Abstract
This study was to evaluate submicron emulsion as a drug carrier for intranasal delivery of zolmitriptan (ZT). Since the drug distribution in submicron emulsion might influence the nasal absorption, two different formulations separately incorporating the drug in oily phase (ZTSE-1) and aqueous phase (ZTSE-2) were assessed. To find the better formulation for rapid-onset intranasal delivery and improvement in brain targeting of ZT, the in vivo nasal absorption of these two formulations was evaluated. The blood and cerebrospinalfluid (CSF) pharmacokinetics of ZTSE-1, ZTSE-2 and ZT solution (ZTS) were evaluated after intranasal administered to anesthetized Wistar rats. The results demonstrated that ZT from ZTSE-1 and ZTSE-2 had better brain targeting efficiency than the ZTS. In plasma and CSF, the ZTSE-2 reached peak concentration much faster than ZTSE-1 and ZTS. The ZTSE-2 also presented significantly higher initial ZT levels in CSF compared with the ZTSE-1 and ZTS. The results indicated that incorporation of ZT in the aqueous phase of submicron emulsion was effective for rapid intranasal delivery of drug to blood and brain, which would offer patients the benefits of rapid relief from migraine.
Introduction
Zolmitriptan (ZT) is widely used in the effective treatment of migraine associated with menses, migraine with aura and cluster headache (CitationVyas et al., 2005). It is a second-generation triptan being marketed after sumatriptan. The lipophilicity of zolmitriptan (logD value at pH7.4 is -1) is much higher than that of sumatriptan (CitationRance et al., 1997). The clinical research of ZT indicates that it has a better efficacy and tolerability profile at low doses of 2.5–10 mg (CitationSpencer et al., 1999). Currently, ZT is available on the market in form of oral tablets (conventional and orodispersible) and a nasal spray (CitationJain et al., 2010). The current oral therapies present drawbacks, such as slow onset of action, low bioavailability (40–45%), nausea and incomplete pain relief with recurrence of headaches (CitationJain et al., 2010). The main goals in the treatment of migraine are to provide patients with highly effective and rapid relief from migraine symptoms during an attack (CitationMacGregor et al., 2003; CitationLipton et al., 2002). It was found that almost 70% of migraine patients expressed a wish to continue using zolmitriptan nasal spray rather than current therapy, and a fast onset of action was the most important factor in choosing to continue using zolmitriptan nasal spray (CitationDahlöf et al., 2004). The aim of this study was to develop a new dosage form for rapid-onset intranasal delivery of ZT.
Nasal delivery has been explored as an alternative administration route to targeting drugs directly to the brain via the olfactory neurons (CitationIllum, 2000; CitationMathison et al., 1998). After intranasal administration, drug can be absorbed into the systemic circulation and subsequently reaches the brain by crossing the blood brain barrier (BBB). In addition, drug can also directly travel from the nasal cavity to the cerebrospinalfluid (CSF) and brain tissue through the olfactory pathway and the trigeminal neural pathway (CitationIllum, 2000; CitationThorne et al., 2004). The mechanism for the treatment of migraine of ZT is via binding to 5-HT1B/1D receptors both at the vascular level and in the brain parenchyma. Hence, enhancing the levels of ZT in blood or brain can facilitate to treat migraine and it is necessary to evaluate the brain targeting of a ZT preparation for intranasal administration.
It has been reported of zolmitriptan microemulsion for intranasal application (CitationVyas et al., 2005). However, reports revealed that microemulsion formulations exhibited a certain irritation to gastrointestinal or nasal mucosa because it contained a relatively large amount of surfactants (CitationKawakami et al., 2002; CitationZhang et al., 2004). Submicron emulsion was a relatively safe formulation for nasal administration because the biocompatible lecithin was used as the main emulsifier, and the total surfactants used were relatively less (CitationYu et al., 2009). In this study, submicron emulsion as a new drug carrier for nasal delivery of ZT was evaluated.
The mechanism for using emulsion as drug carrier to enhance nasal absorption is still unclear. Submicron emulsion formulation with drug incorporated into the oily phase enhanced drug absorption after intranasal administration (CitationYu et al., 2009). It was also reported that increase in amount of drug in the oil droplets of emulsion delayed intranasal absorption by the perfusion method (CitationAikawa et al., 1998). However, the pharmacokinetic and brain targeting were not evaluated for the different formulations of emulsion with different drug distribution in the report (CitationAikawa et al., 1998). It seemed reasonable that dispersing drug to aqueous phase of emulsions exhibited faster intranasal delivery of drug to blood or brain than incorporating drug in the oily phase. In this study, we explored the suitable phase (oily or aqueous phase) for incorporation of drug for rapid-onset intranasal delivery of ZT. Two different formulations separately incorporating ZT in oily and aqueous phases were prepared. The blood and CSF pharmacokinetics of these two formulations were evaluated aiming to choose the better formulation for intranasal delivery of ZT.
Materials and Methods
Materials
ZT was purchased from Beijing Gaobo Pharm-Chemicals Tech. Co. in China; Egg lecithin (Lipoid E170) and medium-chain triglyceride (MCT) were purchased from Lipoid KG (Ludwigshafen, Germany); Glycerol was supplied by Zhejiang Suichang Glycerol Plant (Zhejiang, China); Poloxamer 188 (Pluronic F68®) was obtained from BASF AG (Ludwigshafen, Germany); All other reagents were of analytical grade or the highest grade commercially available. Microdialysis probes were U-shaped and made of hollow cellulose fiber (DM-22, 200 μm inner diameter and 220 μm outer diameter, EICOM CORP, Japan). The membrane of the probes for brain targeting study was 4 mm in length with a molecular weight cut-off of 5000Da and artificial CSF were the same as described in the earlier study (CitationChen et al., 2008). The Wistar rats used in this study were supplied by the Animal Center of Shenyang Pharmaceutical University (Shenyang, China).
Preparation of ZTS, ZTSE-1 and ZTSE-2
The ZTS for intravenous (i.v.) and intranasal (i.n.) administration was prepared by dissolving citric acid (1.3%, w/w), disodium hydrogen phosphate (2.8%, w/w) and ZT (0.5%, w/w) in water. After stirring to obtain a clear solution, the pH was adjusted to about 5.0 with disodium hydrogen phosphate. The prepared ZT solution was 5 mg/ml.
Egg lecithin (3%, w/w) and ZT (0.5%, w/w) were dissolved in alcohol, and the solution was under stirring at 75°C until alcohol evaporated. Then the lecithin-ZT mixture was obtained and MCT (20%, w/w) was added to produce the oil phase with stirring at 75°C. The ZT–phospholipid complex was probably formed by this method because more ZT could be dissolved in MCT by dissolving the lecithin–ZT mixture than just dissolving physical mixture of ZT and lecithin (CitationLu et al., 2009). Egg lecithin (1%, w/w), Poloxamer 188 (0.4% w/w), sodium oleate (0.05%, w/w), glycerol (2.5%, w/w), EDTA-2Na (0.05%, w/w) and benzalkonium bromide (0.01%, w/w) were dispersed in water at 75 °C to produce the water phase. The oil phase was added to the aqueous phase with continuous stirring using a high shear mixer (ULTRA TURRAX T18 basic, IKA WORKS Guangzhou, Germany) at 14,000rpm for 5min to obtain the primary emulsion. This primary emulsion was passed through a high pressure homogenizer (Niro Soavi NS10012k, Niro Soavi S.p.A., Via M. Da Erba, Italy), then the 5 mg/ml ZTSE-1 (pH 9.5) for i.n. administration was obtained. Finally, the preparation was gassed with N2 and sealed in 10ml glass bottles.
The preparation process of ZTSE-2 was similar to that of ZTSE-1. Briefly, egg lecithin (3%, w/w) and ZT (0.5%, w/w) were dissolved in alcohol, and then the MCT (20%, w/w) and stearylamine (0.5%, w/w) were added to produce the oil phase after evaporation of alcohol. Egg lecithin (1%, w/w), Poloxamer 188 (0.4% w/w), glycerol (2.5%, w/w), EDTA-2Na (0.05%, w/w) and benzalkonium bromide (0.01%, w/w) were dispersed in water to make the water phase. The primary emulsion was prepared by the same method described above, and the pH was adjusted to about 5.0 using 0.1 M HCl solution. After passing the primary emulsion through the high pressure homogenizer, the 5 mg/ml ZTSE-2 for i.n. administration was obtained.
High-performance liquid chromatographic analysis in vitro
The content of ZT was determined by using a high performance liquid chromatographic (HPLC) method at a wavelength of 229nm. The HPLC equipment consisted of a Jasco PU-980 pump (Japan) and a Jasco UV-975 detector. A C18 column (250 mm × 4.6 mm, particle size 5μm) was used for the separation of ZT. The mixture of methanol, 0.025mol/L potassium dihydrogen phosphate and triethanolamine (25:75:0.5, v/v/v, pH was adjusted to 3.5 with phosphoric acid) was used as the mobile phase. ZT could be completely separated from the other components by this HPLC method. The linear range of ZT was 2.0~100.0μg/ml. The repeatability variation was 0.72% and recovery was 99.9 ± 1.2%. The method had an acceptable linear range, good precision and accuracy.
Solubility of ZT in different buffer solutions
Excess quantity of ZT was added to 2ml buffer solutions (pH4.0, 5.0, 7.0 and 9.0) and distilled water, respectively. Apparent solubility study was performed by standardized shake flask method at 37 ± 0.5°C. After shaking for 72 h, the supernatant solution was analyzed for drug content after centrifugation.
Physicochemical properties and entrapment efficiency of ZTSE-1 and ZTSE-2
The particle size and zeta potential were measured using a NICOMPTM 380 Zeta Potential/Particle Sizer (Particle Sizing Systems, Santa Barbara, USA). The mean particle size and distribution were measured using photon correlation spectroscopy (PCS, dynamic light scattering, DLS) which is a powerful and versatile tool for estimating the particle size distribution of fine-particle materials ranging from a few nanometers to several micrometers (CitationKomatsu et al. 1995). The zeta potential was determined based on an electrophoretic light scattering (ELS) technique. The emulsion sample was diluted 1:5000 and 1:50 respectively with double-distilled water before the measurement of particle size and zeta potential. The determination was carried out at 25 °C.
The microdialysis method was chosen to determine the entrapment efficiency of submicron emulsion (CitationLiu et al., 2009). The microdialysis probes were the same as described in an earlier study (CitationLiu et al., 2009). The membrane of microdialysis probes had a molecular weight cut-off of 5000Da, so nano sized oil droplets incorporating drug in submicron emulsion could not pass through the semipermeable membrane. To calculate the entrapment efficiency, the concentration of free drug in the aqueous phase was determined by the microdialysis method (CitationLiu et al., 2009).
Operations on rats and drug administration for pharmacokinetic study
The nasal cavity was isolated from the respiratory and gastrointestinal tracts using a procedure described in literatures (CitationHirai et al., 1981; CitationHuang et al., 1985). Briefly, the trachea was cannulated with a polyethylene tube to maintain respiration, and another polyethylene tube was inserted through the esophagus toward the posterior part of the nasal cavity and ligated. The animal model was shown in . The passage of the nasopalatine tract was sealed with an adhesive agent to prevent the drainage of the solution from the nasal cavity to the mouth. A polyethylene tube was inserted into the jugular vein for blood sampling.
For i.n. administration, ZTSE-1, ZTSE-2 and ZTS were instilled into the right nostril with a micropipette at a dose of 0.9 mg kg−1 respectively. Blood samples (0.3 ml) were drawn from the tube in the jugular vein into heparin stabilized test-tubes at different times: 0.083, 0.25, 0.5, 0.75, 1, 1.5, 2, 3, 4, 6, and 8h. After each blood withdrawal, the same volume of sterile normal saline was put back into the circulation to maintain the total blood volume. Plasma was separated by centrifugation at 3000 rpm for 15 min and kept frozen at -20°C for subsequent analysis.
Intravenous administration was carried out by injecting a dose of 0.9 mg kg−1 ZTS via the femoral vein. The method of sample collection was the same as described above.
Disposition of biological samples
Briefly, 0.1 ml plasma was spiked with 20μl internal standard (sumatriptan succinate, 0.5mg/ml) methanol solution and 40μl 0.1M NaOH. The sample was then vortexed with methyl tert-butyl ether (3 ml) for 2 min and, after 10min of mechanical shaker, it was centrifuged for 5 min at 4000 rpm. The supernatant was transferred to a new tube and then evaporated to dryness in a water bath at 40°C under N2 flow. The residue was dissolved in 200μl methanol, and 5μl was injected into the UPLC-MS/MS system for analysis.
Operations on rats and drug administration for evaluation of ZT levels in CSF
The nasal cavity was isolated from the respiratory and gastrointestinal tracts using the procedure described above (CitationHirai et al., 1981; CitationHuang et al., 1985). In addition, a cranial burr hole was drilled with a dental drill to a depth of 3.1 mm and the hole was 1.5 mm right lateral and 0.9 mm posterior to the bregma. The microdialysis probe was stereotaxically inserted through the cranial burr hole and attached to the skull with dental cement. Both i.n. and i.v. administration were performed following the successful implantation of a microdialysis probe and stabilization for 1 h with drug-free artificial CSF perfused at 3μl min−1. For i.n. administration, the ZTSE-1, ZTSE-2 and ZTS were instilled into the right nostril with a micropipette at a dose of 0.9 mg kg−1 respectively. Intravenous administration was carried out by injecting a dose of 0.9 mg kg−1 ZTS via the femoral vein. Artificial CSF was perfused at 3μl min−1, and the perfusate samples were collected at the intervals of 20 min for 8h. CSF perfusate samples were injected directly into the UPLC-ESI-MS/MS system for ZT analysis without any pre-treatment.
Calibration of microdialysis probe for evaluation of ZT levels in CSF
Microdialysis probe recovery was calibrated by triplicate measurements of in vitro recovery and delivery and in vivo delivery (CitationMano et al., 2002; CitationQiao et al., 2004). In vitro recovery was determined by placing the microdialysis membrane in different concentrations of ZT artificial CSF solution (5, 25, 50ng ml−1, Cm) and perfusing with drug free artificial CSF at 3μl min−1 (n = 3). Samples of the dialysate were collected at 20min intervals after stabilization for 0.5h. The ZT concentrations of the samples were analyzed by UPLC-MS/MS to give the in vitro relative recovery. In vitro delivery was determined by perfusing different concentrations of ZT artificial CSF solution (5, 25, 50ng ml−1, Cp) into a microdialysis probe the dialysis membrane of which is placed in a vial containing drug-free artificial CSF solution. In vitro relative delivery was calculated using the difference in ZT concentration between perfusate and dialysate (n = 3). The probe relative recovery, R, was defined by equation: R=(Cd/Cm)×100 and probe relative delivery, D, was defined by equation: D=(Cp-Cd)/Cp×100, where Cd is the concentration in the dialysate, Cm is the concentration of the medium and Cp is the concentration in the perfusate.
The in vivo delivery was calculated by perfusing artificial CSF solution (5ng ml−1) into a microdialysis probe. The dialysis membrane of the probe was inserted into a rat brain. However, in this study, there were some differences from the reported method of calibrating the probes (CitationMano et al., 2002; CitationQiao et al., 2004). It has been reported that a marked reduction of the in vivo recovery of probe was observed (CitationJensen et al., 2007). The reduction in microdialysis probe recovery was caused by occlusion of the membrane pores by macromolecules in the interstitium (CitationJensen et al., 2007). In this study, in vivo recovery of the microdialysis probe at each time interval was evaluated. Artificial CSF solution containing a concentration of ZT (5ng ml−1) was perfused into the probe at 3μl min−1. The microdialysate samples were collected every 20 min for 8h after implantation of probe (n = 4). The in vivo recovery at each time point of the microdialysis probe was calculated by the delivery of probe in vivo at each time point using the following equation:(R/D)in vivo=(R/D)in vitro.
Values of the in vitro recovery and delivery were 26.9 ± 4.8% and 49.2 ± 4.8%, respectively. The in vitro recovery and delivery of microdialysis were concentration independent for all the three different concentrations of ZT artifical CSF solutions. The in vivo recovery was calculated to be 36.0%, 35.6%, 30.4%, 26.2%, 22.8%, 18.6%, 18.6%, 16.4% and 15.1% for each time interval in the first 3h. The in vivo recovery decreased only in the first 3h and became constant after 3h. Hence, the in vivo recovery after 3h was calculated from the mean value of all the time points from 3 to 8h, and the calculated mean value was 13.5%.
The unbound concentrations of ZT in the CSF were calculated from the concentrations in the dialysates (Cd) using the following equation: C = Cd/R, where R is the in vivo recovery of probe calculated above. As the R in vivo was different at different time points, the unbound concentration of ZT at different time points was calculated from the R at corresponding time point.
Sample determination by UPLC-ESI-MS/MS
Liquid chromatography was performed on an ACQUITY™ UPLC system (Waters Corp., Milford, MA, USA) with a conditioned autosampler maintained at 4 °C. The separation was performed at a column temperature of 35 °C using an ACQUITY UPLC™ BEH C18 column (50mm×2.1mm i.d., 1.7μm; Waters Corp., Milford, MA, USA). The analysis was achieved with gradient elution and the mobile phase consisted of acetonitrile (A) and water (B, containing 0.1% formic acid). The gradient conditions of the mobile phase were as follows: A was increased linearly from initially 10% to 60% during the first 0.8 min, and then held for 0.8 min. Then the composition was reset to the initial composition (10% A) within 0.05min and equilibrated at the initial composition for 0.35min. The flow rate was kept constant at 0.2ml/min and the sample injection volume was 5μl using the partial loop mode.
The Waters ACQUITY™ TQD triple-quadrupole tandem mass spectrometer (Waters Corp., Manchester, UK) was connected to the UPLC system via the electrospray ionization (ESI) interface. The ESI source was operated in positive ionization mode for both ZT and IS. The ESI source parameters were as follows: capillary 0.4kV, cone voltage 34V, extractor 3.0V and RF 0.0V. The temperature of the source and desolvation was set at 100 and 400 °C, respectively. Nitrogen was used as the desolvation gas (550 L h−1) and cone gas (50 L h−1) for nebulization. For collision-induced dissociation (CID), argon was used as the collision gas at a pressure of approximately 2.91 × 10−3 mbar. The collision energy was 20 eV for both ZT and IS. The multiple reaction monitoring (MRM) mode was used for quantification. The fragmentation transitions for MRM were m/z 288.13 → 58.13 amu for ZT, and m/z 296.12 → 57.90 amu for IS. All data collected in centroid mode were acquired using MassLynx™ NT4.1 software (Waters Corp., Milford, MA, USA). Post-acquisition quantitative analyses were carried out using a QuanLynx™ program (Waters Corp., Milford, MA, USA). In plasma and CSF, ZT were detected sensitively without any interference. The method had acceptable precision and accuracy, confirming that it was a suitable analytical method.
Data analysis
The area under the concentration–time curve (AUC) was calculated using the trapezoidal rule and the absolute bioavailability was calculated using the equation below:
Where Xi.n. is the dose administered intranasally and Xi.v. is the dose administered intravenously.
Statistically significant differences between i.n. and i.v. administration were concluded using the unpaired Student’s t-test and a value of P < 0.05 was considered statistically significant. Results were presented as mean values ± SD.
Results
Solubility of ZT in different buffer solutions
The solubility of ZT in pH4.0, 5.0, 7.0, 9.0 buffer solutions and distilled water were 56.20, 53.07, 7.56, 4.66 and 1.74mg/ml, respectively. Obviously, with the increase of the pH value of the buffer solutions, solubility of ZT became lower.
Physicochemical properties and entrapment efficiency of ZTSE-1 and ZTSE-2
The particle size of ZTSE-1 and ZTSE-2 were 153.5 ± 55.0 nm and 170.5 ± 46.0 nm, respectively. The zeta potential of ZTSE-1 and ZTSE-2 were -31.4 ± 1.4 mv and +42.3 ± 1.1 mv, respectively. Egg lecithin was used as the major emulsifier, and with the help of sodium oleate in ZTSE-1 and stearylamine in ZTSE-2, the zeta potential was greater than 20 mv which was responsible for the stability by preventing coalescence of oily droplets upon random collision (CitationJumaa & Muller, 2002.). The determined entrapment efficiency of ZTSE-1 and ZTSE-2 were 77.9% and 10.9%, respectively.
Pharmacokinetic study
The pharmacokinetic parameters were calculated using DAS (drug and statistics) version 2.0 software (Mathematical Pharmacology Professional Committee of China, Shanghai, China) using non-compartmental pharmacokinetic methods. Selected pharmacokinetic parameters after i.v. and i.n. administration are shown in (), where the values of AUC, Cmax, t1/2 and Tmax can be found.
Table 1. Pharmacokinetic parameters of ZT after administration of ZTS (i.v.), ZTS (i.n.), ZTSE-1(i.n.) and ZTSE-2 (i.n.) to rats (n = 5).
After i.n. administration of ZTS, ZTSE-1 and ZTSE-2, the absolute biological availability were 84.0 ± 11.1%, 84.3 ± 12.0% and 85.0 ± 30.5%, respectively. The mean value of Tmax of ZTSE-2 (0.3h) group was obviously shorter than that of ZTS (1.0h) and ZTSE-1(1.2h) groups after i.n. administration. Tmax value of the ZTSE-2 group was statistically significantly shorter than that of the ZTS group (P < 0.05). However, no statistically significant difference was found for Tmax values between the ZTSE-2 group and the ZTSE-1 group (P > 0.05). Compared with ZTS and ZTSE-1, absorption of ZT in ZTSE-2 to blood was much faster.
Evaluation of ZT levels in CSF
The microdialysis method is used for continuous measurement of free, protein-unbound concentrations in extracellular tissue fluids. CSF is mainly produced in the lateral and third ventricles. The coordinate for the right lateral ventricle in literature are 0.8 mm posterior, 1.5mm right lateral from the bregma and 3 mm from the brain surface, which was approximately the same location for the probe being inserted in this study (CitationDai & Elmquist, 2003). To check the position of the probe whether or not being located at the right lateral ventricle, the brains were removed after the microdialysis probe was inserted to the brain. Sections (5μm) of the brains were cut on a microtome, stained with hematoxylin and eosin to verify the area of lesion. It was observed that the tendency of the lesion line was toward the right lateral ventricle (Labeled by the arrow in ) according to the atlas of Paxinos and Watson (CitationPaxinos & Watson, 1998). Some residue caused by the lesion was observed in the right lateral ventricle but none was seen in the left lateral ventricle (Labeled by circles in the ). The results demonstrated that the semipermeable membrane of the probe was located at the right lateral ventricle. So the concentration of ZT determined by microdialysis is referred to as the concentration of unbound ZT in the CSF.
The calculated concentrations of unbound ZT in CSF after i.n. and i.v. administration are shown in (), and the important parameters are listed in (). The Tmax of ZTSE-2 (2.0 ± 0.9h) was significantly shorter than that of ZTS (4.0 ± 1.1h) or ZTSE-1 (4.0 ± 1.4h) after i.n. administration (P < 0.05). This result showed that ZT from ZTSE-2 was transported to CSF much faster than that from ZTS or ZTSE-1 by the nasal route. As is shown in (), ZTSE-2 had higher initial ZT levels in CSF compared with ZTSE-1 and ZTS after i.n. administration. Obviously, ZTSE-2 had the potential advantage in terms of a faster onset of action of ZT.
Table 2. Pharmacokinetic parameters of unbound ZT in CSF and values of AUCCSF/AUCPlasma after administration of ZTS (i.v.), ZTS (i.n.), ZTSE-1(i.n.) and ZTSE-2 (i.n.) to rats (n = 5).
Figure 3. The mean concentration–time curves of unbound ZT in CSF in rats after intranasal and intravenous administration of the dosage forms at the dose of 0.9mg kg-1. (n = 5, mean ± SD).
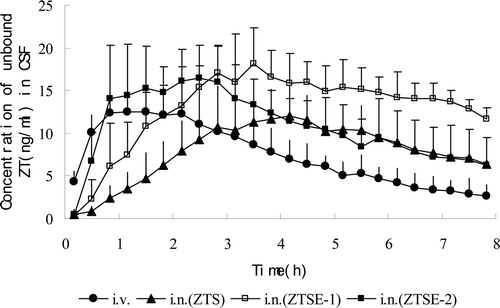
The AUCCSF/AUCplasma is a well accepted approach to evaluate an additional contribution of the nose-CSF pathway to the uptake of drugs into the CSF (CitationWang et al., 2006). The AUCCSF/AUCPlasma value of i.n. administration of ZTS was not significantly higher than that of i.v. administration (P > 0.05), which meant that i.n. administration of ZTS did not exhibit an obviously higher brain-targeting efficiency. Compared with i.v. administration of ZTS, i.n. administration of ZTSE-1 and ZTSE-2 had significantly higher AUCCSF/AUCPlasma values (P < 0.05). The results demonstrated that ZTSE-1 and ZTSE-2 had the potential advantage of directly transporting drug to the brain over the ZTS.
Discussion
Solubility study showed that with the increase of the pH value of the buffer solutions, solubility of ZT became lower. ZT is a faintly alkaline drug and has a pKa of 9.6. At higher pH, more ZT exists in the unionized form which has a higher lipophilicity than the ionized form. As a result, solubility of ZT decreased as the pH value increased. For this reason, a larger amount of ZT was dispersed in the aqueous phase after adjusting submicron emulsion to a relatively low pH value. However, phenomenon of emulsion creaming was observed within several hours after adjusting the pH value of ZTSE-1 to 5.0. As ZT is a faintly alkaline, there existed a large amount of cations caused by ionization effect of ZT at low pH. The cations made the submicron emulsions unstable because the zeta potential of the formulation was negative. Stearylamine additive into submicron emulsion of ZT made zeta potential of submicron emulsion positive. After adjusting the pH value to 5.0, the prepared submicron emulsion (ZTSE-2) was stable for at least 6 months at 4°C and the entrapment efficiency of ZTSE-2 (pH5.0) was extremely lower than that of ZTSE-1(pH 9.5).
Penetration enhancers such as surfactants, betacyclodextins, bile salts, phospholipids and lysophospholipids can significantly increase the permeability of drug across nasal mucosa (CitationArora et al., 2002). Hence, the surfactants in ZTSE-2 probably acted on nasal mucosa and promoted nasal permeability of ZT. Further more, it was reported that incorporation of insulin into the continuous aqueous phase of an o/w emulsion appeared to favor insulin absorption (CitationMitra et al., 2000). However, the surfactants alone did not induce any enhancement of nasal absorption of insulin (CitationMitra et al., 2000). Hence, there was also a possibility that the nano-sized oil droplets in submicron emulsion could improve the action of the surfactants on the nasal mucosal and thus, further increase the mucosal permeability than using surfactants alone.
As ZT is a faintly alkaline drug, the amount of drugs existing in the unionized form in ZTSE-1 (pH 9.5) was much more than that in ZTSE-2 (pH 5.0). On the basis of the pH-partition theory, unionized species are absorbed better than ionized species for nasal absorption (CitationJadhav Kisan et al., 2007). Surfactants in ZTSE-1 and ZTSE-2 were approximately the same. However, ZT in ZTSE-1 was transported to blood and CSF much more slowly than that in ZTSE-2 although more drugs were in the unionized form in ZTSE-1. It was presumed that ZT in ZTSE-1 was absorbed after being released from the emulsion droplets and thus absorption of ZT postponed. Partitioning of ZT to the aqueous phase of submicron emulsion was a better approach for rapid-onset intranasal delivery of ZT compared with incorporation of ZT in the oily phase. For patients, a faster onset of action is the main reason for preferring antimigraine treatment over another (CitationPascual et al., 2001; CitationLipton & Stewart, 1999). As a result, ZTSE-2 will offer patients the benefits of rapid relief from migraine.
Intranasal administration of the two submicron emulsions of ZT had significantly higher values of AUCCSF/AUCPlasma than i.v. administration. However, the AUCCSF/AUCPlasma value of i.n. administration of ZTS was not significantly higher than that of i.v. administration. The results showed that partition of ZT in aqueous phase or oily phase of submicron emulsion could enhance delivery efficiency of ZT to CSF by the nasal route. The surfactants in submicron emulsions can significantly increase the permeability of drug across the nasal mucosa resulting in higher uptake of drug to brain (CitationArora et al., 2002; CitationVyas et al., 2005).
In the previous report, the absolute biological availability of ZT was 40% after administration of nasal spray to healthy volunteers (CitationRapoport et al., 2004). In this study, the absolute biological availability after intranasal administration of ZTS, ZTSE-1 and ZTSE-2 was much higher. Absorption of drug in the surgically prepared anesthetized animal was much greater than that in awake animals (CitationDaugherty et al., 1988). The reason was suggested to be the lack of airflow through the nares of the surgically treated animal and the fixed position of the animals on their backs. In addition, the anaesthetic decreased mucociliary clearance and caused the higher intranasal absorption of drug (CitationMayor & Illum, 1997). Similarly, the surgically prepared anesthetized rats were used in this study and the animals were immobilized on their backs during the experiments. As a result, a relatively high biological availability of ZT was obtained.
In this study, submicron emulsion as a new drug carrier for nasal delivery of ZT exhibited higher brain targeting efficiency than a simple nasal solution formulation. To explore the suitable phase (oily or aqueous phase) for incorporation of drug for rapid-onset intranasal delivery of ZT, two different formulations incorporating the drug in oily (ZTSE-1) and aqueous phase (ZTSE-2) separately were assessed. The results indicated that incorporation of ZT in aqueous phase of the submicron emulsion was effective for rapid intranasal delivery of drug into blood and brain. Development of such a submicron emulsion formulation for intranasal delivery of ZT is valuable and will offer patients the benefits of rapid relief from migraine.
Acknowledgements
Dr. David B. Jack is gratefully thanked for correcting the manuscript.
Declaration of interest
The authors declare no conflicts of interest.
References
- Aikawa K, Matsumoto K, Uda H, Tanaka S, Shimamura H, Aramaki Y, Tsuchiya S.(1998). Prolonged release of drug from O/w emulsion and residence in rat nasal cavity. Pharm Dev Technol. 3: 461–469.
- Arora, P., Sharma, S., Garg, S. (2002). Permeability issues in nasal drug delivery. Drug Discov Today. 7: 967–975.
- Chen, J., Wang, X., Wang, J., Liu, G., Tang, X. (2008). Evaluation of brain-targeting for the nasal delivery of ergoloid mesylate by the microdialysis method in rats. Eur J Pharm Biopharm. 68: 694–700.
- Dahlöf, C.G.H., Linde, M., Kerekes, E. (2004). Zolmitriptan nasal spray provides fast relief of migraine symptoms and is preferred by patients: a Swedish study of preferencein clinical practice. J Headache Pain. 5: 237–242.
- Dai, H.Q., Elmquist, W.F. (2003). Drug Transport Studies Using. In Nag S, editor. The blood-brain barrier: biology and research protocols, Totowa: Humama Press. p 249–264.
- Daugherty, A.L., Liggitt, H.D., McCabe, J.G., Moore, J.A., Patton, J.S. (1988). Absorption of recombinant methionyl-human growth hormone (Met-hGH) from rat nasal mucosa. Int J Pharm. 45: 197–206.
- Hirai, S., Yashiki, T., Matsuzawa, T., Mima, H. (1981). Absorption of drugs from the nasal mucosa. Int J Pharm. 7:317–325.
- Huang, C.H., Kimura, R., Nassar, R.B., Hussain, A. (1985). Mechanism of nasal absorption of drugs. I. Physicochemical parameters influencing the rate of in situ nasal absorption of drugs in rats. J Pharm Sci. 74:608–611.
- Illum, L. (2000). Transport of drugs from the nasal cavity to the central nervous system. Eur J Pharm Sci.11:1–18.
- Jadhav Kisan, R., Gambhire Manoj, N., Shaikh Ishaque, M., Kadam Vilarsrao, J., Pisal Sambjahi, S. (2007). Nasal Drug Delivery System-Factors Affecting and Applications. Current Drug Therapy. 2:27–38.
- Jain, R., Nabar, S., Dandekar, P., Patravale, V. (2010). Micellar nanocarriers: potential nose-to-brain delivery of zolmitriptan as novel migraine therapy. Pharm Res. 27: 655–664.
- Jensen, S.M., Hansen, H.S., Johansen, T., Malmlöf, K. (2007). In vivo and in vitro microdialysis sampling of free fatty acids. J Pharm Biomed Anal. 43:1751–1756.
- Jumaa, M., Muller, B.W. (2002). Parenteral emulsions stabilized with a mixture of phospholipids and PEG-660-12-hydroxystearate: evaluation of accelerated and long-term stability. Eur J Pharm Biopharm. 54: 207–212.
- Kawakami, K., Yoshikawa, T., Hayashi, T., Nishihara,Y., Masuda, K. (2002). Microemulsion formulation for enhanced absorption of poorly soluble drugs. II. In vivo study. J. Control. Release 81: 75–82.
- Komatsu, H., Kitajima, A., Okada, S. (1995). Pharmaceutical characterization of commercially available intravenous fat emulsions: estimation of average particle size, size distribution and surface potential using photon correlation spectroscopy. Chem Pharm Bull 43:1412–1415.
- Lipton, R.B., Hamelsky, S.W., Dayno, J.M. (2002). What do patients with migraine want from acute migraine treatment? Headache. 42:3–9.
- Lipton, R.B., Stewart, W.F. (1999). Acute migraine therapy: do doctors understand what patients with migraine want from therapy? Headache. 39:20–26.
- Liu, X., Zhang, Y., Tang, X., Zhang, H. (2009). Determination of entrapment efficiency and drug phase distribution of submicron emulsions loaded silybin. J Microencapsul. 26:180–186.
- MacGregor, E.A., Brandes, J., Eikermann, A. (2003). Migraine prevalence and treatment patterns: the global Migraine and Zolmitriptan Evaluation Survey. Headache. 43:19–26.
- Mano, Y., Higuchi, S., Kamimura, H. (2002). Investigation of the high partition of YM992, a novel antidepressant, in rat brain - in vitro and in vivo evidence for the high binding in brain and the high permeability at the BBB. Biopharm Drug Dispos. 23:351–360.
- Mathison, S., Nagilla, R., Kompella, U.B. (1998). Nasal route for direct delivery of solutes to the central nervous system: fact or FFFFFB01ction? J Drug Target. 5: 415–441.
- Mayor, S.H., Illum, L. (1997). Investigation of the effect of anaesthesia on nasal absorption of insulin in rats. Int J Pharm. 149: 123–129.
- Mitra, R., Pezron, I., Chu, W.A., Mitra, A.K. (2000). Lipid emulsions as vehicles for enhanced nasal delivery of insulin. Int J Pharm. 205:127–134.
- Pascual, J., Muñoz, R., Leira, R. (2001). An open preference study with sumatriptan 50 mg and zolmitriptan 2.5 mg in 100 migraine patients. Cephalalgia. 21:680–684.
- Paxinos, G., Watson, C. (1998). The rat brain in stereotaxic coordinates, CD-ROM. 4th ed. San Diego: Academic Press.
- Qiao, J.P., Abliz, Z., Chu, F.M., Hou, P.L., Zhao, L.Y., Xia, M., Chang, Y., Guo, Z.R. (2004). Microdialysis combined with liquid chromatography-tandem mass spectrometry for the determination of 6-aminobutylphthalide and its main metabolite in the brains of awake freely-moving rats. J Chromatogr B Analyt Technol Biomed Life Sci. 805:93–99.
- Rapoport, A.M., Bigal, M.E., Tepper, S.J., Sheftell, F.D. (2004). Zolmitriptan (ZOMIG). Expert Rev Neurother. 4:33–41.
- Rance, D., Clear, N., Dallman, L., Llewellyn, E., Nuttall, J., Verrier, H. (1997). Physicochemical comparison of eletriptan and other 5-HT1D-like agonists as a predictor of oral absorption potential. Headache 37:328.
- Spencer, C.M., Gunasekara, N.S., Hills, C. (1999). Zolmitriptan: a review of its use in migraine. Drugs. 58:347–374.
- Thorne, R.G.., Pronk, G.J., Padmanabhan, V., Frey, W.H. (2004). Delivery of insulin-like growth factor-I to the rat brain and spinal cord along olfactory and trigeminal pathways following intranasal administration. Neuroscience. 127: 481–496.
- Vyas, T.K., Babbar, A.K., Sharma, R.K., Misra, A. (2005). Intranasal mucoadhesive microemulsions of zolmitriptan: preliminary studies on brain-targeting. J Drug Target. 13:317–324.
- Wang, X., He, H., Leng, W., Tang, X. (2006). Evaluation of brain-targeting for the nasal delivery of estradiol by the microdialysis method. Int J Pharm. 317: 40–46.
- Vyas, T.K., Shahiwala, A., Marathe, S., Misra, A. (2005). Intranasal drug delivery for brain targeting. Curr Drug Deliv. 2:165–175.
- Yu, C., Meng, J., Chen, J., Tang, X. (2009). Preparation of ergoloid mesylate submicron emulsions for enhancing nasal absorption and reducing nasal ciliotoxicity. Int J Pharm. 375: 16–21.
- Zhang, Q., Jiang, X., Xiang, W., Lu, W., Su, L., Shi, Z. (2004). Preparation of nimodipine-loaded microemulsion for intranasal delivery and evaluation of the targeting efficiency to brain. Int. J. Pharm. 275: 85–96.