Abstract
Liposomes are potential carriers for targeting and controlled drug delivery by the intravenous route. Carboxymethyl chitosan (CMC) is a ramification of chitosan with intrinsic water-solubility. The aim of this study is to prepare itraconazole-loaded liposomes coated by carboxymethyl chitosan (CMC-ITZ-Lips), to evaluate its physico-chemical characteristics and the tissue targeting after being injected intravenously (i.v.). This study uses a film dispersion method to prepare itraconazole-loaded liposomes (ITZ-Lips) prior to coating them with CMC. The concentrations of ITZ in selected organs were determined using reversed-phase high-performance liquid chromatography (HPLC) following i.v. administration of ITZ-Sol, ITZ-Lips, and CMC-ITZ-Lips. CMC-ITZ-Lips had an average diameter of 349.3 ± 18 nm with a zeta potential of −35.71 ± 0.62 mV and the in vitro antifungal activity was not inhibited by the entrapment. The CMC-ITZ-Lips exhibited a longer elimination half life (t1/2β) in vivo compared with ITZ-Sol and ITZ-Lips after i.v. injection to mice. The biodistribution in mice was also changed after ITZ was encapsulated in CMC coated liposomes. CMC-ITZ-Lips performed significant lung targeting efficiency with AUC, Te and Re of lung all showed obvious elevation. In this study itraconazole was successfully encapsulated into carboxymethyl chitosan-modified liposomes for application of injection.
Introduction
The incidence, severity, and mortality of invasive fungal infections (IFIs) such as candidiasis, aspergillosis, and cryptococcosis have risen dramatically over the last 20 years. Fungal infection can be a serious medical problem occuring as patients’ immunological systems have become increasingly compromised (CitationAscioglu et al., 2002), in response to disease states such as AIDS, cancer, and diabetes, as well as the use of more aggressive medical and surgical strategies, including life-support systems, broad-spectrum antibiotics, high dose cytotoxic chemotherapy, and organ transplantation (CitationGong et al., 2003). These infections are associated with significant morbidity and mortality, with fatality rates for aspergillosis as high as 60%.
A preferred treatment for cutaneous and systemic fungal infections involves administration of antifungal azole compounds. Itraconazole (ITZ) is a triazole antifungal agent against a broad spectrum of fungal species, and it is widely clinically used for a variety of serious fungal infections in normal and immunocompromised hosts, including Aspergillosis, Cryptococcus, Candida, Blastomyces, disseminated Penicillium mameffei infections, and Histoplasma capsulatum for the last 2 decades (CitationGrant & Clissold, 1989; CitationKaufman & Carver, 1997; CitationJain and Sehgal, 2001). It is in great demand to develop an intravenous formulation of ITZ, since many neutropenic and other immunocompromized patients have difficulty in swallowing the oral capsule formulation of the drug. However, it is very difficult to develop an intravenous formulation of itraconazole because of its insolubility in aqueous media (S = 1 ng/mL at neutral pH and S = 4 μg/mL at pH 1), and the calculated log P is 6.2 (CitationPeeters et al., 2002). Sporanox® I.V. is the only commercially available intravenous formulation of ITZ solubilized by hydroxylpropyl-β-cyclodetrin (HP-β-CD), and facilitates the establishment of high and dependable levels of the active compound in plasma. Nevertheless, Sporanox® I.V. is prohibited from clinical use in patients with impaired renal function because of its renal impairment possibility from the toxicity of the adjuvant HP-β-CD. Each milliliter of Sporanox® I.V. contains 10 mg of itraconazole solubilized by 400 mg of HP-β-CD as an inclusion complex (CitationKim et al., 2010). Following a single intravenous dose of 200 mg Sporanox® I.V. to the subjects with severe renal impairment, clearance of HP-β-CD was 6-fold reduced compared with subjects with normal renal function. Although its clinical relevance is unknown, it has been reported that HP-β-CD produced pancreatic adenocarcinoma in a rat carcinogenicity study (CitationWalsh PM.2003; CitationYi et al. 2007). Hence, an intravenous formulation of itraconazole without HP-β-CD is of great potential.
Liposomes are possible carriers for targeted drug delivery by the intravenous route (CitationBanerjee, 2001). Liposomes have shown advantages in biocompatibility, biodegradability, and in-vivo toxicity, and share a similar composition to the natural cell membrane (CitationGregoriadis, 1988). Liposomes have been extensively used in an attempt to improve the selective delivery and the therapeutic index of antifungal agents. A successful application of liposomes as drug carriers is amphotericin B liposome for injection, which is much safer than its free form. However, conventional liposome is rapidly recognized and cleared by the reticuloendothelial system (RES) from the blood stream, and thus its application as a drug delivery system is limited (CitationBakker-Woudenberg, 2002; CitationZhang & Hou, 2003). Many methods have been applied to reducing the RES uptake in order to prolong the duration of liposomes in the circulatory system. Modified materials on the surface of the liposomes can prevent the aggregation of the liposomes by increasing the repulsion of the particles, forming conformational clouds to produce big stereospecific blockade, or making a hydration shell on the surface of the liposomes to reduce the recognization and uptake of RES, and the stability was increased both in vivo and in vitro.
Carboxymethyl chitosan (CMC) is a ramification of chitosan, with good water solubility (CitationMuzzarelli, 1988). Carboxymethyl can increase the solubility and make the CMC negative. We apply CMC to the surface modification of the liposomes to form a hydration shell to reduce the recognization and uptake of RES, in order to increase the stability in vivo (CitationTakeuchi et al., 1994).
The aim of this study was to prepare itraconazol-loaded liposomes coated by CMC, to study the physico-chemical properties of the liposomes, the antifungal activities in vitro, safety evaluation in vivo and in vitro, and the pharmacokinetics and tissue distributions in vivo.
Materials and methods
Materials
Injectable soya lecithin was provided by Shanghai TaiWei Pharmaceutical Co., Ltd (Shangai, China). Cholesterol was purchased from Shanghai Medical Chemical Reagent Co., Ltd. (Shangai, China). ITZ was purchased from Fukang Pharmaceutical Co., Ltd (Shouguang, China). CMC was purchased from Haidebei Marine Biological Co., Ltd (Ji’nan, China). Itraconazole Injection (ITZ-Sol) was purchased from Xian-Janssen Pharmaceutical, Ltd (Xi’an, China). All the other chemicals were of analytical grade or higher.
Strain and culture conditions
The C. albicans strain (CA2, MIC = 16 μg/ml) used in this study was kindly donated by Shandong Provincial Qianfoshan Hospital of China, and stored in medium containing 20% glycerol at −80°C. The strain was grown in either YPD medium (2% tryptone, 1% yeast extract, 2% glucose) (Oxo Ltd., London, UK), YPD solid medium supplemented with 2% agar (Oxo Ltd.), or RPMI 1640 broth (Hyclone, Logan, UT, USA) buffered with 0.165M MOPS (morpholinepro-panesulfonic acid; (Amresco, Solon, Ohio, USA)) at pH 7. Prior to test, the isolate was sub-cultured at least twice on YPD solid medium to ensure purity and viability.
Preparation of ITZ-Lips
The thin film dispersion method was used to prepare ITZ-Lips (CitationHuang et al., 2007; CitationXu et al., 2008). The required amounts of ITZ, phospholipid, and cholesterol were added directly into chloroform. After complete dissolution, the organic solvent was slowly removed at reduced pressure, in a 35°C water bath on a rotary evaporator, to form a thin film of the phospholipid mixture on the inner wall of the flask. Hydration of the dry lipid film is accomplished simply by adding an aqueous solution (phosphate buffer at pH 7.4) to the container of the dry lipid film and agitating at a temperature above the phase transition temperature of the lipid. Large multilayer liposomes are produced and then treated with exploring needle ultrasound (power 500 W) for 20 s to produce a liposome suspension with a definite particle diameter. The CCNU-Lips suspension was either stored at 4°C or lyophilized for long-term storage.
CMC-coated liposomes
Cationic liposomes were prepared by adding octadecylamine to the lipid. The preparation method was the same as described above.
Carboxymethyl chitosan (0.04 g) was dissolved in distilled water (20 ml) by using a magnetic stirrer at room temperature to obtain a carboxymethyl chitosan solution of concentration 0.2%, which was used throughout the study. Carboxymethyl chitosan solution was added drop-wise to liposomal suspension under magnetic stirring at room temperature. After addition of the carboxymethyl chitosan, the mixture was left under stirring for ~ 1 h then incubated overnight at −4°C.
Determination of drug entrapment efficiency
The entrapment efficiency (EE) of ITZ-Lips was determined by first using a 0.8 μm membrane filter to separate ITZ-Lips and the free drug, and then the content of ITZ was determined by a UV spectrophotometer. Briefly, 1 ml of ITZ-Lips was passed through a 0.8 μm membrane filter to remove the non-entrapped ITZ, the remaining liposomes were disintegrated by the addition of methanol prior to ITZ measurement. For the calculation of the encapsulation efficiency, the total amount of ITZ in the sample was measured prior to filtering.
Characterization of liposome
The morphology of Lips was examined by transmission electron microscopy (JEM-1200EX, Tokyo, Japan): Liposome suspension diluted with double-distilled water to 1:3–1:5 of its original concentration was dropped in a dedicated copper screen, stained with 1.5% phosphotungstic acid, and allowed to air-dry slowly and evenly. Photographs were obtained while observing the stained liposome under the microscope.
The size and zeta potential of the liposomes were determined by light scattering technique (Zetasizer 3000 HAS; Malvern Instruments Ltd., Malvern, Worcestershire, UK). The measured liposomes were diluted with physiological saline.
Influence of CMC concentration on the stability of liposomes in plasma
CMC solutions with concentrations of 0.1%, 0.2%, and 0.3% were used to coat liposomes. The three kinds of coated liposomes were added to plasma to make a final plasma concentration of 10% and then put into a water bath at 37°C. Absorbance of the suspension at 600 nm was measured at different times by comparison with the zero-time absorbance, in order to exam the stability of liposomes.
Measurement of MICs
Susceptibilities were determined with a slight modification of the fungal microbroth dilution method M27-A of the NCCLS (CitationWayne, 1997; CitationWu et al., 2009). The stock solution of CMC-coated ITZ liposomes was serially diluted (2-fold) in RPMI 1640 medium, and 100 μl aliquots were dispensed into microdilution plates for a final drug concentration ranging from 0.015–30 μg/ml (). Then, 100 μl of yeast inoculum containing 1–5 × 103 CFU/ml was added to each well. The plates were incubated at 30°C for 24 h. The MIC was the lowest concentration that demonstrated no visible growth. Itraconazole injection is operated the same. All assays were performed at least twice on separate occasions.
Table 1. Design of antifungal tests in vitro.
Pharmacokinetics and tissue distribution
The pharmacokinetics and tissue distribution experiments were carried out with female or male Kunming mice weighed between 18–22 g provided by the Medical Animal Test Center of Shandong University. Three groups of mice with 55 mice in each group were used for the in vivo pharmacokinetics and distribution study. One group was tested for ITZ-Lips, one was for CMC coated ITZ-Lips (equal to 2 mg/kg ITZ), and the other group for ITZ-Sol as control. Five mice in each group were taken out randomly at each time interval (0.083, 0.25, 0.5, 0.75, 1, 2, 4, 6, 8, 12, 24 h). Blood samples were collected from the ocular artery directly and placed into heparinized test tubes. Mice were sacrificed, dissected, and the organs were removed. Plasma was immediately collected by centrifugation (4000 rpm) for 15 min and stored at −20°C until analysis. Each organ sample was washed with NS and the remaining liquid was removed with absorbent paper. Accurately weighed tissue specimen was homogenized with 1 ml NS (liver 2 ml) in an ice bath by a homogenizer.
The plasma sample and homogenized tissue of mice were extracted with methanol prior to analysis by HPLC. Briefly, 0.3 ml of plasma or homogenized tissue was added to 0.45 ml methanol and the mixture was vortexed for 1 min. Upon centrifugation at 4000 rpm for 10 min, the supernatant was transferred to a 2 ml centrifuge tube and filtered through a 0.22 μm membrane before analysis by HPLC.
HPLC analysis of ITZ plasma and tissue samples
Concentrations of ITZ were measured by HPLC (CitationCompas et al., 1996; CitationKhatri et al., 2001). The analytical column was a DiamosilTM-C18 column (150 × 4.6 mm, 5 μm). ITZ was monitored at the wavelength of 262 nm. The mobile phase was methanol–water (84:16, v/v) at a flow rate of 1.0 ml/min. Twenty microliters was injected for HPLC analysis. Specificity test results satisfied the measurement requirement. The detection limit of ITZ was 20 ng, and the quantitation limit was 50 ng. The LOQ achieves determination requirements. Three different concentrations of ITZ plasma sample (0.2, 5.0, 40.0 μg/ml) were prepared to determine the intra-day and inter-day variations. Intra-day and inter-day variations were less than 4% and 6%, respectively. Mean recovery rates of each organ exceeded 80%, RSD < 5%.
Data analysis
Data of ITZ concentrations in plasma and tissue obtained from mice were averaged for mean values. Pharmacokinetic parameters in plasma and tissue were calculated by a statistical moment algorithm-Drug and Statistics by DAS program (Department of Clinical Pharmacology, Shandong Qilu Hospital, Ji’nan, China).
Results and discussion
Characterization of Lips
We discover an increase of the size of liposome due to the carboxymethyl chitosan coating layer (). The diameters of ITZ-Lips and CMC-ITZ-Lips are 170.1 ± 15 nm and 349.3 ± 18 nm, respectively, with a zeta potential of −9.39 ± 0.75 mV and −35.71 ± 0.62 mV. Surface morphological studies on the shape of prepared systems using transmission electron microscopy indicated that the systems were almost spherical (). We also had an excellent visual confirmation of the carboxymethyl chitosan surrounding the liposomes. The hydrophilic polymer coating of liposomes would depend on the ability of the polymer to adhere to the lipid bilayers. The interaction between carboxymethyl chitosan and liposomes is due to a combination of adsorption coagulation and bridging between them (CitationFilipovic-Grcic et al. 2001). The surface of cationic liposomes carried positive charges, with a zeta potential of 38.87 ± 0.58mV (n = 3), while when coated the charges were negative and the zeta potential was −35.71 ± 0.62 (n = 3). Drug loading was 4.32% ± 0.33 (n = 3), and the entrapment efficiency was 83.4% ± 2.49 (n = 3).
Influence of CMC concentration to the stability of liposomes in plasma
The absorbance changes in plasma of ITZ liposomes modified with three different concentrations of CMC are shown in , absorbance minus zero in serum at different times is shown in . The results indicated that liposome was unstable in blood plasma when coated with CMC of 0.1% because the absorbance difference was increased. When coated with CMC of 0.2% and 0.3%, the absorbance difference was not obvious and it indicated the liposome was stable in plasma.
Table 2. Absorbance minus zero in serum at different times of different concentrations of CMC-modified liposomes.
In vitro antifungal test
In the present study, we have examined the antifungal properties of CMC-coated ITZ-Lips and in vitro with ITZ injection as contrast. Using Candida albicans in broth cultures and a micro-dilution method, comparative efficacies of CMC-coated ITZ-Lips and ITZ injection were examined in vitro. Both of the two preparations have a MIC of 0.06 μg/ml, indicating itraconazole-loaded liposomes maintained a strong antifungal activity in vitro and it was not inhibited by the encapsulation of liposomes.
Pharmacokinetics study and tissue distribution study in mice
Plasma concentration vs time profiles of the ITZ-Sol, ITZ-Lip, and CMC-ITZ-Lips were analyzed using the DAS 2.0 (Drug and Statistics for Windows) program. Pharmacokinetic parameters are shown in , and the mean plasma concentration vs time curves of the ITZ-Sol, ITZ-Lip, and CMC-ITZ-Lips after intravenous administration are shown in . The results of the pharmacokinetic studies indicated that ITZ-Sol, ITZ-Lip, and CMC-ITZ-Lips all followed the open two-compartment model after i.v. administration. Results of tissue distribution are shown in , tissue targeting parameters are shown in .
Table 3. The comparative plasma pharmacokinetic parameters after i.v. administration of ITZ-Sol, ITZ-Lip, and CMC-ITZ-Lips in mice (n = 5).
Table 4. Tissue targeting parameters of ITZ-Sol, ITZ-Lip, and CMC-ITZ-Lips after i.v. administration in mice (n = 5).
Figure 4. Mean drug plasma concentration-time curve after intravenous administration of ITZ-Sol, ITZ-Lip, and CMC-ITZ-Lips in mice (n = 5).
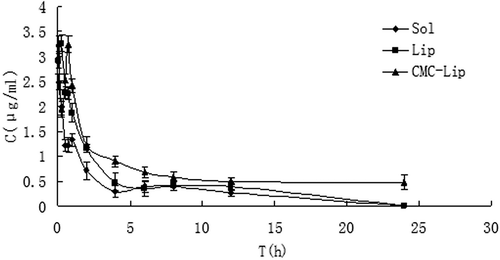
Figure 5. The distribution of mice heart tissues at different time points after the intravenous administration of ITZ-Sol, ITZ-Lip, and CMC-ITZ-Lip (n = 5).
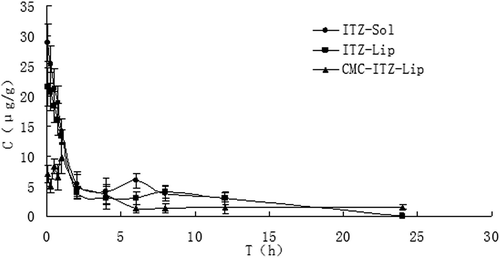
Figure 6. The distribution of mice liver tissues at different time points after the intravenous administration of ITZ-Sol, ITZ-Lip, and CMC-ITZ-Lip (n = 5).
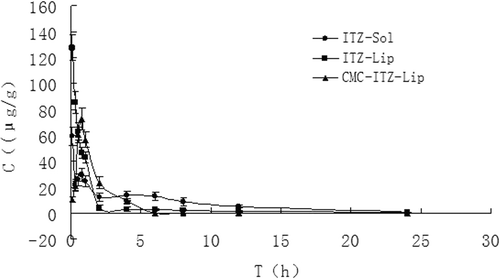
Figure 7. The distribution of mice spleen tissues at different time points after the intravenous administration of ITZ-Sol, ITZ-Lip, and CMC-ITZ-Lip (n = 5).
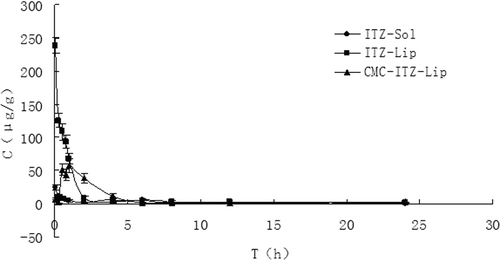
Figure 8. The distribution of mice lung tissues at different time points after the intravenous administration of ITZ-Sol, ITZ-Lip, and CMC-ITZ-Lip (n = 5).
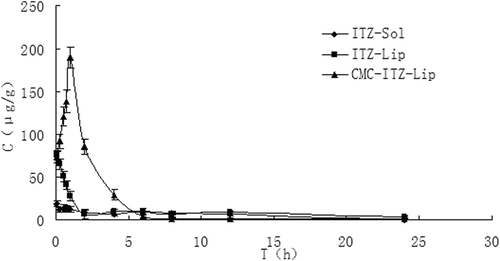
Figure 9. The distribution of mice kidney tissues at different time points after the intravenous administration of ITZ-Sol, ITZ-Lip, and CMC-ITZ-Lip (n = 5).
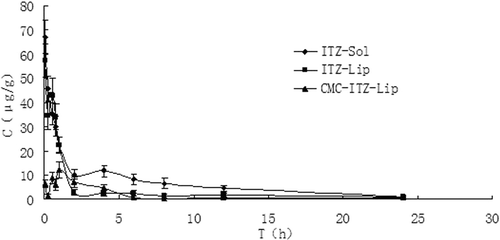
Figure 10. The distribution of mice brain tissues at different time points after the intravenous administration of ITZ-Sol, ITZ-Lip, and CMC-ITZ-Lip (n = 5).
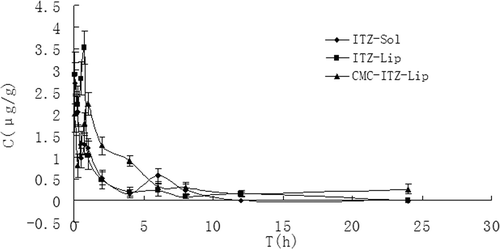
This project was designed to prepare itraconazole-loaded liposomes so as to reduce the renal toxicity caused by HP-β-CD, which was widely used as an adjuvant in the current commercially available itraconazole injection, and CMC-coated liposomes were designed to increase the hydrophilicity of liposomes surface to prolong the circulation time in vivo and to reduce the drug intake in macrophage-rich tissues such as liver and spleen. and showed that the circulation time of both ITZ-Lips and CMC-ITZ-Lips in vivo was significantly longer than that of the itraconazole injection. Compared with the commercially available itraconazole injection, MRT of CMC-Lip and CMC-ITZ-Lip extended from 4.706 h to 13.479 h and 17.432 h, and AUC increased from 6.427 to 13.479 and 17.432, respectively. Drug distribution of ITZ-Lips in the liver and spleen was increased due to the macrophage phagocytosis, and the AUC increased respectively from 139.150 μg/g*h and 48.821 μg/g*h to 192.007 μg/g*h and 230.059 μg/g*h. However, compared with the unmodified liposomes, the drug distribution in liver and spleen decreased after ITZ was wrapped in CMC-coated liposomes and the AUC was 134.284 and 180.285 μg/g*h, respectively. It showed that the hydrophilic modification of CMC on the liposomes surface reduced the recognition and phagocytosis of macrophages, resulting in the increase of the drug circulation time in vivo.
Drug targeting to the lungs is very useful in the clinical treatment because the systemic fungal infections occur mostly in the lungs. Compared with the injection, drug distribution in the lungs of ITZ-Lips was greatly improved, and the AUC increased from 102.955 μg/g*h to 222.309 μg/g*h. This may because there are a large number of pulmonary macrophages in the lung. The drug distribution of the CMC-modified liposomes was further increased than unmodified ones in the lungs, and the relative uptake rate increased from 2.159 to 4.243. At the same time, the targeting efficiency increased from 27.31% to 49.71%, and the Cmax was increased significantly too. There have been some studies showing that cationic liposomes have a significant initiative targeting to the lung, and can significantly reduce the drug concentrations in other tissues, thereby reducing the side-effects of drugs. The liposomes prepared in our study were coated by carboxymethyl chitosan and the surface was negative. When the preparation was injected into the circulation in vivo, the environment changed and the physical adsorption may be gradually lifted to bare the cationic liposome core, and the cationic liposomes tend to target the lungs, gradually.
Entrapment in the liposomes also reduced the drug distribution in heart and kidneys significantly, resulting in a reduction of the toxicity in heart and kidney. After being modified by CMC, the drug distribution in heart and kidney was further reduced. Compared with the solution, Te of the unmodified liposomes and the modified ones were reduced from 13.54% to 7.42% and 5.96% in the heart, and decreased from 31.14% to 11.09% and 5.32% in the kidney, respectively. Cmax in heart and kidney was significantly reduced too.
Conclusion
In this study, we successfully developed ITZ-containing liposomes with a mean diameter smaller than 200 nm using a film dispersion method with high encapsulation efficiency and then we modified them with CMC to increase the circulation time in vivo. In vitro antifungal testing showed that CMC-ITZ-Lips exhibited a strong antifungal activity. Compared with the control group, ITZ-Lips presented a prolonged residence time of the drug in mice, and significantly improved the efficiency to RES organs of mice such as liver and spleen. After being modified with CMC, the CMC-ITZ-Lips showed a longer residence time than ITZ-Lips, and the efficiency to RES organs was reduced remarkably. Targeting efficiency to the lung is particularly evident. Furthermore, CMC-ITZ-Lips and ITZ-Lips could both reduce the Cmax in non-RES organs such as heart and kidney, potentially decreasing the risk of cardiac toxicity and nephrotoxicity.
Declaration of Interest
The authors report no conflicts of interest. The authors alone are responsible for the content and writing of the paper.
References
- Ascioglu, S., Rex, J. H., de Pauw, B., Bennett, J. E., Bille, J., Crokaert, F., Denning, D. W., Donnelly, J. P., Edwards, J. E., Erjavec, Z., Fiere, D., Lortholary, O., Maertens, J., Meis, J. F., Patterson, T. F., Ritter, J., Selleslag, D., Shah, P. M., Stevens, D. A., Walsh, T. J. (2002). Defining opportunistic invasive fungal infections in immunocompromised patients with cancer and hematopoietic stem cell transplants: An International consensus. Clin Infect Dis. 34:7–14.
- Bakker-Woudenberg, I.A.J.M. (2002). Long-circulating sterically stabilized liposomes as carriers of agents for treatment of infection or for imaging infectious foci. Int J Antimicrobial Agents. 19:299–311.
- Banerjee, R. (2001). Liposomes: Applications in Medicine. J Biomater Appl. 16:3–21.
- Compas, D., Touw, D.J., Goede, P.N. (1996). Rapid method for the analysis of itraconazole and hydroxyitraconazole in serum by high-performance liquid chromatography. J Chrom B Biomed Appl. 687:453–5.
- Filipovic-Grcic, J., Skalko-Basnet, N., Jalsenjak, I. (2001). Mucoadhesive chitosan-coated liposomes: Characteristics and stability. J Microencapsul. 18:3–12.
- Gong, G., Xu, S., Xiang, Q., Duan, X.. (2003). Yeast infection in intensive care units: An analysis and survey. Chin J Nosocomiol. 13:282–3.
- Grant, S., Clissold, S. (1989). ITR: A review of its pharmacodynamic and pharmacokinetic properties and therapeutic use in superficial and systemic mycoses. Drugs. 37:310–4.
- Gregoriadis, G. (1988). Liposomes as drug carriers. Recent trend and progress. New York: John Wiley & Sons.
- Huang, G.H., Zhu, X.L., Zhang, N., Zhang, B.J. (2007). Preparation of lomustine thermo-sensitive liposomes and investigation of anti-tumor activity in vitro. Chin Pharm J. 42:914–8.
- Jain, S., Sehgal, V. (2001). ITR: An effective oral antifungal for onchyomychosis. Int J Dermatol. 40:1–5.
- Kauffman, C.A., Carver, P.L. (1997). Antifungal agents in the 1990s: Current status and future development. Drugs. 53:539–49.
- Khatri, J., Qassim, S., Abed, O., Abraham, B., Al-Lami, A., Masood, S. (2001). A novel extractionless HPLC fluorescence method for the determination of glyburide in the human plasma:application to a bioequivalence study. J Pharm Sci. 4:201–3.
- Kim, J.K., Park, J.S., Kim, C.K. (2010). Development of a binary lipid nanoparticles formulation of itraconazole for parenteral administration and controlled release. Int J Pharm. 383:209–15.
- Muzzarelli, R.A.A. (1988). Carboxymethylated chitins and chitosans. Carbohydr Polym. 8:l–21.
- Takeuchi, H., Yamamoto, H., Nina, T., Hino, T., Kawashlma, Y. (1994). Mucoadhesion of polymer-coated liposomes to rat intestine in vitro. Chem Pharm Bull. 42:1954–6.
- Peeters, J., Neeskens, P., Tollenaere, J.P., Van Remoortere, P., Brewster, M.E. (2002). Characterization of the interaction of 2-hydroxypropyl-beta-cyclodextrin with itraconazole at pH 2, 4 and 7. J Pharm Sci. 91:1414–22.
- Walsh PM. (2003). Physicians’ desk reference[M], 57ed, Montvale NJ: Thomson PDR, 1794–1797.
- Wayne, P.A. (1997). National Committee for Clinical Laboratory Standards, reference method for broth dilution antifungal susceptibility testing of yeasts. Approved standard. NCCLS document M27-A. Wayne, Pennsylvania National Committee for Clinical Laboratory Standards.
- Wu, X.Z., Cheng, A.X., Sun, L.M., Sun, S.J., Lou, H.X. (2009). Plagiochin E, an antifungal bis(bibenzyl), exerts its antifungal activity through mitochondrial dysfunction-induced reactive oxygen species accumulation in Candida albicans. Biochim Biophys Acta. 1790:770–7.
- Xu, J., Yang, Zh Q., Pan, P., Zhang, X.N., Wu, H.S.H. (2008). Studies on preparation and releasing of cyclosporin a liposome in vitro. Ant Inf Pharm. 5:222–5.
- Yi, Y., Yoon, H.J., Kim, B.O., Shim, M., Kim, S., Hwang, S., Seo, M.H. (2007). A mixed polymeric micellar for mulation of itraconazole.Characteristics, toxicity and phammcokinetics[J]. J Contr Rel. 117:59–67.
- Zhang, X., Hou, X. (2003). Comparative efficacy and distribution of Evans blue liposome modified with DSPE 2PEG, Tween80, and Brij35. J Chin Pharm Sci. 12:71–5.