Abstract
The purpose of the study is to design and evaluate curcumin loaded gelatin microspheres (C-GMS) for effective drug delivery to the lung. C-GMS was prepared by the emulsification-linkage technique and the formulation was optimized by orthogonal design. The mean encapsulation efficiency and drug loading of the optimal C-GMS were 75.5 ± 3.82 % and 6.15 ± 0.44%, respectively. The C-GMS presented a spherical shape and smooth surface with a mean particle diameter of 18.9 μm. The in vitro drug release behavior of C-GMS followed the first-order kinetics. The tissue distribution showed that the drug concentrations at lung tissue for the C-GMS suspension were significantly higher than those for the curcumin solution, and the Ce for lung was 36.19. Histopathological studies proved C-GMS was efficient and safe to be used as a passive targeted drug delivery system to the lung. Hence, C-GMS has a great potential for the targeted delivery of curcumin to the lung.
Introduction
Lung cancer is the most commonly diagnosed cancer leading to the highest mortality (CitationJemal et al., 2007). Chemotherapy plays an important role in the therapy of lung cancer. Many different drugs or drug combinations have been used for lung cancer treatments. Curcumin (), a hydrophobic polyphenol extracted from the rhizome of plants, which belong to Zingiberaceae, Curcuma Longa (such as turmeric), has multiple anticancer activities (CitationAnand et al., 2007; CitationAnand et al., 2008; CitationMaheshwari et al., 2006). Recent studies have proven that curcumin can lead to the apoptosis of human lung cancer cell lines---A549 and H1299 (Radhakrishna et al., 2004), reduce the number of lung tumor nodules, and increase the life span of experimental animals (CitationMenon et al., 1995). Moreover, curcumin has been shown to be extremely safe even at a very high dose in various animal models and human studies. Therefore, it possesses a great potential in the treatment of lung cancer (Radha et al., 2007).
Though curcumin has multiple pharmacological activities, there are still some problems such as chemical instabilities in developing formulations for clinical application. At neutral to basic pH conditions, the structure of curcumin is destroyed because the proton shifts from the phenolic group. Due to this problem, a large amount of curcumin is decomposed in the metabolism process (CitationWang et al., 1997). Preclinical and clinical pilot studies suggested that concentrations of curcumin in plasma and target tissues were very low (CitationAnand et al., 2007; CitationPan et al., 1999; SharCitationMa et al., 2007). Therefore, the traditional preparations, such as injection and tablet, cannot effectively protect drug and delivery drug to the target site at therapeutic concentration. Nowadays, there is a lot of interest in the development of alternative dosage forms to overcome these problems.
A promising way of solving these problems is targeting preparations, which can change the distribution of drugs. This is done by targeting specific organs or cells and increasing the drug concentration in the target site while simultaneously decreasing the amount distributed to other parts of the body. Microspheres have emerged as a remedial measure to improve site-specific drug delivery to a considerable extent. It has been reported that biodegradable polymeric microsphere occupies many advantages including site-specific or organ-directed targeting, protection of drug from vivo environment, and improved pharmacokinetics/pharmacodynamics properties (CitationKawai et al., 2000; CitationIkada et al., 1998). Gelatin is a natural polymer derived from collagen, which is commonly used for pharmaceutical and medical applications because of its biodegradability and biocompatibility in physiological environment. When drug is encapsulated in gelatin microspheres, not only can the drug target to the specific tissues, but also the drug stability increases significantly (CitationBatycky et al., 1997; CitationFreiberg et al., 2004; Simon et al., 2005). To date, curcumin loaded gelatin microspheres (C-GMS) for lung targeting has not been reported.
In the present study, the preparation, optimization, characterization, crystalline state, in vitro release behavior, and stability test of C-GMS were described. In addition, the targeting potential to the lung tissue in vivo was evaluated by tissue distribution experiment, targeting parameter, and histopathological study.
Materials and methods
Materials
Curcumin was purchased from the Third Reagent Factory of Shanghai (Shanghai, China). A-type gelatin, liquid paraffin, and span-80 were purchased from Guangcheng Reagent Corporation (Tianjin, China). Dialysis bags (MWCO 20000) were purchased from the Luosenbo Corporation (Xi’an, China). All the other used chemicals were of analytical grade.
Preparation of curcumin loaded gelatin microspheres
Curcumin loaded gelatin microspheres (C-GMS) was prepared by emulsification-linkage method. Curcumin was dispersed in pre-swelled gelatin solution (10%-20%, W/V) by stirring at 600 rpm for 5 min to form the aqueous phase. Then, the aqueous phase was dropped into the 55 ± 1°C liquid paraffin containing span-80 and stirred at 1100 rpm to obtain stable water/oil (w/o) emulsion. After emulsifying for 30 min, the system was immediately transferred to the 4°C ice water bath and stirred at 800 rpm for 30 min. Then, 25% glutaraldehyde aqueous solution was added to the system to cross-link the gelatin droplets for 30 min. Isopropyl alcohol was also added to the system to dehydrate the gelatin droplets. The mixture was dried by vacuum filtrations and triple washed by petroleum ether and isopropyl alcohol. Then, the production was dried at 40°C under vacuum condition to remove the residual organic solvent (CitationRahman et al., 2006; CitationLu 1998, CitationMathew et al., 2007; CitationDinarvand et al., 2005). The important parameters of preparation would be optimized by orthogonal design as the following part described.
Optimization of C-GMS formulation with orthogonal design
The formulation was optimized by orthogonal design experiment with the encapsulation efficiency (EE) as the index. The effects on EE of mainly relative factors such as the volume ratio of aqueous phase and oil phase (A), the weight ratio of drug and polymer (B), the gelatin concentration (C), and the surfactant concentration (D) were investigated according to the L9 (34) orthogonal design. The factors and levels of the orthogonal design experiment were listed in .
Table 1. Factors and levels of the orthogonal design.
Encapsulation efficiency and drug loading
The accurately weighed amount of C-GMS (10 ± 0.2 mg) was dispersed in 9 mL 80% (v/v) ethanol solution. The system was ultrasonicated for 30 min and centrifuged at 3500 rpm for 15 min. The supernatant was withdrawn and the above operations were repeated three times. Then, the total supernatant was diluted with 80% ethanol solution and filtered through a 0.45 μm filter. The drug content was determined using a UV spectrometer (UV-2102, Unico, China) at 432 nm. EE was calculated as the ratio of the amount of curcumin loaded in microspheres to that added initially (Eq. 1), and the drug loading (DL) was obtained from the ratio of the amount of curcumin loaded in microspheres to the amount of C-GMS (Eq. 2) (CitationPaharia et al., 2007).
From the above equations, “Wloaded” is the amount of curcumin loaded in the microspheres, “Wadded” is the amount of curcumin added initially in the system, and “WC-GMS” is the weight of C-GMS added to the system.
Morphological observation
The morphology of C-GMS was observed by scanning electron microscopy (SEM, S-520 Hitachi, Japan). The samples for SEM were prepared by lightly sprinkling the C-GMS on a double adhesive tape which was stuck to an aluminum stub. The stub was then coated with gold using a sputter coater and observed at 12 kV.
Particle size distribution
C-GMS were dispersed with distilled water and vortexed for 3 min. The particle diameters of 500 microspheres under optical microscope were determined using a micrometer. The average diameter (Dav) was chosen as the mean diameter of C-GMS (CitationPaharia et al., 2007).
Where n1, n2…nn is the particle number and d1, d2…dn is the particle diameter.
Flowability
The flow properties of C-GMS were usually evaluated by the angle of repose and the bulk density. The angle of repose was determined by the fixed-base cone method and the bulk density was measured by cylinder method as previously reported (CitationRahman et al., 2006). Each experiment was performed thrice.
DSC analysis
DSC analysis was performed to provide further information on the drug–polymer relationship using a differential scanning calorimeter (CDR-4P, Shanghai Tianping Instrument Ltd., Shanghai, China). The accurately weighed amount (10 ± 0.2 mg) of the crude curcumin powder, gelatin, mixture of curcumin and gelatin, blank gelatin microspheres, and C-GMS (optimal formulation) were added into aluminum pans and then hermetically sealed with aluminum lids, respectively. The thermograms were obtained at a scanning rate of 10°C/min over a temperature range from 31°C to 400°C (CitationLu et al., 1999).
Stability test
The desiccated C-GMS were sealed up in brown vials and stored at 3 - 5°C, 15 - 25°C, and 37°C with 75% relative humidity for 3 months. At the end of the storage period, C-GMS was evaluated by physical appearance, surface morphology, and drug content.
In vitro release study
The in vitro release behaviors of C-GMS and curcumin were investigated by dialysis method with 40% ethanol physiological saline as the dissolution medium. C-GMS (20 ± 0.2 mg) and the equivalent amount of curcumin were accurately weighed and gently placed into dialysis bags, respectively. The bag was then placed into a 200 mL dissolution vessel containing 100 mL of dissolution medium with a rotating rate of 100 rpm in a 37 ± 0.5°C water bath. 3 mL of dissolution medium was withdrawn at predetermined time intervals and the same volume of fresh release medium was added to maintain a constant volume. The concentrations of curcumin in dissolution medium were determined by UV at 432 nm. All experiments were carried out in triplicates (CitationEsposito et al., 1995; CitationMladenovska et al., 2002).
Tissue distribution study
The study was approved by Animal Care and Use Committee of the People’s Republic of China. Tissue distribution study was performed in rabbits to evaluate the targeting potential of C-GMS. Thirty three healthy rabbits with similar weights (2.5 ± 0.5 kg) were selected. These animals were kept in well-spaced ventilated cages and were maintained in healthy and fixed diets. All animals were kept on starvation 12 h with free access to water before injection. The animals were divided into two groups. The first group containing 15 rabbits served as control, in which curcumin solution prepared with dimethylacetylamide (15%), polyethyleneglycol 400 (45%) and 5% dextrose (40%) as the co-solvents based on the previous report (CitationMa et al., 2007) was injected via the ear vein at a dosage of 3 mg/kg. The second group containing 18 rabbits was injected C-GMS suspension through the same route at a dosage equivalent to the plain drug of 3 mg/kg. After administration, blood samples were collected via the ear vein and placed into heparinized test tubes at predetermined time intervals. The rabbits were then sacrificed (during one minute) immediately and the heart, liver, spleen, lung, and kidney were isolated.
Plasma was separated from blood by centrifugation at 3500 rpm for 15 min. Tissues were washed by normal saline and precisely weighed. 1 g of each tissue was taken and homogenized with the proper amount of normal saline (weight ratio of tissue to normal saline was 1:4 for lung and 1:2 for other tissues) to obtain a homogenate. Then, 20 μL of hydrochloric acid (0.2 mol/L) and 3 mL of acetidin were added to 0.5 mL of plasma, or 20 μL hydrochloric acid (0.2 mol/L) and 6 mL acetidin were added to 1 mL tissue homogenate, respectively (CitationMa et al., 2007). The mixture was vortexed for 3 min and centrifuged for 15 min at 3500 rpm. The supernatant was dried under a steam of 45°C nitrogen. The dry sample was redissolved in the proper amount of methanol (blood and lung, 500 μL; other tissues, 250 μL) and centrifuged at 10000 rpm for 10 min. Then, the supernatant (20 μL) was taken and injected into HPLC for analysis (CitationJain et al., 2007).
HPLC analysis
HPLC system (Agilent 1120, Agilent Co., USA) with column C18 (4.6 mm×250 mm, 5μm) was used for the analysis of curcumin in plasma and tissues. The mobile phase consisted of methanol/water (containing 3.6% glacial acetic acid) at the ratio of 73:27 at a flow rate of 1.0 mL/min. The column temperature was room temperature and the analysis wavelength was set at 428 nm.
Evaluation of lung-targeting ability
The targeting parameter Ce was used to evaluate the lung-targeting characteristic. The value of Ce was calculated using the following equation (CitationSahu et al., 2008; CitationLim et al., 2001):
From the above equation, Cmax represents the peak concentration, p represents the microsphere group, and s represents the control group.
Histopathological examination by light microscopy
After 15 min of the administration, animals of both groups were sacrificed and the lung were dissected and washed with cold normal saline. The lungs were pressed between filter pads and fixed in 10% neutral formalin using standard techniques. Then they were cut at 5μm thickness and stained with hematoxylin and eosin for histopathological examination. The lung tissue samples were examined under light microscopy with 400×magnification (CitationNanji et al., 2001).
Data analysis
Data was statistically analyzed by Student’s t test. Statistical significance was evaluated at 95% confidence interval (p < 0.05). The mean and standard deviation of the results were reported.
Results and discussion
Preparation and optimization of C-GMS
The commonly used cross-linking agents were formaldehyde and glutaraldehyde. Glutaraldehyde was chosen as the cross-linking agent because it could solidify in neutral conditions while formaldehyde could only solidify completely in basic conditions, in which curcumin was very easy to decompose (CitationZeng et al., 1996, CitationEsposito et al., 1996). The function of the cross-linking agent was to solidify and precipitate the microspheres from water, improve the thermal and mechanical stability of the microspheres under physiological conditions, and control the rate of degradation in vivo (CitationTabata et al., 1999).
Given the pharmaceutical and pharmacological advantages of high EE, EE was chosen as the index. EE can be improved through the optimization of formulation and preparation technology. The parameters affecting EE in the production of C-GMS, including stirring speed, emulsification temperature, and emulsification time, were investigated based on a previous report (CitationWang et al., 2003). The final optimal parameters were as follows, stirring speed at 1100 rpm, emulsification temperature at 55 ± 1°C, and emulsification time at 30 min.
Furthermore, the formulation factors affecting EE were optimized with orthogonal design and the results were shown in . The range (R) reflected the effect of each factor on EE. A bigger R meant the factor had a greater effect on EE. Since RD > RA > RB > RC, the order of the factors affecting EE were as follows: D > A > B > C. K1, K2, and K3 represented the mean EE value of each level. A higher EE could be achieved in the level that had a higher mean value. As seen from , the sequences of different level for the four factors A, B, C, and D affecting on EE were 2 > 3>1, 3 > 2>1, 3 > 1>2, and 3 > 2>1, respectively. The best experimental condition was the combination of the optimal level for the set factors. The optimal formulation combination was A2B3C3D3, namely, the volume ratio of aqueous phase to oil phase was 1:5 (10 mL:50 mL), the weight ratio of drug to polymer was 20:200 (100 mg: 1000 mg), the gelatin concentration was 10% (w/v) (1 g:10 mL), and the surfactant concentration was 1% (w/v) (4.5 g: 45 mL). Based on the obtained conditions, three batches of C-GMS were prepared and the average EE of the optimized formulation was 75.5 ± 3.82 % and DL was 6.15 ± 0.44%.
Table 2. Result of the orthogonal design experiment.
The consistency of the gelatin solution had a relatively great influence on EE. In the study, it was found when the gelatin concentration was over 20%, the solution was so viscous that the gelatin drops were hard to disperse into the organic phase, inducing the adhesion of microspheres or the enlarged particle diameter, thus the drug could not be efficiently entrapped in the microspheres, which was in accordance with the previous reports (CitationGhaderi et al., 1996, CitationRaouf et al., 1996).
The volume ratio of oil to aqueous phase played a role in the formation of microspheres. When the volume of oil phase was increased to 5-fold that of aqueous phase, the collision chance among gelatin drops in the oil phase was significantly reduced and the EE could be obviously improved. However, if too much oil phase (6-fold aqueous phase) was added, some curcumin would scatter to the oil phase resulting in the decrease of EE (CitationIwanaga et al., 2003).
Additionally, the weight ratio of drug to polymer was also an impact factor on the EE. In our study, the EE increased when the weight ratio of drug to polymer changed from 10:200 to 20:200. But when drug was added over ten percent of the polymer, some of the drug could not be encapsulated in the microspheres. This result might indicate that such amount of drug exceeded the loading capacity of the polymer microspheres (CitationPaharia et al., 2007, CitationJain et al., 2007).
Morphology
C-GMS obtained according to the optimal formulation were free flowing, yellow colored powder (shown in ). SEM revealed that C-GMS were discrete and spherical in shape with a smooth surface (shown in ), which might be due to the function of cross-linking agents to stabilize emulsion droplets and hence resulted in well-formed microspheres.
Particle size distribution
The average particle size and size distribution were extremely important because they had great influences on the targeting ability in the lung (CitationYoshioka et al., 1981). Previous reports pointed out that the microspheres with the size range of 5− 25 μm had a notable lung targeting efficacy (CitationKanke et al., 1980). The mean particle diameter was 18.9 μm, and 86.6% of the C-GMS was in the range of 5− 30 μm as shown in . This result suggested that the C-GMS might mainly accumulate in the lung after intravenous administration.
Flowability
The average value of the angle of repose was 41.2°. The average bulk density was 0.583 g/mL. C-GMS showed excellent flowability. The angle of repose can reflect not only the flowability but also the roundness of the microsphere indirectly.
DSC analysis
DSC was very useful in the investigation of the thermal properties of microspheres, providing both qualitative and quantitative information about the physicochemical state of drug inside the microspheres (CitationLv et al., 2009, CitationRahman et al., 2006). There would be no detectable endothermic peak if the drug was present in a molecular dispersion or solid solution state in the polymeric microspheres (CitationMu et al., 2001). DSC thermograms of crude curcumin, gelatin, physical mixture of curcumin and gelatin, blank gelatin microspheres, and C-GMS were shown in . The crude curcumin showed a sharp endothermic peak at 185.5°C while in the physical mixture, the endothermic peak of curcumin appeared at 180.1°C, and 182.5°C for that of curcumin in C-GMS. The change for endothermic peak of curcumin in the mixture or microsphere system was due to the presence of gelatin. It could be concluded from the results that there was no the significant reaction between curcumin and gelatin, curcumin was still present in the crystalline form whether in mixture or in microsphere system.
Stability test
No significant changes were found in the physicochemical parameters containing physical appearance, surface morphology, and drug content after storing at 3 - 5°C, 15 - 25°C, and 37°C with 75% relative humidity for 3 months compared with the original data. The stability of curcumin entrapped in microspheres was significantly improved. Considering that curcumin was sensitive to light, C-GMS should be preserved in low temperature or away from light in room temperature.
In vitro release study
The release profiles of C-GMS and curcumin solution were shown in . The release process of C-GMS fit the first-order kinetics equation, ln (100-Q) = -0.0298t+4.5454 (R = 0.9984), t1/2 = 21.25 h. While the curcumin solution fit the Higuchi equation, Q = 56.187t1/2 + 5.5575 (R = 0.9830), t1/2 = 0.626h. The t1/2 of C-GMS was obviously prolonged compared with the curcumin solution. The drug release of curcumin solution was very fast, with approximately 98% of the curcumin released within 3 h. In contrast, only 14% of curcumin was released from C-GMS within 3 h. The drug release at the initial time was due to the presence of quite a little amount of curcumin dispersed on the surface of the microspheres (CitationMuvaffak et al., 2004). Subsequently, C-GMS exhibited a sustained release property and the accumulated drug release percentage at 48 h was 77%.
The in vitro release of drug-loaded gelatin microspheres mainly depended on three mechanisms: the diffusion of drug through the matrix, the degradation of the polymer, the swelling or dissolution of the polymer. The present system for in vitro release study contained no lysozyme and proteolytic enzymes. And the complete degradation of gelatin microspheres need about two months under the condition of pH >7.0. Therefore, the release mechanisms for C-GMS should mainly be drug diffusion and swelling of the polymer. These factors determined that the gelatin microspheres presented a sustained release property (CitationMu et al., 2001).
Tissue distribution study
For curcumin solution and C-GMS suspension, the drug concentrations of blood, heart, liver, spleen, lung, and kidney at different time intervals were shown in and . Our results proved a completely different distribution of the C-GMS compared to the curcumin solution. The tissue distribution study revealed the reliability of the optimal formulation in achieving the lung targeting effect.
Figure 7. Drug distribution in various tissues following intravenous administration of curcumin solution.
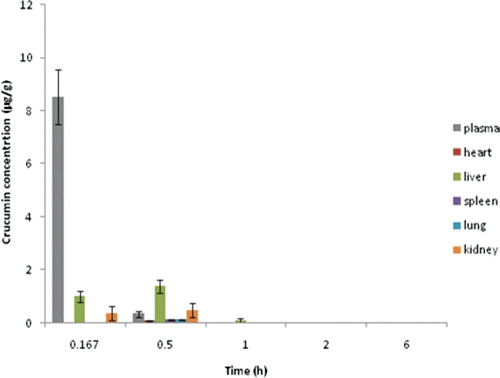
Figure 8. Drug distributions in various tissues following intravenous administration of C-GMS suspension.
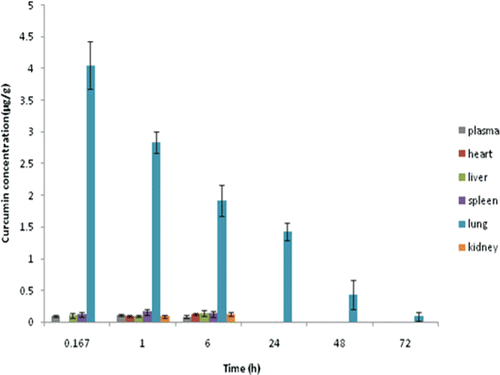
In the case of curcumin solution, most of the drug was distributed in blood. After 3 h from injection, the drug almost could not even be detected in tissues. This indicated that the drug was eliminated very fast and could not concentrate in the lung. For C-GMS suspension, most of the drug was delivered to the lung tissue as shown in . The drug concentration in lung obviously increased and was much higher than other tissues. Also, the drug concentration in lung could be kept at a relatively high level for a long time (48 h). This indicated that the C-GMS could efficiently target to lung and had a good sustained release property. Meanwhile, the drug concentrations in the liver and kidney decreased as compared with the control group, which could improve the treatment efficiency of curcumin for lung diseases and decrease the side effects in non-target sites.
The particle diameter of microspheres played a dominant role in achieving the targeting ability of microspheres. After intravenous injection, microspheres of 7 μm or more in diameter were generally accepted to be rapidly entrapped in the lung by mechanical filtration whereas microspheres with a diameter of less than 5μm were predominantly taken up by cells of the reticuloendothelial system in the liver. As to microspheres with a diameter near 5μm to some extent, they were considered to exhibit the initial distribution in the lung. However, such trapped microspheres were soon washed out from the lung (CitationIllum et al., 1982, CitationKanke et al, 1980).
The particle diameter of most C-GMS was in the range of 5 - 30 μm. When injected through the vein, the C-GMS whose size was larger than 5 μm allowed the efficient uptake in the lung because they could be mechanically trapped by the lung capillary network. Moreover, the curcumin could be embedded into the matrix by the incorporation into the microspheres, thus reducing its exposure to body fluid. Subsequently, the curcumin could be concentrated in the lung resulting in the improved drug concentration in the diseased region and a relatively low toxicity or side-effect on the other non-target parts (CitationYoshioka et al., 1981, CitationPrior et al., 2000).
Evaluation of lung-targeting ability
The extent change in drug distribution of the preparation can be evaluated by the targeting parameter Ce. A high value of Ce indicated that the effect of changing the distribution of drug was obvious. Ce values for different tissues were shown in . The Ce for lung was 36.19, which was much higher than that for other tissues. This result indicated the C-GMS obviously changed the distribution of curcumin and delivery most of the curcumin to the lung.
Table 3. Ce of different tissues (each value represents the mean ± SD, n = 3).
Histopathological examination by light microscopy
Photomicrographs of the lung sections for the control group (A) and the microsphere group (B) after administration 15 min were shown in . C-GMS was present in lung as indicated by the arrows in . From these pictures, it could be concluded that C-GMS were taken up by the pulmonary capillaries. The accumulation of C-GMS in lung was a function of particle size. Thus, the uptake of microspheres by the lung probably took place due to the mechanical filtration caused by the capillary blockade before distribution to the whole body (CitationGallo et al., 1989, CitationIllum et al., 1982). Meanwhile, the cytoarchitecture of the lung tissue of the microsphere group did not show any degenerative changes compared with the control group. Based on these observations, it could be concluded the constituents of the microsphere formulation were efficient and safe to be used as a passive targeted drug delivery system to the lung.
Conclusions
In the present study, C-GMS was successfully prepared by the emulsification-linkage method with a high EE. In vitro release test showed that C-GMS exhibited a sustained release property compared with the control. The C-GMS was preferentially located in the lung tissue and was retained for a long time after intravenous administration. Compared with the curcumin solution, the drug concentration and the accumulated time in the lung tissue were obviously increased while those in non-targeted sites such as heart, kidney, brain, and plasma were much reduced. Based on these results, it can be concluded that C-GMS can be a promising carrier to deliver curcumin to the lung.
Declaration of Interest
The authors report no conflicts of interest. The authors alone are responsible for the content and writing of the paper.
References
- Anand P, Kunnumakkara AB, Newman RA, et al. (2007). Bioavailability of curcumin: problems and promises. Mol Pharm. 4(6): 807–818.
- Anand P, Sundaram C, Jhurani S, et al. (2008). Curcumin and cancer: An “old-age” disease with an “age-old” solution. Cancer Lett. 267(1): 133–164.
- Batycky RP, Hanes J, Langer R, et al. (1997). A theoretical model of erosion and macromolecular drug release from biodegrading microspheres. J Pharm Sci. 86(12):1464–1477.
- Dinarvand R, Mahmoodi S, Farboud E, Salehi M, Atyabi F. (2005). Preparation of gelatin microspheres containing lactic acid-effect of cross-linking on drug release. Acta Pharm. 55(1): 57–67.
- Esposito E, Cortesi R, Nastruzzi C. (1996). Gelatin microsphere: influence of preparation parameters and thermal treatment on chemico-physical and biopharmaceutical properties. Biomaterials. 17(20): 2009–2020.
- Esposito E, Pastesini C, Cortesi R, et al. (1995). Controlled release of 1-/3-D-arabinofuranosylcytosine (ara-C) from hydrophilic gelatin microspheres: in vitro studies. Int. J. Pharm. 117: 151–158.
- Freiberg S, Zhu XX. (2004). Polymer microspheres for controlled drug release. Int. J. Pharm. 282(1-2): 1–18.
- Gallo JM, Gupta PK, Hung CT et al. (1989). Evaluation of drug delivery following the administration of magnetic albumin microspheres containing adriamycin to the rat. J Pharm Sci. 78(3): 190–194.
- Ghaderi R, Struesson C, Carlfors J. (1996). Effect of preparative parameters on the characteristics of poly (d,l-lactideco-glocolide) microspheres made by the double emulsion method. Int. J. Pharm. 141: 205–216.
- Ikada Y, Tabata Y. (1998). Protein release from gelatin matrices. Adv Drug Deliv Rev. 31: 287–301.
- Illum L, Davis SS, Wilson CG, et al. (1982). Blood clearance and organ deposition of intravenously administered colloidal particles: The effects of particle size, nature and shape. Int. J. Pharm. 12: 135–146.
- Iwanaga K, Yabuta T, Kakemi M, et al. (2003). Usefulness of microspheres composed of gelatin with various cross-linking density. J. Microencapsul. 20(6): 767–776.
- Jain A, Gupta Y, Jain SK. (2007). Potential of calcium pectinate beads for target specific drug release to colon. J Drug Target. 15(4): 285–294.
- Jemal A, Siegel R, Ward E, et al. (2007). Cancer statistics, 2007. CA Cancer J. Clin. 57(1): 43–66.
- Kanke M, Simmons GH, Weiss DL, et al. (1980). Clearance of 141C3-labeled microspheres from blood and distribution in specific organs following intravenous and intraarterial administration in beagle dogs. J Pharm Sci. 69(7):755–762.
- Kawai K, Suzuki S, Tabata Y, et al. (2000). Accelerated tissue regeneration through incorporation of basic fibroblast growth factor-impregnated gelatin microspheres into artificial dermis. Biomaterials. 21(5): 489–499.
- Lee J, Im YH, Jung HH, et al. (2005). Curcumin inhibits interferon-alpha induced NF- kappa B and COX-2 in human A549 non-small cell lung cancer cells. Biochem and Biophys Res Communs. 334(2): 313–318.
- Lim ST, Forbes B, Martin GP, et al. (2001). In vivo and in vitro characterization of novel microparticulates based on hyaluronan and chitosan hydroglutamate. AAPS Pharm Sci Tech. 2 (4): E20.
- Lu B, Zhang JQ, Yang H. (1999). Studies on lung targeting gelatin microspheres of carboplatin. Acta Pharmaceutica Sinica. 34: 786–789.
- Lu B. (1998).New Techniques and New Dosage Forms of Drugs. Beijing, China: People’s Health Publishing House.
- Lv Q, Yu A, Xi Y, et al. (2009). Development and evaluation of penciclovir-loaded solid lipid nanoparticles for topical delivery. Int. J. Pharm. 372(1-2): 191–198.
- Ma ZS, Shayeganpour A, Brocks DR, et al. (2007). High-performance liquid chromatography analysis of curcumin in rat plasma: application to pharmacokinetics of polymeric micellar formulation of curcumin. J. Biomed Chromatogr. 21(5): 546–552.
- Maheshwari RK, Singh AK, Gaddipati J, et al. (2006). Multiple biological activities of curcumin: A short review. Life Sci. 78(18): 2081–2087.
- Mathew ST, Devi SG, KV S. (2007). Formulation and Evaluation of Ketorolac Tromethamine-loaded Albumin Microspheres for Potential Intramuscular Administration. AAPS Pharm Sci Tech. 8 (1): E14.
- Menon LG, Kuttan R, Kuttan G. (1995). Inhibition of lung metastasis in mice induced by B16F10 melanoma cells by polyphenolic compounds. Cancer Lett. 95(1-2): 221–225.
- Mladenovska K, Kumbaradzi E, Dodov G, et al. (2002). Biodegradation and drug release studies of BSA loaded gelatin microspheres. Int. J. Pharm. 242(1-2): 247–249.
- Mu L, Feng SS. (2001). Fabrication, characterization and in vitro release of paclitaxel (Taxol) loaded poly (lactic-co-glycolic acid) microspheres prepared by spray drying technique with lipid/cholesterol emulsifiers. J. Control Release. 76(3): 239–254.
- Muvaffak A, Gurhan I, Hasirci N. (2004). Prolonged cytotoxic effect of colchicine released from biodegradable microspheres. J Biomed Mater Res B Appl Biomater. 71(2): 295–304.
- Nanji, AA, Jakelainen, K, Fotouhinia, M, et al. (2001). Increased severity of alcoholic liver injury in female rats: role of oxidative stress, endotoxin and chemokines. Am J Physiol Gastrointest Liver Physiol. 281(6): 1348–1356.
- Paharia A, Yadav AK, Rai G, Jain SK, Pancholi SS, Agrawal GP. (2007). Eudragit-coated Pectin Microspheres of 5-Fluorouracil for Colon Targeting. AAPS Pharm Sci Tech. 8(1): E12.
- Pan MH, Huang TM, Lin JK. (1999). Biotransformation of Curcumin through reduction and glucuronidation in mice. Drug Metab. Dispos. 27(4): 486–494.
- Prior S, Gamazo C, Irache JM, et al. (2000). Gentamicin encapsulation in PLA/PLGA microspheres in view of treating Brucella infections. Int. J. Pharm. 196(1):115–125.
- Radhakrishna Pillai G, Srivastava AS, Hassanein TI, Chauhan DP, Carrier E. (2004). Induction of apoptosis in human lung cancer cells by curcumin. Cancer Lett. 208(2): 163–170.
- Rahman Z, Kohli K, Khar RK, Ali M, Charoo NA, Shamsher AA. (2006). Characterization of 5-Fluorouracil Microspheres for Colonic Delivery. J. AAPS Pharm Sci Tech. 7(2): E47.
- Raouf G, Cecilia S, Johan C. (1996). Effect of preparative parameters on the characteristics of poly (d,l-lactideco-glocolide) microspheres made by the double emulsion method. Int. J. Pharm. 4: 205–216.
- Sahu A, Bora U, Kasoju N, Goswami P. (2008). Synthesis of novel biodegradable and self-assembling methoxy poly(ethylene glycol)–palmitate nanocarrier for curcumin delivery to cancer cells. Acta Biomaterialia. 4 (6): 1752–1761.
- Sharma RA, Steward WP, Gescher A. (2007). Pharmacokinetics and pharmacodynamics of curcumin. Advances in Experimental Medicine and Biology. 595: 453–470.
- Tabata Y, Nagano A, Ikada Y. (1999). Biodegradation of hydrogel carrier incorporating fibroblast growth factor. Tissue Eng. 5(2):127–138.
- Wang SN, Deng YH, Zheng SH, et al. (2003). Studies on preparation of ciprofloxacin liposomes and its tissue distribution in mice. Chin Pharm J. 38: 274–277.
- Wang YJ, Pan MH, Cheng AL, et al. (1997). Stability of curcumin in buffer solutions and characterization of its degradation products. J Pharm Biomed Anal. 15(12): 1867–1876.
- Yoshioka T, Hashlda M, Muranishi et al. (1981). Specific delivery of mitomyein C to the liver, spleen and lung: nano and mierospherical carriers of gelatin. Int. J. Parm. 8: 131–139.
- Zeng FB, Lu B, Yang H, et al. (1996). Studies on gelatin microsphere loaded ligustrazine hydrochloride for lung targeting. Yao Xue Xue Bao. 31(2): 132–137.