion testing, the mucin disc was hydrated by submersion in a 5% solution of mucin for 30 s. Excess surface liquid was removed by gentle blotting. Discs were then horizontally attached to the lower end of the cylindrical probe (length 4 cm, diameter 1 cm) using double-sided adhesive tape. Tablet formulations were placed under the probe with double-sided adhesive tape and a cylindrical probe was then lowered until the tablet was in contact with the surface of the mucin disc. Without delay, a downward force of 0.1 N was applied for a pre-defined time (180 s) to ensure intimate contact between the mucin disc and the tablet formulation. The probe was then moved upwards at a constant speed of 1.0 mm/s and the force required to detach the mucin disc from the surface of each tablet was determined as the peak value in the resulting force–distance plot. The area under the curve (AUC) was calculated from force–distance plot as the mucoadhesion. In addition, the equation given below was used to calculate the work of mucoadhesion (mJ/cm2). Measurements were performed at least with five tablets for each formulation.
where πr2 = the surface of the tablet being in contact with mucin disc.
Mucoadhesion studies via rotating cylinder
The mucoadhesive properties of the formulations were examined using a second system called the rotating cylinder method as follows. Tablets were attached to freshly excised porcine stomach mucosa which was provided from the local slaughterhouse and fixed on a stainless-steel cylinder (diameter 4.4 cm, height 5.1 cm; apparatus 4-cylinder, USP XXX) using a cyanoacrylate adhesive. After that, the cylinder was displaced into the dissolution apparatus and completely immersed into the simulated gastric fluid, pH 1.2 at 37°C. The cylinder was agitated at a speed of 100 rpm. The detachment of the test tablets was determined visually in every 30 min during an observation time of 24 h. This test was performed at least five times for each formulation (CitationBernkop-Schnürch & Steninger, 2000).
Drug release studies
The in vitro release rate of RF5’PNa from matrix tablets was determined by the paddle method according to USP XXX. Tablets were placed in the dissolution test apparatus containing 500 mL of simulated gastric fluid, pH 1.2. The study was performed at 37 ± 0.5°C with an agitation of 100 rpm. Samples were withdrawn at pre-determined time points and replaced with an equal volume of release medium equilibrated at 37°C. Sink conditions were maintained throughout the study. The release rate of RF5’PNa was quantified fluorimetrically at an excitation wavelength of 444 nm and an emission wavelength of 530 nm with a microplate reader (SLT, Spectra Fluor, Tecan, Austria). The amount of RF5’PNa was calculated by interpolation from a standard curve containing increasing concentrations in simulated gastric fluid pH 1.2.
Determination of release mechanism and kinetics
The mechanism of the release from the tablets was investigated using Ritger and Peppas equation (CitationPeppas, 1985; CitationRitger & Peppas, 1987).
where Mt is the drug released at time t, M∞ is the quantity of drug released at infinite time, Mt/M∞ is the fractional release, k is the diffusional constant, and n is the diffusional exponent which characterizes the drug release mechanism.
In addition, four kinetic models were chosen to describe the drug release profiles of the tablet formulations. Therefore, the following kinetic models, represented in equations (4–7), were investigated using a computer program for empirical analysis (CitationEge et al., 2001).
where W is the percent drug release rate at time t and kf, kH, kHC, and k0 are release rate constants.
In vivo studies
The experimental protocol for animal studies was approved by the Local Animal Ethical Committee of Ege University, Faculty of Pharmacy (Approval No. 2007/11-1).
In order to demonstrate the mucoadhesion of the tablets to stomach, the following experiment was performed: Male Albino Wistar rats, weighing 200–250 g (6–8 weeks old), were used for the in vivo study and supplied by the Experimental Animal Center of Ege University (Izmir, Turkey). They were housed in standard environmental conditions and fed with standard rodent diet with water ad libitum. The animals were divided into two groups containing 24 rats for each experimental group. Five milligrams of modified and unmodified mini tablets (diameter: 2.5 mm; dept: 1 mm) were administered orally by placing the tablets deeply into the throat of the rats in order to initiate the swallow-reflex, and immediately water was given to facilitate the same reflex. The dosed rats were fasted for 6 h and kept in restraining cages with free access to water. Afterwards, animals were sacrificed at pre-determined time points and gastrointestinal tract was anatomized, in order to determine the location of the tablets.
Statistical data analysis
Statistical data analyses were performed using t-test with p < 0.05 as the minimal level of significance.
Results and discussion
Chemical characterization of PAA-Cys and Chitosan-TBA
Improved mucoadhesive properties and high cohesiveness of the tablets can be provided with the higher amount of thiol/disulfide content of thiolated polymer (CitationGuggi et al., 2004). Therefore, determination of thiol group was crucial for PAA-Cys and Chitosan-TBA. The total amount of sulfhydryl groups immobilized on the polymer is represented by the summation of free thiol groups and disulfide bonds. In , the amount of thiol groups and disulfide bonds immobilized on the thiomers is listed.
Table 1. The amount of thiol groups and disulfide bonds immobilized on the thiomers.
Physical characterization of the tablets
All the formulations were evaluated with physical appearance, weight variation, hardness, friability, and diameter/thickness ratio, and the tablets were found to meet the pharmacopoeia requirements ().
Table 2. Physical properties of tablets.
Hardness is an important parameter for mechanical strength of the formulations and therefore it was evaluated. In all of the formulations, the thiolated tablets were found to be harder than unmodified ones. As listed in , thiolated PAA-Cys and Chitosan-TBA tablets were found to be nearly 1.171- and 1.348-times harder than unmodified PAA and chitosan tablets, respectively. In addition, both modified and unmodified chitosan tablets were found to be more fragile than PAA tablets.
Friability of all tablet formulations was found to be less than 0.8% and the friability results were also in accordance with the hardness results. The percental weight loss of unmodified PAA and chitosan was found to be 1.231- and 1.812-times more than modified ones. A correlation between degree of thiolation and physical properties like hardness and friability is assumed owing to the similar rank order in both polymers. The lowest friability value was obtained with the hardest PAA-Cys tablets and friability values of chitosan tablets were higher than PAA tablets for all formulations.
In vitro disintegration studies
In this study, tablets prepared with unmodified PAA and chitosan showed a complete disintegration and erosion within 1.06 ± 0.18 h and 1.16 ± 0.28 h in simulated gastric fluid, pH 1.2, respectively. This might be due to the insufficient cohesive forces of the polymer within the tablets. On the other hand, thiolated PAA-Cys tablets showed no disintegration or erosion within 24 h and chitosan-TBA tablets disintegrated in 22.66 ± 1.52 h (significantly different from unmodified tablets, p < 0.001). This result substantiates again the theory that the formation of disulfide bonds within the matrix tablet leads to a cross-linking which improves the cohesiveness of a dosage form (CitationBernkop-Schnürch et al., 2000; Citation2004). Due to this strongly improved structural stability, thiolated tablets disintegrated slower than tablets with unmodified polymer.
Chitosan-TBA tablets were expected to be more stable than PAA-Cys tablets according to the higher amount of thiol groups and disulfide bonds in respect of this theory. However, results of this study showed a difference in disintegration behavior of PAA-Cys and Chitosan-TBA tablets. The reason for this might be the solubility of chitosan in acidic media. Chitosan is a weak base with pKa values ranging from 6.2–7. It is insoluble at alkaline and neutral pH values such as physiological pH of 7.4. However, it is soluble at pH below 6 and the solubilization occurs by protonation of the−NH2 function on the C-2 position of the D-glucosamine repeat unit (CitationRinaudo, 2006). Hence, chitosan-TBA tablets started to disintegrate before PAA-Cys tablets in simulated gastric fluid, pH 1.2.
The disintegration behavior has a great impact on the cohesive and mucoadhesive properties of tablets. A strong adhesion of a delivery system to the mucosa is ineffective if limited cohesive forces cause rapid disintegration. Moreover, a controlled drug release out of a mucoadhesive polymeric carrier matrix will fail if the polymeric network disintegrates too early. Therefore, high cohesive properties of thiomers seem to be advantageous for gastroretentive matrix tablets.
Evaluation of the swelling behavior
The swelling behavior of a mucoadhesive formulation is one of the main parameters of the mechanism, which is responsible for its adhesive and cohesive properties and drug release as well. Therefore, the swelling behavior of tablets was evaluated in simulated gastric fluid pH 1.2, and the results of the swelling study are depicted in . As can be seen from the figure, in the mimicking situation of stomach the swelling behavior of the unmodified tablets differs significantly from that of modified tablets. PAA and chitosan tablets reached the highest swelling ratio after 15 min and showed a 1.100 ± 0.151 and 1.275 ± 0.058-fold weight gain, respectively. Then, they progressively lost their weight until they were completely dissolved and/or eroded over 60 or 90 min.
Figure 1. Comparison of the swelling behavior of unmodified tablets (a) and thiolated tablets (b) incubated in simulated gastric fluid pH 1.2 at 37°C. (Indicated values are the means of three experiments ± SD.).
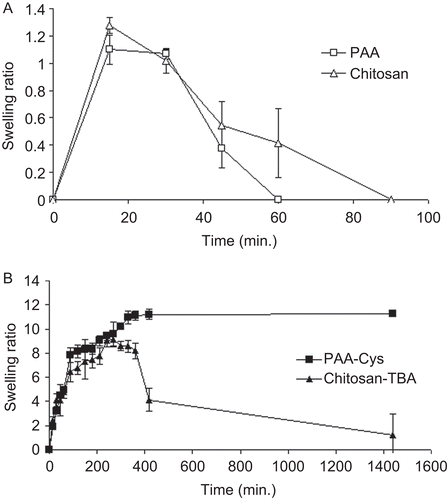
On the other hand, the weight of thiolated PAA-Cys and chitosan-TBA tablets increased continuously over 7 h and 4.5 h with the highest swelling ratios of 11.20 ± 1.49 and 9.12 ± 0.57, respectively. The swelling ratio of PAA-Cys tablets did not change at all during 24 h of experiment, whereas chitosan-TBA tablets started to lose their gained weight during 24 h.
Visual observation indicated that the morphology of PAA-Cys and chitosan-TBA tablets is noticeably different from each other. The matrix tablets of PAA-Cys swelled with formation of viscous gel mass, whereas surface erosion can be seen from swollen chitosan-TBA tablets ().
Evaluation of the mucoadhesive strength
The mucoadhesive properties of the tablets must be determined sensitively because of the great importance to prolong the residence time. Therefore, a TA-XTplus texture analyzer was employed for evaluation of these characteristics.
The mucoadhesive properties of tablet formulations were examined by evaluation of the detachment force required to overcome the adhesive bond between tablet formulations and mucin discs. In addition, work of adhesion was calculated because it provides wider evaluation of the detachment phenomena and represents not only the maximum force of detachment which corresponds to F evaluation, but also the sum of all established bonds (CitationNeves et al., 2008). The results of this study are presented in . It is clear that thiolated PAA-Cys and chitosan-TBA tablets required 1.864- and 1.533-fold higher vertical detachment force to break the mucoadhesive bond than unmodified tablets, respectively. In addition, the mucoadhesion work of thiolated PAA-Cys and chitosan-TBA tablets were 1.341- and 2.139-times higher than unmodified ones. These results were in accordance with previous studies which showed that immobilized thiol groups on the polymer lead to an increase in the mucoadhesion of the formulations. Previously, CitationGuggi et al. (2004) evaluated the mucoadhesion of thiolated tablets with a tensiometer on native porcine intestinal mucosa and the results indicated the total work of adhesion for thiolated tablets was higher in comparison to unmodified ones.
Table 3. Results of mucoadhesion studies performed with TA-XTplus texture analyzer.
As can be seen from , the detachment force and mucoadhesion work of modified chitosan-TBA tablets were higher than PAA-Cys tablets. These improved mucoadhesive properties of chitosan-TBA might be due to the higher amount of thiol and disulfide groups per gram of polymer. On average, 371.11 µmol free thiol groups and 572.74 µmol oxidized thiol groups were immobilized per gram chitosan and these results are 2.147- and 1.515-times higher than PAA-Cys. Generated by the immobilization of sulfhydryl-bearing compounds on the backbone of mucoadhesive polymers, such as chitosan and PAA, thiomers display significantly increased mucoadhesive properties as a result of covalent attachment to mucus glycoproteins. As the degree of modification of the polymer was increased, the mucoadhesive features were enhanced (CitationGuggi et al., 2004).
Consequently, these findings illustrate the usefulness of TA-XTplus texture analyzer for predicting the bioadhesive nature of the thiolated tablets. Also, this study indicates that mucin discs can be used instead of natural mucosal membranes to determine the mucoadhesive strength of the formulation because they are easy to produce and handle.
Mucoadhesion studies via rotating cylinder
Mucoadhesion studies were performed according to the rotating cylinder method. This method takes the disintegration behavior of the tablets in simulated gastric fluid into account. It is, therefore, supposed to be in closer correlation to in vivo conditions. The results showed that the mucoadhesion times of PAA, PAA-Cys, chitosan and chitosan-TBA tablets were 1.5 ± 0.5, 21 ± 1, 1 ± 0.5, 17 ± 1 h, respectively. The results obtained with PAA and chitosan support previous findings about their weak and short-lasting mucoadhesion (CitationGrabovac et al., 2005). The mucoadhesion time of the thiolated PAA-Cys and chitosan-TBA tablets was found to be nearly 14- and 17-times greater than unmodified ones, respectively. Unmodified PAA and chitosan tablets were found to be completely disintegrated during this time period. On the other hand, after the detachment of modified PAA-Cys and chitosan-TBA tablets neither erosion nor disintegration was observed, which also indicates good cohesive characteristics of the thiolated tablets (CitationGuggi et al., 2004). Moreover, this result confirms the theory that the thiol groups of the polymer are able to react with cysteine-rich sub-domains of mucin glycoproteins, thereby forming covalent bonds to the mucus layer. Therefore, an improved mucoadhesion was observed for modified tablets (CitationGuggi et al., 2004).
Chitosan is a cationic polymer and its mucoadhesion is mainly based on ionic interactions with anionic sub-structures of mucus layer (CitationBernkop-Schnürch, 2000). In addition, chitosan-TBA has the advantage that, on the one hand, 2-iminothiolane introduces cationic amidine sub-structures, while, on the other hand, thiol groups are immobilized. This conjugate, therefore, offers a second possibility to affect the functional groups of mucin glycoproteins (CitationBernkop-Schnürch et al., 2003). Hence, the mucoadhesion times of chitosan-TBA was supposed to be higher than those of PAA-Cys tablets as it was substantiated before with mucoadhesion studies performed with a TA-XTplus texture analyzer. However, the mucoadhesion times of chitosan-TBA tablets were significantly shorter than PAA-Cys tablets (p < 0.020)., In the rotating cylinder method, the mucoadhesion times of the formulations were investigated in simulated gastric fluid, and solubility of chitosan in acidic media caused this result.
Drug release studies
Release studies were carried out with matrix tablets and the release rate of RF5’PNa from dosage forms is displayed in .
Figure 3. Release profiles of RF5’PNa from tablets based on PAA, PAA-Cys, chitosan, and chitosan-TBA. Indicated values are the means of three experiments ± SD.
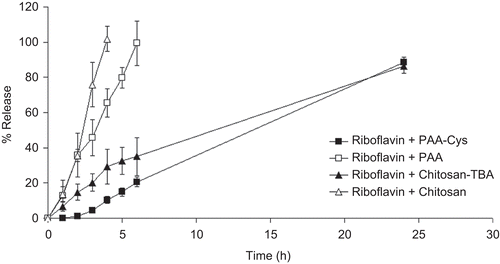
The released amount of RF5’PNa reached 98.45% in 6 h with unmodified PAA tablets and reached 100% in 4 h with unmodified chitosan tablets. The results of disintegration studies showed a complete disintegration of the unmodified tablets almost within 1 h. According to this consideration, it seems that RF5’PNa release continued from tablet fragments after disintegration.
The results demonstrated that thiolated PAA-Cys and Chitosan-TBA tablets released 20.51% and 34.99% of RF5’PNa within 6 h and the released amount reached 88.53 % and 86.34% at the end of 24 h, respectively.
Thiolated PAA-Cys and chitosan-TBA tablets have the ability to hydrate very rapidly and in addition exhibit a great swelling index compared to unmodified tablets as it was shown previously. Drug release might be influenced by this high swelling ratio and exhibited sustained release property. Swelling of the matrix tablets was activated by simulated gastric fluid, pH 1.2, and the drug release was controlled by the interaction among the simulated gastric fluid, the polymer, and the drug. Due to simulated gastric fluid penetration, the tablets were exposed to continuous changes in their structure and the thickness increased with the formation of a gel layer. As the thickness of the gel layer increased, the drug release was slowed down due to the increase of the diffusion path length (CitationLee & Peppas, 1987; CitationColombo et al., 2000).
Another reason for the sustained release seems to be the formation of disulfide bonds within the matrix tablet. This cross-linking process might provide a tightened three-dimensional polymeric network leading to a more controlled drug release. Hence, the advantages of the controlled release system based on the mucoadhesive polymers can be improved by using their thiolated derivatives.
As it can be seen from , release rate of chitosan-TBA tablets is significantly faster than that of PAA-Cys tablets (p < 0.043). The reason is considered to be due to erosion caused by solubility of chitosan in acidic medium.
Overall, the unmodified tablets dissolved/eroded progressively releasing the drug during this process, while modified tablets swelled continuously maintaining a good cohesiveness and releasing the active agent via a controlled diffusion process.
Determination of release mechanism and kinetics
The quantity of the drug released from the matrix tablets is often analyzed as a function of the square root of time; this is typical for systems where the drug release is governed by pure diffusion. However, the use of this relationship in swellable systems is not completely justified, as such systems can be erodible and the contribution of the relaxation of polymeric chains to drug transport has to be taken into account. Therefore, an analysis of the drug release from swellable matrices must be performed with a flexible model that can identify the different contributions to overall kinetics (CitationColombo et al., 2000). An empirical equation (equation 3), proposed by CitationRitger and Peppas (1987), rapidly gained popularity for the analysis of release data in these systems.
In this study, the dissolution data were fitted to equation (3), and best-fit parameters were calculated. According to this equation, when n = 0.45 this indicates case I (Fickian) diffusion or square root of time kinetics, when 0.45 < n < 0.89 this indicates anomalous (non-Fickian) diffusion, when n = 0.89 this indicates case II transport, and n > 0.89 indicates super case II transport.
The values of diffusional exponents (n), release rate constants (k) and correlation coefficients (r2) are listed in . The n values of PAA, PAA-Cys, and Chitosan tablets were determined as 1.004, 1.098, and 1.438, respectively. This result indicated that the drug release from these tablets is of super case II type. In this case, the release was controlled only by polymeric chain relaxation of a plasticization process in the gel layer. The n value of Chitosan-TBA tablets is 0.790 and the drug release mechanism is anomalous (non-Fickian) diffusion for swelling of the materials and the release mechanisms of this formulation were diffusional and polymeric chain relaxation.
Table 4. Drug release parameters of formulations obtained from equation (3).
As can be seen from , release rate constant (k) values of the formulations were significantly different. As the k value becomes higher, the drug is released faster. The k values of PAA-Cys and Chitosan-TBA tablets were 3.183- and 1.254-times lower than PAA and chitosan tablets, respectively, indicating the slower and controlled drug release. The order of k values is in accordance with the results obtained from release studies.
In order to investigate the drug release kinetics, the dissolution data of formulations was evaluated in relation to four kinetic models as mentioned previously by means of a computer program. shows the determination coefficient values (r2) of the formulations. It can be seen in that RF5’PNa+PAA, RF5’PNa+PAA-Cys and RF5’PNa+Chitosan show nearly zero-order drug release and RF5’PNa+Chitosan-TBA shows first order drug release and these results are in accordance with the n values of power law analyzed.
Table 5. The determination coefficients (r2) of release kinetics for matrix tablet formulations.
In vivo studies
In order to substantiate the longer gastroretention time of thiolated tablets, the in vivo study was also performed. Tablet formulations with modified and unmodified polymers were administered orally to the rats by placing the tablets deeply into the throat of the rats in order to initiate the swallow-reflex, and water was given immediately to facilitate the same reflex. The animals were sacrificed every hour after the application and the gastrointestinal tracts were anatomized visually.
The visual investigation of the rats showed that the swollen modified tablets with PAA-Cys were found in the stomach until 5 h after administration in 19 rats (79.17 %) (see ). After 6 h, thiolated tablets began to disintegrate into pieces (see ). In three rats (12.5%), the tablets adhered to the palate and esophagus and did not reach the stomach. In two rats (8.33%), the tablets disintegrated between 4–5 h due to the individual differences between rats.
Figure 4. The appearance of swollen PAA-Cys tablet in the rat stomach at the end of 5 h (a) and the appearance of disintegrated PAA-Cys tablet in the rat stomach at the end of 6 h (b).
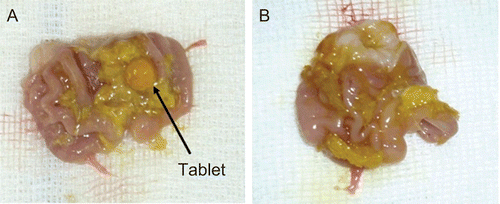
On the other hand, in 22 rats (91.66%) the unmodified PAA tablets were found to be completely disintegrated within the first hour of the experiment. In two rats (8.33%), the tablets were found to be adhered to the palate and esophagus.
The investigation of Chitosan-TBA tablets administered rats showed that in two rats (8.33%), the tablets adhered to the palate and esophagus. In six rats (25%), the tablets disintegrated within less than 5 h. In 16 rats, the tablets were found in the stomach after 5 h (66.66%) and then started to disintegrate (see ).
Figure 5. The appearance of chitosan-TBA tablet in the rat stomach at the end of 5 h (a), and the appearance of disintegrated Chitosan-TBA tablet in the rat stomach at the end of 6 h (B).
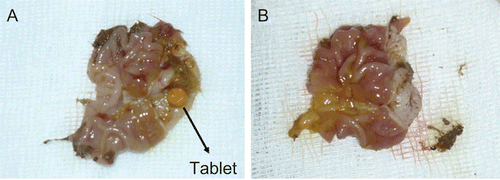
The unmodified chitosan tablets disintegrated completely in 21 rats (87.5%) at 1 h of experiment and in three rats (12.5%) the tablets adhered to the palate and esophagus and did not reach the stomach.
As can be seen from the in vivo results, the observation of nearly 10% of tablets remaining in the esophagus and palate is a serious limitation to the use of these tablets. Therefore, the tablets should be coated in order to prevent mucoadhesion during administration. Previously, CitationGuggi et al. (2003) prepared the mucoadhesive stomach targeted tablets of salmon calcitonin and coated them with a triglyceride (Miglyol 810) and prevented the mucoadhesion in the oral cavity.
The results showed a clear difference between in vitro mucoadhesion study with rotating cylinder method and in vivo study with rats for thiolated tablets. PAA-Cys and Chitosan-TBA tablets were found to be stable only up to 6 h with in vivo experiments, whereas the in vitro disintegration study and mucoadhesion study proved high cohesive properties and improved mucoadhesion properties of tablets up to 24 h. The mechanical stress to which formulations are exposed in the Ph. Eur.-test apparatus during rotating cylinder method do not strictly relate to in vivo conditions. In addition, different from simulated gastric fluid; gastric secretion is an aqueous isotonic solution containing H+, Na+, K+, Cl−, GCO3−, mucus, intrinsic factor, pepsinogen, and gastric lipase (CitationBardonnet et al., 2006). Therefore, motility of the stomach, pH variations, and gastric secretions could be the reason for the difference between in vitro and in vivo results.
Conclusion
Within the present study a gastroretentive matrix tablet formulations of PAA-Cys and chitosan-TBA have been evaluated and compared with the unmodified formulations. The results demonstrated that the thiolated matrix tablet is a promising approach for gastroretentive drug systems due to higher cohesiveness of the tablet, improved mucoadhesion, and the controlled drug release properties. Although the findings illustrate the usefulness of both thiolated matrix tablets, PAA-Cys tablets appear to be more promising than chitosan-TBA tablets due to the solubility of chitosan in acidic media.
Acknowledgements
The scholarship for this work was financed by The Scientific and Technological Research Council of Turkey (TUBITAK). Also this work was supported by Ege University (Project no: 08/ECZ/010).
Declaration of Interest
The authors report no conflicts of interest. The authors alone are responsible for the content and writing of the paper.
References
- Andrews, G.P., Laverty, T.P., Jones, D.S. (2009). Mucoadhesive polymeric platforms for controlled drug delivery. Eur J Pharm Biopharm. 71:505–18.
- Ay Senyigit, Z., Vetter, A., Guneri, T., Bernkop-Schnürch, A. (2010). Gastroretentive particles formulated with thiomers: development and in vitro evaluation. J Drug Target. 18:362–72.
- Bardonnet, P.L., Faivre, V., Pugh, W.J., Piffaretti, J.C., Falson, F. (2006). Gastroretentive dosage forms: overview and special case of Helicobacter pylori. J Contr Rel. 111:1–18.
- Bernkop-Schnürch, A. (2000). Mucoadhesive polymers. In: Dumitriu, S., ed. Polymeric biomaterials. New york: Marcel Dekker, 147–65.
- Bernkop-Schnürch, A. (2005). Thiomers: a new generation of mucoadhesive polymers. Adv Drug Deliv Rev. 57:1569–82.
- Bernkop-Schnürch, A., Hornof, M., Zoidl, T. (2003). Thiolated polymers– thiomers: synthesis and in vitro evaluation of chitosan–2-iminothiolane conjugates. Int J Pharm. 260:229–37.
- Bernkop-Schnürch, A., König, V., Leitner, V.M., Krauland, A.H., Brodnik, I. (2004). Preparation and characterisation of thiolated poly(methacrylic acid)-starch compositions. Eur J Pharm. Biopharm. 57:219–24.
- Bernkop-Schnürch, A., Scholler, S., Biebel, R.G. (2000). Development of controlled drug release systems based on polymer-cysteine conjugates. J Contr Rel. 66:39–48.
- Bernkop-Schnürch, A., Schwarz, V., Steininger, S. (1999). Polymers with thiol groups: a new generation of mucoadhesive polymers? Pharm Res. 16:876–81.
- Bernkop-Schnürch, A., Steninger, S. (2000). Synthesis and characterization of mucoadhesive thiolated polymers. Int J Pharm. 194:239–47.
- Bruschi, M.L., Jones, D.S., Panzeri, H., Gremião, M.P.D., Freitas, O., Lara, E.H.G. (2007). Semisolid systems containing propolis for the treatment of periodontal disease: in vitro release kinetics, syringeability, rheological, textural, and mucoadhesive properties. J Pharm Sci. 96:2074–89.
- Colombo, P., Bettini, R., Santi, P., Peppas, N.A. (2000). Swellable matrices for controlled drug delivery: gel-layer behaviour, mechanisms and optimal performance. Pharm Sci Technolo Today. 3:198–204.
- Ege, M.A., Karasulu, H.Y., Karasulu, E., Ertan, G. (2001). A computer program designed for in vitro dissolution kinetics, in vitro–in vivo kinetic correlations and routine application. 4th Central European Symposium on Pharmaceutical Technology, Vienna, Scientia Pharmaceutica 69:127–8.
- Grabovac, V., Guggi, D., Bernkop-Schnürch, A. (2005). Comparison of the mucoadhesive properties of various polymers. Adv Drug Deliv Reviews. 57:1713–23.
- Gröning, R., Cloer, C., Georgarakis, M., Müller, R.S. (2007). Compressed collagen sponges as gastroretentive dosage forms: in vitro and in vivo studies. Eur J Pharm Sci. 30:1–6.
- Guggi, D., Krauland, A.H., Bernkop-Schnürch, A. (2003). Systemic peptide delivery via stomach: in vivo evaluation of an oral dosage form for salmon calcitonin. J Contr Rel. 92:125–35.
- Guggi, D., Marschütz, M.K., Bernkop-Schnürch, A. (2004). Matrix tablets based on thiolated poly(acrylic acid): pH-dependent variation in disintegration and mucoadhesion. Int J Pharm. 274:97–105.
- Imam, M.E., Bernkop-Schnürch, A. (2005). Controlled drug delivery systems based on thiolated chitosan microspheres. Drug Dev Ind Pharm. 31:557–65.
- Jones, D.S., Bruschi, M.L., Freitas, O., Gremião, M.P.D., Lara, E.H.G., Andrews, G.P. (2009). Rheological, mechanical and mucoadhesive properties of thermoresponsive, bioadhesive binary mixtures composed of poloxamer 407 and carbopol 974P designed as platforms for implantable drug delivery systems for use in the oral cavity. Int J Pharm. 372:49–58.
- Jones, D.S., Woolfson, A.D., Brown, A.F., Coulter, W.A., McClelland, C., Irwin, C.R. (2000). Design, characterization and preliminary clinical evaluation of a novel mucoadhesive topical formulation containing tetracycline for the treatment of periodontal disease. J Contr Rel. 67:357–68.
- Kagan, L., Lapidot, N., Afargan, M., Kirmayer, D., Moor, E., Mardor, Y., Friedman, M., Hoffman, A. (2006). Gastroretentive accordion pill: enhancement of riboflavin bioavailability in humans. J Contr Rel. 113:208–15.
- Kast, C.A., Bernkop-Schnürch, A. (2001). Thiolated polymers: development and in vitro evaluation of chitosan-thioglycolic acid conjugates. Biomaterials. 22:2345–52.
- Klausner, E.A., Lavy, E., Stepensky, D., Friedman, M., Hoffman, A. (2002). Novel gastroretentive dosage forms: evaluation of gastroretentivity and its effect on riboflavin absorption in dogs. Pharm Res. 19:1516–23.
- Lee, P.I., Peppas, N.A. (1987). Prediction of polymer dissolution in swellable controlled-release systems. J Contr Rel. 6:207–15.
- Leitner, V.M., Marschütz, M.K., Bernkop-Schnürch, A. (2003a). Mucoadhesive and cohesive properties of poly(acrylic acid)-cysteine conjugates with regard to their molecular mass. Eur J Pharm Sci. 18:89–96.
- Leitner, V.M., Walker, G.F., Bernkop-Schnürch, A. (2003b). Thiolated polymers: evidence for the formation of disulphide bonds with mucus glycoproteins. Eur J Pharm Biopharm. 56:207–14.
- Marschütz, M.K., Bernkop-Schnürch, A. (2002). Thiolated polymers: self-crosslinking properties of thiolated 450 kDa poly(acrylic acid) and their influence on mucoadhesion. Eur J Pharm Sci. 15:387–94.
- Neves, J., Amaral, M.H., Bahia, M.F. (2008). Performance of an in vitro mucoadhesion testing method for vaginal semisolids: influence of different testing conditions and instrumental parameters. Eur J Pharm Biopharm. 69:622–32.
- Peppas, N.A. (1985). Analysis of fickian and non-fickian drug release polymers. Pharm Acta Helv. 60:110–1.
- Rinaudo, M. (2006). Chitin and chitosan: properties and applications. Prog Polym Sci. 31:603–32.
- Ritger, P.L., Peppas, N.A. (1987). A simple equation for description of solute release II. Fickian and anomalous release from swellable devices. J Contr Rel. 5:37–42.
- Roldo, M., Hornof, M., Caliceti, P., Bernkop-Schnürch, A. (2004). Mucoadhesive thiolated chitosans as platforms for oral controlled drug delivery: synthesis and in vivo evaluation. Eur J Pharm Biopharm. 57:115–21.
- Talukder, R., Fassihi, R. (2004). Gastroretentive delivery systems: a mini review. Drug Dev Ind Pharm. 30:1019–28.
- USP XXX-NF XXV. (2007). United States Pharmacopeial Convention Inc. 12601 Twinbrook Parkway, Rockville, MD 20852.