Abstract
Context: Arsenic is a naturally occurring toxicant that causes acute and chronic adverse health effects, including cancer.
Objective: The study was performed to evaluate the therapeutic efficacy of liposome entrapped flavonoidal quercetin in combating arsenic toxicity mediated oxidative damage in hepatocytes and brain cells in rat model.
Materials and methods: Hepatic and neuronal cell damage in rats was made by daily arsenic (6mg/kg b wt, 9 mg/kg b wt and 12 mg/kg b wt) treatment via oral route for four consecutive months. Liposomal quercetin (2.71mg QC/kg b. wt) were injected s.c. on rats treated with 12 mg/kg b. wt. NaAsO2 twice a week for four months.
Results and Discussion: Inorganic arsenic deposition was found to be most significant in hepatic (9.32 ± 0.100 µg/g tissue) and neuronal (6.21 ± 0.090 µg/g tissue) cells of rats treated with 12 mg/kg b wt of arsenite. Antioxidant levels in hepatic and neuronal cells were reduced significantly by the induction of arsenic. Liposomal quercetin was found most potent for a complete prevention of arsenite-induced reduction in antioxidant levels in the liver and brain of rats. Arsenic induced a substantial increase in hepatic hydroxyproline (HP) and Liposomal quercetin treatment resulted in complete replenishment of the HP level to normal. Liposomal quercetin completely prevented the arsenite-induced upregulation of cytochrome c expression in liver and brain significantly suggesting that the protective effect of Liposomal quercetin could be related to the reduction of arsenic deposition in both the organs. Conclusion: Thus, Liposomal quercetin might prove to be of therapeutic potential against arsenite-induced hepatic and neuronal cell damage in rats.
Introduction
Heavy metals and metalloids contamination of water and soil is a common phenomenon due to their environmental pervasiveness. Arsenic is a metalloid found in water, soil and air from natural and anthropogenic sources. It is one of the most important global environmental toxicant that enters human body mainly through arsenic contaminated drinking water (CitationCelik et al., 2008). Intensity of environmental arsenic has dramatically increased in recent years. Chronic arsenic exposure has been implicated in several noncancerous conditions, in particular, skin disease, hypertension and cardiovascular disease, perturbed porphyrin metabolism, and irreversible noncirrhotic portal hypertension (National Academy Press, Citation2001).
Arsenic causes hepatocellular disorders in many ways. Although mechanism(s) by which arsenic induces such pathological changes in the liver remains poorly understood, the common phenomenon that has emerged is the role of toxic reactive oxygen species (ROS). Chronic arsenic exposure is also associated with neurodegeneration. Peripheral neuropathy is common in persons exposed to arsenic contaminated drinking water-induced chronic toxicity (CitationMazumder et al. 1992).
Oxidative damage is also considered a likely cause of arsenic-induced brain damage because the brain is believed to be particularly vulnerable to oxidative stress due to high oxygen consumption rate and high level of polyunsaturated fatty acids (PUFA), and relatively high rate of oxygen free radical generation without commensurate levels of antioxidant defenses (CitationCoyle et al. 1993).
Drug accessibility to specific target organs restricts the selection of drug permeabilities through the gastrointestinal tract and blood brain barrier. Bioavailability of drugs can be improved when they are administered intravenously upon entrapment in liposomes (CitationGregoriadis et al. 1988). Our previous report indicates that nanoencapsulation of drug enhances its dietary efficiency in combating arsenic–induced acute toxicity in liver and brain of rats (CitationGhosh et al. 2009).
Liposomes are accepted as potent drug carriers not only for their biocompatible nature but also for their negative biological responses that generally occur when a foreign material is introduced in the system (CitationSinha et al. 2000). Although it is unlikely that liposomes can cross the blood-brain-barrier (CitationMicklus et al. 1992), they circulate in the plasma and are ultimately taken up by the liver, digested and the lipid components released and redistributed to other organs (CitationDerksen et al., 1988). For delivery to the brain, liposomes should generally be less than about 1.0 microns in size, more preferably about 0.2 to 0.45 microns. Lower portion of administered small unilamellar liposomes passes through endothelial junction of BBB. Quercetin, the most abundant bioflavonoid in nature, contains a number of phenolic hydroxyl groups and exhibits its therapeutic potential against many diseases, including ischemic heart diseases, liver fibrosis, renal injury, and chronic billiary obstruction (CitationPeres et al. 2000; CitationLee et al. 2003; CitationSingh et al. 2004; CitationTokyol et al. 2006). In our previous study (CitationGhosh et al., 2009), the preventive effect of a single oral dose of nanoencapsulated quercetin over arsenic-induced acute toxicity in liver and brain of adult female rats were studied. Therefore, it seems reasonable to expect that simultaneous multiple dose administration (s.c.) of quercetin encapsulated in liposomes might contribute to reduce chronic NaAsO2-induced oxidative damage in cellular and subcellular parts of hepatic and brain tissues and provide a protective mechanism against liver fibrosis as well as neurodegeneration.
The present study therefore explores the therapeutic potential of liposomal quercetin in treating chronic arsenic toxicity in adult female Swiss albino rats.
Materials and Methods
Materials
1,2-Dimyristoyl-sn-glycero-3-phosphoethanolamine, Cholesterol, Dicetyl phosphate (DCP), p-amino phenyl α-D-galactoside, glutaraldehyde, collagenase, diphenyl hexatriene (DPH), Triton X-100, Glutathione reductase and Quercetin were purchased from Sigma Chemicals (St. Louis, MO). Sodium arsenite (Merck, Darmstadt, Germany) was used for experimental purposes. Chloramine-T was acquired from Loba Chemie Pvt. Ltd. (Mumbai, India) and Fluka (Bluchs, Switzerland), respectively, whereas chloroform and methanol were from E. Merck (India). All other reagents were of analytical grade.
Preparation of Liposomal QC
Multilamellar liposomes were prepared with 1,2-dimyristoyl-sn-glycero-3-phospho ethanolamine, cholesterol, dicetyl phosphate (DCP) and quercetin in molar ratio 7:1:1:1 (CitationDas et al., 2005). In short, PE, cholesterol, DCP, and quercetin were dissolved in chloroform and methanol mixture (2:1, v/v) in a round-bottom flask. The lipid film was made by drying the organic solvents and was desiccated overnight. The thin film was swollen in phosphate-buffered saline (pH 7.2) for 1 h and sonicated in a probe-type sonicator for 60 s. The suspension was centrifuged (10,000 × g) for 1 h and washed twice in PBS.
Determination of size of quercetin-encapsulated liposomes
The size determination of quercetin-encapsulated liposomes was done through dynamic light scattering using a mean laser wavelength of 632.2nm and a 90°-angle measurement. The average diameter of the liposomal quercetin was measured to be 200 nm.
Animal and Experimental Design
Adult female Swiss albino rats, each weighing 120 to 150 g, were acclimatized to conditions in the laboratory (26–28°C, 60–80% relative humidity, 12-h light/dark cycle) for 10 days before the commencement of the treatment, during which they received food (commercial pelleted rat chow, purchased from Hindustan Lever Limited, Maharashtra, India) and drinking water ad libitum. Animals were randomly selected for groups and sodium arsenite and drug were administered as per individual body weight of rat. Rats were divided into eight groups with six animals in each group. Rats in the normal group were fed plain water (0.5 ml) by gavaging syringe. Three groups of rats were fed NaAsO2 (6 mg/kg b. wt., 9 mg/kg b. wt. And 12 mg/kg b. wt) contaminated water (0.5 ml) for four months. Freshly prepared free quercetin (2.71mg QC/kg b. wt) suspension (5 ml/kg b. wt) of 0.2% Tween 80 was injected s.c. on three other groups of rats subsequently treated with the three doses of NaAsO2 twice a week for four months simultaneously. Liposomal quercetin (5 ml/kg b. wt suspension containing 2.71 mg QC/kg b. wt) were injected s.c. twice a week on the group of rats treated with 12 mg/kg b. wt. NaAsO2 simultaneously for four months. After four months of treatment, the rats of all the groups were sacrificed using ether anesthesia and liver and brain tissues were collected from each rat and were frozen for future analysis. Parts of liver and brain were promptly removed and fixed in Bouin’s fixative and processed for histological examination. All the rats used in this study received proper care in compliance with the Animal Ethics Committee, India, Registration No. 147/99/CPC SEA, India. Liver histochemistry for collagen and liver and brain tissue histology were studied by microscopic examination (CitationLin et al. 2005).
Biochemical Analysis and Enzyme Assays
Lipid Peroxidation Assay
Lipid peroxidation in the mitochondrial membrane was determined by measuring the amount of conjugated diene (CitationRecknagel and Glende 1984). Proteins were measured according to CitationLowry et al. (1951).
Reduced Glutathione (GSH) assay
The intracellular GSH contents in the liver and brain tissues were assayed using Ellman’s Reagent [5,5′-dithiobis-2-nitrobenzoic acid (DTNB)] according to the method described by CitationGriffith (1980). A standard reference curve was prepared for each assay.
Assay of cytosolic enzymes
A part of the liver and brain tissue was homogenized in 50 mM sodium phosphate buffer (pH 7.0), and the homogenate was centrifuged at 105,000g for 1 h. The supernatant portion was used for the assay of catalase (CitationAebi 1984) and superoxide dismutase (SOD) (Marklund and Marklund 1984).
Measurement of Arsenic
Parts of liver and brain tissues of rats, treated with sodium arsenite and sodium arsenite with free quercetin or liposomal quercetin were used for arsenic estimation in an atomic absorption spectrophotometer (Spectra AA 30/40, Varian Inc, Palo Alto CA) fitted with a graphite furnace (CitationFlora et al. 1995).
Estimation of Hepatic 4-Hydroxyproline
4-Hydroxyproline (4-HP) levels from liver tissues were measured by the method of CitationSarin et al. 1999. The absorbance was read at 558 nm, and values were plotted against a standard graph using known amounts of 4-HP.
Western Blot to detect cytochrome c
Cytosolic fractions of liver and brain tissues were used for Western blot analysis of cytochrome c. The cytosolic fractions were subjected to 12.5% SDS-PAGE at 100 volts followed by electroblotting to PVDF membrane. The membranes were blocked with 5% non fat milk and probed with an anti-cytochrome c (1:2000) antibody. Following the secondary antibody incubation, the membranes were rinsed and bound antibodies were detected using BCIP-NBT salts (CitationManiatis et al. 1975).
Statistical analysis
The mean and standard deviation were calculated for all data. Significant differences between means were evaluated by an analysis of variances. A difference was considered significant when P<0.05.
Results
Effect of liposomal quercetin on NaAsO2-induced formation of conjugated diene in liver and brain tissues of rats.
The mean ± SE values of diene that are generated in liver and brain tissues of rats by chronic NaAsO2 oral treatment (6mg/kg b wt, 9mg/kg b wt and 12 mg/kg b wt) is summarized in . Chronic NaAsO2 oral treatment of 6 mg/kg. b. wt. and 9 mg/kg. b. wt. showed substantial increase in diene level both in liver (3.64 ± 0.057 and 4.04 ± 0.074 simultaneously) and brain (4.59 ± 0.069 and 5.23 ± 0.102 simultaneously) tissues of rats. Simultaneous treatment of those rats with s.c. injections of free quercetin (2.71 mg QC/kg b. wt) twice a week resulted in no significant changes in diene level in both liver and brain tissues. NaAsO2 oral treatment of 12 mg./kg. b. wt. showed a marked increase in diene level in both liver (4.72 ± 0.070) and brain (4.06 ± 0.185) tissues of rats (). From the values it is very much clear that the effect of chronic NaAsO2 treatment of 12mg/kg. b. wt. on diene level is pretty close to that produced by 9 mg/kg. b. wt. NaAsO2 treatment. Simultaneous treatment of these group of rats (treated with 12 mg/kg. b. wt. NaAsO2) with s.c. injections of free quercetin (2.71mg QC/kg b. wt) twice a week hardly produced any significant difference in the diene level (). Upon simultaneous treatment with s.c. injections of liposomal quercetin (2.71mg QC/kg b. wt.) twice a week, the diene levels decreased significantly both in liver (1.39 ± 0.043) and brain (2.03 ± 0.061) tissues of rats ().
Table 1. Effect of liposomal Quercetin on the formation of conjugated diene in liver and brain tissues of rats simultaneously treated with chronic arsenic doses of 6 mg/kg b wt, 9 mg/kg b wt and 12 mg/kg b wt.
Effect of liposomal quercetin on chronic NaAsO2-induced changes in antioxidant defence system in liver and brain tissues of rats.
Chronic oral treatment of NaAsO2 induced alterations in different enzymes viz SOD and catalase in liver and brain tissues of rats is shown in . Oral treatments of NaAsO2 (6 mg/kg. b. wt., 9 mg/kg. b. wt. and 12mg/kg. b. wt.) resulted in significant depletion of antioxidant level both in liver and brain tissues of rats (). No significant protection was observed in the case of rats treated simultaneously with s.c. injections of free quercetin. Liposomal quercetin treatments given s.c. twice a week on rats that were fed with 12mg/kg. b. wt. NaAsO2 exerted significant protection to these enzymes in both liver and brain tissues of rat.
Table 2. Effect. of liposomal quercetin on the antioxidant enzyme activities in brain and liver tissues of adult rats simultaneously treated with chronic arsenic doses of 6 mg/kg b wt, 9 mg/kg b wt and 12 mg/kg b wt.
Glutathione, the most important intracellular antioxidant significantly decreased in the liver and brain tissues of rats treated with chronic arsenic (). The cellular GSH content was significantly increased in both liver and brain tissues of rats that received liposomal quercetin ().
Figure 1. GSH concentration in liver and brain tissues of chronic arsenic treated rats (each group containing 6 rats). Values are mean ±S.E. *, P<.001, significantly different from NaAsO2 treated rats. 1, Normal. 2, NaAsO2 (6mg./kg. b wt) treated. 3, NaAsO2 (9mg./kg. b wt) treated. 4, NaAsO2 (12 mg./kg. b wt.) treated. 5, NaAsO2 (12 mg./kg. b wt.) + Free QC treated. 6, NaAsO2 (12 mg./kg. b wt) + Liposomal QC treated. * Indicates significant difference from normal, ** Indicates significant difference from NaAsO2 (6mg./kg. b wt) treated, *** Indicates significant difference from NaAsO2 (12 mg./kg. b wt) treated.
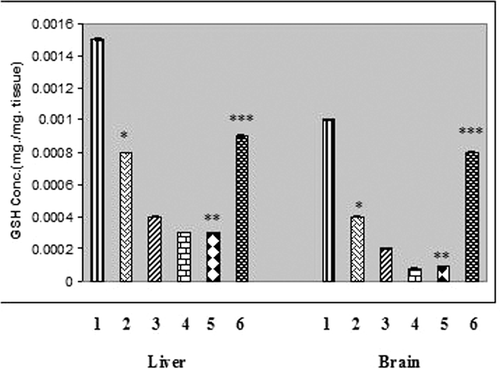
Effect on liposomal quercetin on arsenic deposition in liver and brain tissues of chronic arsenic treated rats.
Chronic arsenic treatment resulted in deposition of arsenic in liver and brain tissues of rats at the levels of 9.32 ± 0.100 µg/g tissue and 6.21 ± 0.090 µg/g tissue respectively. The elevated arsenic level in liver and brain tissues of experimental animals was found to be reduced maximally by the treatment with liposomal quercetin (), where no detectable amount of arsenic was present in the liver or brain tissue in normal rats as determined by atomic absorption spectrophotometer.
Figure 2. Arsenic concentration in liver and brain tissues of chronic arsenic treated rats (each group containing 6 rats). Values are mean ±S.E. *, P<.001, significantly different from NaAsO2 treated rats. 1, Normal. 2, NaAsO2 (6mg./kg. b wt) treated. 3, NaAsO2 (9mg./kg. b wt) treated. 4, NaAsO2 (12 mg./kg. b wt.) treated. 5, NaAsO2 (12 mg./kg. b wt.) + Free QC treated. 6, NaAsO2 (12 mg./kg. b wt) + Liposomal QC treated. * Indicates significant difference from normal, ** Indicates significant difference from NaAsO2 (6mg./kg. b wt) treated. In both the cases P values were <0.001.
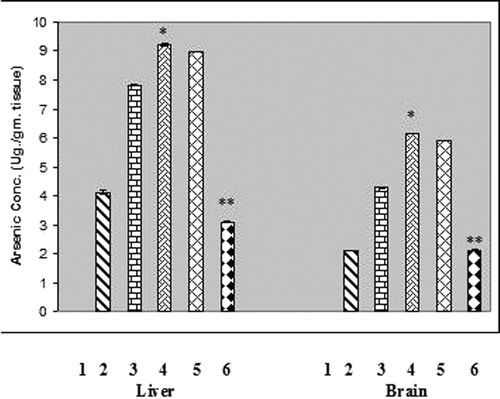
Effect of liposomal quercetin treatment on hepatic 4-HP content in NaAsO2-treated rats.
Chronic NaAsO2 (6 mg/kg. b. wt., 9 mg/kg. b. wt. and 12mg/kg. b. wt.) oral treatments induced an appreciable increase in hepatic 4-HP (). Little reduction of 4-HP was noticed by the treatment of free quercetin, whereas appreciable content of generation of 4-HP was observed when rats were treated with liposomal quercetin ().
Table 3. Effect of liposomal Quercetin instead of Effect of Liposomal Quercetin
Effect on liposomal quercetin on cytochrome c protein expression in liver and brain tissues of NaAsO2-treated rats.
Chronic arsenic treatment resulted in the release of cytochrome c protein in liver and brain tissues of experimental rats () as observed from Western blot analysis. Free quercetin hardly could check the increased release of the protein whereas liposomal quercetin could successfully check the release of cytochrome c ().
Figure 3. Western Blot analysis of cytochrome c protein expression in liver (A) and brain (B) tissues of experimental rats (each group containing 6 rats). 1. Normal, 2. NaAsO2 treated, 3. NaAsO2+NPQC treated, 4. NaAsO2+NPDMSA treated, 5. NaAsO2+NP (QC+DMSA) treated. M, protein marker. Histogram showing mean pixel intensities (arbitrary units) of five immunoblots performed with different individual rats (n = 6). * Indicates significant difference from normal, ** Indicates significant difference from arsenic treated control. In both the cases P values were <0.001.
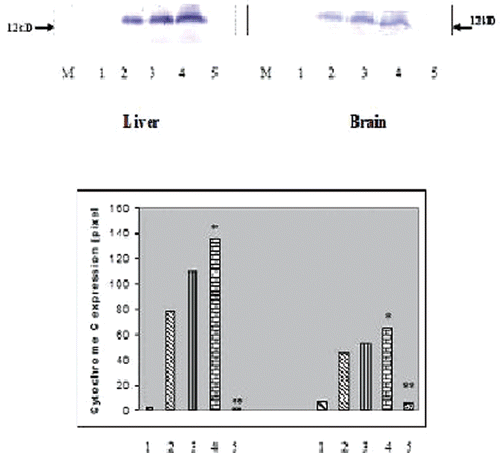
Histopathology of liver and brain tissues of experimental rats.
Hematoxylin and eosin stained liver sections of normal rats showed () the cords of normal hepatocytes, normal looking sinusoids lined by Kupffer cells. Central veins are normal. The histology was within normal limits, but the positive histological changes in the areas of hepatocellular and fatty metamorphosis, branched blood vessels showing anastomosis, Kupffer cell hyperplasia, and localised fibrosis in the periportal region resulted due to chronic oral treatment of arsenic (12 mg./kg. b. wt) for four consecutive months (). Post oral treatments with free quercetin hardly showed any drastic improvements (). The normal histological feature of the cells was almost restored in rats treated with liposomal quercetin ().
Figure 4. Histopathological examination of Hematoxylin and eosin stained liver section of normal and experimental rats (each group containing 6 rats). with magnification × 400. A. Normal, B. NaAsO2 treated (6 mg./kg. b wt.) C. NaAsO2 (9 mg./kg. b wt.) treated, D-E. NaAsO2(12 mg./kg. b wt.), F. NaAsO2+Free QC treated, G. NaAsO2+Liposomal QC treated, ↓↓, fibrosis; ↓, congested central vein; ↓, Fatty metamorphosis.
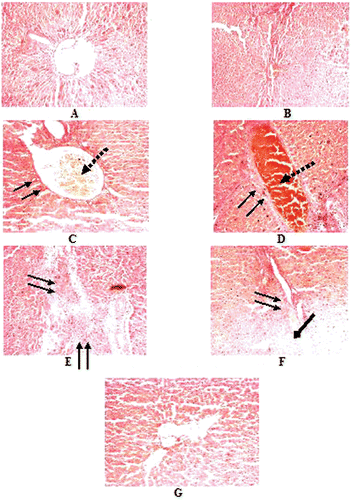
Brain sections of normal rats stained with Hematoxylin and Eosin exhibited normal distribution of pyramidal nerve cells (). The histology was within normal limits, but sections from the rats treated with NaAsO2 () showed significant nerve cell loss. Clustering of nerve cells due to cell loss was also featured in NaAsO2 treated rats given s.c. treatments of free quercetin (). Regular nerve cell distribution was observed in brain sections of rats given s.c. treatments of liposomal quercetin ().
Figure 5. Histopathological examination of Hematoxylin and eosin stained Brain sections of normal and experimental rats (each group containing 6 rats) with magnification × 400. A. Normal, B. NaAsO2 treated (6 mg./kg. b wt.) C. NaAsO2 (9 mg./kg. b wt.) treated, D. NaAsO2(12 mg./kg. b wt.), E. NaAsO2+Free QC treated, F. NaAsO2+Liposomal QC treated. ↓, nerve cell loss, ↓↓, clustering of nerve cells.
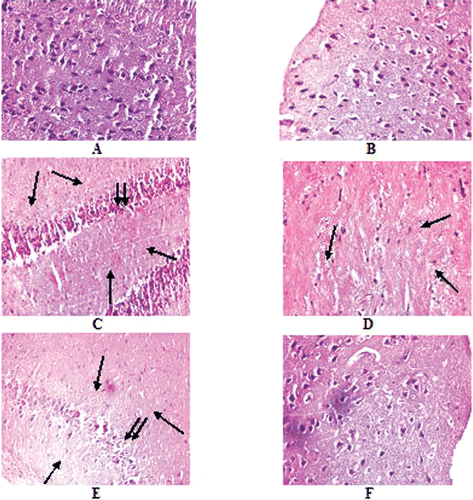
Discussion
Chronic exposure to inorganic arsenic (iAs) by use of contaminated water supplies occurs worldwide, producing a wide range of adverse health effects (CitationChen et al. 2005.). Arsenic has a direct toxic effect on cellular respiration in liver mitochondria with an evidence of oxidative stress and hepatic collagenesis in human (CitationPi et al. 2002; CitationDas et al. 2005). Hepatic fibrosis is a disease state characterized by exuberant synthesis and deposition of collagen in the extracellular matrix. Fibrogenesis is expressed by an increase in the hepatic hydroxyproline levels (CitationTesta et al. 1993). Besides, in the peripheral nervous system, chronic arsenic exposure leads to peripheral neuropathy.
A greater number of drugs including arsenic specific antidotes have been tested to reduce hepatic damage or necrosis and to inhibit liver fibrogenesis. However, none of them is liver-specific or cell type-specific (CitationGuha Mazumder et al. 2001). Quercetin, a polyphenolic flavonoidal compound has been suggested in preventing the development of hepatic fibrosis (CitationLee et al. 2003). It is also known to reduce toxicant-induced liver damage (CitationPeres et al. 2000).
The development of new drugs for the brain has not kept pace with the progress in the molecular neurosciences because most of the drugs do not cross the blood brain barrier (BBB) endothelial cells (EC). It completely restricts flow of water-soluble components from peripherial blood into central nervous system and decrease concentrations of components by the action of enzymes or P-glycoprotein mediated efflux pump (CitationChen et al. 2004). Some of the drugs that show potent antifibrotic activities in vitro often show only minor effects in vivo because of insufficient concentrations of drugs accumulating around the target cell and their adverse effects as a result of affecting other non-target cells (CitationFeng et al. 2009).
Many drugs including arsenic specific antidotes have been tested to reduce hepatic or neuronal cell damage (CitationGuhaMazumder et al. 2001). During chronic arsenic exposure, arsenic gains access into the cell and it becomes mandatory for a drug to cross cell membrane to chelate the intracellular arsenic. Hepatoprotective effect of a single injection of galactosylated liposomal quercetin on acute arsenite treated rats has been already studied by us in our previous observation (CitationMandal et al. 2007). The same observation was made by CitationDatta et al., 2003. They demonstrated that the liposome-entrapped compound interacts with target cells at a much faster rate than that of free components. Treatment with quercetin has been shown to prevent liver damage and suppress overexpression of iNOS, which is induced by various inflammatory stimuli (CitationPavanato et al., 2003). In addition, studies have shown that quercetin can chelate transition-metal ions, including iron, thus preventing iron-catalyzed Fenton reaction (CitationFerrali et al., 1997). Besides, it is widely accepted that the beneficial effects of quercetin against arsenic toxicity are mainly due to its antioxidant properties and also due to regulation of signalling pathway. The preventive role of quercetin encapsulated in nanoparticles against NaAsO2-induced acute liver and neuronal toxicity in rats has been shown in our previous work (CitationGhosh et al. 2009) where a single oral dose of nanoformulation was found to be effective against acute arsenic toxicity. Inorganic arsenic when administered for a longer duration (chronic arsenic treatment) of time gets metabolized by a process involving a two-electron reduction of pentavalent arsenic to trivalent arsenic, exerting more oxidative pressure to cellular macromolecules. But acute toxicity of arsenic is related to its chemical form and oxidation state. Acute toxicity of trivalent arsenic is greater than its pentavalent form. Administration of acute pentavalent arsenic causes no such oxidative attack on cellular components.
However, a single oral dose of nanoencapsulated quercetin might not serve the purpose of treating almost irrevocable pathological manifestations of chronic arsenicosis. Herein, we demonstrated the increased hepatoprotective as well as neuroprotective effects of long term administration (s.c.) (for four consecutive months, twice a week) of liposomal quercetin against chronic arsenite-induced oxidative damage in adult female rats.
The present study displays arsenic-induced reductions in the activities of antioxidant enzymes, SOD and catalase in liver and brain tissues of the rats (). Upon treatment with liposomal quercetin the levels of the antioxidant enzymes were increased significantly. The glutathione level decreased significantly in the liver and brain by the induction of arsenic (). The depletion of the glutathione level either in the liver or brain exerts a potential threat of further oxidative attack as it maintains the cellular redox status and plays an important role in protecting against arsenic toxicity. The depletion in GSH and consequent oxidative damage in neuronal cells has been suggested to be an indication of apoptotic cell death. The loss of GSH triggers the activation of neuronal 12-lipoxygenase, which leads to the production of peroxide, an influx of calcium, and ultimately cell death (Schultz et al., 2000). The higher reduction of GSH observed in the liver as well as in the brain () may be due to higher arsenic accumulation in the liver ().
ROS induces lipid peroxidation and thus generates lipohydroperoxides in biological systems. In our observations, chronic NaAsO2 treatment leads to increase in conjugated diene formation in liver and brain tissues of rats (). A significant reduction in the diene level in both liver and brain tissues was observed upon treatment with liposomal quercetin ().
Arsenic has a direct toxic effect on cellular respiration in liver mitochondria with an evidence of oxidative stress and hepatic collagenesis in human (Yamauchi et al. 2002; CitationMandal and Das 2005). Fibrogenesis is expressed by an increase in the hepatic hydroxyproline levels (CitationTesta et al. 1993). The exact mechanism of fibrogenesis by arsenic is not known. Arsenic induces the production of lactic acid because of an imbalance in cellular energy metabolism, and the enol acid thus utilized by cells significantly increases the intracellular proline pool and collagen synthesis by stimulating the activity of prolyl hydroxylase. The index of intrahepatic proline level increased significantly when compared to normal by the induction of arsenic and it reduced significantly by liposomal quercetin treatment ().
Recent progress in studies on apoptosis revealed that cytochrome c is a pro-apoptotic factor. It is released from its places on the outer surface of inner mitochondrial membrane at the early steps of apoptosis and combining with some cytosolic proteins, activates conversion of the latent apoptosis promoting procaspase-9 to its active form (CitationMartin et al. 2002). Studies have shown that critical mitochondrial alterations lead to cytochrome c release during arsenic induced apoptosis in mouse embryonic fibroblasts (CitationBustamante et al. 2005). A positive correlation between arsenic accumulation and cytochrome c release from hepatic and brain cells could be made from our observation ( and ).
Thus our present report suggests that the mechanism of the protective effect of liposomal quercetin against NaAsO2 induced cytochrome c release () from liver and brain tissues of experimental rats could be related primarily to the reduction of arsenic deposition in both the organs ().
Besides, protective role of liposome encapsulated quercetin against NaAsO2 induced hepatotoxicity and neurotoxicity has also been confirmed by our histopathological analysis ().
Conclusion
The oral application of the herbal polyphenolic compound quercetin in a liposomal drug delivery system might be useful therapeutically to prevent chronic arsenic induced hepatic and cerebral oxidative damage. Further studies are planned to perform targeted drug delivery in chronic arsenic induced oxidative damage by way of receptor mediated endocytosis. This might help in exploring new therapeutic potential of liposome mediated drug delivery.
Acknowledgement
The Indian Council of Medical Research (ICMR), Ad-hoc Project number 58/17/2003-BMS, Government of India, and the Council of Scientific and Industrial Research (CSIR), Government of India, New Delhi supported this work.
Declaration of Interest
The authors have no declaration of interest.
References
- Aebi H. (1984). Catalase in vitro. Methods in Enzymology, 105, 121–126.
- Bustamante J, Leta N, Onreniu S, Gogvadze V. (2005). Arsenic stimulates release of cytochrome c from isolated mitochondria via induction of Mitochondrial permeability transition. Toxicol Appl Pharmacol, 207, 110–6.
- Celik I, Gallicchio L, Boyd K, Lam TK, Matanoski G, Tao X, Shiels M, Hammond E, Chen L, Robinson KA, Caulfield LE, Herman JG, Guallar E, Alberg AJ. (2008) Arsenic in drinking water and lung cancer: a systematic review. Environ. Res. 108, 48–55.
- Chen CJ, Hsu LI, Wang CH, Shih WL, Hsu YH, Tseng MP, et al. (2005). Biomarkers of exposure, effect, and susceptibility of arsenic-induced health hazards in Taiwan. Toxicol Appl Pharmacol, 206, 198–206.
- Chen Y, Dalwadi G, Benson HA. (2004). Drug delivery across the blood brain barrier. Curr Drug Deliv, 1, 361–376.
- Coyle JT, Puttfarken P. (1993). Oxidative stress, glutamate and neurodegenerative disorders. Science, 262, 689–695.
- Das S, Santra A, Lahiri S, Guhamajumder DM. (2005). Implications of oxidative stress and hepatic cytokines (TNF-alpha and IL-6) response in the pathogenesis of hepatic collagenesis in chronic arsenic toxicity. Toxicology and Applied Pharmacology, 204, 18–26.
- Datta N, Mukherjee S, Das L and Das PK. (2003). Targeting of immunostimulatory DNA cures experimental visceral leishmaniasis through nitric oxide up regulation and T-cell activation. Eur J Immunol, 33, 1508–1518.
- Derksen JTP, Morselt HWM, Scherphof GL (1988) Uptake and processing of immunoglobulin-coated liposomes by subpopulations of rat liver macrophages. Biochim Biophys Acta 971:127–136.
- Feng Li & Wang Ji-yao. (2009). Targeted delivery of drugs for liver fibrosis. Expert Opinion on Drug Delivery, 6, 531–541.
- Ferrali M, Signorini C, Caciotti B, Sugherini L, Ciccoli L, Giachetti D, Comporti M. (1997). Protection against oxidative damage of erythrocyte membrane by the flavonoid quercetin and its relation to iron chelating activity. FEBS Lett, 416, 123–129.
- Flora SJS, Dube SN, Arora U, Kannan GM, Malhotra PR. (1995). Therapeutic potential of meso 2,3 dimercaptosuccinic acid or 2,3-dimercaptopropane 1-sulphonate in chronic arsenic intoxication in rats. Biometal, 8, 111–116.
- Ghosh A, Mandal AK, Sarkar S, Panda S, Das N. (2009). Nanoencapsulation of quercetin enhances its dietary efficacy in combating arsenic-induced oxidative damage in liver and brain of rats. Life Sciences, 84, 75–80.
- Gregoriadis G, Poste G. (1988). Targeting of Drugs, Anatomical and Physical considerations, Plenum Press, NY.
- Griffith OW. (1980). Determination of glutathione and glutathione disulfide using glutathione reductase and 2-vinylpyridine. Analytical Biochemistry, 106, 207–212.
- Guha Mazumder DN, De BK, Santra A, Ghosh N, Das S, Lahiri S, Das T. (2001). Randomized placebo controlled trial of 2,3-dimercapto-1-Propanesulphonate (DMPS) in therapy of chronic arsenicosis due to drinking arsenic contaminated water. Clinical Toxicology, 39, 665–674.
- Guha Mazumder DN, Das Gupta J, Chakraborty AK, Chatterjee A, Das D, Chakraborti D. (1992). Environmental pollution and arsenicosis in South Calcutta. Bull World Health Organ, 70, 481–485.
- Lee ES, Lee HE, Shin JY, Yoon S, Moon JK. (2003). The flavonoid quercetin inhibits dimethyl nitrosamine-induced liver damage in rats. Journal of Pharmacy and Pharmacology, 55, 1169–1174.
- Li SX, Zhang CL, Zhao XL, Yu SF, Xie KO. (2006). Effect of subchronic exposure to acrylamide induced on the expression of bcl-2, bax and Caspase-3 in the rat nervous system. Toxicology, 217, 46–53.
- Lin Y, Li HB, Huang JT. (2005). Following the fate of murine epidermal Stem cells in a syngeneic dermal equivalent in vivo. Zhong Zheng Xing Wai Ke Za Zhi 21, 452–456.
- Lopez E, Pozas E, Rivera R, Ferrer I. (1999). Bcl-2, Bax and Bcl-x expression Following kainic acid administration at convulsant doses in the rat. Neuroscience, 91, 1461–1470.
- Lowry OH, Rosebrough NJ, Farr Al Randall, RJ. (1951). Protein measurement with the folin phenol reagent. Journal of Biological Chemistry, 193, 265–275.
- Mandal AK, Das S, Basu MK, Chakraborti RN, Das N. (2007). Hepatoprotective activity of liposomal flavonoid against arsenite-induced liver fibrosis. Journal of Pharmacology and Experimental Therapeutics, 320, 994–1001.
- Mandal AK, Das N. (2005). Sugar coated liposomal flavonoid: a unique formulation In combating carbontetrachloride induced hepatic oxidative damage. Journal Of Drug Targeting, 13, 305–315.
- Maniatis T, Jeffrey A, Kleid DG. (1975). Nucleotide sequence of the rightward operator of phage lambda. Proc Natl Acad Sci USA, 72(3), 1184–1188.
- Marklund S, Marklund G. (1974). Involvement of the superoxide anion radical in The autoxidation of pyrogallol and a convenient assay for superoxide dismutase. Eur J Biochem, 47, 469–74.
- Martin O, Robertson JD, Gogradze V, Zhivotovsky B, Onrenius S. (2002) Cytochrome c release from mitochondria proceeds by a two-step process. PNAS, 99(3), 1259–1263.
- Micklus MJ, Greig NH, Tung J, Rapport SI. (1992). Organ distribution of liposomal formulations following the intra carotid infusion in rats. Biochem. Biophys. Acta, 1124, 7–12.
- Mitra M, Mandal AK, Chatterjee TK, Das N. (2005). Targeting of mannosylated liposome incorporated benzyl derivative of Penicillium nigricans derived compound MT81 to reticuloendothelial systems for the treatment of visceral leishmaniasis. J. Drug Target, 13, 285–293.
- National Research Council. Arsenic in drinking water. 2001 Update. Washington, D.C.: National Academy Press. Tsai, S-M, T-N Wang and Y- C Ko, 1999.
- Pavanato A, Tunon MJ, Sanchez-Campos S, Marroni CA, Llesuy S, Gonzalez-Gallego J, Marroni N. (2003). Effects of quercetin on liver damage in rats with carbon tetrachloride-induced cirrhosis. Dig Dis Sci, 48, 824–829
- Peres W, Tunon MJ, Collado PS, Herrmann S, Marroni N, Gonzalez-Gallego J. (2000). The flavonoid quercetin ameliorates liver damage in rats with biliary obstruction. Journal of Hepatology, 33, 742–750.
- Pi J, Yamauchi H, Kumagai Y, Sun G, Yoshida T, Aikawa H. (2002). Evidence for Induction of oxidative stress caused by chronic exposure of Chinese residents to arsenic contained in drinking water. Env Health Perspect, 110, 331–336.
- Recknagel RO, Glende Jr EA. (1984). Spectrophotometric detection of lipid conjugated dienes. Methods in Enzymology, 105, 331–337.
- Sarin SK, Sharma G, Banerjee S, Kathayat R, Malhotra V. (1999). Hepatic fibrogenesis using chronic arsenic ingestion: studies in a murine model. Ind. J. Exp. Biol, 37, 147–151.
- Schulz JB, Lindenau J, Seyfried J, Dichgans J. (2000). Glutathione, oxidative stress and neurodegeneration. Eur. J. Biochem, 267, 4904–4911,
- Sing D, Chander V, Chopra K. (2004). The effect of quercetin, a bioflavonoid on ischemia/repurfusion induced renal injury in rats. Archives of Medical Research 35, 484–494.
- Sinha J, Das N, Basu MK. (2000). Targeting of glycoside grafted liposomes to specific hepatocellular sites. J Surface Sci Technol, 16, 200–209.
- Testa R, Bindi P, Risso D, Borzone S, Caglieris S, Bardellini E, rasso AG, Celle G. (1993). Serum prolyl- hydroxylase as an index of fibrogenetic activity in chronic active hepatitis and cirrhosis. Eur J Gastroenterol Hepatol, 5, 103–107.
- Tokyol C, Yilmaz S, Kahraman A, Cakar H, Polat C. (2006). The effects of Desferrioxamine and quercetin on liver injury induced by hepatic ischemia repurfusion in rats. Acta Chirurgica Belgica, 106, 68–72.